DOI:
10.1039/D0FO01145B
(Paper)
Food Funct., 2020,
11, 5933-5943
Tea polyphenols as a strategy to control starch digestion in bread: the effects of polyphenol type and gluten†
Received
4th May 2020
, Accepted 31st May 2020
First published on 1st June 2020
Abstract
The inhibitory effect of tea polyphenols on starch digestibility can contribute to the control of the glycaemic index of starchy food. In this study, wheat bread and gluten-free bread were co-digested in vitro with different amounts of tea polyphenols. The kinetics of starch digestion and polyphenol bio-accessibility during in vitro digestion were monitored. The results showed that co-digestion of bread with tea polyphenols dose-dependently slowed the starch digestion kinetics and this effect is influenced by the types of polyphenols and the presence of gluten. The presence of gluten lowered the inhibitory efficacy of tannins on starch digestibility to 7.4% and 47.5% when 25 mg of tannins were co-digested with wheat bread and gluten-free bread, respectively. In contrast, the presence of gluten had little impact on the inhibitory efficacy of monomeric polyphenols. This study shows that the release of tea polyphenols in the digestive environment is a promising strategy for controlling the glycaemic index of starchy food and that monomeric and polymeric tea polyphenols differently affect starch digestion according to the presence of gluten.
1. Introduction
The number of people affected by type 2 diabetes is growing worldwide.1 The control of the postprandial glucose level is critical for diabetic patients. This could be achieved by the inhibition of α-amylase and α-glucosidase, the two key digestive enzymes that convert starch to glucose.2 Some antidiabetic drugs, such as acarbose, miglitol, and voglibose can substantially inhibit α-amylase and α-glucosidase, but they often cause side effects.3 However, studies report that the inhibition of starch digestive enzymes can also be achieved, to certain extent, by compounds present in our diet, such as polyphenols.4
Among polyphenols, tea polyphenols have received considerable attention because of their health benefits like anti-inflammatory and anti-oxidant effects as well as management of diabetes.5 Green tea and black tea are two of the most popular hot beverages in the world. Green tea is non-fermented tea in which the most abundant polyphenol is epigallocatechin gallate (EGCG).2 Black tea is fermented tea, and its distinctive polyphenols are theaflavins and thearubigins.6 Theaflavins have benzotropolone structures and therefore give a red colour to a black tea infusion and thearubigins are polymeric polyphenols.7
Previously, the inhibitory effect of tea polyphenols on digestive enzymes has been characterized by determination of the half inhibition concentration (IC50), kinetics of inhibition, and fluorescence quenching to further explain the inhibition type and mechanism.8 Most studies tested the inhibition in a simple reaction system (a simple mixture of a digestive enzyme and a substrate), ignoring the influence of the interactions among polyphenols, digestive enzymes, starch and other food components on starch digestibility.
In this study, wheat bread and gluten-free bread were chosen as model starchy foods. Green tea and black tea were chosen as sources of polyphenols. Wheat bread and gluten-free bread were co-digested with tea extract to simulate a meal having bread and tea simultaneously. The kinetics of starch digestion and polyphenol bio-accessibility during in vitro digestion were monitored. Then, the influence of gluten on the inhibitory efficacy of polyphenols on starch digestibility and bio-accessibility of polyphenols was discussed. To further explain the different behaviours of monomeric polyphenols (catechins) and polymeric polyphenols (tannins), polymeric fractions were separated from black tea extract and were investigated for their impact on starch digestibility. Finally, the breakdown of the bread matrix after co-digestion with polymeric fractions was investigated.
2. Materials and methods
2.1 Materials
Green tea, black tea, wheat flour (11.4% moisture, 73% starch, 11% gluten, 1.6% fat and 2.2% fibre), gluten-free flour (AmiFoods, 12.5% moisture, 86% wheat starch, 0.21% protein, 0.02% fat and 0.05% fibre), and dry yeast were purchased from the local supermarket.
Polyphenol standards including catechin (C), epicatechin (EC), epigallocatechin (EGC), gallocatechin (GC), catechin gallate (CG), epicatechin gallate (ECG), gallocatechin gallate (GCG), epigallocatechin gallate (EGCG), theaflavins (TF1), theaflavin-3-gallate (TF2A), theaflavins-3′-gallate (TF2B), theaflavins-3,3′-gallate (TF3), pepsin (4268 units mg−1), porcine pancreatin (P7545; 4XUSP specifications; amylase activity 40 units mg−1), amyloglucosidase (P300 units mL−1), porcine α-amylase (A4268, 1096 units mg−1), wheat gluten, bovine serum albumin (BSA), sodium dodecyl sulfate (SDS), triethanolamine (TEA) and ferric chloride were purchased from Sigma-Aldrich (St Louis, MO, USA). Trifluoroacetic acid, acetonitrile, methanol and absolute ethanol were of HPLC grade. All other chemicals were of analytical grade.
2.2 Experimental design
In this study, wheat bread and gluten-free bread were co-digested with different amounts of green tea extract, black tea extract and polymeric fractions from black tea extract. Starch digestibility, bio-accessibility of polyphenols, the interactions of polyphenols with nutrients and the breakdown of the bread matrix during digestion were investigated.
2.3. Tea extract preparation and separation of the polymeric fractions
The extract of tea polyphenols was prepared according to a previous method with some modifications.9 Ten grams of green tea and black tea samples were extracted with 100 mL of absolute methanol for 30 min using ultrasound equipment (Sonication, China) at room temperature. The mixture was centrifuged (4000g, 15 min) and the residue was re-extracted twice. The supernatants were combined and the tea extract powder was obtained using a freeze dryer (Alpha 2-4 LD plus, Christ). The green tea extract (GTE) and the black tea extract (BTE) powder were stored at −20 °C until analysis. The yields of GTE and BTE were calculated and those values were used for calculating the corresponding amount of tea cups.
The separation of polymeric fractions from BTE was performed according to a previous method with some modifications.10 Briefly, 1 g of BTE was dissolved in a 5% aqueous ethanol solution (ethanol
:
water = 5
:
95, v/v). Then 100 mL of ethyl acetate was added to extract the organic fraction. The organic fraction containing monomeric and oligomeric fractions was discarded. The aqueous fraction containing polymeric tannins was collected and concentrated using a rotary evaporator at 40 °C. Finally, the powder of polymeric fractions was obtained using a freeze dryer (Alpha 2-4 LD plus, Christ) and stored at −20 °C.
2.4 Polyphenol characterization
2.4.1 HPLC-DAD.
HPLC-DAD was used for analysing the catechin composition of tea polyphenol extract according to a previous method with some modifications.11 HPLC analysis was performed using an HPLC system equipped with a diode array detector. A Varian Polaris 5 C18-A (4.6 × 150 mm) column was used for the separation of tea polyphenols. The UV wavelength was set in the range of 200–400 nm. The UV detecting channel was set at 290 nm. The gradient elution was composed of solvent A (water, pH = 2.5 adjusted by trifluoroacetic acid) and solvent B (absolute acetonitrile). The flow rate was 1 mL min−1. The mobile phase composition started from 100% solvent A to 58% solvent A within 20 min and was kept at 58% solvent A for 5 min. Finally the mobile phase composition was brought back to its initial conditions in 1 min for the next run. All the tea catechin and theaflavin standards were used to make calibrations. The tea polyphenol content in the TEs (tea extracts) was expressed as mg per gram of TE.
2.4.2 BSA precipitation.
The tannin content of TEs (tea extracts) was measured by the BSA precipitation method.12 Briefly, 0.5 mL of the sample was mixed with 1.5 mL of acetic acid/NaCl buffer (200 mM acetic acid and 170 mM NaCl, pH = 4.9) containing BSA (1 mg mL−1). The mixture was incubated at room temperature with slow agitation. After 15 min, the mixture was centrifuged to pellet the protein–tannin precipitate (13
000g, 5 min). The pellet was washed with acetic acid/NaCl buffer and then dissolved in a buffer containing 5% TEA (v/v) and 5% SDS (w/v). For tannin analysis, 875 μL of the dissolved sample was mixed with 125 μL of ferric chloride reagent (10 mm ferric chloride reagent in 10 mm HCl). The mixture was incubated at room temperature for 10 min. The absorbance was measured at 510 nm. The catechin standard was used for calibration. The tannin content was expressed as mg catechin equivalents per gram of sample.
2.5 α-Amylase inhibition assay
The inhibition of α-amylase was performed according to a previous method.9 Two types of α-amylases were used in this study: porcine pancreatic α-amylase and porcine pancreatin. The α-amylase activity of these two enzymes are 1096 units mL−1 and 40 units mg−1, respectively. The two enzymes were dissolved in phosphate buffer to give an α-amylase activity of 400 U mL−1. Briefly, 400 μL of methanol or TE solution was mixed with 200 μL of starch and incubated at 25 °C for 10 min, and finally 200 μL of enzyme was added to start the reaction. The final concentrations of the TEs in the reaction solution were 0.025, 0.125, 0.25, 1.25, 2.5, 5, 12.5 and 25 mg mL−1. After incubated at 25 °C for 3 min, 400 μL of 96 mM dinitrosalicylic acid reagent was added and the mixture was placed in a boiling water bath for 5 min. After cooling down, 4 mL of water was added before the absorbance was measured at 540 nm using a microplate reader. Finally the α-amylase inhibitory effect was expressed as IC50 (mg mL−1), which is defined as the concentration of TEs in the reaction solution required to inhibit 50% of the enzyme activity.
2.6 Bread preparation
Normal wheat bread and gluten-free bread were prepared by mixing 350 g of wheat flour or gluten-free flour, 220 mL of water and 7 g of yeast. No additional ingredients were added. All of the ingredients were added to a baking machine (Philips, HD 9020) and breads were made using a standard program (baking temperature: 120 °C and baking time: 15 min). All the bread samples were dried using a freeze dryer (Alpha 2-4 LD plus, Christ). Then the dried samples were sieved through 2 sieves (315 and 125 μm) to make sure the particle size of the obtained samples is in the range of 125–315 μm. The sieved bread samples were stored at −20 °C for further analysis.
2.7 The hydration properties of bread samples
The hydration properties of bread samples were determined according to the previous method.13 The water holding capacity (WHC) is the amount of water retained by the bread sample. Briefly, 1 g of dried bread sample was mixed with 10 mL of water and kept at room temperature for 24 h. Then the supernatant was removed gently. The WHC was expressed as grams of water retained per gram of bread (g g−1). The swelling volume (SV) was calculated by dividing the total swollen volume by the initial weight of the sample. Briefly, 1 g of dried bread sample was placed in a graduated cylinder and mixed with 10 mL of distilled water. After 24 h, the volume of the swollen sample was measured. The SV was expressed as the volume of the swollen sample per gram of the initial sample (mL g−1).
2.8
In vitro digestion
2.8.1
In vitro digestion model.
Co-digestion of wheat bread with different amounts of TEs was performed in this study. Firstly 2.5 grams of dried wheat bread was hydrated with 2.5 mL of water. Then 5 g of the mixture was mixed with 0, 50, 125, 250, 500, and 1000 mg of TEs and marked as controls, 1, 2.5, 5, 10 and 20% co-digestion. Co-digestion of gluten-free bread with 5% TEs was carried out, i.e. 5 g of hydrated gluten-free bread was mixed with 250 mg of TEs. Finally co-digestion of wheat bread and gluten-free bread with 1, 2.5 and 5% polymeric fractions from BTE were performed, i.e., 5 g of hydrated wheat bread and gluten-free bread were mixed with 50, 125 and 250 mg of polymeric fractions from BTE, respectively.
A standard in vitro simulated process was used in this study which was modified for the amount of α-amylase.14 Briefly, the sample was initially treated with simulated gastric fluids and pepsin. The pH of the mixture was adjusted to 3 and incubated at 37 °C with agitation for 2 h. Then, simulated intestinal fluids and pancreatin were added to the mixture to give a final α-amylase activity of 200 U mL−1 and the corresponding trypsin activity was 15 U mL−1. Then the pH was adjusted to 7. The mixture was incubated at 37 °C with agitation for 2 h. During the intestinal phase, 0.1 mL of the sample was collected at different time points (0, 10, 20, 40, 60, 80, 100, and 120 min). Then 0.4 mL of absolute ethanol was added to stop the reaction and the mixture was centrifuged at 13
000g for 10 min. Finally, 2 mL of amyloglucosidase (27.16 U mL−1) was added and incubated at 37 °C for an extra hour to complete starch digestion.
2.8.2 Determination of the percentage of digested starch.
The GOPOD kit was used for glucose measurement. Digested starch = released glucose/0.9. The initial amount of total starch in bread was measured by using the total starch assay kit. The results were expressed as the percentage of digested starch (% of digested starch = digested starch/initial amount of starch). A first-order kinetics model was applied to describe the kinetics of glucose release from starch digestion.15 | Ct − C0 = C∞(1 − e−kt) | (1) |
where Ct, C0 and C∞ correspond to the percentage of digested starch at time t, 0 and infinite time, respectively and k is the kinetic constant. Parameter estimation was performed using the solver of Excel software by minimizing the sum of square values.
2.9 The bio-accessibility of tea polyphenols
The bio-accessibility of tea polyphenols after gastric phase and intestinal digestion was measured according to a previous method with some modifications.16 Gastric supernatant and intestinal supernatant were collected at the time points 0 and 120 min, respectively (see section 2.7). The amount of polyphenols from both supernatants was measured as described in section 2.3. The bio-accessibility of polyphenols was expressed as the percentage of polyphenols in the supernatant compared to the initial amount in TEs.
2.10 The interactions between tea polyphenols and bread components
The interactions among tea polyphenols, digestive enzymes and bread nutrients (gluten and starch) were investigated. Briefly, 250 mg of TEs was mixed with digestive fluids as a control. Then digestive enzymes (pepsin and pancreatin), wheat bread, gelatinized starch and gluten were added separately. All of the chemicals were added the same amount as described in section 2.7. Gelatinized starch (1.8 g) and gluten (0.3 g) were added considering the amount present in 5 g of hydrated bread. After 2 min, the mixture was centrifuged (4000g, 15 min), and the amount of polyphenols in the supernatant was measured as described in section 2.3. The results were expressed as the percentage of polyphenols in the supernatant compared with the initial amount in TEs.
2.11 The breakdown of wheat bread and gluten-free bread during digestion
Size distribution was analysed according to a previous method.13 Intestinal digested wheat bread and gluten-free bread after co-digestion with different amounts of polymeric fractions from BTE were collected for size distribution analysis. The particle size distribution was analysed using the Mastersizer 3000 (Malvern Instruments, Worcestershire UK). D[3, 2] (surface weight mean diameter), D[4, 3] (volume-weight mean diameter) and D (50) (volume median diameter) values were obtained.
2.12 Statistical analysis
All analyses were performed in triplicate. Bread preparation and in vitro digestion experiments were repeated twice. The results were expressed as mean ± standard deviation (SD). One-way analysis of variance (ANOVA) followed by Duncan's multiple range test was used to compare the mean values of different groups using the SAS 9.4 software (SAS Institute Inc., Cary, NC, USA). Differences were considered significant at P < 0.05.
3. Results
3.1 Polyphenol composition and α-amylase inhibition
Table 1 shows the polyphenol composition of BTE and GTE. Four flavan-3-ols (EGC, EC, EGCG, and ECG) and 3 theaflavins (theaflavin, theaflavin-3-gallate, and theaflavin-3′-gallate) were detected in BTE. In total, 410 mg g−1 of catechins, 43 mg g−1 of theaflavins and 50 mg g−1 of tannins were found in BTE. GTE showed a less diverse polyphenol profile. In total, 388 mg g−1 of flavan-3-ols was quantified, and EGCG was the major catechin in GTE. No theaflavin or tannins were found in GTE.
Table 1 Polyphenol composition of green tea and black tea extracts and polymeric fractions from BTE and their IC50 values of α-amylase inhibition
Polyphenols |
GTE (mg g−1) |
BTE (mg g−1) |
Polymeric fraction from BTE (mg g−1) |
Caffeine |
89 ± 1 |
72 ± 2 |
nd |
Epigallocatechin (EGC) |
nd |
179 ± 8 |
nd |
Epicatechin (EC) |
73 ± 2 |
116 ± 1 |
nd |
Epigallocatechin gallate (EGCG) |
276 ± 3 |
78 ± 1 |
nd |
Epicatechin gallate (ECG) |
39 ± 1 |
37 ± 0 |
nd |
Theaflavin |
nd |
16 ± 0 |
nd |
Theaflavin-3-gallate |
nd |
9 ± 0 |
nd |
Theaflavin-3′-gallate |
nd |
18 ± 0 |
nd |
Tannins |
nd |
50 ± 1 |
100 ± 1 |
Total amount of monomeric polyphenols |
388 ± 1 |
453 ± 9 |
nd |
Total amount of polyphenols |
388 ± 1 |
503 ± 10 |
100 ± 1 |
|
Enzyme |
GTE (mg mL−1) |
BTE (mg mL−1) |
Polymeric fraction from BTE (mg mL−1) |
The values were expressed as mean ± standard deviation. BTE, black tea extract; GTE, green tea extract. nd: not detectable. Total amount of monomeric polyphenols means the sum of all catechins and theflavins. Total amount of polyphenols means the sum of all catechins, theaflavins and tannins. IC50 values mean the concentration of the extract (mg mL−1) in the final reaction solution required to inhibit 50% of the α-amylase activity. |
IC50 |
Pancreatin |
16.4 ± 0.0 |
26.6 ± 1.6 |
nd |
α-Amylase |
1.6 ± 0.2 |
1.9 ± 0.3 |
2.5 ± 0.3 |
The inhibitory effect of TEs on α-amylase was measured as reported in Table 1 and Fig. S1.† Both TEs showed strong inhibition of α-amylase, as indicated by low IC50 values, i.e., 1.6 and 1.9 mg mL−1 for GTE and BTE, respectively. Both TEs showed much lower inhibition of α-amylase when pancreatin was used as the source of α-amylase, as indicated by high IC50 values, i.e. 16.4 and 26.6 mg mL−1 for GTE and BTE, respectively.
3.2 The starch digestibility of wheat bread and gluten-free bread
3.2.1 The starch digestibility of wheat bread.
Fig. 1A and B shows the starch digestibility curve of wheat bread when co-digested with TEs. The starch digestibility of wheat bread was fitted to a first order kinetics model and the kinetic parameters are shown in Table 2. A clear dose-dependent inhibition was observed when wheat bread was co-digested with different amounts of TEs (Fig. 1A and B). As shown in Table 2, a reduction of 23.3%, 31.4% and 66% was found when wheat bread was co-digested with 250, 500 and 1000 mg of BTE, respectively. Similar inhibition was found when wheat bread was co-digested with GTE compared with the same amount of BTE. The initial rate of starch digestion was also clearly reduced by co-digestion of wheat bread with different amounts of TEs (Table 2). Fig. 1C shows the starch digestibility curve of wheat bread when co-digested with different amounts of polymeric fractions from BTE. As shown in Table 2, a reduction of 1.9%, 5.2% and 7.4% was observed when wheat bread was co-digested with 50, 125 and 250 mg of polymeric fractions from BTE, respectively and a clear reduction can also be observed from the starch digestibility curve, as shown in Fig. 1C.
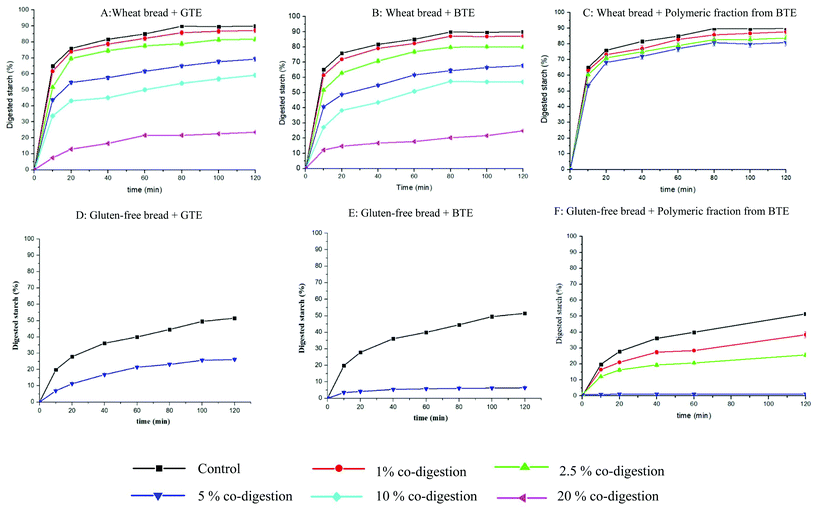 |
| Fig. 1
In vitro starch hydrolysis profiles of wheat bread and gluten-free bread co-digested with different amounts of BTE, GTE and polymeric fractions from BTE. The present data were expressed as mean ± standard deviation (n = 3). The statistical analysis of the data is shown in Table 2. Note: 5 g of hydrated wheat bread and gluten-free bread were mixed with 0, 50, 125, 250, 500, and 1000 mg of GTE (A & D), BTE (B & E) or polymeric fractions from BTE (C & F) and marked as controls, 1, 2.5, 5, 10 and 20% co-digestion. | |
Table 2 Estimated kinetic parameters for starch digestion obtained from in vitro digestion of wheat bread co-digested with different amounts of TEs and polymeric fractions from BTE
Wheat bread GTE co-digestion |
Control (wheat bread) |
1% GTE (50 mg) |
2.5% GTE (125 mg) |
5% GTE (250 mg) |
10% GTE (500 mg) |
20% GTE (1000 mg) |
k (min−1) |
0.13 ± 0.00 a |
0.12 ± 0.00 b |
0.10 ± 0.00 c |
0.10 ± 0.00 c |
0.08 ± 0.00 d |
0.04 ± 0.00 e |
C
∞ (%) |
87.0 ± 0.4 a |
84.0 ± 1.3 b |
79.2 ± 1.3 c |
64.5 ± 1.2 d |
53.8 ± 1.1 e |
23.3 ± 0.5 f |
% * min−1 |
10.9 ± 0.3 a |
10.4 ± 0.2 a |
8.2 ± 0.1 b |
6.6 ± 0.1 c |
4.5 ± 0.1 d |
0.9 ± 0.0 e |
Sum of square |
75.4 ± 9.9 |
58.6 ± 1.8 |
25.1 ± 0.8 |
81.9 ± 2.7 |
107.1 ± 4.4 |
4.0 ± 0.2 |
Wheat bread BTE co-digestion |
Control (wheat bread) |
1% BTE (50 mg) |
2.5% BTE (125 mg) |
5% BTE (250 mg) |
10% BTE (500 mg) |
20% BTE (1000 mg) |
k (min−1) |
0.13 ± 0.00 a |
0.11 ± 0.00 b |
0.10 ± 0.00 c |
0.08 ± 0.00 d |
0.05 ± 0.00 f |
0.06 ± 0.00 e |
C
∞ (%) |
87.0 ± 0.4 a |
84.3 ± 0.4 b |
77.8 ± 0.1 c |
63.7 ± 0.8 d |
55.6 ± 0.3 e |
21.0 ± 0.7 f |
% * min−1 |
10.9 ± 0.3 a |
9.1 ± 0.6 b |
7.4 ± 0.2 c |
5.3 ± 0.0 d |
3.1 ± 0.0 e |
1.3 ± 0.1 f |
Sum of square |
75.4 ± 9.9 |
95.4 ± 28.4 |
74.2 ± 25.4 |
103.0 ± 11.8 |
69.3 ± 9.0 |
35.5 ± 2.2 |
Wheat bread polymeric fraction co-digestion BTE co-digestion |
Control (wheat bread) |
1% polymeric fraction (50 mg) |
2.5% polymeric fraction (125 mg) |
5% polymeric fraction (250 mg) |
k (min−1) |
0.13 ± 0.00 a |
0.13 ± 0.00 a |
0.13 ± 0.00 a |
0.11 ± 0.00 a |
C
∞ (%) |
87.0 ± 0.4 a |
85.1 ± 0.9 b |
81.8 ± 0.8 c |
79.6 ± 0.8 d |
% * min−1 |
10.9 ± 0.3 a |
10.9 ± 0.1 a |
10.5 ± 0.1 a |
8.7 ± 0.1 b |
Sum of square |
75.4 ± 9.9 |
96.0 ± 1.9 |
65.7 ± 1.3 |
49.5 ± 1.0 |
Gluten-free bread polymeric fraction and TEs co-digestion |
Control (gluten-free bread) |
1% polymeric fraction (50 mg) |
2.5% polymeric fraction (125 mg) |
5% polymeric fraction (250 mg) |
5% GTE (250 mg) |
5% BTE (250 mg) |
The values were expressed as mean ± standard deviation. The values followed by different letters in the same row are significantly different (p < 0.05). BTE, black tea extract; GTE, green tea extract. Polyphenol content in GTE: 50, 125, 250, 500, 1000 mg of GTE contained 19.4, 48.5, 97, 194 and 388 mg of monomeric polyphenols. Polyphenol content in BTE: 50, 125, 250, 500, and 1000 mg of BTE contained 22.6, 56.6, 113.2, 226.5 and 453 mg of monomeric polyphenols and 2.5, 6.25, 12.5, 25 and 50 mg of tannins. Polyphenol content in polymeric fraction: 50, 125 and 250 mg of polymeric fractions contained 5, 12.5 and 25 mg of tannins. |
k (min−1) |
0.04 ± 0.00 e |
0.05 ± 0.00 d |
0.06 ± 0.00 c |
0.17 ± 0.00 a |
0.03 ± 0.00 f |
0.07 ± 0.00 b |
C
∞ (%) |
48.6 ± 0.5 a |
34.7 ± 1.6 b |
23.1 ± 1.0 d |
1.1 ± 0.0 f |
27.3 ± 0.3 c |
6.1 ± 0.1 e |
% * min−1 |
1.9 ± 0.0 a |
1.6 ± 0.0 b |
1.4 ± 0.0 c |
0.2 ± 0.0 f |
0.7 ± 0.0 d |
0.4 ± 0.0 e |
Sum of square |
58.5 ± 1.2 |
51.8 ± 18.7 |
17.0 ± 3.1 |
0.0 ± 0.0 |
1.7 ± 0.0 |
0.6 ± 0.0 |
3.2.2 The starch digestibility of gluten-free bread.
Fig. 1D and E shows the starch digestibility curve of gluten-free bread co-digested with 250 mg of TEs. Only 48.6% of starch was digested in gluten-free bread, which is much lower than the starch digestibility of wheat bread (87%), as shown in Table 2. GTE caused a similar reduction in the starch digestibility of wheat bread (22.5%) and gluten-free bread (21.3%). BTE caused a higher reduction in the starch digestibility of gluten-free bread (42.5%) than that in the starch digestibility of wheat bread (23.3%). Fig. 1F shows the starch digestibility curve of gluten-free bread when co-digested with different amounts of polymeric fractions from BTE. A clear dose-dependent reduction in the starch digestibility was observed. Only 1.1% of starch in gluten-free bread was digested when co-digested with 250 mg of polymeric fraction from BTE (Table 2).
3.3 The bio-accessibility of tea polyphenols
3.3.1 The bio-accessibility of tea polyphenols when co-digested with wheat bread.
As shown in Fig. 2 (upper panels), regarding GTE, EC was the most bio-accessible polyphenol after gastric digestion (33–39%), followed by EGCG (8–12%) and ECG (5–9%). After intestinal digestion, more catechins became bio-accessible from GTE, i.e., 76–87% EC, 34–45% EGCG and 31–40% ECG. The bio-accessibility of catechins from BTE was much lower than the bio-accessibility of catechins from GTE. No tannins were released from BTE after gastric and intestinal digestion.
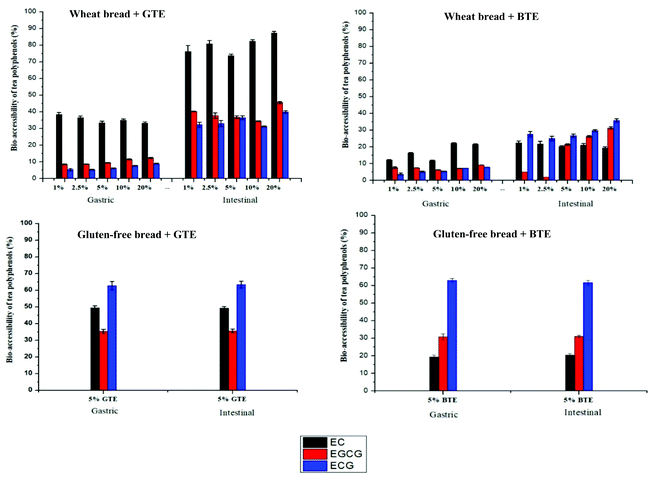 |
| Fig. 2 Bio-accessibility of tea polyphenols after gastric and intestinal digestion when co-digested with wheat bread or gluten-free bread. The results expressed as the percentage of bio-accessible polyphenols at the end of gastric and intestinal digestion compared to the initial amount of polyphenols in TEs. No tannins were detected after gastric and intestinal digestion. The amounts of EGC and theaflavins from BTE were not shown because the peak area is too small for accurate calculation. The present data were expressed as mean ± standard deviation (n = 3). EC, epicatechin; EGCG, epigallocatechin gallate; ECG, epicatechin gallate; and EGC, epigallocatechin. | |
3.3.2 The bio-accessibility of tea polyphenols when co-digested with gluten-free bread.
As shown in Fig. 2 (lower panels), after gastric digestion, the bio-accessibility of tea polyphenols when co-digested with gluten-free bread (Fig. 2, lower panels) was higher than the bio-accessibility of tea polyphenols co-digested with wheat bread (Fig. 2, upper panels). E.g., EGCG was more bio-accessible when GTE was co-digested with gluten-free bread (38%) than when the same amount of GTE was co-digested with wheat bread (10%). The bio-accessibility of polyphenols from BTE showed a similar trend. For gluten-free bread and both TEs, no clear increase in the bio-accessibility of tea polyphenols was observed after intestinal digestion compared with their bio-accessibility after gastric digestion.
3.4 The interactions of tea polyphenols with the bread matrix
Fig. 3 shows the percentage of free tea polyphenols in the supernatant after mixing with digestive enzymes (pepsin and pancreatin), wheat bread, wheat starch (the corresponding amount in wheat bread) and wheat gluten (the corresponding amount in wheat bread). For both TEs, less than 80% catechin was available in the supernatant in the control samples. Regarding BTE, 98% tannins was found in the supernatant in the control sample. When digestive enzymes and wheat bread were separately added to the control sample, 87% and 5% tannins were found in the supernatant, respectively. Catechins from both TEs showed the same trend as tannins, i.e. substantial amount of polyphenols interacted with the bread matrix. To investigate the preferential interaction of tea polyphenols, bread components, starch and gluten were added separately. Surprisingly, a large amount of tea polyphenols interacted with gluten considering its lower content in wheat flour (11%) than starch (73%).
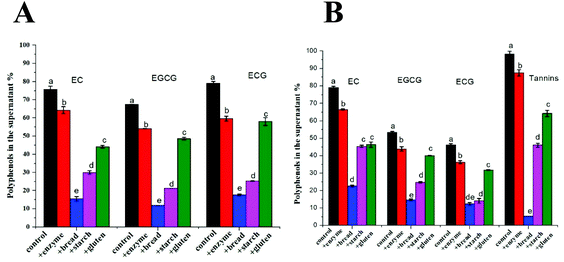 |
| Fig. 3 Relative percentage of polyphenols in the supernatant upon addition of digestive enzymes and bread components (gluten and starch) compared to the initial content in TEs. The TEs were mixed with digestive fluids as a control. Then the mixture was mixed either with digestive enzyme, or with bread, or with starch, or with gluten (the amounts of gluten and starch added correspond to their amounts in the bread). The present data were expressed as mean ± standard deviation (n = 3).The different letters mean significant differences. A: GTE, green tea extract, B: BTE, black tea extract. EC, epicatechin; EGCG, epigallocatechin gallate; ECG, epicatechin gallate. | |
3.5 Bread hydration and the breakdown of the bread matrix during digestion
Gluten-free bread samples showed the lowest water holding capacity (2.0 g g−1) and swelling volume (2.6 mL g−1). The water holding capacity and swelling volume of wheat bread were two times higher than those of gluten-free bread (Table 3).
Table 3 Hydration properties of bread samples and size distribution of the digested samples from co-digestion of wheat bread and gluten free bread with different amounts of polymers
Wheat bread |
Measurement |
Control (wheat bread) |
1% polymeric fraction (50 mg) |
2.5% polymeric fraction (125 mg) |
5% polymeric fraction (250 mg) |
Undigested bread |
WHC (g g−1) |
4.0 ± 0.1 |
na |
na |
na |
SV (mL g−1) |
4.7 ± 0.2 |
na |
na |
na |
Intestinal digested sample |
D
50 (μm) |
41.2 ± 1.2 c |
41.8 ± 0.3 c |
96.3 ± 1.3 b |
200.5 ± 2.5 a |
D[3,2] (μm) |
21.0 ± 1.0 d |
23.5 ± 0.5 c |
31.8 ± 0.3 b |
44.4 ± 0.6 a |
D[4,3] (μm) |
60.2 ± 1.2 d |
64.8 ± 0.3 c |
125.0 ± 2.0 b |
225.5 ± 1.5 a |
Gluten free bread |
Measurement |
Control (gluten-free) |
1% polymeric fraction (50 mg) |
2.5% polymeric fraction (125 MG) |
5% polymeric fraction (250 mg) |
D[50], volume median diameter; D[3,2], surface area moment mean; D[4,3], volume moment mean; the values were expressed as mean ± standard deviation. The values followed by different letters in the same row are significantly different (p < 0.05). |
Undigested bread |
WHC (g g−1) |
2.0 ± 0.1 |
na |
na |
na |
SV (mL g−1) |
2.6 ± 0.1 |
na |
na |
na |
Intestinal digested sample |
D
50 (μm) |
16.2 ± 0.1 c |
16.4 ± 0.0 c |
17.0 ± 0.1 b |
18.2 ± 0.0 a |
D[3,2] (μm) |
13.9 ± 0.0 b |
13.7 ± 0.1 b |
13.9 ± 0.0 b |
14.7 ± 0.1 a |
D[4,3] (μm) |
17.0 ± 0.0 c |
17.5 ± 0.1 b |
17.7 ± 0.1 b |
21.5 ± 0.2 a |
The size distributions of intestinal digested wheat and gluten-free bread when co-digested with different amounts of polymeric fractions from BTE were determined as well (Table 3 and Fig. S2†). The D50 (volume median diameter) values of intestinal digested gluten-free samples (16.2 μm) were much lower than those of intestinal digested wheat bread (41.2 μm). D[3,2], surface area moment mean and D[4,3], volume moment mean showed the same trend as D50. A significant increase in the size distribution of the intestinal digested wheat bread was observed when co-digested with different amounts of polymeric fractions from BTE. E.g., the D50 value of the intestinal digested wheat bread increased from 41.2 to 96.3 and 200.5 μm when co-digested with 125 and 250 mg of polymeric fractions from BTE, respectively. In contrast, only a small increase in the D50 value of the intestinal digested gluten-free bread was observed when co-digested with 250 mg of polymeric fraction from BTE. D[3,2] and D[4,3] showed a similar trend.
4. Discussion
The inhibitory effect of tea polyphenols on starch digestion has been widely reported, but most of the studies focused on the interactions between polyphenols and α-amylase, ignoring the effect of the food matrix on such interactions.3,17–19 In our previous study, we reported that the interaction of berry polyphenols with starch or gluten affected the efficacy of polyphenols on the inhibition of starch digestion.9 Moreover, we highlighted that the interaction of polyphenols with starch may be an additional mechanism for polyphenol inhibition of starch digestion.9 In the present study, we investigated in more detail how the multiple interactions among polyphenols, α-amylase, other digestive enzymes, starch and gluten influence starch digestibility using tea polyphenols as model phenolics and wheat and gluten-free bread as model starchy foods.
Data showed that co-digestion of bread with both black and green tea polyphenols significantly reduced the kinetics rate and extent of starch digestion (Fig. 1 and Table 2). An in vitro study showed that co-digestion with green tea catechins could slow down starch digestion of steamed and baked bread, which was in line with our results.16 In the present study, we observed a trend for the reduction in both k and C∞ when the amount of co-digested polyphenols from black and green tea increased (Fig. 1). Since a significant reduction in the C∞ value may decrease the time needed to achieve C∞ and thus increase the k values as we observed in a previous study,9 the initial rates were also calculated and their values confirmed a dose-dependent decrease of the digestion rate (Table 2).
Monomeric and polymeric polyphenols showed different inhibition degrees on starch digestibility of wheat bread and gluten-free bread. It is interesting to notice that GTE caused a similar reduction in the starch digestibility of both wheat bread (Fig. 1A) and gluten-free bread (Fig. 1D), but BTE caused a larger reduction in the starch digestibility of gluten-free bread (Fig. 1E) than in the starch digestibility of wheat bread (Fig. 1B). Considering the different polyphenol profiles in BTE and GTE (Table 1), we proposed that polymeric polyphenols from BTE are responsible for the different BTE inhibition efficacies when co-digested with wheat bread and gluten-free bread. It has been already proposed that polymeric polyphenols may be more effective in reducing the rate and the extent of starch digestion than monomers. In one study, a sorghum extract rich in tannins increased the resistant starch content in normal corn starch by around two times compared to an extract with monomeric polyphenols.20 To better understand the role of the polymeric fraction in the inhibition of starch digestion, the polymeric fraction was separated from BTE for testing its inhibitory effect on starch digestion. Surprisingly, the polymeric fraction was more effective than the whole BTE extract in gluten-free bread but much less effective in wheat bread (Fig. 1). By comparing the polyphenol composition and inhibitory efficacy of the whole BTE and polymeric fraction, we could quantify the relative contribution of the monomeric and polymeric fractions to the observed inhibition. In wheat bread, the contribution of the monomeric and polymeric fractions to the reduction of the starch digestibility caused by 250 mg of BTE (23.3%) was quantified as 18.1% and 5.2% respectively. In gluten-free bread, the contribution of the monomeric and polymeric fractions to the reduction of the starch digestibility caused by 250 mg of BTE (42.5%) was quantified as 17% and 25.5%, respectively. We concluded that the presence of gluten had a minor influence on the inhibitory efficacy of monomeric polyphenols, since the monomeric polyphenols caused a similar reduction in the starch digestibility of wheat bread (18.1%) and gluten-free bread (17%) when they were co-digested with BTE. This is in line with the fact that GTE has similar inhibitory efficacy in wheat bread (22.5%) and gluten-free bread (21.3%), as shown in Table 2, since GTE only contained monomeric polyphenols (Table 1). However, the presence of gluten clearly reduced the inhibitory efficacy of polymeric polyphenols, since tannins caused a much larger reduction in the starch digestibility of gluten-free bread (25.5%) than that in the starch digestibility of wheat bread (5.2%) when both bread samples were co-digested with 250 mg of BTE. We can also conclude that the presence of the gluten network is key to understand the inhibitory efficacy of polymeric polyphenols on starch digestibility of a complex food matrix like bread.
Regardless of whether polyphenols are monomeric or polymeric, their inhibitory effects on starch digestibility of bread can be explained by multiple mechanisms involving the interactions among tea polyphenols, α-amylase, other digestive enzymes, starch and gluten. The occurrence of those interactions is confirmed by the bio-accessibility data reported in Fig. 2 and 3. The first mechanism is a direct inhibition of α-amylase. We initially hypothesized that this inhibition would be proportional to the amount of polyphenols that are not bound components other than α-amylase. One of those components is the pancreatic enzymes. Some researchers reported that polyphenol-rich extracts from green tea and black tea competitively inhibit pancreatic α-amylase with IC50 values of 0.20 and 0.46 mg mL−1 for GTE and BTE, respectively, and the inhibition was likely attributed to the galloyl moiety, a common substituent in tea polyphenols.4 Although a lot of research has been carried out on α-amylase inhibition,19,21 most studies used isolated pancreatic α-amylase rather than the whole pancreatin. Pancreatin is a mixture of α-amylase, trypsin, chymotrypsin, lipase and colipase and is frequently used for the in vitro digestion study. Therefore, the interaction between polyphenols and other digestive enzymes is usually neglected. For better understanding the role of other enzymes in α-amylase inhibition during in vitro digestion, the IC50 values were calculated for the whole pancreatin and purified α-amylase. As shown in Table 1 and Fig. S1,† higher IC50 values were calculated for pancreatin for both TEs. This was likely because the other enzymes from pancreatin could also interact with polyphenols, thus affecting the polyphenol–amylase interaction.22 The other components to interact with polyphenols are represented by the food matrix components. Previous studies reported that polyphenols have high affinity for proteins and bond to them via hydrophobic interactions and hydrogen bonds.23 Similarly to what has been reported in our previous study with berry polyphenols (mostly anthocyanins and procyanidins), we observed here a strong interaction between tea polyphenols and gluten.9 However, the amount of polyphenols bound to starch was even higher, confirming that in a relatively rich source of starch like bread, the contribution of starch in binding polyphenols may be substantially higher than that of gluten. However, when the supernatant of the samples after gastric digestion was tested for α-amylase inhibition, no such inhibition was found, suggesting that the role of unbound polyphenols in the observed decrease in starch digestibility is negligible.
The second mechanism for the inhibitory effect of polyphenols on starch digestion may derive from the direct interaction between tea polyphenols and starch. It was reported that polyphenols can interact directly with starch through hydrophobic forces and hydrogen bonding, thus reducing the available surface of starch granules to react with enzymes.24 The authors in particular speculated that the presence of polyphenols would block the access of amylase to pores and channels that are typical of cereal starches. This may have happened also in our bread system. In this case we would expect that the presence of gluten would reduce the efficacy of inhibition as Fig. 1D and E seem to suggest. However, it has been reported that polyphenols that are adsorbed onto starch may exert an inhibitory activity against α-amylase.25 It is difficult to determine experimentally but the inhibition of amylase by polyphenols bound to starch can be tested by measuring the inhibition of amylase in the bread matrix. Clearly, the presence of the gluten matrix may reduce the amount of polyphenols able to interact with starch and thus able to inhibit starch digestion whatever the exact mechanism is in place in close proximity to the glucan chains. Moreover, we cannot exclude that the observed inhibition is partly caused by an indirect effect on the extent of gluten digestion, which would make starch granules more accessible to amylase. It is known that polyphenols can reduce the rate and extent of protein digestion.18,26–28 This seems to be confirmed by the particle size distribution data of bread during digestion, where bigger particles are detected in bread co-digested with polyphenols, suggesting that the whole bread is digested at a slower rate in these systems (Table 3). It is also interesting to notice that the starch digestibility of gluten free bread (45%) is much lower than that of wheat bread (87%). Opposite results were reported in other studies in which the presence of gluten slowed down starch digestion.29 The finding of this study could be attributed to the much weaker hydration properties of gluten free bread compared to wheat bread (Table 3), which might hinder in vitro starch digestion.13,30,31
Although tea polyphenols can inhibit starch digestion, to achieve a level of inhibition that is physiologically relevant, we considered how realistically the effective amounts reported in this study may be achieved in real life through tea consumption (Table 4). To achieve an inhibitory effect from co-digestion of 100 g of bread and 1 g of TEs, 2 cups of black tea and 2.5 cups of green tea would be needed to be co-digested with 100 g of wheat bread, as shown in Table 4. Higher amounts of tea polyphenols for co-digestion with bread would be hard to achieve through realistic tea consumption habits.
Table 4 Actual amount of tea cups required to achieve the inhibitory effect of BTE or GTE co-digested with 100 g of wheat bread
Co-digestion |
Required tea extract (g) |
Yield of tea extract (%) |
Required tea powder (g) |
Required tea (cups) |
Black tea |
Green tea |
Black tea |
Green tea |
Black tea |
Green tea |
One cup of tea contained 2 g of tea powder (this information was provided by the tea manufacturer). |
1% |
1 |
|
|
4 |
5 |
2 |
2.5 |
2.5% |
2.5 |
|
|
10 |
12.5 |
5 |
6.3 |
5% |
5 |
25 |
20 |
20 |
25 |
10 |
12.5 |
10% |
10 |
|
|
40 |
50 |
20 |
25 |
20% |
20 |
|
|
80 |
100 |
40 |
50 |
5. Conclusion
In this study, the effects of tea polyphenols on starch digestibility of wheat bread and gluten-free bread were investigated. The inhibitory effect of tea polyphenols on starch digestibility was attributed to the multiple interactions among tea polyphenols, α-amylase, other digestive enzymes, starch and gluten. The use of a gluten free bread made almost exclusively of wheat starch allowed us to prove that the presence of gluten had little influence on the inhibitory efficacy of monomeric polyphenols on starch digestibility, but clearly reduced the inhibitory efficacy of polymeric polyphenols on starch digestibility. Future investigation should elucidate the exact mechanism behind the observed decrease in the starch digestibility in the presence of polyphenols. Data showed that the presence of tea polyphenols in the digestive medium is a promising strategy for controlling the glycaemic index of starchy food products but this strategy must be adapted to the specific food matrix considered.
Conflicts of interest
There are no conflicts of interest to declare.
Acknowledgements
The authors would like to thank our technicians Geert Meijer and Erik Meulenbroeks for their assistance in the lab work. The author Lijiao Kan received a PhD scholarship from the China Scholarship Council (No. 201706820018).
References
- R. Moradi-Marjaneh, M. Paseban and A. Sahebkar, Natural products with SGLT2 inhibitory activity: Possibilities of application for the treatment of diabetes, Phyther. Res., 2019, 33, 2518–2530 CrossRef CAS PubMed.
- S. C. Forester, Y. Gu and J. D. Lambert, Inhibition of starch digestion by the green tea polyphenol, (-)-epigallocatechin-3-gallate, Mol. Nutr. Food Res., 2012, 56, 1647–1654 CrossRef CAS PubMed.
- A. Pyner, H. Nyambe-Silavwe and G. Williamson, Inhibition of human and rat sucrase and maltase activities to assess antiglycemic potential: Optimization of the assay using acarbose and polyphenols, J. Agric. Food Chem., 2017, 65, 8643–8651 CrossRef CAS PubMed.
- L. Sun, F. J. Warren, G. Netzel and M. J. Gidley, 3 or 3′-Galloyl substitution plays an important role in association of catechins and theaflavins with porcine pancreatic α-amylase: The kinetics of inhibition of α-amylase by tea polyphenols, J. Funct. Foods, 2016, 26, 144–156 CrossRef CAS.
- L. Xing, H. Zhang, R. Qi, R. Tsao and Y. Mine, Recent advances in the understanding of the health benefits and molecular mechanisms associated with green tea polyphenols, J. Agric. Food Chem., 2019, 67, 1029–1043 CrossRef CAS.
- G. Pereira-Caro, J. M. Moreno-Rojas, N. Brindani, D. Del Rio, M. E. J. Lean, Y. Hara and A. Crozier, Bioavailability of Black Tea Theaflavins: Absorption, Metabolism, and Colonic Catabolism, J. Agric. Food Chem., 2017, 65, 5365–5374 CrossRef CAS PubMed.
- S. Zhang, C. Yang, E. Idehen, L. Shi, L. Lv and S. Sang, Novel Theaflavin-Type Chlorogenic Acid Derivatives Identified in Black Tea, J. Agric. Food Chem., 2018, 66, 3402–3407 CrossRef CAS PubMed.
- L. Striegel, B. Kang, S. J. Pilkenton, M. Rychlik and E. Apostolidis, Effect of black tea and black tea pomace polyphenols on α-glucosidase and α-amylase inhibition, relevant to type 2 diabetes prevention, Front. Nutr., 2015, 2, 1–6 Search PubMed.
- L. Kan, T. Oliviero, R. Verkerk, V. Fogliano and E. Capuano, Interaction of bread and berry polyphenols affects starch digestibility and polyphenols bio-accessibility, J. Funct. Foods, 2020, 68, 103924 CrossRef CAS.
- M. Kyraleou, S. Kallithraka, S. Koundouras, K. Chira, S. Haroutounian, H. Spinthiropoulou and Y. Kotseridis, Effect of vine training system on the phenolic composition of red grapes (Vitis vinifera L. cv. Xinomavro), OENO One, 2015, 49, 71 CrossRef CAS.
- L. Kan, S. Nie, J. Hu, S. Wang, Z. Bai, J. Wang, Y. Zhou, J. Jiang, Q. Zeng and K. Song, Comparative study on the chemical composition, anthocyanins, tocopherols and carotenoids of selected legumes, Food Chem., 2018, 260, 317–326 CrossRef CAS.
- W. E. Zeller, M. L. Sullivan, I. Mueller-Harvey, J. H. Grabber, A. Ramsay, C. Drake and R. H. Brown, Protein precipitation behavior of condensed tannins from Lotus pedunculatus and Trifolium repens with different mean degrees of polymerization, J. Agric. Food Chem., 2015, 63, 1160–1168 CrossRef CAS PubMed.
- E. De La Hera, M. Gomez and C. M. Rosell, Particle size distribution of rice flour affecting the starch enzymatic hydrolysis and hydration properties, Carbohydr. Polym., 2013, 98, 421–427 CrossRef CAS PubMed.
- M. Minekus, M. Alminger, P. Alvito, S. Ballance, T. Bohn, C. Bourlieu, F. Carrière, R. Boutrou, M. Corredig, D. Dupont, C. Dufour, L. Egger, M. Golding, S. Karakaya, B. Kirkhus, S. Le Feunteun, U. Lesmes, A. Macierzanka, A. Mackie, S. Marze, D. J. McClements, O. Ménard, I. Recio, C. N. Santos, R. P. Singh, G.
E. Vegarud, M. S. J. Wickham, W. Weitschies and A. Brodkorb, A standardised static in vitro digestion method suitable for food – an international consensus, Food Funct., 2014, 5, 1113–1124 RSC.
- A. C. Dona, G. Pages, R. G. Gilbert and P. W. Kuchel, Digestion of starch: In vivo and in vitro kinetic models used to characterise oligosaccharide or glucose release, Carbohydr. Polym., 2010, 80, 599–617 CrossRef CAS.
- R. Goh, J. Gao, V. K. Ananingsih, V. Ranawana, C. J. Henry and W. Zhou, Green tea catechins reduced the glycaemic potential of bread: An in vitro digestibility study, Food Chem., 2015, 180, 203–210 CrossRef CAS.
- X. Yang and F. Kong, Effects of tea polyphenols and different teas on pancreatic α-amylase activity in vitro, LWT – Food Sci. Technol., 2016, 66, 232–238 CrossRef CAS.
- Q. He, Y. Lv and K. Yao, Effects of tea polyphenols on the activities of α-amylase, pepsin, trypsin and lipase, Food Chem., 2007, 101, 1178–1182 CrossRef CAS.
- Q. Fei, Y. Gao, X. Zhang, Y. Sun, B. Hu, L. Zhou, S. Jabbar and X. Zeng, Effects of Oolong tea polyphenols, EGCG, and EGCG3′′Me on pancreatic α-amylase activity in vitro, J. Agric. Food Chem., 2014, 62, 9507–9514 CrossRef CAS PubMed.
- F. Barros, J. M. Awika and L. W. Rooney, Interaction of tannins and other sorghum phenolic compounds with starch and effects on in vitro starch digestibility, J. Agric. Food Chem., 2012, 60, 11609–11617 CrossRef CAS PubMed.
- M. Yilmazer-Musa, A. M. Griffith, A. J. Michels, E. Schneider and B. Frei, Grape seed and tea extracts and catechin 3-gallates are potent inhibitors of α-amylase and α-glucosidase activity, J. Agric. Food Chem., 2012, 60, 8924–8929 CrossRef CAS PubMed.
- G. J. McDougall, N. N. Kulkarni and D. Stewart, Berry polyphenols inhibit pancreatic lipase activity in vitro, Food Chem., 2009, 115, 193–199 CrossRef CAS.
- L. Jakobek, Interactions of polyphenols with carbohydrates, lipids and proteins, Food Chem., 2015, 175, 556–567 CrossRef CAS.
- D. B. Amoako and J. M. Awika, Polymeric tannins significantly alter properties and in vitro digestibility of partially gelatinized intact starch granule, Food Chem., 2016, 208, 10–17 CrossRef CAS.
- L. Sun, M. J. Gidley and F. J. Warren, Tea polyphenols enhance binding of porcine pancreatic α-amylase with starch granules but reduce catalytic activity, Food Chem., 2018, 258, 164–173 CrossRef CAS PubMed.
- S. Lamothe, N. Azimy, L. Bazinet, C. Couillard and M. Britten, Interaction of green tea polyphenols with dairy matrices in a simulated gastrointestinal environment, Food Funct., 2014, 5, 2621–2631 RSC.
- P. Bandyopadhyay, A. K. Ghosh and C. Ghosh, Recent developments on polyphenol-protein interactions: effects on tea and coffee taste, antioxidant properties and the digestive system, Food Funct., 2012, 3, 592–605 RSC.
- C. Ren, W. Xiong, D. Peng, Y. He, P. Zhou, J. Li and B. Li, Effects of thermal sterilization on soy protein isolate/polyphenol complexes: Aspects of structure, in vitro digestibility and antioxidant activity, Food Res. Int., 2018, 112, 284–290 CrossRef CAS.
- G. A. Camelo-Méndez, E. Agama-Acevedo, C. M. Rosell, M. de J. Perea-Flores and L. A. Bello-Pérez, Starch and antioxidant compound release during in vitro gastrointestinal digestion of gluten-free pasta, Food Chem., 2018, 263, 201–207 CrossRef PubMed.
- E. De La Hera, C. M. Rosell and M. Gomez, Effect of water content and flour particle size on gluten-free bread quality and digestibility, Food Chem., 2014, 151, 526–531 CrossRef CAS PubMed.
- A. Briffaz, P. Bohuon, J. M. Méot, M. Dornier and C. Mestres, Modelling of water transport and swelling associated with starch gelatinization during rice cooking, J. Food Eng., 2014, 121, 143–151 CrossRef CAS.
Footnote |
† Electronic supplementary information (ESI) available. See DOI: 10.1039/d0fo01145b |
|
This journal is © The Royal Society of Chemistry 2020 |