DOI:
10.1039/C9FO02442E
(Paper)
Food Funct., 2020,
11, 921-931
Effect of high hydrostatic pressure extraction on biological activities of stinging nettle extracts
Received
18th October 2019
, Accepted 24th December 2019
First published on 26th December 2019
Abstract
Stinging nettle is traditionally used as a therapeutic herb. In the present work, the biological properties and toxicity of novel nettle leaf extracts obtained by high pressure assisted extraction (HPE) were studied and compared with similar extracts obtained with the same solvent under atmospheric pressure conditions. The studied extracts were obtained at pressure levels of 200 and 500 MPa, ≈10 min, 0 to 70% ethanol
:
water (v/v). Each extract was characterized for its individual compound profile and different biological properties, such as antioxidant activity, pro-oxidant activity (DNA degradation capacity) and antihypertensive activity, as well as cytotoxicity against Caco-2 and HaCat cell cultures. The main results indicate that in addition to the antioxidant and antihypertensive activities observed for the control extracts, a clear improvement of all the biological activities of the extracts obtained by HPE was observed. The extracts obtained at 200 MPa, 10 min, 35 and 70% ethanol were the ones presenting higher concentrations of phenolic acids and flavonoids, such as chlorogenic acid, isoferulic acid, and rutin; besides, they showed better results concerning all the studied biological activities. Those extracts also showed potential for DNA protection, since they were able not only to cause less damage in the DNA molecule than the controls, but also showed no pro-oxidant activity. Concerning cytotoxicity, it was observed that HPE extracts, at a concentration up to 1.0 mg mL−1, presented a metabolism inhibition below 10 and 15% for Caco-2 and HaCat cell lines, respectively.
1 Introduction
Medicinal herbs have been used for their health promotion properties associated with the high diversity and concentration of bioactive compounds, such as phenolic acids, flavonoids, and phytosterols. So, they have received increasing attention due to consumer's growing demand for natural foods and awareness with health. It is well established that phenolic compounds have a beneficial impact on human health, this being attributed to a wide range of biological properties.1Urtica dioica (commonly known as stinging nettle) can be used as a drug, food, dye, or cosmetic and it has been used for centuries as a folk medicine for rheumatism, eczema, arthritis, gout, and anaemia. Some properties have been also demonstrated by recent research namely, diuretic, natriuretic and hypotensive effects.2 Nettle extracts are a rich source of chlorogenic acid, rutin, and isoquercitrin, confirming the potential of nettle for antioxidant, DNA-protective, anti-inflammatory, antihypertensive and analgesic properties.2,3 Phenolic compounds are secondary metabolites found in plant extracts, and are usually linked to several biological activities, majorly antioxidant properties, since phenolics have the capacity of scavenging free radicals by the donation of hydrogen atoms, electrons or chelating metal cations.4 Several studies have shown that a diet rich in food products with a high content of phenolic compounds may contribute to the delay of the aging process since their consumption can lead to the decrease of inflammation and oxidative stress risk, related to chronic diseases.4,5 These compounds have also shown potential as ingredients in cosmetics and as anti-ageing agents, as proven by in vitro studies using dermal fibroblasts or epidermal keratinocytes cell lines (such as HaCaT).6
Extraction is the first step for the isolation and purification/concentration of such components from herbal materials, in order to explore those biological activities. Extraction is defined as the mass transport phenomenon where the compounds present in a matrix are transferred into an appropriate solvent.7,8 Nowadays, there are several conventional extraction methods being used, such as Soxhlet, maceration, or extraction at room temperature. Nevertheless, these methods have some disadvantages, including low efficiency, the need for mild to high temperatures (60–90 °C), the use of large volumes of organic solvents, long extraction times, environmental pollution, and may convey some toxic compounds to the final extracts.9,10 Therefore, there is a need for the development of new methods, called “the green concept”, which incorporate the creation and research of new techniques for the extraction of valuable compounds from herbs, using compatible sustainable and affordable approaches that minimize environmental impact.1 Among these novel technologies is high pressure assisted extraction (HPE) and it is characterized as environmentally friendly having great potential in food and pharmaceutical industries.11 One of the most important advantages of high pressure is the absence of heating, avoiding compound denaturation and ensuring the extraction of thermo-sensitive components.12 In the last few years, HPE has been used for the extraction of carotenoids, ginsenosides, phenolic acids, flavonoids, and anthocyanins, among others, from a diversity of food products, such as fruits, vegetables, food by-products and herbs.9 To the best of our knowledge, HPE in combination with a non-food grade solvent, such as ethanol, has still not been used as a technique for obtaining extracts from fresh stinging nettle leaves with improved biological activities, such as DNA protective (with no pro-oxidant activity) and anti-hypertensive activity.
So, the present work aimed to investigate the biological activities and safety of stinging nettle leaf extracts obtained by HPE using food grade solvents. The HPE effect was evaluated on the individual compound profile, antioxidant activity by oxygen radical absorbance capacity (ORAC) assay, and antihypertensive activity and genotoxicity and cytotoxicity of the final extracts were evaluated.
2 Materials and methods
2.1 Chemical materials
Abz-Gly-Phe(NO2)-Pro (o-aminobenzoylglycyl-p-nitophenylalanylproline) was acquired from Bachem Feinchemikalien (Bubendorf, Germany). Tris-HCl (Tris hydrochloride) buffer was purchased from Fluka (Bucharest, Romania). Sodium dihydrogen phosphate, NaCl (sodium chloride), ZnCl2 (zinc chloride), and H2O2 (hydrogen peroxide) were acquired from Merck (Darmstadt, Germany). Fluorescein, potassium phosphate dibasic, AAPH (2,20-azo-bis-(2-methylpropionamidine)-dihydrochloride), and Trolox (6-hydroxy-2,5,7,8-tetramethylbroman-2-carboxylic acid) were purchased from Sigma–Aldrich. ACE (peptidil-dipeptidase A, EC 3.4.15.1), DNA (deoxyribonucleic acid salt) Type I (fibers) from calf thymus, bromophenol blue sodium salt, and PMS (phenazine methosulfate solution) were purchased from Sigma Chemical (St Louis, MO, USA). Agarose and GreenSafe Premium were purchased from Nztech (Lisboa, Portugal). FeCl3 (Iron(III) chloride) was acquired from Panreac (Barcelona, Spain). TAE buffer (Tris-Acetate EDTA buffer) was purchased from Grisp (Porto, Portugal). Human colon carcinoma (Caco-2) cells were obtained from the European Collection of Authenticated Cell Cultures (ECACC 8601020) through Sigma-Aldrich (St Louis, USA; ECACC) (reference 09042001). XTT (2,3-bis-(2-methoxy-4-nitro-5-sulfophenyl)-2H-tetrazolium-5-carboxanilide) was purchased from Thermo Fisher Scientific. DMEM (Dulbecco's Modified Eagle's Medium), Pen-Strep, and non-essential amino acids 100× were purchased from Lonza (Basel, Switzerland). FBS (Fetal Bovine Serum) was obtained from Biowest (Nuaillé, France).
2.2 Biological samples
Stinging nettle was harvested on May 2016, from the Castro Daire region, Portugal. A batch of 4 kg was collected, transported under refrigeration, and washed with tap water. After removal of the excess water, nettle was separated into leaves, stems, and roots, ground and then packed under vacuum and stored at −45 °C until further analyses.
2.3 Extraction conditions
HPE was used as the extraction method and was carried out at room temperature (20–25 °C), using different extraction times (≈10.0 min), different food grade solvents (0% ethanol (aqueous), 35% ethanol
:
water, v
:
v, and 70% ethanol
:
water, v
:
v), and different pressure levels (200 and 500 MPa). These conditions were selected due to the optimization process performed by using the response surface methodology using a central faced centred composite design described elsewhere (data submitted). Table 1 shows the nomenclature of each extract, and the conditions used to obtain each extract. The extracts were obtained by placing 1.0 g of the fresh plant material in a container together with 20 mL of the extraction solvent. Samples were homogenized with an Ultraturrax T25 homogeniser (Janke & Kunkel IKA-Labortechnik) and the mixture was then placed in low permeability polyamide–polyethylene bags (Albipack-Packaging Solutions, Águeda, Portugal) that were heat sealed manually with care to avoid leaving air inside the bags. HPE experiments were carried out on an industrial scale high pressure equipment (Hiperbaric 55, Hiperbaric, Burgos, Spain) with a pressure vessel of 200 mm inner diameter and 2000 mm length with a maximum operation pressure of 600 MPa. Control samples were maintained at atmospheric pressure (0.1 MPa) under the same conditions of time and solvent concentration, kept in the dark and surrounded by water to mimic all the conditions of the samples under pressure except for the high pressure. These controls at 0.1 MPa were used to mimic conventional extraction.13 After extraction, each mixture was centrifuged at 15
000 rpm, for 10 min, at 4 °C (Heraeus Biofuge Stratos, Thermo, Electron Corporation, Massachusetts, USA). The supernatant was then filtered using a 10–13 μm filter (Whatman no. 1 equivalent, 1250 Filter-Lab, Filtros Anoia, S.A., Barcelona, Spain), and the filtrates were collected and stored at −80 °C until further analyses. All extracts were obtained in triplicate.
Table 1 Identification of extracts by pressure level, extraction time and concentration of ethanol used as the solvent
|
Pressure level |
Extraction time |
Solvent |
Nomenclature |
Extract 1 |
200 MPa |
10.6 min |
0% ethanol |
N200/10.6/0 |
Extract 2 |
0.1 MPa (control) |
10.6 min |
0% ethanol |
N0.1/10.6/0 |
Extract 3 |
500 MPa |
10.0 min |
25% ethanol |
N500/10/25 |
Extract 4 |
0.1 MPa (control) |
10.0 min |
25% ethanol |
N0.1/10/25 |
Extract 5 |
200 MPa |
10.6 min |
35% ethanol |
N200/10.6/35 |
Extract 6 |
0.1 MPa (control) |
10.6 min |
35% ethanol |
N0.1/10.6/35 |
Extract 7 |
200 MPa |
10.6 min |
70% ethanol |
N200/10.6/70 |
Extract 8 |
0.1 MPa (control) |
10.6 min |
70% ethanol |
N0.1/10.6/70 |
2.4 Total phenolic compounds
Total phenolic compounds were quantified using the Folin–Ciocalteu method.14 The Folin–Ciocalteu reagent (100 μL) was mixed with 20 μL of extract in a microplate with 96 wells. After resting for 4 min, 75 μL of sodium carbonate solution were added and the mixture was rested for 2 h in the dark, at room temperature; the absorbance was recorded at 750 nm (Multiskan Go microplate spectrophotometer, Thermo Fisher Scientific Inc., USA). Gallic acid was used as the standard (0–200 mg L−1) and the results are expressed as milligram of gallic acid equivalents per 100 grams of dry matter (mgGAE per 100 g).
2.5 LC-MS/MS analysis of phenolic acids
The identification/quantification of phenolics was conducted on a UPLC Ultimate 3000, Dionex liquid chromatograph coupled to an UHR-QqTOF (Ultra-High Resolution Qq-Time-Of-Flight) mass spectrometer (Impact II™, Bruker, Massachusetts, USA). Mobile Phase: Solvent A: ultra-pure water (100%) (Millipore system) with 0.1% formic acid (Sigma-Aldrich, Germany), and Solvent B: acetonitrile (100%) (Merck pure grade) with 0.1% formic acid; at a flow rate of 0.25 mL min−1. The following gradient was employed: 0–10 min (0% B); 10–14 min (21% B); 14–18.3 min (27% B); 18.3–20 min (58% B); 20–21.5 min (95% B) and 21.5–22 min (0% B). Each run took 21 min to complete. The capillary voltage of the electrospray ionization (ESI) was set to 2500 V and the capillary temperature was 200 °C. Spectra were recorded in negative-ion mode between m/z 20 and 1000. Phenolics were identified and quantified by comparison with commercially available standards and respective calibration curves (Table 2). The results are expressed as milligram per 100 grams of dry matter (mg per 100 g DM).
Table 2 Calibration curves and LC-MS/MS results used to identify and quantify phenolic compounds
|
Calibration curve |
R
2
|
t
retention (min) |
[M − H]− experimental |
MS/MS fragments |
Total phenolic compounds |
y = 0.0054 × (gallic acid equivalents) − 0.0591 |
0.994 |
— |
— |
— |
— |
— |
Neochlorogenic acid (3-caffeoylquinic acid) |
y = 41 461 × (chlorogenic acid equivalent) + 8260.7 |
0.997 |
7.1 |
353.0747 |
191.0491 (100) |
179.0283 (76) |
135.0398 (16) |
Caftaric acid |
y = 82 077 × (caffeic acid equivalent) − 5643.9 |
0.998 |
7.3 |
311.0461 |
179.0374 (100) |
149.0117 (46) |
135.0477 (21) |
5-p-Coumaroylquinic acid |
y = 22 757 × (p-coumaric acid equivalent) + 1986.2 |
0.997 |
8.2 |
337.0985 |
191.0586 (20) |
163.0425 (100) |
119.0523 (12) |
Chlorogenic acid (5-caffeoylquinic acid) |
y = 41 461 × (chlorogenic acid) + 8260.7 |
0.997 |
8.4 |
353.0741 |
191.0488 (100) |
161.0178 (2) |
179.0277 (1) |
Fertaric acid |
y = 17 500 × (ferulic acid equivalent) + 2998.2 |
0.992 |
9.3 |
325.0625 |
193.0525 (100) |
149.0633 (5) |
134.0394 (19) |
4-p-Coumaroylquinic acid |
y = 22 757 × (p-coumaric acid equivalent) + 1986.2 |
0.997 |
9.8 |
337.0982 |
191.0583 (100) |
173.0481 (10) |
— |
2-O-Caffeoylmalic acid |
y = 82 077 × (caffeic acid equivalent) − 5643.9 |
0.998 |
10.3 |
295.0512 |
179.0373 (38) |
133.0168 (100) |
— |
p-Coumaric acid |
y = 22 757 × (p-coumaric acid) + 1986.2 |
0.997 |
11.1 |
163.0397 |
163.0446 (15) |
119.0547 (100) |
— |
Rutin |
y = 39 773 × (rutin) + 2773.2 |
0.993 |
11.7 |
609.1679 |
300.0332 (100) |
— |
— |
p-Coumaroylmalic acid isomer 1 |
y = 22 757 × (p-coumaric acid equivalent) + 1986.2 |
0.997 |
11.9 |
279.0581 |
163.0449 (100) |
133.0183 (5) |
119.0549 (16) |
p-Coumaroylmalic acid Isomer 2 |
y = 22 757 × (p-coumaric acid equivalent) + 1986.2 |
0.997 |
12.3 |
279.0558 |
163.0447 (64) |
133.0190 (100) |
119.0544 (18) |
Isoferulic acid |
y = 17 500 × (ferulic acid equivalent) + 2998.2 |
0.992 |
12.5 |
193.0531 |
134.0410 (100) |
178.0306 (2) |
— |
2.6 Determination of antioxidant activity
The oxygen radical absorbance capacity (ORAC) assay was performed.15 Briefly, the reaction was carried out at 40 °C in black polystyrene 96-well microplates (Nunc, Denmark) using 75 mM phosphate (PBS) buffer (pH 7.4); the final assay mixture contained 120 μL of fluorescein (116.7 nM), 60 μL of AAPH (48 nM), and 20 μL of antioxidant (Trolox or sample). The fluorescence was recorded over 97 min in a FluoSTAR OPTIMA microplate reader (BMG Labtech, Offenburg, Germany), with 485 nm excitation and 520 nm emission filters. The equipment was controlled by the FluoSTAR Control software version 1.32 R2 for fluorescence measurement. All reaction mixtures were prepared in duplicate and at least three independent runs were performed for each sample. The antioxidant activity of extracts was expressed as milligrams of Trolox equivalents per gram of dry matter (mg per g DM).
2.7 Determination of antihypertensive activity
The antihypertensive activity was determined by the angiotensin-I converting enzyme (ACE) inhibition assay with some modifications.16 To each black polystyrene 96-well microplate (Nunc, Denmark), 160 μL of fluorescent substrate Abz-Gly-Phe(NO2)-Pro in Tris-HCl buffer and 40 μL of each extract with different concentrations were added. The enzyme reaction was initiated by the addition of 2 mU of ACE (peptidil-dipeptidase A, EC 3.4.15.1), dissolved in glycerol (50%) and prepared in Tris-HCl buffer solution (150 mM) with 0.1 mM of ZnCl2, at pH 8.3 and were immediately mixed and incubated at 37 °C. The generated fluorescence was measured after 30 min by using a multiscan microplate fluorimeter, using the software FluoSTAR Control version 1.32 R2. The excitation and emission wavelengths were 350 and 420 nm, respectively. The antihypertensive activity of the extracts was expressed as percentage of the inhibition of the angiotensin-I converting enzyme (%).
2.8 Determination of the genotoxicity of extracts by DNA assay
The method of DNA using electrophoresis was used to access the level of denaturation of DNA when in the presence of the extract from where the antioxidant protection and pro-oxidant activity can be envisaged.17
2.8.1. DNA protection assessment (antioxidant assay).
The DNA solution (0.25 mg mL−1) was incubated in the presence of two degradation systems: (i) H2O2 50%, v/v and (ii) H2O2 50%, v/v with 10 mM FeCl3 and varying concentrations of extracts, with a range of volumes (400, 300, 200, and 100 μL), in PBS buffer. Also, the mixture of 400 μL of H2O2 with y μL of sample, 400–y μL of PBS and 200 μL of DNA, to a final volume of 1000 μL was done (when studying the FeCl3 system, 10 μL of 10 mM FeCl3were added, and this volume was discounted on PBS volume). The DNA solution without H2O2/FeCl3 was used as a positive control (no degradation). The mixture was made in duplicate for each extract. After 1 h incubation at 37 °C in the dark, agarose gel electrophoresis was run (see section 2.8.3).
2.8.2. DNA degradation assessment (pro-oxidant assay).
The DNA solution (0.25 mg mL−1) was incubated in the presence/absence of 10 mM FeCl3 and varying concentrations of extracts, with a range of volumes (400, 300, 200, and 100 μL), in PBS buffer. Also, the mixture of y μL of sample, 800–y μL of PBS, and 200 μL of DNA, to a final volume of 1000 μL was done (when studying the FeCl3 system, 10 μL of 10 mM FeCl3 were added, and this volume was discounted on PBS volume). The DNA solution without FeCl3 was used as a positive control (no degradation) for the assays using the FeCl3 system. The mixture was made in duplicate for each extract. After 1 h incubation at 37 °C in the dark, agarose gel electrophoresis was run (see section 2.8.3).
2.8.3. Electrophoresis.
Each sample was mixed in the ratio of 1
:
4 with the loading buffer (25 mg of bromophenol blue, 10 mL of Tris EDTA (TE) buffer 1× pH 8.0, and 20 mL of glycerol, pH 8.0) and 10 mL aliquots were transferred into a 0.75% (w/v) agarose gel prepared using Tris-Acetate EDTA buffer (TAE) supplemented with 0.03 mL mL−1 GreenSafe Premium. Electrophoresis was then run for 1.25 h at 150 mV. The gels were analysed using a molecular imager GelDOC XR+ (BioRad, Hercules, California, USA) and the resulting image was processed using Image Lab Software v5.1 (BioRad, Hercules, California, USA). The results were given as the percentage of inhibition of the DNA band degradation (for the antioxidant assay) (see eqn (1)) or as percentage of DNA band degradation (for the pro-oxidant assay) (see eqn (2)) | 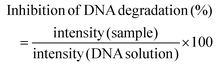 | (1) |
| 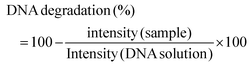 | (2) |
where intensity (sample) is the intensity of each sample band, and intensity (DNA solution) refers to the intensity of the intact DNA solution (positive control).
2.9 Cellular assays
2.9.1 Cell culture.
Human colon carcinoma (Caco-2) cells were obtained from the European Collection of Authenticated Cell Cultures (ECACC 8601020 and were grown using high glucose (4.5 g L−1) DMEM supplemented with 10% (v/v) heat inactivated FBS, 1% (v/v) penicillin–streptomycin–fungizone (Lonza, Verviers, Belgium), and 1% (v/v) of non-essential amino acids 100× (Sigma, Germany). Human keratinocyte cell line (HaCat) was obtained from Cell Line Services (Appenheim, Denmark) and was cultivated in DMEM with 4.5 g L−1 glucose, L-glutamine without pyruvate containing 10% (v/v) FBS and 1% (v/v) penicillin–streptomycin–fungizone (Lonza, Verviers, Belgium). All cells were incubated at 37 °C in a humidified atmosphere with 5% CO2. Caco-2 cells were assayed between passages 50 and 52 and HaCat cells were assayed between passages 77 and 80.
2.9.2 Cytotoxicity assay.
The cytotoxic potential of produced extracts was assessed for the cell lines using the XTT colorimetric assay in accordance with ISSO 10993-5. Briefly, cells were seeded at 1 × 105 cells per mL in the wells of a 96 well microplate and allowed to adhere. After 24 h, the media was removed, the cells were washed with PBS. Following this media with extracts at various concentrations (1.0 and 5.0 mg mL−1) were added. After 24 h, 25 μL of XTT were added to each well and the cells were incubated, in the dark, for 2 h. The optical density (OD) at 485 nm was then measured using a microplate reader (FLUOstar, OPTIMA, BMG Labtech, Ortenberg, Germany). The impact of extracts upon the cellular metabolism was quantified according to the following formula (eqn (3)): | 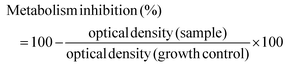 | (3) |
All extracts were assessed through two individual experiments executed within a week of each other. In each individual experiment all conditions were assayed in quintuplicate.
2.10. Statistical analysis
Each parameter was studied in triplicate and three independent samples were analyzed each time, except for cytotoxicity, for which five measurements were done for each sample. Statistical analysis of the results was performed using one-way analysis of variance (ANOVA) followed by Tukey's HSD test, at a 5% level of significance using the Minitab Statistical Software v.17.0. The Pearson correlations were evaluated by the Pearson's correlation coefficient (R) and the statistical significance of the coefficient (p-value) was evaluated using Minitab Statistical Software v.17.0. The results are expressed as mean ± standard deviation.
3 Results and discussion
3.1 Individual compounds
The phenolic composition of herbs can be affected by several factors, such as variety and genotype of the plant, climate, harvest time, storage, processing or even the treatment of the plant.18 The total phenolic content of the extracts under study has already been described and discussed (data submitted). Generally, the control extracts (extraction at 0.1 MPa, using 0–70% ethanol as the solvent) had a lower concentration of phenolics than the extracts obtained after HPE (Table 3), showing the highest total phenolic content registered for aqueous extracts after HPE at 200 MPa (585.26 ± 26.57 mg GAE per 100 g DM). In the eight extracts under study it was possible to identify and quantify 12 phenolic compounds and among the phenolic acids, isoferulic acid was the one with a higher concentration (p < 0.05), with values ranging from 1.59 ± 0.19 to 116.56 ± 11.68 mg per 100 g, while p-coumaroylmalic acid isomers and chlorogenic acid were found at lower levels (p < 0.05) (Table 3). Concerning the flavonoids, rutin was the only one, which was detected and quantified (see Tables 2 and 3), mainly in ethanolic extracts (N200/10.6/35, N0.1/10.6/35, N200/10.6/70, and N0.1/10.6/70). Several studies indicate that a high content of hydroxycinnamic acids, such as chlorogenic and caffeic acids, as well as flavonoids, is an important feature of an extract since these compounds are highly correlated with antioxidant, anti-inflammatory, antimicrobial, and anticancer activities.19
Table 3 Quantitative profile of phenolic and flavonoid compounds found in stinging nettle extracts. The results are shown as mg per 100 g DM. Different letters indicate significant differences (p < 0.05) between extracts for each condition set (differences analysed by row), i.e., values with the same letters are not significantly different from each other. Values with different letters are significant different from each other
Identification of extracts |
200 MPa |
0.1 MPa |
500 MPa |
0.1 MPa |
200 MPa |
0.1 MPa |
200 MPa |
0.1 MPa |
10.6 min |
10.6 min |
10.0 min |
10.0 min |
10.6 min |
10.6 min |
10.6 min |
10.6 min |
0% ethanol |
0% ethanol (control) |
25% ethanol |
25% ethanol (control) |
35% ethanol |
35% ethanol (control) |
70% ethanol |
70% ethanol (control) |
n.d. means that the compound was not detected. |
Total phenolic compounds
|
585.3 ± 26.57 |
255.8 ± 30.87 |
285.7 ± 23.81 |
81.44 ± 10.10 |
174.2 ± 6.795 |
80.70 ± 8.068 |
398.1 ± 71.38 |
111.8 ± 4.301 |
Phenolic acids
|
Neochlorogenic acid (3-caffeoylquinic acid) |
n.d. |
n.d. |
n.d. |
n.d. |
0.28 ± 0.06c |
n.d. |
23.77 ± 3.04a |
9.39 ± 1.19b |
Caftaric acid |
n.d. |
n.d. |
1.77 ± 0.22c |
n.d. |
1.38 ± 0.03c |
n.d. |
6.55 ± 0.38a |
3.38 ± 0.30b |
5-p-Coumaroylquinic acid |
3.05 ± 0.32d |
2.68 ± 0.01d |
12.60 ± 1.08b |
n.d. |
18.27 ± 1.75a |
13.10 ± 0.17b |
21.02 ± 1.11a |
7.63 ± 0.90c |
Chlorogenic acid (5-caffeoylquinic acid) |
n.d. |
n.d. |
0.40 ± 0.08c |
n.d. |
1.56 ± 0.44c |
n.d. |
108.04 ± 7.30a |
40.99 ± 4.32b |
Fertaric acid |
2.83 ± 0.07b |
1.85 ± 0.16b |
6.92 ± 1.10a |
n.d. |
7.47 ± 1.45a |
3.14 ± 0.04b |
9.90 ± 1.36a |
2.87 ± 0.08b |
4-p-Coumaroylquinic acid |
n.d. |
n.d. |
10.82 ± 0.80b |
n.d. |
10.28 ± 1.41b |
8.09 ± 0.38bc |
14.87 ± 1.72a |
6.26 ± 0.27c |
2-O-Caffeoylmalic acid |
n.d. |
n.d. |
5.71 ± 0.12b |
n.d. |
7.84 ± 0.30b |
n.d. |
49.86 ± 2.91a |
9.72 ± 0.15b |
p-Coumaric acid |
10.27 ± 0.23a |
6.36 ± 0.46b |
n.d. |
n.d. |
n.d. |
n.d. |
n.d. |
n.d. |
p-Coumaroylmalic acid Isomer 1 |
12.20 ± 0.85bc |
10.21 ± 0.20c |
15.28 ± 1.47b |
n.d. |
29.30 ± 1.12a |
15.84 ± 1.08b |
15.98 ± 1.43b |
6.24 ± 0.26d |
p-Coumaroylmalic acid Isomer 2 |
4.76 ± 0.13bc |
4.31 ± 0.14bcd |
2.80 ± 0.14cd |
n.d. |
8.14 ± 0.62a |
6.15 ± 0.91ab |
7.85 ± 1.31a |
2.25 ± 0.12de |
Isoferulic acid |
36.65 ± 3.49c |
34.56 ± 1.72c |
96.92 ± 11.72a |
1.59 ± 0.19d |
116.56 ± 11.68a |
67.83 ± 0.43b |
113.36 ± 4.06a |
59.27 ± 10.90bc |
Flavonoids
|
Rutin |
n.d. |
n.d. |
0.71 ± 0.03c |
n.d. |
1.28 ± 0.03c |
0.22 ± 0.03c |
64.88 ± 4.45a |
30.19 ± 0.40b |
N200/10.6/0 (extract obtained at 200 MPa, 0% ethanol) was the only one where p-coumaric acid could be found, at a concentration of 10.27 ± 0.23 mg per 100 g DM (Table 3). Also Pinelli et al. (2008)20 were able to identify p-coumaric acid in fresh nettle samples (after an extraction at room temperature, using 70% ethanol
:
water (v/v) as the solvent) at a concentration of 5.20 mg per100 g. This indicates that HPE can effectively perform the extraction of some phenolic compounds with no need of toxic organic solvents for extraction. Furthermore, the extracts obtained after HPE at 200 MPa, using 35 and 70% of ethanol, were the ones with more individual compounds identified (Table 3). This is probably due to the non-polar nature of phenolic compounds.21 Nevertheless, it is interesting to note that although the extract N200/10.6/0 was the one with a higher concentration of total phenolic compounds, it was the extract N200/10.6/70 which presented a higher quantity of individual compounds such as chlorogenic, caftaric, and fertaric acids and rutin (Table 3). The chromatograms of all samples were recorded at 320 nm and indicated the presence of several individual phenolic compounds in different concentrations. Isoferulic acid was the only compound identified in all studied extracts (Table 3). The highest concentration (p < 0.05) of isoferulic acid was determined in N200/10.6/35 (116.56 ± 11.68 mg per 100 g DM) and the lowest concentration in N0.1/10/25 (1.59 ± 0.19 mg per 100 g DM), indicating a clear improvement of individual compound extraction using HPE (Table 3). Chlorogenic and neochlorogenic acids, known for their cardioprotective and anti-diabetic effects were only identified in extracts N200/10.6/35, N200/10.6/70, and N0.1/10.6/70 (extracts with 35 and 70% ethanol) (Table 3). The highest value (p < 0.05) was registered for extracts obtained at 200 MPa, 70% ethanol (N200/10.6/70) with a value of 108.04 ± 7.30 mg per 100 g DM (Table 3). These results are according to the ones obtained by Orcic et al. (2014),2 who reported a concentration of 123 mg per 100 g of chlorogenic acid after an extraction with methanol for 48 hours using nettle leaves. These results allow us to conclude that HPE is an effective and fast methodology for the extraction of individual compounds from stinging nettle leaves. Relative to rutin, the flavonoid found in the highest concentration (p < 0.05) in the extracts (Table 3) showed the highest concentration once more in N200/10.6/70 (64.88 ± 4.45 mg per 100 g DM). These results were a clear improvement compared to the results of Pinelli et al. (2008),20 Otles and Yalcin, (2012),22 and Zenão et al. (2017)19 who obtained, with 70% ethanol and 80% methanol as solvents, a rutin concentration of 17.30, 19.11, and 10.60 mg per 100 g, respectively.
3.2 Antioxidant activity
Different assays are frequently used to estimate the antioxidant capacities of several compounds, such as ABTS˙+ (2,2-azinobis (3-ethyl-benzothiazoline-6-sulfonic acid)) and DPPH˙+ (2,2-diphenyl-1-picrylhydrazyl) radical scavenging assays, FRAP (ferric reducing antioxidant power), and ORAC (oxygen radical antioxidant capacity) methods. Although ABTS˙+ and DPPH˙+ assays are easier and faster to use when screening for antioxidant properties, they lack biological context, since they typically disregard the molecules that the antioxidants could be protecting, such as the DNA molecule; some authors state that the ORAC assay is considered to be more pertinent and that it better resembles the biological systems.23,24 This method measures the ability of antioxidant compounds present in the extracts under study to protect a fluorescent molecule from damage by free radicals. Also, the ORAC assay is known for mimicking the antioxidant activity of phenolic compounds in biological systems, since it uses biologically relevant free radicals and integrates both time and the degree of antioxidant activity.25
The antioxidant capacity of the studied extracts was quantified by ORAC assay. In Table 4 it is possible to observe that among the aqueous extracts (N200/10.6/0 (HPE) and N0.1/10.6/0 (control)) and 25% ethanol
:
water extracts (N500/10/25 (HPE) or N0.1/10/25 (control)) there were no significant differences (p > 0.05). Nevertheless, when analysing the extracts obtained using 35 and 70% ethanol (N200/10.6/35 and N0.1/10.6/70), it is possible to observe a clear improvement (p < 0.05) of antioxidant capacity after stinging nettle leaves were subjected to HPE (Table 4). For extracts with 35% ethanol as the solvent, an increase (p < 0.05) of about 260% was observed (from 73.98 ± 2.860 to 266.9 ± 6.086 mgTrolox g−1 DM, when comparing extracts obtained at atmospheric pressure and by HPE, respectively) (Table 4). For the extracts with 70% ethanol, the increase (p < 0.05) was about 121% (from 114.2 ± 7.629 to 252.9 ± 18.12 mgTrolox g−1 DM for N0.1/10.6/70 and N200/10.6/70, respectively) (Table 4). To the best of our knowledge, this is the first study where this analysis is fully reported for stinging nettle. Nevertheless, Skąpska et al. (2017)26 reported a nettle extract (250 mg L−1) with an antioxidant capacity of 187.4 ± 50.6 mgTrolox g−1, which was used as an additive in a fruit drink. This allows us to corroborate the fact that stinging nettle has a high antioxidant capacity and when the extraction occurs by high pressure, the extracts present even a greater capacity than when obtained at atmospheric pressure. These results can also be correlated with the individual compound profile present in the extracts (see Table 5), since the extracts with higher antioxidant activity (N200/10.6/35 and N200/10.6/70) were also the extracts which presented higher concentrations of 5-p-coumaroylquinic acid and 5-O-caffeoylquinic acid (Table 3), compounds that were already associated with a high antioxidant power.2,27
Table 4 Antioxidant activity measured by ORAC assay and for antihypertensive activity by IACE inhibition assay; genotoxicity (expressed in percentage of DNA degradation inhibition for antioxidant activity and percentage of DNA degradation for pro-oxidant activity) and in vitro cytotoxicity (value presented obtained through two individual experiments each with 5 individual replicates per sample). Different letters indicate significant differences (p < 0.05) between extracts for each assay (differences analysed by column), i.e., values with the same letters are not significantly different from each other. Values with different letters are significant different from each other
Identification of extracts |
Antioxidant activity (ORAC assay) (mg Trolox g−1 DM) |
Antihypertensive activity (IACE assay) (%) |
Genotoxicitya |
Cytotoxicity |
Antioxidant activity (%) |
Pro-oxidant activity (%) |
With FeCl3 |
Without FeCl3 |
With FeCl3 |
Without FeCl3 |
Caco-2 (metabolism inhibition %) |
HaCat (metabolism inhibition %) |
Results presented only for the higher concentrations (in mg DM per mL) tested for each extract: extract 1: 5.06; extract 2: 5.12; extract 3: 4.77; extract 4: 4.87; extract 5: 4.94; extract 6: 4.94; extract 7: 4.72; and extract 8: 4.71.
|
N200/10.6/0 |
200 MPa |
53.74 ± 9.185d |
92.03 ± 0.1361a |
111.4 ± 13.19a |
13.0 ± 3.61de |
16.6 ± 1.30ab |
21.1 ± 18.0ab |
−6.35 ± 1.37ab |
10.88 ± 2.92ab |
10.6 min |
0% ethanol |
N0.1/10.6/0 (control) |
0.1 MPa |
50.03 ± 3.518d |
86.17 ± 1.820a |
57.6 ± 6.26bc |
19.5 ± 2.06cd |
42.7 ± 2.24a |
30.6 ± 9.27ab |
5.14 ± 4.25a |
13.63 ± 0.53a |
10.6 min |
0% ethanol |
N500/10/25 |
500 MPa |
95.35 ± 10.22bc |
86.82 ± 4.129a |
59.3 ± 6.11b |
7.5 ± 2.25e |
−31.7 ± 18.3c |
−7.08 ± 6.33c |
−30.3 ± 4.14c |
10.83 ± 2.82ab |
10.0 min |
25% ethanol |
N0.1/10/25 (control) |
0.1 MPa |
96.23 ± 9.052bc |
70.46 ± 3.954b |
20.2 ± 0.881c |
11.4 ± 2.58e |
36.9 ± 10.5a |
40.1 ± 5.87a |
−5.09 ± 2.60ab |
6.31 ± 2.31b |
10.0 min |
25% ethanol |
N200/10.6/35 |
200 MPa |
266.9 ± 6.086a |
82.53 ± 3.702a |
148.6 ± 30.57a |
48.5 ± 2.89a |
−8.77 ± 6.59bc |
13.5 ± 3.87bc |
−25.9 ± 4.16c |
14.12 ± 1.53a |
10.6 min |
35% ethanol |
N0.1/10.6/35 (control) |
0.1 MPa |
73.98 ± 2.860cd |
69.75 ± 1.792b |
50.2 ± 4.83c |
34.3 ± 5.74b |
33.0 ± 4.94a |
30.2 ± 5.99ab |
5.24 ± 4.22a |
9.70 ± 3.06ab |
10.6 min |
35% ethanol |
N200/10.6/70 |
200 MPa |
252.9 ± 18.12a |
85.45 ± 1.715a |
124.3 ± 28.91a |
22.9 ± 2.30c |
−15.4 ± 12.3c |
6.45 ± 20.34bc |
−26.0 ± 5.01c |
— |
10.6 min |
70% ethanol |
N0.1/10.6/70 (control) |
0.1 MPa |
114.2 ± 7.629b |
67.66 ± 1.598b |
59.0 ± 5.58b |
23.9 ± 2.85c |
23.7 ± 13.6a |
12.0 ± 7.22abc |
−9.46 ± 5.36b |
— |
10.6 min |
70% ethanol |
Table 5 Pearson correlations (R value) and respective p-value in parenthesis. It should be noted that the table is labelled with a colour code (only the significant correlations (p < 0.05) are highlighted in grey cells, and more intense colour represents the highest correlation values (three levels: light grey: R-value below 0.650; medium grey: R-value from 0.650 to 0.750; dark grey: R-value above 0.750)
3.3 Antihypertensive activity
Hypertension can also be called arterial high blood pressure, and it refers to the pressure acting on the walls of the arteries, which is the major risk for cardiovascular accidents in the world. Hypertension has the characteristic of high pressure indicators, either equal to/higher than 140 mmHg for systolic and 90 mmHg for diastolic pressure.28 Also, hypertension can be characterized by a permanent state of oxidative stress, resulting from the lack of equilibrium between the generation/destruction of reactive oxygen species.29 One of the cellular paths to achieve a lowering of blood pressure is to use angiotensin II type I receptor antagonists and angiotensin converting enzyme (ACE) inhibitors, which leads to vasoconstriction relaxation and the release of renal sodium ions. Nevertheless, there are a few studies concerning the study of ACE inhibitory activity of plant species, and this being the first study reporting on stinging nettle.
Similar to the results obtained for the ORAC assay, all the extracts obtained at atmospheric pressure present a high antihypertensive activity, with values above 68% for the inhibition for all extracts (Table 4). Nevertheless, after subjecting the nettle extracts to HPE, a clear improvement of this property was observed, except for aqueous extracts, where no significant difference (p > 0.05) was observed between the HPE and the controls (Table 4). For example, for N200/10.6/70 and N0.1/10.6/70 (70% ethanol, HPE and control, respectively) an increase was observed (p < 0.05) of 26.3%, from 67.7 ± 1.60 to 85.5 ± 1.72% ACE inhibition (Table 4). These results can be correlated to the content of phenolic compounds and flavonoids described in section 3.1, since a significant (p < 0.05) Pearson correlation (value of 0.835) (Table 5) between the ACE inhibition activity and the concentration of total phenolic compounds from the extracts was found. It is also noteworthy that the extracts that presented a greater improvement after HPE (70% ethanol) were also the ones which presented a higher concentration of flavonoids such as rutin, and phenolic acids, such as chlorogenic acid (see Table 5). Another important note about extracts presenting high ACE inhibitory activity and the presence of particular phenolic compounds is the particularly high concentration of fertaric and ferulic acids present in N200/10.6/0, N0.1/10.6/0, N500/10/25, N200/10.6/35 and N200/10.6/70 (with ACE inhibition above 82%) (Table 3); high Pearson correlation values were also found between these variables (Table 5). These results may indicate that there is a correlation between these factors which has still not been reported in the literature, since only flavonoids and coumarins from plant materials are reported to have hypotensive effects.30,31 Furthermore, some studies have reported that supplementation of ferulic acid to spontaneously hypertensive rats can have beneficial effects,32 and a study developed by Vajic et al. (2018)33 reported that periodical supplementation of nettle extracts to hypertensive rats (10–200 mg kg−1 day−1) allowed reducing both systolic and diastolic blood pressure, as well as the cardiac index.
3.4 DNA antioxidant protection and pro-oxidant activity
The efficiency of the different extracts in preventing DNA damage induced by the presence of H2O2 (oxidation) was determined by the DNA assay, using electrophoresis to access the level of denaturation of DNA when in the presence of the extract (maximum concentration of ∼5.0 mg DM per mL) (see Fig. 1). Two distinct activities can be evaluated by this assay, the antioxidant activity (measuring the level of inhibition of DNA denaturation) and pro-oxidant activity (measuring the level of DNA denaturation). The addition of FeCl3 to the damaging system composed of H2O2 allowed studying the extract effects on a more relevant biological standpoint. The combination of H2O2 and FeCl3 leads to a greater damage caused to the DNA molecule than using H2O2 alone (indicating that H2O2, in spite of being considered a reactive oxygen species, has limited DNA damage inducing capabilities on its own).17
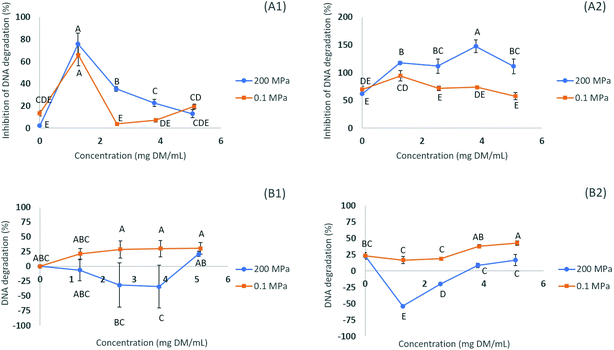 |
| Fig. 1 Antioxidant activity, i.e. prevention of DNA oxidation (by H2O2 (A1) or and H2O2/FeCl3 system (A2)). Pro-oxidant activity (in the absence (B1) and presence (B2) of iron cations) for aqueous extracts (N200/10.6/0 and N0.1/10.6/0). | |
When the extracts were added at a concentration of ∼5.0 mg DM per mL in the presence of FeCl3, the DNA damage protective effect (antioxidant assay) measured ranged from 59.3 to 148.6% (p < 0.05), the order being N200/10.6/35 > N200/10.6/70 > N200/10.6/0 > N500/10/25, for the extracts subjected to HPE, while for the controls the higher inhibition of DNA denaturation was 59.0% for the N0.1/10.6/70 (70% ethanol) (Table 4). It is noteworthy that, in some cases, the values for the inhibition of DNA degradation appear above 100%, indicating a higher fluorescence intensity of the band after exposure to the extract compounds which may indicate the possibility of some interactions between the DNA molecule and the compounds.17 Relative to the pro-oxidant assay, the DNA damage in the presence of FeCl3 occurred in a range from −31.7 to 16.6% (p < 0.05) for the extracts subjected to HPE, while for the controls, the lower value was 23.7% for the N0.1/10.6/70 (Table 4). As can be seen in Fig. 1 (B1 and B2) all the tested concentrations of the N200/10.6/0 (submitted to HPE at 200 MPa) presented a lower DNA degradation rate than the control (N0.1/10.6/0) using the same concentrations. These results can be correlated to the concentration of individual compounds found in each extract (see Table 5), since chlorogenic acid and its isomers are known for their DNA-protective activities, and N200/10.6/70 is the richest of all extracts, being the one with a higher concentration of chlorogenic acid (5-caffeoylquinic acid) and neochlorogenic acid (3-caffeoylquinic acid) (Table 3).27 This indicates that HPE allows one to obtain extracts with improved DNA protective properties, when compared to the controls at 0.1 MPa and with no pro-oxidant activity.
3.5 Cytotoxicity assays
The Caco-2 cell line is a continuous line of heterogeneous human epithelial colorectal adenocarcinoma cells usually being used as a cellular model for the evaluation of the cytotoxic effect of several extracts, since, when confluent, these cells mimic the intestinal epithelium. The HaCaT cell line is composed of immortalized human keratinocytes and has been extensively used to study epidermal homeostasis and to access the potential biocompatibility of extracts as a cosmetic ingredient. The results in the present work demonstrated that the nettle extracts at a concentration of 1.0 mg DM per mL obtained by HPE did not exert any inhibition of the cellular metabolism of Caco-2 cells, appearing to, in fact, stimulate it, exhibiting negative values for the percentage of metabolism inhibition (ranging from −30.3 to −6.4%) (see Table 4). When compared to the viability of cells cultured in the absence of extracts (0 mg lyophilized extract per mL −1.74 ± 0.18% metabolism inhibition), Caco-2 cells cultured in the presence of different concentrations of HPE extracts demonstrated higher metabolic activity in comparison with the positive control (culture medium and cells), which can be attributed to the supplementation of the culture media with nutrients from the extracts.34 For HaCat cells, all the extracts at 1.0 mg mL−1 led to some metabolism inhibition (below 20%), with HPE extracts (N200/10.6/0, N500/10/25 and N200/10.6/35) presenting slightly higher metabolism inhibition values than the controls, although not statistically significant (p > 0.05) (Table 4). These results may indicate that, although not significantly high, stinging nettle extracts could present some antitumor activity. Nevertheless, more research should be performed to test this hypothesis.
In Table 5 it is possible to observe that there is a strong significant (p < 0.05) correlation between the cytotoxicity of extracts and the compound 2-O-caffeoylmalic acid, while a more moderate correlation appears for the p-coumaroylmalic acid isomer 1, indicating that a slight metabolism inhibition for Caco-2 cells occurs probably due to the presence of phenolic compounds in the extracts.
Furthermore, when comparing these results to those of the controls, it can be seen that the presence of extracts obtained at 0.1 MPa led to lower inhibition percentages (with values ranging from −9.5 to 5.2%) (p > 0.05). Extracts from stinging nettle have already been reported as not cytotoxic against HepG2, Hep2c, RD and L2OB cell lines.35,36
4 Conclusion
The main results of the present work indicate that stinging nettle leaves have relevant biological activities, such as antioxidant and antihypertensive activities. The extracts obtained at 200 MPa, 10 min, 35 and 70% ethanol, were the ones which presented higher concentrations of phenolic acids (5-O-caffeoylquinic acid, and fertaric and isoferulic acid) and flavonoids (rutin), these compounds being related to the highest antioxidant, DNA-protective, and antihypertensive activities. These results scientifically validate the ancient application of stinging nettle in traditional medicine and demonstrate that emerging technology may be used to obtain more active and safer extracts (either in food or cosmetic applications). These bioactive extracts require in vivo studies to validate the positive in vitro properties explored in this study, and the strong antioxidant activity opens the need for further research concerning other biological activities still yet to be discovered.
Conflicts of interest
There are no conflicts of interest to declare.
Acknowledgements
The authors are thankful to the University of Aveiro and FCT/MCT for financial support for the QOPNA research Unit (FCT UID/QUI/00062/2019) and CBQF (FCT UID/Multi/50016/2019) through national founds and, where applicable, co-financed by the FEDER, within the PT2020 Partnership Agreement. Silvia A. Moreira is grateful for the financial support from FCT through the Doctoral Grant SFRH/BD/110430/2015.
References
- J. Giacometti, D. Bursać Kovačević, P. Putnik, D. Gabrić, T. Bilušić, G. Krešić, V. Stulić, F. J. Barba, F. Chemat, G. Barbosa-Cánovas and A. Režek Jambrak, Extraction of bioactive compounds and essential oils from mediterranean herbs by conventional and green innovative techniques: A review, Food Res. Int., 2018, 113, 245–262 CrossRef CAS.
- D. Orcic, M. Franciskovic, K. Bekvalac, E. Svircev, I. Beara, M. Lesjak and N. Mimica-Dukic, Quantitative determination of plant phenolics in Urtica dioica extracts by high-performance liquid chromatography coupled with tandem mass spectrometric detection, Food Chem., 2014, 143, 48–53 CrossRef CAS PubMed.
- M. K. Akalın, S. Karagöz and M. Akyüz, Application of response surface methodology to extract yields from stinging nettle under supercritical ethanol conditions, J. Supercrit. Fluids, 2013, 84, 164–172 CrossRef.
-
I. O. Minatel, C. V. Borges, M. I. Ferreira, H. A. G. Gomez, C.-Y. O. Chen and G. P. P. Lima, in Phenolic Compounds - Biological Activity, Intechopen, 2017, ch. 1, DOI: DOI:10.5772/66368.
-
W. Vermerris and R. Nicholson, in Phenolic Compound Biochemistry, Springer, Netherlands, 2006, ch. 7 Search PubMed.
- O. V. Zillich, U. Schweiggert-Weisz, P. Eisner and M. Kerscher, Polyphenols as active ingredients for cosmetic products, Int. J. Cosmetic Sci., 2015, 37, 455–464 CrossRef CAS PubMed.
- H. S. Lee, H. J. Lee, H. J. Yu, W. Ju do, Y. Kim, C. T. Kim, C. J. Kim, Y. J. Cho, N. Kim, S. Y. Choi and H. J. Suh, A comparison between high hydrostatic pressure extraction and heat extraction of ginsenosides from ginseng (Panax ginseng CA Meyer), J. Sci. Food Agric., 2011, 91, 1466–1473 CrossRef CAS PubMed.
- K. N. Prasad, E. Yang, C. Yi, M. Zhao and Y. Jiang, Effects of high pressure extraction on the extraction yield, total phenolic content and antioxidant activity of longan fruit pericarp, Innov. Food Sci. Emerg. Technol., 2009, 10, 155–159 CrossRef CAS.
- H.-W. Huang, C.-P. Hsu, B. B. Yang and C.-Y. Wang, Advances in the extraction of natural ingredients by high pressure extraction technology, Trends Food Sci. Technol., 2013, 33, 54–62 CrossRef CAS.
- X. He, W.-B. Yoon, S.-J. Park, D.-S. Park and J. Ahn, Effects of pressure level and processing time on the extraction of total phenols, flavonoids, and phenolic acids from Deodeok (Codonopsis lanceolata), J. Food Sci. Biotechnol., 2011, 20, 499–505 CrossRef CAS.
-
US-FDA, Kinetics of microbial inactivation for alternative food processing technologies - high pressure processing, http://www.fda.gov/Food/FoodScienceResearch/SafePracticesforFoodProcesses/ucm101456.htm.
-
E. Hogan, A. L. Kelly and D.-W. Sun, in Emerging Technologies for Food Processing, ed. D.-W. Sun, Academic Press, London, 2005, DOI:10.1016/B978-012676757-5/50003-7, ch. 1, pp. 3–32.
- A. Belscak-Cvitanovic, D. Komes, K. Durgo, A. Vojvodic and A. Busic, Nettle (Urtica dioica L.) extracts as functional ingredients for production of chocolates with improved bioactive composition and sensory properties, J. Food Sci. Technol., 2015, 52, 7723–7734 CrossRef CAS.
-
V. L. Singleton, R. Orthofer and R. M. Lamuela-Raventós, in Methods in Enzymology, Academic Press, 1999, vol. 299, ch. 14, pp. 152–178 Search PubMed.
- A. G. N. Amorim, J. M. T. Souza, R. C. Santos, B. Gullón, A. Oliveira, L. F. A. Santos, A. L. E. Virgino, A. C. Mafud, H. M. Petrilli, Y. P. Mascarenhas, C. Delerue-Matos, M. E. Pintado and J. R. S. A. Leite, HPLC-DAD, ESI–MS/MS, and NMR of lycopene isolated from P. guajava L. and its biotechnological applications, Eur. J. Lipid Sci. Technol., 2018, 120, 1700330 CrossRef.
- M. Ángel Sentandreu and F. Toldrá, A fluorescence-based protocol for quantifying angiotensin-converting enzyme activity, Nat. Protoc., 2006, 1, 2423 CrossRef PubMed.
- S. Silva, E. M. Costa, S. Vicente, M. Veiga, C. Calhau, R. M. Morais and M. E. Pintado, DNA agarose gel electrophoresis for antioxidant analysis: Development of a quantitative approach for phenolic extracts, Food Chem., 2017, 233, 45–51 CrossRef CAS PubMed.
- S. Dobrinas, G. Stanciu and S. Lupsor, Total phenolic content and HPLC characterization of some culinary herbs, J. Sci. Arts, 2017, 321–330 Search PubMed.
- S. Zenão, A. Aires, C. Dias, M. J. Saavedra and C. Fernandes, Antibacterial potential of Urtica dioica and Lavandula angustifolia, extracts against methicillin resistant Staphylococcus aureus isolated from diabetic foot ulcers, J. Herb. Med., 2017, 10, 53–58 CrossRef.
- P. Pinelli, F. Ieri, P. Vignolini, L. Bacci, S. Baronti and A. Romani, Extraction and HPLC analysis of phenolic compounds in leaves, stalks, and textile fibers of Urtica dioica L, J. Agric. Food Chem., 2008, 56, 9127–9132 CrossRef CAS PubMed.
- D. Lin, M. Xiao, J. Zhao, Z. Li, B. Xing, X. Li, M. Kong, L. Li, Q. Zhang, Y. Liu, H. Chen, W. Qin, H. Wu and S. Chen, An overview of plant phenolic compounds and their importance in human nutrition and management of type 2 diabetes, Molecules, 2016, 21, 1374 CrossRef PubMed.
- S. Otles and B. Yalcin, Phenolic compounds analysis of root, stalk, and leaves of nettle, Sci. World J., 2012, 2012, 1–12 CrossRef PubMed.
- K. Thaipong, U. Boonprakob, K. Crosby, L. Cisneros-Zevallos and D. Hawkins Byrne, Comparison of ABTS, DPPH, FRAP, and ORAC assays for estimating antioxidant activity from guava fruit extracts, J. Food Compos. Anal., 2006, 19, 669–675 CrossRef CAS.
- R. L. Prior, H. Hoang, L. Gu, X. Wu, M. Bacchiocca, L. Howard, M. Hampsch-Woodill, D. Huang, B. Ou and R. Jacob, Assays for hydrophilic and lipophilic antioxidant capacity (oxygen radical absorbance capacity (ORAC(FL)) of plasma and other biological and food samples, J. Agric. Food Chem., 2003, 51, 3273–3279 CrossRef CAS PubMed.
- C. Lucas-Abellan, M. T. Mercader-Ros, M. P. Zafrilla, J. A. Gabaldon and E. Nunez-Delicado, Comparative study of different methods to measure antioxidant activity of resveratrol in the presence of cyclodextrins, Food Chem. Toxicol., 2011, 49, 1255–1260 CrossRef CAS PubMed.
- S. Skąpska, K. Marszałek, Ł. Woźniak, K. Zawada and I. Wawer, Aronia dietary drinks fortified with selected herbal extracts preserved by thermal pasteurization and high pressure carbon dioxide, LWT-Food Sci. Technol., 2017, 85, 423–426 CrossRef.
- J.-G. Xu, Q.-P. Hu and Y. Liu, Antioxidant and DNA-protective activities of chlorogenic acid isomers, J. Agric. Food Chem., 2012, 60, 11625–11630 CrossRef CAS PubMed.
-
C. R. Foundation, Cardio-vascular illnesses - Arterial Hypertension, http://www.fondation-recherche-cardio-vasculaire.org/en/your-cardio-vascular-health/cardio-vascular-illnesses/arterial-hypertension/, (accessed 17/12/2019).
- A. Baradaran, H. Nasri and M. Rafieian-Kopaei, Oxidative stress and hypertension: Possibility of hypertension therapy with antioxidants, J. Res. Med. Sci., 2014, 19, 358–367 Search PubMed.
- A. Gasparotto Junior, F. M. Gasparotto, E. L. B. Lourenço, S. Crestani, M. E. A. Stefanello, M. J. Salvador, J. E. da Silva-Santos, M. C. A. Marques and C. A. L. Kassuya, Antihypertensive effects of isoquercitrin and extracts from Tropaeolum majus L.: Evidence for the inhibition of angiotensin converting enzyme, J. Ethnopharmacol., 2011, 134, 363–372 CrossRef CAS PubMed.
- W. D. Seo, J. Y. Kim, H. W. Ryu, J. H. Kim, S.-I. Han, J.-E. Ra, K. H. Seo, K. C. Jang and J. H. Lee, Identification and characterisation of coumarins from the roots of Angelica dahurica and their inhibitory effects against cholinesterase, J. Funct. Foods, 2013, 5, 1421–1431 CrossRef CAS.
- N. Kumar and V. Pruthi, Potential applications of ferulic acid from natural sources, Biotechnol. Rep., 2014, 4, 86–93 CrossRef PubMed.
- U.-J. Vajic, J. Grujic-Milanovic, Z. Miloradovic, D. Jovovic, M. Ivanov, D. Karanovic, K. Savikin, B. Bugarski and N. Mihailovic-Stanojevic, Urtica dioica L. leaf extract modulates blood pressure and oxidative stress in spontaneously hypertensive rats, Phytomedicine, 2018, 46, 39–45 CrossRef CAS PubMed.
- D. Rodrigues, A. R. Costa-Pinto, S. Sousa, M. W. Vasconcelos, M. M. Pintado, L. Pereira, T. A. P. Rocha-Santos, J. P. D. Costa, A. M. S. Silva, A. C. Duarte, A. M. P. Gomes and A. C. Freitas,
Sargassum muticum and Osmundea pinnatifida enzymatic extracts: chemical, structural, and cytotoxic characterization, Mar. Drugs, 2019, 17, 209 CrossRef CAS PubMed.
- A. Di Sotto, G. Mazzanti, N. Savickiene, R. Starselskyte, V. Baksenskaite, S. Di Giacomo and A. Vitalone, Antimutagenic and antioxidant activity of a protein fraction from aerial parts of Urtica dioica, Pharm. Biol., 2015, 53, 935–938 CrossRef CAS PubMed.
- Z. Zeković, A. Cvetanović, J. Švarc-Gajić, S. Gorjanović, D. Sužnjević, P. Mašković, S. Savić, M. Radojković and S. Đurović, Chemical and biological screening of stinging nettle leaves extracts obtained by modern extraction techniques, Ind. Crops Prod., 2017, 108, 423–430 CrossRef.
|
This journal is © The Royal Society of Chemistry 2020 |