DOI:
10.1039/D0FO00472C
(Paper)
Food Funct., 2020,
11, 4026-4037
Aryl hydrocarbon Receptor activation during in vitro and in vivo digestion of raw and cooked broccoli (brassica oleracea var. Italica)†
Received
24th February 2020
, Accepted 14th April 2020
First published on 15th April 2020
Abstract
Broccoli is rich in glucosinolates, which can be converted upon chewing and processing into Aryl hydrocarbon Receptor (AhR) ligands. Activation of AhR plays an important role in overall gut homeostasis but the role of broccoli processing on the generation of AhR ligands is still largely unknown. In this study, the effects of temperature, cooking method (steaming versus boiling), gastric pH and further digestion of broccoli on AhR activation were investigated in vitro and in ileostomy subjects. For the in vitro study, raw, steamed (t = 3 min and t = 6 min) and boiled (t = 3 min and t = 6 min) broccoli were digested in vitro with different gastric pH. In the in vivo ileostomy study, 8 subjects received a broccoli soup or a broccoli soup plus an exogenous myrosinase source. AhR activation was measured in both in vitro and in vivo samples by using HepG2-Lucia™ AhR reporter cells. Cooking broccoli reduced the AhR activation measured after gastric digestion in vitro, but no effect of gastric pH was found. Indole AhR ligands were not detected or detected at very low levels both after intestinal in vitro digestion and in the ileostomy patient samples, which resulted in no AhR activation. This suggests that the evaluation of the relevance of glucosinolates for AhR modulation in the gut cannot prescind from the way broccoli is processed, and that broccoli consumption does not necessarily produce substantial amounts of AhR ligands in the large intestine.
1. Introduction
Health benefits of consuming broccoli and cabbage, which belong to the genus Brassica, have been partly attributed to phytochemicals such as glucosinolates.1 Recently, broccoli (Brassica oleracea) consumption was shown to alter the microbiota,2 and attenuate chemically induced colitis in mice through an aryl hydrocarbon receptor (AhR)-dependent pathway.3 Intestinal sources of AhR ligands play an important role in immune function in the gut by recruiting intraepithelial lymphocytes to the epithelium, enhancing the gut defences, triggering anti-inflammatory pathways and controlling microbial load and composition through innate lymphoid cell production of IL-22.4–6
Brassica vegetables are rich in glucosinolates, which can be converted into AhR ligands by myrosinase, a plant enzyme that can enzymatically hydrolyse the chemically stable glucosinolates.4,7,8 Myrosinase and glucosinolates are physically separated from each other in intact vegetable tissues, but mastication in the oral cavity allows them to come into contact with each other, promoting enzymatic hydrolysis of glucosinolates into a variety of breakdown products (BP).9 Glucosinolate hydrolysis is influenced by many endogenous factors like pH, ascorbic acid concentration, and exogenous factors like storage and processing conditions, which can affect myrosinase activity and breakdown product formation.8 Indolyl glucosinolates, i.e. those containing an indole group, undergo a similar pathway, albeit different from several aspects. After removal of the glucose moiety by myrosinase, an intermediate thiohydroxamate-O-sulfonate forms that can convert either in an indoyl isothiocyanate or into indole-3-acetonitrile (I3N). The isothiocyanate spontaneously converts in indole-3-carbinol (I3C). The relative concentration of I3C and I3N depends on the residual epithiospecifier protein (ESP) activity.10 ESP is a myrosinase co factor, that can favour the formation of nitriles over isothiocyanates in an iron-dependent manner.10 In addition, presence of the epithiospecifier modifier protein (ESM1), can inhibit the ESP mediated nitrile formation leading to an increase in ITC and thus of I3C.11 At low pH, nitriles are the main BP from glucosinolates. Additionally, nitriles can be produced during autolysis of fresh plant material. When ascorbic acid is present at a pH between 4 and 7, ascorbigen and thiocyanates will be the main BP of indole glucosinolates. At pH 6–7, the main BP are isothiocyanates (ITC) I3C subsequently condenses in di-, tri- and tetramers, such as indolo-[3,2b]carbazole (ICZ), cyclic triindole (CT) and 3,3′-diindolylmethane (DIM) under relatively mild acidic conditions like those in the gastric environment.12,13 The biological functions of I3C include reducing DNA-adduct formation, inducing apoptosis and inhibiting tumour growth.14,15 It is also a weak AhR ligand, while the products of the acid-catalyzed oligomerization DIM and ICZ have a high AhR binding capacity.16 The generation of indole AhR ligands from glucobrassicin is shown in Fig. 1.
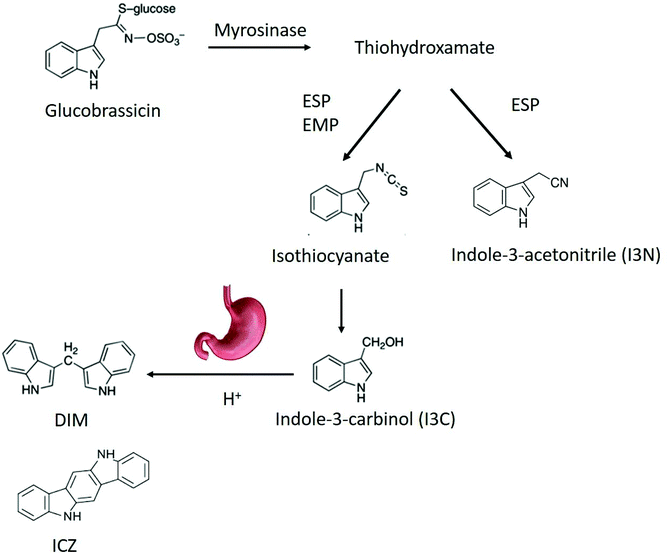 |
| Fig. 1 Breakdown pathway of glucobrassicin into AhR ligands. DIM,3,3′-diindolylmethane; ICZ, indolo-[3,2b]carbazole; ESP, epithiospecifier protein; EMP, epithiospecifier modifier protein. | |
Broccoli is commonly cooked before consumption, which influences glucosinolate degradation and conversion into AhR ligands.17,18 The formation of AhR ligands is influenced by gastric pH,19 but the a direct measure of AhR activation after cooking has not been reported yet. The gastric pH can be influenced by fasted or fed state, but also by different food products when combined in a meal, which can act as buffer in the gastric environment.19–21
The aim of this research was to investigate the effect of broccoli processing and consumption using in vitro and in vivo digestion methods on AhR activation. We investigated the effect of cooking method (boiling versus steaming) and gastric pH on AhR activation by glucosinolate derivatives from broccoli in vitro. To investigate AhR activation in humans and the effect of absorption, which is lacking in in vitro systems, we also measured AhR activity in the ileal fluid samples collected from ileostomy subjects after consumption of a broccoli soup with or without supplementation of an exogenous source of active myrosinase.
2. Experimental
2.1. Sample preparation and thermal treatments
2.1.1. Broccoli preparation and thermal treatments for in vitro study.
One batch of broccoli was purchased at the local supermarket (Jumbo, The Netherlands). The stems of the broccoli were removed and the florets were cut into pieces of approximately 3 × 3 × 3 cm. The florets were equally divided into 5 groups and treated as follows: raw (group 1), boiled for 3 min (group 2), boiled for 6 min (group 3), steamed for 3 min (group 4) and steamed for 6 min (group 5). Broccoli (265 g) was boiled in 1.4 L water (groups 2 and 3), or 240 g broccoli was steamed in a steam oven (groups 4 and 5), for 3 or 6 min. Thereafter, the broccoli was drained of water for 5 min, immediately frozen by liquid nitrogen and freeze-dried. The trays used for freeze-drying were weighed before and after freeze-drying to determine the loss in water content of the samples. After freeze-drying, the samples were milled (2 min cooling time, 7 min milling time) into a fine powder, using a freeze miller (SpexEuropa, SPEX SamplePrep) and stored at −20 °C until further analysis.
2.1.2. Broccoli preparation and thermal treatments for in vivo study.
Beneforte super broccoli, a hybrid of Brassica oleracea & Brassica villosa22 was supplied by Staples Vegetables Ltd (Boston, Lincolnshire, UK) and mustard seeds were sourced from a local supermarket (ASDA, Reading, UK). Broccoli florets were cut (∼4 to 5 cm from top) mixed together, 50 g portions placed in LDPE polyethylene bags (24 × 24 cm) and vacuum-packed to prevent glucoraphanin leaching during processing. Broccoli was cooked in a water bath at 100 °C for 12 min. Mustard seeds were ground to a powder and added as a source of myrosinase (at 2% w/w) at the end of the soup preparation (∼60 °C). All raw and cooked super broccoli samples were frozen in dry ice before storage at −80 °C. Subsequently, samples were lyophilized (VirTis SP Scientific, UK), ground using a coffee grinder and sieved (30 mesh). Samples were stored at −20 °C until soup preparation.
A 200 ml portion of soup was prepared per participant as follows, (Table 1): broccoli powder was added to boiling water (150 mL) and cooked for 2 min. The remaining ingredients, except mustard seed powder, were dissolved in 50 mL cold water and then added to the boiling soup. The soup was boiled for 3 additional min to inactivate ESP.10 Afterwards, the soup was left to cool down to 60 °C, followed by addition of the mustard seed powder. The soup maintained in a water bath at 60 °C and served after 10 minutes.
Table 1 Ingredients of 200 mL broccoli soup
Broccoli soup (200 mL) |
Gram |
Wheat flour, cheddar cheese powder (16%), cheese powder (16%), maize starch, skimmed milk powder, salt, yeast extract, flavouring, maltodextrin, whey powder, mustard powder, colours (curcumin, paprika extract).
|
Water |
200 |
Broccoli powder |
11 |
Sauce mixa |
11 |
Cheese powder (parmesan cheese) |
5 |
Salt |
0.3 |
Mustard seed powder |
0.16 |
2.2. Determination of glucosinolate content and myrosinase activity
Glucosinolates of the thermally treated broccoli samples were extracted according to Oliviero et al.17 with and without the pre-addition of water to estimate the myrosinase activity. To determine the glucosinolates concentrations, 0.1 g freeze dried broccoli was mixed with 2.4 mL 100% methanol (75 °C) and 200 μL 3 mM glucotropaeolin (Sigma-Aldrich), after which the samples were incubated in a water bath at 75 °C for 5 min. The samples were centrifuged at 1363g for 10 min and the supernatants were collected, while the pellets were re-extracted twice by adding 2 mL 70% methanol (75 °C). All supernatants were combined and stored at −20 °C until desulphation. Glucosinolates were converted into desulphoglucosinolates according to Oerlemans et al.23 The extracted desulphated glucosinolates were separated using an Ultimate 3000 U-HPLC (Thermo Scientific, Bremen, Germany) with Licospher 100 RP 18 column (125 × 4 mm) with an injection volume of 20 μL and a flow rate of 1 mL min−1. Two eluents were used, eluent A (100% milliQ) and eluent B (100% Acetonitrile). The HPLC ran for 25 min with a gradient elution of 100% A for 2 min, 92% A for 5.5 min, 75% A for 10.5 min and back to 100% A for 2 min. The peaks were measured with a UV detector at a wavelength of 229 nm. The glucosinolates were identified by means of their absorption spectra and quantified by comparing their area to the area of a standard glucosinolate; glucotropaeolin (benzylglucosinolate).
In order to estimate myrosinase activity, the thermally treated broccoli samples were pre-incubated with water for 5 min, after which the glucobrassicin concentration was measured as described above. The myrosinase activity was expressed as percent of glucobrassicin reduction where the glucobrassicin concentration with and without water addition was compared.
2.3. Digestion: in vitro and in vivo
2.3.1. INFOGEST in vitro digestion.
To simulate complete human digestion, the protocol of Minekus et al. (2014) was used with some modifications.24 In short, 0.5 g freeze dried broccoli (samples from group 1 to 5) was added to 4.5 g milliQ and incubated for 5 min at 37 °C while rotating to simulate the oral phase. This was mixed with 3.5 mL simulated salivary fluid (1.475 mL milliQ, 25 μL 0.3 M CaCl2) and incubated for 2 min at 37 °C while rotating at speed 10 (Salm en Kipp B.V.). Subsequently, the oral bolus went to the gastric phase where the pH was decreased to pH 3 with HCl and mixed with 7.5 mL simulated gastric fluid (5 μL 0.3 M CaCl2, milliQ and 1.6 mL 2000 U mL−1 pepsin, Sigma-Aldrich), this mixture was incubated for 2 hours at 37 °C while rotating. Subsequently, the food bolus went to the intestinal phase where the pH was increased to pH 7 using NaOH and mixed with 11 mL simulated intestinal fluid (40 μL 0.3 M CaCl2, milliQ and pancreatin; based on trypsin activity of 100 U mL−1 in final mixture (Sigma-Aldrich)). Again, this was incubated for 2 hours at 37 °C while rotating. Samples were taken after the gastric and intestinal phases and centrifuged at 9000g for 12 min. The supernatants were stored at −20 °C until further analysis.
2.3.2.
In vitro gastric digestion of broccoli samples.
To simulate human gastric digestion and vary the gastric pH, a simplified in vitro digestion method was used. Freeze-dried broccoli powder (0.5 g samples from groups 1–5) was added to 4.5 mL milliQ and incubated for 5 min at 37 °C while rotating. Subsequently, 5 mL dichloromethane (Actu-All Chemicals) was added and incubated for 2 min at room temperature while shaking, followed by centrifugation at 2670g for 5 min. This procedure was repeated twice. Supernatant was collected and dichloromethane was evaporated using N2, after which 1 mL acetonitrile (Actu-All Chemicals) was added to re-dissolve the active compounds. This was mixed with 6.5 mL milliQ, and the pH was decreased to either pH 2, 3 or 5 using HCl. Subsequently, milliQ was added to reach a final volume of 10 mL with the correct pH and the samples were incubated for 2 hours at 37 °C while rotating. After incubation, the pH was increased to pH 7 using NaOH and the samples were centrifuged for 12 min at 4816g. The supernatants were stored at −20 °C until further analysis.
2.3.3.
In vivo dietary intervention study with ileostomy patients.
To compare the in vitro broccoli digestion with in vivo digestion, ileostomy patients were used. Ileostomy studies provide insight into the processes of biotransformation and absorption in the upper gastrointestinal tract that impact the phytochemicals in the ingested plant material, in the case of broccoli potentially influencing AhR bioactivity and health benefits.25 Ethical approval (14/SC/1326) for the ileostomy feeding study was received from South Central – Hampshire Research Ethics Committee and Ulster University. All participants gave written informed consent and the study was conducted in accordance with the Helsinki Declaration. Participants were recruited between January 2015 and February 2015 from clinics at Altnagelvin Hospital. The intervention study ran between March 2015 and April 2015. The study was registered at clinicaltrials.gov (Identifier: NCT04113928). The study was conducted in 11 ileostomy subjects (7 males, 4 females) age range 32–63 years, who had undergone terminal ileostomies, were at least 1.5 years post-operative prior to the study and were non-smokers.
The study was an acute randomised, single-blind, placebo-controlled crossover feeding trial with a 1-week washout period. After obtaining informed consent, participants were randomly assigned, in blocks of four using a random-number generator (http://www.randomization.com), to either the intervention or the control. In total, 12 participants were randomised to 2 groups. One participant subsequently withdrew from the study prior to sampling. Participants were asked to follow a restriction diet, avoiding dark green leafy vegetable and mustard-like vegetables especially broccoli, cabbage, Brussel sprouts, watercress, rocket, spinach, onions, spring onions, radish, horseradish for 48 h before each clinic visit. Following an overnight fast, the participants provided an ileal fluid sample (T 0 h) then consumed 200 mL of freshly prepared broccoli soup (described above) with or without addition of mustard seed (containing myrosinase). A second ileal fluid sample was collected 4 hours post consumption (T 4 h). The ileal fluid samples were collected and processed as described in McDougall et al.26 Weights and pH of the ileal fluid were recorded, before dilution with ice cold distilled water as required dependent on viscosity, before being homogenised in a chilled Waring blender for 30 seconds, and storage of aliquots at −80 °C in preparation for subsequent analysis. An available subset of 8 participants were used to assess AhR activation, where 4 subjects started with the broccoli soup and the other 4 with the broccoli soup + mustard seeds.
Freeze-dried ileostomy sample (0.5 g) was extracted using dichloromethane as described in section 2.3.2. After evaporation, the compounds were redissolved in 2 mL MEM growth medium (Gibco, USA) and centrifuged at 3000g for 5 min. The supernatant was added in a 1% final concentration to HepG2-Lucia™ AhR reporter cells (InvivoGen, France) to measure AhR activation (section 2.4). In addition, cytotoxicity was measured using the CytoTox 96 Non-Radioactive Cytotoxicity Assay according to the manufacturer's instructions (Promega, USA).
Samples were further processed using solid phase extraction (SPE), using Strata-E C18 reversed phase columns (Phenomenex), in order to clean up for quantification. The columns were conditioned with 1 mL methanol and equilibrated with 1 mL milliQ. Pre-extracted ileostomy sample (100 μL) was loaded, after which 1 mL 5% methanol in milliQ was loaded. The column was dried under vacuum for 5 min and the sample was eluted with 1 mL methanol. Afterwards, the methanol was evaporated and samples were redissolved in 150 μL milliQ until quantification using LC-MS/MS (2.6).
2.4. AhR activation
HepG2-Lucia™ AhR reporter cells (InvivoGen) were grown according to the manufactures instructions in Minimum Essential Medium Eagle – Alpha Modification (α-MEM; Gibco USA), 10% (v/v) heat inactivated fetal calf serum (Gibco), 1× non-essential amino acids (Gibco), Penicillin–Streptomycin (100 U mL−1–100 μg mL−1; Gibco), 100 μg mL−1 Normocin™ (InvivoGen) and 100 μg mL−1 Zeocin™ (InvivoGen). To measure AhR activation, 20 μl sample was added in triplicate to a 96-wells plate (Corning, USA). Thereafter, 180 μl cell suspension (∼20
000 cells per well) was added to each well and the plate was incubated for 48 hours at 37 °C in a CO2 incubator. After incubation, 20 μL stimulated cell supernatant was transferred into a white walled clear bottom 96-wells plate (Corning), followed by addition of 50 μL QUANTI-Luc™ (InvivoGen). Luminescence was measured immediately using a Spectramax M5 (Molecular devices, USA). AhR activation was expressed as percentage of the activity of the positive control (5 μM β-naphthoflavone in DMSO). Results of AhR activation were corrected for their corresponding negative controls, either DMSO or medium.
2.5. Detection of I3C and DIM
To detect I3C and DIM in the ileostomy samples, the samples were extracted by the procedure described previously by Dominguez-Perles et al., with some modifications.27 Briefly, the frozen ileal fluid samples were thawed and vortexed, and duplicate 5.0 ± 0.1 g samples were weighed into 15 mL centrifuge tubes. These samples were extracted using 2 mL methanol
:
water (80
:
20, v/v) with 1% formic acid. The tubes were vortexed for 3 × 30 s and then sonicated in a water bath for 1 min. All procedures were carried out at 5 °C. After centrifugation (4000g, 15 min, 4 °C), the supernatants were transferred into new tubes. The pellets were extracted twice using 3 mL of the same solution and the supernatants combined and vortexed. The extracts were further extracted using SPE Strata-X cartridges (33u Polymeric Strong Cation), following the manufactureŕs instructions. Cartridges were conditioned with 2 mL of MeOH and equilibrated with 2 mL ultrapure water/formic acid (98
:
2, v/v). After this step, the extracted ileal simples were applied to the column. Then, the SPE cartridges were washed with water/formic acid (98
:
2, v/v) and aspirated until dryness. The target analytes including I3C and DIM, were eluted with 2 mL of MeOH/formic acid (98
:
2, v/v) and dried using a Speed Vac concentrator. The extract was reconstituted with 200 μL of solvent A/B (90
:
10, v/v) until analysis.
The targeted analysis of I3C and DIM was performed on an Ultra High Performance Liquid Chromatography Photo Diode Array mass spectrometer system (UHPLC-PDA-MS; Thermo Scientific, USA) comprising of a UHPLC pump, a PDA detector scanning from 200 to 600 nm, and an autosampler operating at 4 °C (Dionex Ultimate 3000 RS, Thermo Corporation). Separations of I3C and DIM were based on a 100 × 2.1 mm i.d. 1.8 μm Zorbax SB-C18 RRHD column (Agilent, Santa Clara, CA) preceded with a precolumn with the same stationary phase (Waters, Spain) which was maintained at 40 °C and eluted using two mobile phases: A: deionized water with 13 mM of ammonium acetate (pH 4.0 with acetic acid) and B: acetonitrile with 1% acetic acid, over the course of 20 min at 0.2 mL min−1. The gradient started with 3% of B rising 20% B in 5 min, then rising 52% B in 3 min following 95% of B after 4.5 min, maintained for 2.5 min and finally return to 3% B in 1 min and maintained during 4 min to equilibrate the column to the initial conditions. After passing through the flow cell of the PDA detector, the eluate went directly to an Exactive Orbitrap mass spectrometer (Thermo Scientific) fitted with a Heated Electrospray Ionization Probe (HESI) operating in positive ionization mode. Full scans were recorder in m/z range from 100 to 800 with a resolution of 50.000 Hz and with a full AGC target of 100
000 charges, using 2 microscans. Analyses were also based on scans with in-source collision-induced dissociation (CID) at 25.0 eV. MS experiment condition with HESI in positive ionization mode was: capillary temperature 325 °C, heater temperature 300 °C, sheath gas 18 units, auxiliary gas 2 units, and the spray voltage 4.0 kv. Targeted identifications of I3C and DIM were achieved by comparing the exact mass and the retention time with available standards. Quantification of the compounds was carried out by selecting the theoretical exact mass of the molecular ion by reference to standard curves prepared in diluted ileal fluid extract.
2.6. Detection of indole derivatives
To detect indole derivatives in the ileostomy samples, a Thermo UltiMate 3000 U-HPLC system coupled to a TSQ Quantum triple quadrupole mass spectrometer (Thermo Fisher Scientific, Germany) was used. Chromatography was carried out with a Phenomenex Kinetex 1.7 μm EVO C18 100 Å LC column (100 × 2.1 mm) maintained at 45 °C. Mobile phases consisted of milliQ (A) and methanol (B) at a flow rate of 0.3 mL min−1 with the following gradient: 0–2 min, 0.1% B; 2–6 min, 0.1–25% B; 6–10 min, 25–80% B; 10–12 min, 80–90% B; 12–15 min, 90% B; 15–16 min, 90–0.1% B; then re-equilibration for 10 min. The samples were kept at 5 °C in an auto sampler (WPS-3000 TRS, Thermo Fisher Scientific) throughout the run and 10 μL per sample was injected for analysis under positive mode. The electrospray sources parameters were set as follows: spray voltage, 4.5 kV; capillary temperature, 275 °C; sheath gas, 40 arbitrary units; auxiliary gas, 0 arbitrary units. Table S1 (ESI†) listed the exact mass precursor ions, exact mass product ions, retention time (RT), collision energy (CE), and tube lens (TL) for each metabolite. Data analysis was performed using Thermo Xcalibur 4.0 software (Thermo Fisher Scientific).
2.7. Statistical analysis
All statistical analyses were performed using Graphpad Prism 5 (La Jolla, USA). Results were expressed as mean ± standard error of the mean (SEM). Differences were considered significant when p < 0.05. Differences in glucosinolates were tested using a one-way ANOVA followed by a Tukey post-hoc test. AhR activation among the differently treated broccoli, were tested using a one-way ANOVA followed by a Tukey post-hoc analysis. AhR activation among different pH was tested using a repeated measures ANOVA followed by a Bonferroni post-hoc test. The samples before and after in vitro digestion were tested using a one-way repeated measures ANOVA followed by a Bonferroni post-hoc test. Letters above bars represent classes of statistically significant different responses compared to each sample. Each graph bar with the same letter is not statistically significant different.
3. Results and discussion
3.1. Glucobrassicin concentration and myrosinase activity
We quantified the level of glucobrassicin in the raw and thermally treated broccoli samples (Fig. 2a). Glucobrassicin is the only glucosinolate responsible for AhR activation as it can be converted into AhR ligands DIM and ICZ via I3C.3,16,28,29 The glucobrassicin concentration in the 3 min steamed broccoli was the highest, with 466 μmol per 100 g, which was significantly higher than the raw broccoli (280 μmol per 100 g, p < 0.05) and boiled broccoli samples (293 and 199 μmol per 100 g after 3 and 6 min boiling, p < 0.05 and p < 0.01 respectively). The concentration of glucobrassicin is highly dependent on the type and batch of broccoli, and our values lie within the previously reported ranges such as those reported by Oliviero et al., and Vallejo et al.17,30 The loss of total glucosinolates during heating has been previously reported and attributed to leaching of glucosinolates into the cooking water (up to 90%),30–35 and a time-dependent glucosinolate loss by thermal treatment.17,36,37 A lower amount of glucobrassicin was measured in raw broccoli compared to steamed broccoli (3 min, p < 0.05), due to an increased extractability in the thermally treated broccoli matrix, as reported previously.17,18,38
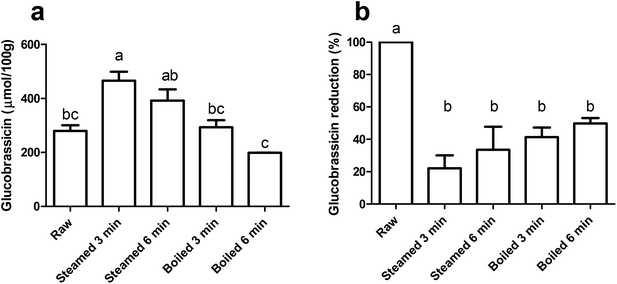 |
| Fig. 2 Effect of cooking method on glucobrassicin content and myrosinase activity. (a) Glucobrassicin concentration (μmol per 100 g freeze dried broccoli) for raw, steamed (3 or 6 min) and boiled (3 or 6 min) broccoli (n = 2). (b) Glucobrassicin reduction (%) with addition of water before extraction compared to no addition of water before extraction (n = 3). Data are expressed as mean ± SEM. Bars with different letters denote treatments that are significantly different (one-way ANOVA followed by Tukey post-hoc test, p < 0.05). | |
To estimate the myrosinase activity in the thermally treated samples, extraction was performed with pre-incubation of water, in order to facilitate hydrolysis of glucosinolates by myrosinase. Fig. 2b shows the glucobrassicin reduction in the thermally treated samples after the addition of water. The raw broccoli had the highest myrosinase activity, as there was no glucobrassicin found after incubation with water (p < 0.05). All thermal treatments reduced the myrosinase activity, with no significant differences between the treatments. Myrosinase was not completely inactivated, but a reduction of ≥50% was observed. From this we can conclude that the thermal treatments retained a significant myrosinase activity and that there were no differences observed among the thermal treatments.
3.2. AhR activation by undigested broccoli
Before testing the AhR activation of DIM and I3C in the differently treated broccoli samples, we checked for AhR activation of I3C and DIM in a range of concentrations using a newly developed AhR reporter cell line (Fig. 3a and b). DIM gave higher AhR activation at a lower concentration compared to I3C, namely 75% of the β-naphthoflavone positive control, in the concentration range 25 to 500 μM, while I3C only reached this level of activation when cells were stimulated with concentrations ranging from 100 to 250 μM. This implies that DIM is a more potent AhR ligand, which is confirmed by literature,3,13 but also I3C can activate AhR in a dose-dependent manner. Beside DIM and I3C, also indole-3-acetonitrile (I3N) was previously shown to be a weak AhR ligand, together with ICZ, the latter being the most potent AhR ligand among those obtained from glucobrassicin breakdown.39,40
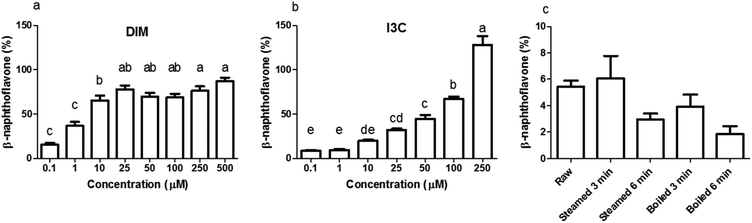 |
| Fig. 3 AhR activation from different concentrations of glucobrassicin breakdown products and from differently cooked broccoli. AhR activation was measured using HepG2-Lucia™ AhR cells, with luciferase production as read out, expressed as percentage of positive control (β-naphthoflavone, 5 μM). (a) 3,3′-Di-indolylmethane (DIM), (b) indole-3-carbinol (I3C). (c) undigested raw, 3 and 6 min steamed, and 3 and 6 min boiled broccoli (1 mg mL−1). Data is expressed as mean ± SEM, n = 3. Bars with different letters denote treatments that are significantly different (one-way ANOVA followed by Tukey post-hoc test, p < 0.05). | |
When undigested broccoli samples were tested, activation of AhR was relatively low but the highest AhR activation was observed in the raw broccoli and broccoli steamed for 3 min, with no cytotoxicity induced by any of the samples (data not shown). The thermal treatments reduced the AhR activation considerably (p < 0.1), with a general trend of AhR activation decreasing as the intensity of the thermal treatment increases (Fig. 3c). However, steaming for 3 min and raw broccoli showed the same level of AhR activation. Boiling is known to result in significant losses of glucosinolates in the cooking water, as can be seen in Fig. 2 by comparing the glucobrassicin content of broccoli steamed and boiled for a comparable time. The loss of glucosinolates by cooking meant that less glucosinolates were available to be hydrolysed into AhR ligands. Loss of myrosinase activity can decrease glucosinolate hydrolysis during mastication and digestion, which decreases AhR activation.31,41 Besides the amount of glucosinolates present and the residual myrosinase activity, AhR activation in broccoli can be modulated by the residual epithiospecifier protein (ESP) activity10 as well as the residual epithiospecifier modifier protein (ESM1), which both determine the relative amount of I3C and I3N.11 However, although I3C and I3N both are AhR ligands,40 only I3C can be further metabolised into the more potent AhR ligands DIM and ICZ.42,43 Compared with raw broccoli, a mild steaming can inactivate ESP leaving enough myrosinase activity to produce I3C, whereas in raw broccoli the largely intact ESP activity may direct glucobrassicin mostly towards I3N.10 The impact of ESP on the AhR activation from broccoli products is difficult to predict, mostly because the relative potency of I3C and I3N as AhR ligands has never been compared. Our results show that light steaming did not decrease AhR activation and that a partial inactivation of myrosinase does not necessarily compromise formation of AhR ligands from glucobrassicin in broccoli. Therefore, we hypothesise that the lower AhR activation observed after more intense thermal treatments is likely the result of loss of glucosinolates rather than of myrosinase activity (Fig. 3c).
3.3. The effect of gastric pH on AhR activation
In a separate set of experiments, we sought to investigate the effect of gastric pH on the formation of AhR ligands and therefore potential AhR ligand activation by broccoli. Therefore, all samples were digested by a simplified in vitro gastric digestion with various pHs (pH 2, 3 and 5). As reported in Fig. 4, raw and 3 min steamed broccoli show significantly higher AhR activation at pH 2 and 3 compared to 3 and 6 min boiled and 6 min steamed broccoli (p < 0.01). At pH 5, the only significant difference was between raw and boiled broccoli, while steamed broccoli did not show a higher activation compared to boiled broccoli. These results are in line with the AhR activation of the undigested samples (Fig. 3), where it was observed that raw and 3 min steaming results in the highest AhR activation.
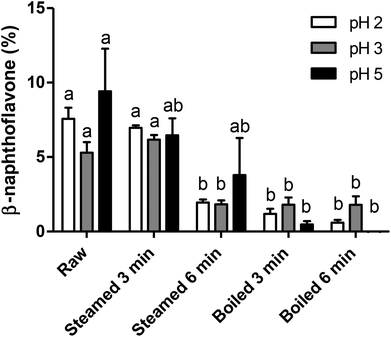 |
| Fig. 4 Effect of gastric pH on AhR activation from differently cooked broccoli. AhR activation was measured using HepG2-Lucia™ AhR cells, with luciferase production as read out, expressed as percentage of positive control (β-naphthoflavone, 5 μM) in raw broccoli, steamed broccoli (t = 3 min, t = 6 min) and boiled broccoli (t = 3 min, t = 6 min), digested at different pH. Data is expressed as mean ± SEM, of 3 independent digestions (3 independent digestions for 6 min steamed broccoli at pH 5). Bars with different letters denote treatments that are significantly different (one-way ANOVA followed by Tukey post-hoc test, p < 0.05). | |
The different pH tested did not significantly influence AhR activation suggesting that in vivo the gastric pH will not affect the formation of AhR ligands in the stomach. Previous research showed that ITC formation is favoured at neutral pH (pH 4–7), while nitrile formation is favoured at acidic pH.8,12,19,44,45 DIM and ICZ are more potent AhR ligands than I3C and I3N.40 As DIM and ICZ are formed from I3C, one could conclude that AhR activation should be highest at neutral pH.8,16,42 In addition, De Kruif et al. (1991) found that the highest amount of DIM formed was found at pH 4–5,20 whereas Grose & Bjeldanes (1992) found that the highest amount of ICZ formed was found at pH close to neutrality.46 In our study, we might have favoured the conversion of isothiocyanates by including an oral phase at neutral pH. Overall, it is difficult to correlate the expected effect of pH on glucobrassicin breakdown products to AhR activation because the observed level of activation is a complex balance of relative levels of I3C, I3N, DIM and ICZ.
Brassica vegetables are normally consumed as part of a meal. The effect of digestion on glucosinolates and AhR activation may therefore also be influenced by the composition of the meal. Although in our study pH showed no significant effect, the presence of ferrous ions at acidic pH increases nitrile formation and could therefore indirectly decrease the formation of DIM and ICZ. Additionally, ascorbic acid is a co-factor of myrosinase and directs hydrolysis towards thiocyanates and ascorbigen formation, which decreases I3C formation and possibly decreases AhR activation.8,19,44,47 As mentioned previously, formation of AhR ligands is theoretically higher at neutral pH, although the literature is not completely in agreement. The pH in an empty stomach can be less than 2, but when food enters the stomach, the buffering effect exerted by food components, primarily by proteins, can increase the pH to 5 or even higher.20,48 This would imply that it could be beneficial to consume raw broccoli in combination with a protein rich product, like meat, although in our study no clear effects of gastric pH were observed, contradicting previous studies.
3.4.
In vitro digestion: gastric and small intestinal effects on AhR
To study the effect of the intestinal digestion on AhR activation, all broccoli samples were digested using the INFOGEST standard in vitro digestion protocol with a pH of 3 during the gastric phase. In Fig. 5, we report the AhR activation of the digesta supernatant, i.e. the activation resulting from AhR ligands formed in the broccoli matrix and released from the matrix during the gastric phase. This would represent the AhR ligands available for absorption or activation in the small intestine. AhR activation of raw broccoli during standard digestion was significantly higher after the gastric phase (p < 0.001) than the cooked broccoli. Also, 3 min steamed broccoli had a higher AhR activation than both 3 and 6 min boiled (p < 0.05). After the intestinal phase, no AhR activation was observed in any of the samples (data not shown). No cytotoxicity was observed after addition of any of the samples (data not shown). Results in Fig. 5 confirm the effect of thermal treatment on formation of AhR ligands from broccoli. However, when results in Fig. 5 and 3c are compared (AhR activation by broccoli before digestion), it appears that the gastric step has activated raw broccoli more than the thermally treated broccoli and, given that the myrosinase activity is not significantly different between the samples and the gastric pH is the same for all the treatments, the differences in activation can only stem from initial differences in the glucobrassicin content, resulting in differences in the levels of AhR ligands. The results in Fig. 3c only show the AhR activation by broccoli in a concentration of 1 mg mL−1, whereas the digested samples were diluted with digestive fluids. Therefore, the AhR activation after the gastric phase might be underestimated. Besides, part of the ligands may have remained entrapped in the broccoli matrix and are possibly released upon fermentation of the polysaccharide matrix in the gut.
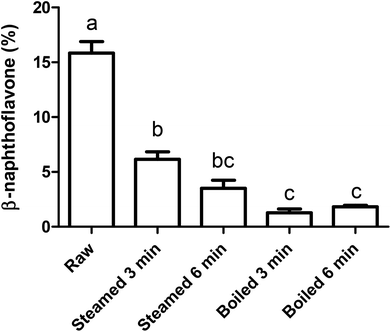 |
| Fig. 5 Effect of gastric digestion on AhR activation from differently treated broccoli. AhR activation measured with HepG2-Lucia™ AhR cells, using luciferase production as read out, expressed as percentage of positive control (β-naphthoflavone, 5 μM). The bioaccessible fraction (supernatant) of glucobrassicin-derived AhR ligands from raw broccoli, steamed broccoli (t = 3 min, t = 6 min) and boiled broccoli (t = 3 min, t = 6 min) after the gastric phase using in vitro digestion were tested. Data is expressed as mean ± SEM with n = 3 for the raw and steamed and n = 2 for the boiled broccoli. Bars with different letters denote treatments that are significantly different (one-way ANOVA followed by Tukey post-hoc test, p < 0.05). | |
To explain the absence of AhR activation after the intestinal phase, despite the absence of an absorption step in our static model of digestion, we tested the degradation or further conversion of I3C and DIM at the neutral pH of the small intestine (Fig. 6). Results show that there is a degradation of both I3C and DIM during 2 hours incubation at pH 7 at 37 °C, ranging from 20% at a low concentration to 5% at a higher concentration.
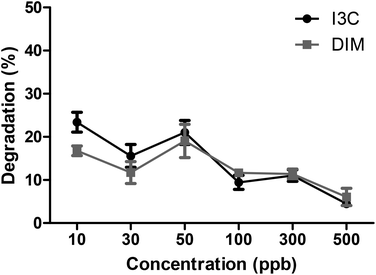 |
| Fig. 6 Degradation (%) of different concentrations of I3C and DIM at pH 7 at 37 °C for 2 hours to simulate the in vitro small intestine. Results expressed as mean ± SEM, n = 3. | |
We also tested the possible interference of digesta fluids on the cells by testing the AhR activation of I3C spiked in pancreatin. Results showed that AhR activation was completely absent when 40 μM I3C was mixed with pancreatin, probably due to cell growth inhibition, which could partially explain the lack of AhR activation found in samples from the in vitro intestinal phase. Of course, the dilution of gastric digesta with simulated intestinal fluids during in vitro digestion also needs to be considered but does not account for the complete loss of AhR activation that was observed. On the other hand, AhR ligands may be slowly released from the broccoli matrix during the 2 hours of the intestinal phase, resulting in a lack of AhR activation.
3.5.
In vivo digestion: ileal fluids and its effects on AhR activation
In order to confirm our in vitro data, ileal fluid samples from ileostomy patients were tested for AhR activation. This was the first time that ileal fluid samples have been tested for their potential to activate AhR. Therefore, these samples may provide useful information on the presence and transformation of AhR ligands during small intestinal digestion, and thus on the potential activation of AhR in the colon. Results show that none of the ileal samples from the 8 ileostomy subjects, who consumed either a broccoli soup (with inactivated myrosinase) or a broccoli soup plus mustard seeds (containing an exogenous source of myrosinase to convert glucobrassicin into I3C), contained AhR ligands in amounts that were high enough to activate AhR in our reporter cell line (data not shown). Also, no cytotoxicity was observed after addition of any of the samples (data not shown). Probably, the AhR ligands are either broken down, diluted too much or absorbed in the very first part of the duodenum. In order to rule out interference from the pancreatic juice on the AhR assay, we spiked one ileostomy sample with I3C which was then tested for AhR activation. Results showed that spiking an ileostomy sample with an amount of I3C, which produced a final concentration of 77 μM in the AhR assay, led to an AhR activation that reached 57% of the positive control, while in the non-spiked sample only 1% AhR activation was found. In relation to Fig. 3b, this implies that the extraction method is effective for I3C and besides, if there had been any AhR ligand present in high enough concentrations in the ileostomy samples, there would have been an AhR response. Indole AhR ligands were detected in the ileal fluid samples (Table 2), but I3C was only found in very limited amount (ranging from 0–71 ng g−1 wet weight ileal fluid, data not shown) and DIM was not detected at all. Although DIM was not detected, we did detect other indole derivatives. Kynurenine is a product of endogenous tryptophan metabolism, while the others (tryptamine, indole-3-aldehyde, indole-3-acetic acid and indole-3-lactic acid) are reported as products of bacterial tryptophan metabolism and possibly produced by the ileal microbiota from dietary tryptophan.49 However, their concentrations were too low to trigger a significant AhR activation in our reporter assay.50
Table 2 Indole derivatives detected by LC-MS/MS in freeze-dried ileal fluid samples after subjects consumed either first a broccoli soup or a broccoli soup + mustard seeds (200 mL). T1 is before ingestion and T2 is 4 hours after ingestion. n = 8 donors. Data is expressed as μg or ng per g dry weight ileostomy sample ± SEM
|
Broccoli soup |
Broccoli soup + Mustard |
T1 |
T2 |
T1 |
T2 |
Tryptophan (μg) |
60.8 ± 3.3 |
117.5 ± 23.2 |
79.2 ± 9.9 |
133.7 ± 18.0 |
Kynurenine (ng) |
87.6 ± 29.5 |
99.5 ± 17.7 |
67.8 ± 24.2 |
42.8 ± 11.5 |
Tryptamine (ng) |
155.8 ± 96.5 |
11.7 ± 5.2 |
111.6 ± 87.0 |
13.2 ± 4.6 |
Indole-3-lactic acid (ng) |
412.5 ± 150.5 |
88.6 ± 9.9 |
1098.6 ± 848.1 |
308.8 ± 99.6 |
Indole-3-aldehyde (ng) |
13.0 ± 8.4 |
34.4 ± 12.1 |
21.5 ± 15.1 |
103.9 ± 12.8 |
Indole-3-acetic acid (ng) |
50.9 ± 30.7 |
18.2 ± 12.6 |
87.8 ± 58.5 |
28.0 ± 17.5 |
Overall, the data from the ileostomy study indicate that the concentration of indole derivatives arriving in the ileum level after broccoli soup consumption was too low to produce a significant increase in the AhR activation with the in vitro assay used herein. This may be due to a limited formation of AhR ligands from glucobrassicin or their absorption through the small intestinal epithelium.8,51 It is possible that after preparation of the broccoli soup there is not enough glucobrassicin present to produce a measurable AhR activation, or that the exogenous myrosinase activity provided with mustard seeds was insufficient to hydrolyse glucobrassicin efficiently. One of the limitations of the present study is the that fate of broccoli glucosinolates as well as the generation of GLs-derived AhR ligands in the colon was not explored. Indeed glucosinolates can also reach the colon intact as reported by Maskell (1990).52 Elfoul et al. (2001) reported that 55% of the initial dose of glucosinolates was still intact in the colon of rats.53 The role played by intact glucobrassicin reaching the colon is not known. Microbiota present in the colon can hydrolyse glucosinolates with a myrosinase activity which is about 10% of the activity present in broccoli, possibly resulting in AhR ligand formation.8,42,54,55 The formation of sulforaphane from glucoraphanin by colonic microbiota has been demonstrated, but not the formation of I3C or its further cyclization into AhR ligands.55 Therefore, it might be that the broccoli particles entering the colon, still containing glucosinolates, can be converted into AhR ligands by colonic microbiota and generate AhR activation in the slightly acidic conditions of the ascending colon. Nonetheless, AhR is highly expressed in, for example, intraepithelial lymphocytes and T-helper 17 cells, which are more abundant in the small intestine.6,56 Therefore, it is important that most AhR ligands at least reach the small intestine, in this in vitro study, we show that there was AhR activation after the gastric phase, indicating the presence of AhR ligands available for the stomach and small intestine.
Another limitation of the present study is that the relative concentrations of several glucobrassicin-derived AhR ligands have not been quantified in the digestive fluids of the in vitro experiments. This includes also I3N, one of the direct product of glucobrassicin hydrolysis by myrosinase. Knowledge of the relative concentrations of all the ligands may help to mechanistically explain the changes in AhR activation produced by the thermal treatments. Furthermore, BPs of other indole GLs (4-methoxybrassicin, 4-hydroxybrassicin, neoglucobrassicin, etc.) may potentially contribute to AhR activation in vivo. Whereas 4-methoxybrassicin, 4-hydroxybrassicin are present in low amounts in broccoli, the content of neoglucobrassicin can contribute substantially to the total indolyl glucosinolates in broccoli.57,58 However, the relative potency of their BPs and corresponding condensation products is unknown.
Finally, it is worth noticing that, in broccoli, BPs from indolyl-GLs are not the only potential source of dietary AhR ligands. Flavonoids are also reported as AhR ligands.59 In the present study, it is apparent that the level of such flavonoids is too small to produce activation of AhR in our in vitro assay and in ileostomy samples. We have previously measured the activation induced by quercetin, a flavonoids relatively abundant in broccoli in our previous study and could not find a significant activation from it.50
4. Conclusions
This research showed that activation of AhR from consumption of broccoli can be modulated by the thermal treatments during domestic cooking or industrial processing. In general, cooking broccoli decreased AhR activation and raw broccoli showed the highest AhR activation. However, a thermal treatment is not necessarily deleterious for the generation of AhR ligands, because mild thermal treatments, like steaming, preserve glucobrassicin and myrosinase activity. Furthermore, AhR activation after the gastric phase during in vitro digestion was higher for raw broccoli but not for the steamed or boiled broccoli, and pH in the gastric phase had no significant effect on AhR activation. However, no AhR activation was found after in vitro digestion or in ileal fluid samples from ileostomy subjects after consumption of broccoli soup, which could be due to processing of the broccoli, uptake of all AhR ligands in the small intestine or interference with the digestive enzymes used in the in vitro assay. Overall, it can be concluded that broccoli consumption resulted in AhR activation after the oral and gastric phase, with the highest AhR activation measured using raw broccoli. In the ileal fluid samples, the concentration of AhR ligands at ileum level was too low to induce significant AhR activation on our cell-assay, but production of AhR ligands in the stomach and their absorption by the small intestine epithelium could have beneficial effects on health.
Abbreviations
AhR | Aryl hydrocarbon receptor |
BP | Breakdown products |
CT | Cyclic triindole |
DIM | 2-(Indol-3-ylmethyl)-3,3′-diindolylmethane |
ESP | Epithiospecifier protein |
I3N | Indole-3-acetonitrile |
I3C | Indole-3-carbinol |
ICZ | Indolo-[3,2b]carbazole |
ITC | Isothiocyanate |
Author contributions
JK performed most of the experiments and wrote the first draft of the manuscript; MK and ZH performed part of the experiments; JW and VF participated in the supervision of the project and the revision of the draft; EC participated in the study design of the in vitro part, supervision of the project and revision of the draft. CG, IR, GmD, GP-C and KP participated in the design and execution of the in vivo study, to the analysis of glucobrassicin BPs and revision of the draft.
Conflicts of interest
The authors declare no competing interests.
Acknowledgements
Research presented in this publication was financially supported by the Graduate School VLAG.
References
- J. Kapusta-Duch, A. Kopec, E. Piatkowska, B. Borczak and T. Leszczynska, The beneficial effects of Brassica vegetables on human health, Rocz. Panstw. Zakl. Hig., 2012, 63, 389–395 CAS.
- J. L. Kaczmarek, X. Liu, C. S. Charron, J. A. Novotny, E. H. Jeffery, H. E. Seifried, S. A. Ross, M. J. Miller, K. S. Swanson and H. D. Holscher, Broccoli consumption affects the human gastrointestinal microbiota, J. Nutr. Biochem., 2019, 63, 27–34 CrossRef CAS PubMed.
- T. D. Hubbard, I. A. Murray, R. G. Nichols, K. Cassel, M. Podolsky, G. Kuzu, Y. Tian, P. Smith, M. J. Kennett and A. D. Patterson, Dietary broccoli impacts microbial community structure and attenuates chemically induced colitis in mice in an Ah receptor dependent manner, J. Funct. Foods, 2017, 37, 685–698 CrossRef CAS PubMed.
- Y. Li, S. Innocentin, D. R. Withers, N. A. Roberts, A. R. Gallagher, E. F. Grigorieva, C. Wilhelm and M. Veldhoen, Exogenous stimuli maintain intraepithelial lymphocytes via aryl hydrocarbon receptor activation, Cell, 2011, 147, 629–640 CrossRef CAS PubMed.
- L. V. Hooper, You AhR what you eat: linking diet and immunity, Cell, 2011, 147, 489–491 CrossRef CAS PubMed.
- J. Qiu, J. J. Heller, X. Guo, E. C. Zong-ming, K. Fish, Y.-X. Fu and L. Zhou, The aryl hydrocarbon receptor regulates gut immunity through modulation of innate lymphoid cells, Immunity, 2012, 36, 92–104 CrossRef CAS PubMed.
- J. W. Fahey, A. T. Zalcmann and P. Talalay, The chemical diversity and distribution of glucosinolates and isothiocyanates among plants, Phytochemistry, 2001, 56, 5–51 CrossRef CAS PubMed.
- B. Holst and G. Williamson, A critical review of the bioavailability of glucosinolates and related compounds, Nat. Prod. Rep., 2004, 21, 425–447 RSC.
- R. Kissen, J. T. Rossiter and A. M. Bones, The ‘mustard oil bomb’: not so easy to assemble?! Localization, expression and distribution of the components of the myrosinase enzyme system, Phytochem. Rev., 2009, 8, 69–86 CrossRef CAS.
- N. V. Matusheski, R. Swarup, J. A. Juvik, R. Mithen, M. Bennett and E. H. Jeffery, Epithiospecifier protein from broccoli (Brassica oleracea L. ssp. italica) inhibits formation of the anticancer agent sulforaphane, J. Agric. Food Chem., 2006, 54, 2069–2076 CrossRef CAS PubMed.
- M. Burow, Z.-Y. Zhang, J. A. Ober, V. M. Lambrix, U. Wittstock, J. Gershenzon and D. J. Kliebenstein, ESP and ESM1 mediate indol-3-acetonitrile production from indol-3-ylmethyl glucosinolate in Arabidopsis, Phytochemistry, 2008, 69, 663–671 CrossRef CAS PubMed.
-
J. Higdon, V. J. Drake and D. E. Williams, Indole-3-carbinol, Linus Pauling Institute, Oregon State University.[Online]. Available: http://lpi.oregonstate.edu/infocenter/phytochemicals/i3c/[December2008], 2008.
- L. F. Bjeldanes, J.-Y. Kim, K. R. Grose, J. C. Bartholomew and C. A. Bradfield, Aromatic hydrocarbon responsiveness-receptor agonists generated from indole-3-carbinol in vitro and in vivo: comparisons with 2, 3, 7, 8-tetrachlorodibenzo-p-dioxin, Proc. Natl. Acad. Sci. U. S. A., 1991, 88, 9543–9547 CrossRef CAS PubMed.
- B. B. Aggarwal and H. Ichikawa, Molecular targets and anticancer potential of indole-3-carbinol and its derivatives, Cell Cycle, 2005, 4, 1201–1215 CrossRef CAS PubMed.
- L. P. Nguyen and C. A. Bradfield, The search for endogenous activators of the aryl hydrocarbon receptor, Chem. Res. Toxicol., 2007, 21, 102–116 Search PubMed.
- P. H. Jellinck, P. Gekforkert, D. S. Riddick, A. B. Okey, J. J. Michnovicz and H. L. Bradlow, Ah receptor binding properties of indole carbinols and induction of hepatic estradiol hydroxylation, Biochem. Pharmacol., 1993, 45, 1129–1136 CrossRef CAS PubMed.
- T. Oliviero, R. Verkerk and M. Dekker, Effect of water content and temperature on glucosinolate degradation kinetics in broccoli (Brassica oleracea var. italica), Food Chem., 2012, 132, 2037–2045 CrossRef CAS.
- T. Oliviero, R. Verkerk and M. Dekker, Isothiocyanates from Brassica Vegetables—Effects of Processing, Cooking, Mastication, and Digestion, Mol. Nutr. Food Res., 2018, 62, 1701069 CrossRef PubMed.
- V. Gil and A. MacLeod, The effects of pH on glucosinolate degradation by a thioglucoside glucohydrolase preparation, Phytochemistry, 1980, 19, 2547–2551 CrossRef CAS.
- C. De Kruif, J. Marsman, J. Venekamp, H. Falke, J. Noordhoek, B. Blaauboer and H. Wortelboer, Structure elucidation of acid reaction products of indole-3-carbinol: detection in vivo and enzyme induction in vitro, Chem.-Biol. Interact., 1991, 80, 303–315 CrossRef CAS PubMed.
- F. Kong and R. Singh, Disintegration of solid foods in human stomach, J. Food Sci., 2008, 73, R67–R80 CrossRef CAS PubMed.
- M. H. Traka, S. Saha, S. Huseby, S. Kopriva, P. G. Walley, G. C. Barker, J. Moore, G. Mero, F. van den Bosch and H. Constant, Genetic regulation of glucoraphanin accumulation in Beneforté® broccoli, New Phytol., 2013, 198, 1085–1095 CrossRef CAS PubMed.
- K. Oerlemans, D. M. Barrett, C. B. Suades, R. Verkerk and M. Dekker, Thermal degradation of glucosinolates in red cabbage, Food Chem., 2006, 95, 19–29 CrossRef CAS.
- M. Minekus, M. Alminger, P. Alvito, S. Ballance, T. Bohn, C. Bourlieu, F. Carrière, R. Boutrou, M. Corredig and D. Dupont, A standardised static in vitro digestion method suitable for food–an international consensus, Food Funct., 2014, 5, 1113–1124 RSC.
- G. J. McDougall, J. W. Allwood, G. Pereira-Caro, E. M. Brown, S. Verrall, D. Stewart, C. Latimer, G. McMullan, R. Lawther and G. O'Connor, Novel colon-available triterpenoids identified in raspberry fruits exhibit antigenotoxic activities in vitro, Mol. Nutr. Food Res., 2017, 61, 1600327 CrossRef PubMed.
- G. J. McDougall, S. Conner, G. Pereira-Caro, R. Gonzalez-Barrio, E. M. Brown, S. Verrall, D. Stewart, T. Moffet, M. Ibars and R. Lawther, Tracking (Poly) phenol components from raspberries in ileal fluid, J. Agric. Food Chem., 2014, 62, 7631–7641 CrossRef CAS PubMed.
- R. Dominguez-Perles, S. Medina, D. Á. Moreno, C. García-Viguera, F. Ferreres and Á. Gil-Izquierdo, A new ultra-rapid UHPLC/MS/MS method for assessing glucoraphanin and sulforaphane bioavailability in human urine, Food Chem., 2014, 143, 132–138 CrossRef CAS PubMed.
- N. Fujioka, C. E. Ainslie-Waldman, P. Upadhyaya, S. G. Carmella, V. A. Fritz, C. Rohwer, Y. Fan, D. Rauch, C. Le and D. K. Hatsukami, Urinary 3, 3′-diindolylmethane: a biomarker of glucobrassicin exposure and indole-3-carbinol uptake in humans, Cancer Epidemiol., Biomarkers Prev., 2014, 23, 282–287 CrossRef CAS PubMed.
- C. P. Wong, A. Hsu, A. Buchanan, Z. Palomera-Sanchez, L. M. Beaver, E. A. Houseman, D. E. Williams, R. H. Dashwood and E. Ho, Effects of sulforaphane and 3, 3′-diindolylmethane on genome-wide promoter methylation in normal prostate epithelial cells and prostate cancer cells, PLoS One, 2014, 9, e86787 CrossRef PubMed.
- F. Vallejo, F. Tomás-Barberán and C. García-Viguera, Glucosinolates and vitamin C content in edible parts of broccoli florets after domestic cooking, Eur. Food Res. Technol., 2002, 215, 310–316 CrossRef CAS.
- M. Dekker, R. Verkerk and W. M. Jongen, Predictive modelling of health aspects in the food production chain: a case study on glucosinolates in cabbage, Trends Food Sci. Technol., 2000, 11, 174–181 CrossRef CAS.
- L. Song and P. J. Thornalley, Effect of storage, processing and cooking on glucosinolate content of Brassica vegetables, Food Chem. Toxicol., 2007, 45, 216–224 CrossRef CAS PubMed.
- R. Verkerk, M. Van der Gaag, M. Dekker and W. Jongen, Effects of processing conditions on glucosinolates in cruciferous vegetables, Cancer Lett., 1997, 114, 193–194 CrossRef CAS PubMed.
- E. A. Rosa and R. K. Heaney, The effect of cooking and processing on the glucosinolate content: studies on four varieties of Portuguese cabbage and hybrid white cabbage, J. Sci. Food Agric., 1993, 62, 259–265 CrossRef CAS.
- G.-F. Yuan, B. Sun, J. Yuan and Q.-M. Wang, Effects of different cooking methods on health-promoting compounds of broccoli, J. Zhejiang Univ., Sci., B, 2009, 10, 580 CrossRef PubMed.
- R. B. Jones, J. D. Faragher and S. Winkler, A review of the influence of postharvest treatments on quality and glucosinolate content in broccoli (Brassica oleracea var. italica) heads, Postharvest Biol. Technol., 2006, 41, 1–8 CrossRef CAS.
- E. Ciska and H. Kozłowska, The effect of cooking on the glucosinolates content in white cabbage, Eur. Food Res. Technol., 2001, 212, 582–587 CrossRef CAS.
- R. Verkerk and M. Dekker, Glucosinolates and myrosinase activity in red cabbage (Brassica oleracea L. var. Capitata f. rubra DC.) after various microwave treatments, J. Agric. Food Chem., 2004, 52, 7318–7323 CrossRef CAS PubMed.
- I. A. Murray and G. H. Perdew, Ligand activation of the Ah receptor contributes to gastrointestinal homeostasis, Curr. Opin. Toxicol., 2017, 2, 15–23 CrossRef PubMed.
- T. D. Hubbard, I. A. Murray and G. H. Perdew, Indole and Tryptophan Metabolism: Endogenous and Dietary Routes to Ah Receptor Activation, Drug Metab. Dispos., 2015, 43, 1522–1535 CrossRef PubMed.
- V. Rungapamestry, A. J. Duncan, Z. Fuller and B. Ratcliffe, Effect of cooking brassica vegetables on the subsequent hydrolysis and metabolic fate of glucosinolates, Proc. Nutr. Soc., 2007, 66, 69–81 CrossRef CAS PubMed.
- E. Katz, S. Nisani and D. A. Chamovitz, Indole-3-carbinol: a plant hormone combatting cancer, F1000Research, 2018, 7 CAS , F1000 Faculty Rev-689.
- C. Bradfield and L. Bjeldanes, Structure—activity relationships of dietary indoles: A proposed mechanism of action as modifiers of xenobiotic metabolism, J. Toxicol. Environ. Health, Part A, 1987, 21, 311–323 CAS.
- L. A. Howard, E. H. Jeffery, M. A. Wallig and B. P. Klein, Retention of phytochemicals in fresh and processed broccoli, J. Food Sci., 1997, 62, 1098–1104 CrossRef CAS.
- A. Mahn and A. Reyes, An overview of health-promoting compounds of broccoli (Brassica oleracea var. italica) and the effect of processing, Food Sci. Technol. Int., 2012, 18, 503–514 CrossRef PubMed.
- K. R. Grose and L. F. Bjeldanes, Oligomerization of indole-3-carbinol in aqueous acid, Chem. Res. Toxicol., 1992, 5, 188–193 Search PubMed.
- Y. Uda, T. Kurata and N. Arakawa, Effects of pH and ferrous ion on the degradation of glucosinolates by myrosinase, Agric. Biol. Chem., 1986, 50, 2735–2740 CAS.
- M. Minekus, M. Alminger, P. Alvito, S. Ballance, T. Bohn, C. Bourlieu, F. Carriere, R. Boutrou, M. Corredig and D. Dupont, A standardised static in vitro digestion method suitable for food–an international consensus, Food Funct., 2014, 5, 1113–1124 RSC.
- H. M. Roager and T. R. Licht, Microbial tryptophan catabolites in health and disease, Nat. Commun., 2018, 9, 3294 CrossRef PubMed.
- J. E. B. Koper, L. M. P. Loonen, J. M. Wells, A. D. Troise, E. Capuano and V. Fogliano, Polyphenols and Tryptophan Metabolites Activate the Aryl Hydrocarbon Receptor in an in vitro Model of Colonic Fermentation, Mol. Nutr. Food Res., 2019, 63, 1800722 CrossRef PubMed.
- A. R. Patel, S. D. Spencer, M. B. Chougule, S. Safe and M. Singh, Pharmacokinetic evaluation and In Vitro–In Vivo Correlation (IVIVC) of novel methylene-substituted 3, 3′ diindolylmethane (DIM), Eur. J. Pharm. Sci., 2012, 46, 8–16 CrossRef CAS PubMed.
-
I. E. Maskell, Nutritional aspects of feeding rapeseed products to rats and pigs, University of Newcastle upon Tyne, 1990 Search PubMed.
- L. Elfoul, S. Rabot, N. Khelifa, A. Quinsac, A. Duguay and A. Rimbault, Formation of allyl isothiocyanate from sinigrin in the digestive tract of rats monoassociated with a human colonic strain of Bacteroides thetaiotaomicron, FEMS Microbiol. Lett., 2001, 197, 99–103 CrossRef CAS PubMed.
- S. Tian, X. Liu, P. Lei, X. Zhang and Y. Shan, Microbiota: a mediator to transform glucosinolate precursors in cruciferous vegetables to the active isothiocyanates, J. Sci. Food Agric., 2018, 98, 1255–1260 CrossRef CAS PubMed.
- E. Capuano, M. Dekker, R. Verkerk and T. Oliviero, Food as pharma? The case of glucosinolates, Curr. Pharm. Des., 2017, 23, 2697–2721 CrossRef CAS PubMed.
- B. Stockinger, P. D. Meglio, M. Gialitakis and J. H. Duarte, The aryl hydrocarbon receptor: multitasking in the immune system, Annu. Rev. Immunol., 2014, 32, 403–432 CrossRef CAS PubMed.
- I. Schonhof, A. Krumbein and B. Brückner, Genotypic effects on glucosinolates and sensory properties of broccoli and cauliflower, Food/Nahrung, 2004, 48, 25–33 CrossRef CAS PubMed.
- A. Rybarczyk-Plonska, S. F. Hagen, G. I. A. Borge, G. B. Bengtsson, M. K. Hansen and A.-B. Wold, Glucosinolates in broccoli (Brassica oleracea L. var. italica) as affected by postharvest temperature and radiation treatments, Postharvest Biol. Technol., 2016, 116, 16–25 CrossRef CAS.
- B. Lamas, J. M. Natividad and H. Sokol, Aryl hydrocarbon receptor and intestinal immunity, Mucosal Immunol., 2018, 11, 1024–1038 CrossRef CAS PubMed.
Footnote |
† Electronic supplementary information (ESI) available. See DOI: 10.1039/d0fo00472c |
|
This journal is © The Royal Society of Chemistry 2020 |