Amyloid hybrid membranes for removal of clinical and nuclear radioactive wastewater†
Received
25th July 2020
, Accepted 28th August 2020
First published on 1st September 2020
Abstract
Nuclear medicine uses various radioactive compounds for the administration into patients to diagnose and treat diseases, which generates large amounts of radioactively contaminated water. Currently, radioactively contaminated hospital wastewater has to be stored until the contained radionuclides have sufficiently decayed because cost-effective and efficient removal technologies are not available. Similar considerations apply in the nuclear power industry, with, however, decay times of the radionuclides several orders of magnitude higher. Previously, we reported hybrid membranes composed of amyloid fibrils produced from cheap and readily available proteins and activated carbon, which efficiently removed heavy metal ions and radioactive compounds from water. Here, we show that these membranes are highly efficient in the adsorption & removal of diverse, clinically relevant radioactive compounds from hospital wastewater by single-step filtration. The radionuclides technetium (Tc-99m), iodine (I-123) and gallium (Ga-68) can be removed from water with efficiencies above 99.8% in one single step. We also demonstrate the purification of a real clinical wastewater sample from a Swiss hospital containing iodine (I-131) and lutetium (Lu-177). With the use of single-photon emission computed tomography (SPECT) and positron emission tomography (PET), we were able to visualize the accumulation of the radioactive compounds within the membrane and demonstrate its outstanding performance. By converting large volumes of radioactive wastewater into low volumes of solid radioactive waste, the present technology emerges as a possible game-changer in the treatment of nuclear wastewater.
Water impact
The use of radionuclides in nuclear medicine and nuclear power industry generates large amounts of radioactively contaminated liquids. Currently, radioactive wastewater has to be stored until the radionuclides have sufficiently decayed. This puts a substantial logistical and financial burden on many hospitals and nuclear power plants. This work reports hybrid membranes composed of milk protein amyloid fibrils and activated carbon which are highly efficient in the adsorption of radioactive wastewater and allow the conversion of large volumes of liquid radioactive waste into much smaller volumes of solid radioactive waste. The technology is scalable, cost-effective, completely sustainable and highly efficient.
|
1 Introduction
Nuclear medicine comprises the administration of radioactive substances1 into a patient to treat or visualize diseases, which are mostly cancer-related.2,3 These substances are either being used in their elemental form or they are incorporated into larger molecules.4,5 In a way, nuclear medicine can be considered as “endoradiology” because it utilizes radiation emitted from within the patient's body,6 opposite to radiology, where the patient is being exposed to radiation sources, coming from outside.
After the administration of nuclear medicine,7 the radionuclides leave the patient via excretion through their body fluids. Therefore, until having reached sufficiently low levels of radioactivity,8,9 all excretions of the patient have to be collected and treated as radioactive liquid waste.10,11 At the moment, there is no satisfactory solution to the problem of radioactive wastewater disposal.12–14 Depending on the nature of the radionuclides, hospitals rely on on-site storage of radioactively contaminated liquid waste until radionuclide decay, after which liquid waste has to be either conditioned (solidified) or suitably disposed depending on its hazard. Both processes are very costly. The Swiss Federal Office of Health calculates 94
000 CHF m−3 only for the conditioning and interim storage of liquid radioactive waste.15,16 Similar consideration, but on a much larger scale, apply to the storage and disposal of liquid radioactive waste in the nuclear power industry. Rahman et al. clearly described in their review about the liquid radioactive waste treatment technologies available for radioactive waste management.16 It summarizes the application of different conventional treatment processes (adsorption, ion exchange resins) and emerging technologies such as continuous electrodeionization (CEDI) technology and hydrothermal oxidation method. In both nuclear power plant and clinical radionuclide wastewater cases, traditional technologies such as reverse osmosis and nanofiltration cannot be used efficiently for the purification of radionuclides, as they lead to a many-fold increase of contaminant concentration in the residual backwater solution, thus producing lower volumes of liquid wastewater, which are, however, even more contaminated, presenting a dramatic threat to public health. It is still echoing the statement of the Japanese government announcing that they will have to dump by 2022 to the Pacific Ocean more than a million tons of radioactively contaminated water in storage since the Fukushima tragedy caused by the 2011 tsunami, due to a lack of space in nuclear radioactive liquid wastewater storage and the inefficiency of other technologies in handling radioactive liquid waste.17 The must for any remediation technology in radioactive waste, is to convert large volumes of radioactive liquid wastewater into much smaller volumes of solid radioactive waste, which is significantly easier to be stored and disposed.
In 2016, we reported a broadly applicable and highly efficient filtering system made of amyloid–carbon hybrid membranes,18 which were made of β-lactoglobulin, the major component of whey by-product of the dairy industry, and activated carbon.19 Initial studies have shown filtering efficacies of three to five orders of magnitude for a broad spectrum of heavy metal ions as well as for numerous other compounds including fluoride,20 organic pollutants21 and bacteria. These adsorption-based depth filter membranes were also found to be able to remove model radioactive waste such as uranyl acetate and phosphorus-32.18 Based on the outstanding performance of these membranes, the absence of secondary pollutants and notably the affordable and sustainable nature of the main components, active charcoal and by-product proteins from the cheese-making process, this technology is emerging as a game-changer in the broad water purification field, although the potential in the remediation of radioactive wastewater contamination remains to be assessed.
In our earlier reports, we demonstrated the generality of the approach by studying the filtering efficiency of various hybrid amyloid fibrils based on egg lysozyme fibrils, bovine serum albumin, whey protein isolate and β-lactoglobulin fibrils.18 Amyloid fibrils produced from the very inexpensive whey protein isolate (a by-product of the dairy industry and the industrial precursor of β-lactoglobulin) showed efficiencies comparable to those of purified β-lactoglobulin, at a significantly lower cost related to the waste nature of the original protein source, since no separation/purification steps are needed using this protein compared to β-lactoglobulin (obtained by dialysis from whey). Here we show that hybrid adsorption membranes for efficient removal efficiency of radionuclides can be produced in a scalable, easy and cost-effective way by employing whey protein fibrils instead of β-lactoglobulin.
Here, we demonstrate that amyloid–carbon hybrid membranes can provide a highly sought solution to the problem of hospital radioactive wastewater purification and nuclear wastewater in general. In order to demonstrate the feasibility of the approach in real context, we use membranes made not of pure β-lactoglobulin, as in our previous works, but made using the more affordable low-grade by-product whey protein. We test the purification performance of amyloid–carbon hybrid filters for various clinically relevant radioactive substances with different half-life periods such as Tc-99m,22–24 I-123,25,26 and Ga-68.27,28 Further real wastewater obtained from Inselspital in Bern (Switzerland) containing I-13129 and Lu-17730 was also tested with an outstanding performance of the membranes. We finally, assessed the reusability of the membranes, as well as the scalability of the whey amyloid membranes for large-scale wastewater treatment, inferring that large-scale use of this technology on radioactive wastewater treatment is possible.
2 Results and discussion
Fig. 1a shows the schematic representation of the experimental unit developed to conduct clinical radioactive material removal using the amyloid hybrid membrane. The membrane is cropped into a disk of a diameter of 53 mm (Fig. 1b). The radioactively contaminated water is passed through the filtration cell by applying gentle pressure with a syringe (ESI† Fig. S1) with a flow rate of approximately 5 ml min−1. We used 10 wt% whey hybrid membrane for all testing protocols. Fig. 1c shows the scanning electron microscope (SEM) image of the amyloid hybrid membrane surface. The SEM image clearly shows the cellulose scaffold and the activated carbon components, while amyloids are revealed only at higher magnification, as in the transmission electron microscope image of Fig. 1d, the amyloid fibrils have contour lengths of the order of 1 μm or beyond and an average diameter of 4–6 nm (see ESI† Fig. S2 for AFM images). The pore distribution in the membrane and additional SEM images are provided in ESI† Fig. S3 and S4. We assume that the amyloid fibrils specifically bind and therefore adsorb the radioactive heavy metal compounds. As will be shown below, amyloids are the main radionuclide-adsorbing component of the membranes: Fig. 1e schematically illustrates the antiparallel cross-beta sheet structure of the amyloid fibrils and the putative binding sites for the radionuclide ions. The separation mechanism of heavy metal ions by the amyloid fibrils has been discussed in detail in our earlier reports.18,31 The basic concept and technology are inspired by the growth of amyloid plaques in vivo catalyzed by the presence of the metal ions. Accordingly, also in artificial amyloid membranes heavy metal ions adsorb very strongly via supramolecular metal–ligand interactions, providing an outstanding separation performance. In our earlier reports, we extensively studied the thermodynamic binding process of metal ions by amyloid fibrils by performing adsorption isotherms, isothermal titration calorimetry (ITC)31 and molecular docking simulations.
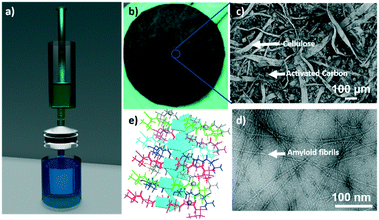 |
| Fig. 1 (a) Schematic diagram of syringe-aided filtration setup. (b) Cropped filter membrane. (c) SEM image of the membrane surface. (d) Transmission electron microscope image of the amyloid fibrils. (e) Schematic representation of the putative binding of radionuclides on to the amyloid fibrils surface. | |
2.1 Performance of the amyloid hybrid membrane
Several medicine-relevant radioactive compounds having various half-life periods such as technetium (Tc-99m), iodine (I-123), gallium (Ga-68) and the real clinical wastewater obtained from Inselspital in Bern containing Iodine (I-131) and Lutetium (Lu-177), were passed through the membrane to measure the adsorption of these compounds within the hybrid amyloid membrane. A volume of 20 mL was used in each experiment. The background-subtracted concentrations of these radionuclides measured before and after the filtration using a Wastewater Counter ISOMED 2151 are reported in Fig. 2. Removal efficiencies (E, %) were calculated from averaged counts per minute (cpm) measurement values, and determined using the concentrations in membrane feed streams (Cf) and permeate (Cp):
Removal efficiencies of 99.999% for Tc-99m pertechnetate (Fig. 2a), 99.999% for I-123 (Fig. 2b) and 99.822% for Ga-68 (Fig. 2c) were achieved with the syringe-aided setup equipped with a 10 wt% amyloid membrane. Also, even the Swiss hospital wastewater, when being in a mixture of both Lu-177 and I-131, radionuclides were removed with an efficiency of 99.995% and 99.95%, respectively. As a control, the hybrid membrane formed by cellulose and carbon (with the same ratio as in the case of amyloid membranes, but without any protein) featured, as shown in Fig. 2d (yellow column) Lu-177 removal efficiency of 34.5% and I-131 removal efficiency of 3%. These findings indicate that the adsorption is carried out primarily by amyloid fibrils and demonstrate a very high affinity of the amyloid–carbon hybrid membrane for all the tested radioactive compounds. The radioactivity of the water after filtration was below the level of detection and thus, non-radioactive. These results demonstrate clear applicability of amyloid–carbon hybrid membranes in treating radioactively contaminated clinical wastewater.
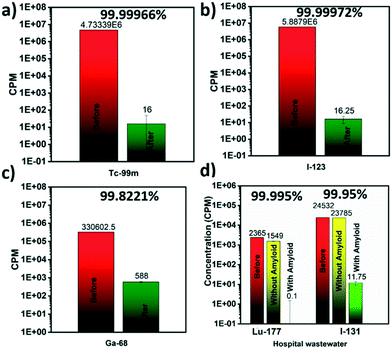 |
| Fig. 2 Removal efficiencies for the different radioactive substances. Aqueous solutions filtrated by amyloid–carbon hybrid membranes using syringe aided setup for: (a) Tc-99m pertechnetate, (b) I-123, (c) Ga-68, and (d) hospital wastewater having the mixture of Lu-177 and I-131. | |
Further, reusability and reproducibility of the results by membrane filtration were tested by multiple filtration cycles. After each round of filtration, the membranes were imaged mainly by an automatic gamma counter (Fig. 3) without any regeneration step to avoid secondary pollution issues with radioactive waste. Selected membranes were imaged with planar scintigraphy, single-photon emission computed tomography (SPECT) or positron emission tomography (PET) depending on the respective radionuclide type (cf. Material and methods in the ESI†).
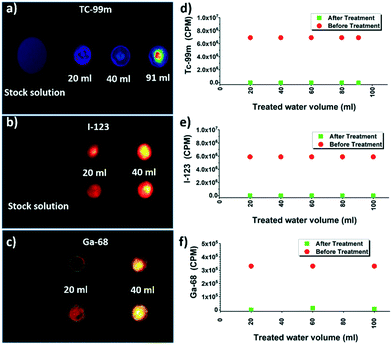 |
| Fig. 3 Planar scintigraphy of: (a) Tc-99m, (b) I-123 and PET/CT images of (c) Ga-68 filters after filtration of different volumes of stock solution, with corresponding gamma counter measurements of the filtrate in (d)–(f), respectively. | |
Tc-99m, I-123, and Ga-68 after multiple cycles of the filtration are shown in Fig. 3a–c respectively. Planar scintigraphy imaging in Fig. 3a and b show that with an increased number of filtration cycles, radioactivity inside the membrane is increased due to the accumulation of the adsorbed radionuclide ions with each cycle, compared to the stock solution. Fig. 3c shows the PET/CT images of Ga-68. The control stock solution Ga-68 does not show visible colors in the planar scintigraphy; however radioactive intensity increases and becomes visible after up-concentrating on the filter membrane after multiple filtration cycles. Fig. 3d–f shows that even with multiple cycles of filtrations, the removal efficiencies still did not decrease. This clearly shows the membrane was not saturated during these cycles (Fig. 3b and c). These results are reproducible: testing with different membrane batches showed identical results.
2.2 Filtration of original radioactive hospital wastewater
In a saturation experiment for real radioactive hospital wastewater containing a mixture of Lu-177 at 2.79 MBq l−1 and I-131 at 482 kBq l−1 sequentially, samples of 20 ml were filtered with the hybrid membrane after pre-treatment with a cellulose filter (Fig. 4). The imaging results and bacterial removal efficiency are also presented in Fig. 4. Imaging of membranes used for the syringe aided filtration of the mixture of Lu-177 and I-131 revealed the accumulation of radioactive compounds on the corresponding membranes in correlation with the amount of polluted water that was filtered by the corresponding membranes (Fig. 4a and b). Moreover, the membrane was not saturated for any of the compounds after being subjected to five subsequent cycles of filtration. While the control hybrid membrane formed by cellulose and carbon (with the same ratio as in the case of amyloid membranes, but without any protein) was not able to remove any of the two compounds (Fig. 4c), both Lu-177 and I-131 were removed successfully by the amyloid–carbon hybrid membrane: This demonstrates unambiguously that amyloids are the active components adsorbing radionuclides. In addition to radionuclides, the tested clinical wastewater also contained biological pollutants associated with the physiological cycle. The water before and after the filtration was thus plated on an LB-agarose gel. Before filtration (Fig. 4d) bacterial colonies' growth was observed on the agarose gel after the filtration of the water sample; in contrast, no biological colony growth is to be observed after amyloid filtration (Fig. 4e). By exploiting size exclusion, the hybrid membrane is equally efficient in removing biological contaminants along with the radioactive radionuclides.
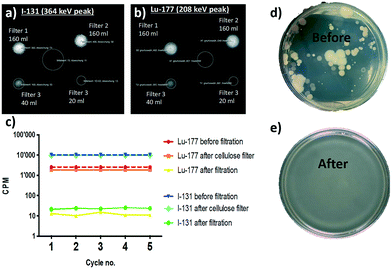 |
| Fig. 4 (a) Planar scintigraphy of I-313 and (b) Lu-177 filters after filtration of increasing volumes. (c) Five gamma counter measurements for each filtrate. Biological contamination in the wastewater: wastewater plated on LB-agarose gel (d) before and (e) after filtration, respectively. | |
2.3 Scalability of the filtration technology
To test the scalability of this method to remove the clinical radionuclides, three large membranes having the size of the 20 × 10 cm were tested. A Pulcino® crossflow filter setup was equipped with three amyloid–carbon hybrid membrane filters shown in Fig. 5a. After one test-run with water, approximately 4 liters of water (3847.8 g) containing 3 ml of Tc-99m pertechnetate solution with an activity of 13.71 MBq were passed through the filter system by gravity flow, i.e. without external energy supply. The membranes have high mechanical strength, with a wet bursting strength of 1.5 bar differential pressure. Samples were taken before and after filtration and measured for radioactivity. Finally, the filter membranes were removed from the setup and planar scintigraphy was obtained. Before filtration, the sample showed an activity of 0.096 MBq. The sample after filtration resulted in the radioactivity of 0.000 MBq. Scintigraphy of the membranes clearly showed that the radioactive isotopes were immobilized on the hybrid membrane (Fig. 5c). Due to radioactivity laboratory regulations, we were not able to treat very large volumes to identify the adsorption saturation capacity of the membrane.
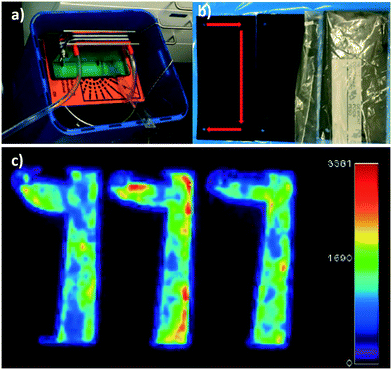 |
| Fig. 5 (a) Planar scintigraphy of amyloid–carbon hybrid membranes after filtering of radioactively contaminated water. Filtration of the aqueous solution of Tc-99m pertechnetate using a Pulcino® crossflow filter setup equipped with three amyloid–carbon hybrid membrane filters. (b) Hybrid membranes after filtering radioactively contaminated water ready for planar scintigraphy. Red arrows indicate the flow path of the filtrate. (c) Planar scintigraphy of hybrid membranes shows the accumulation of Tc-99m on the three filter membranes. | |
Moreover, the scintigraphy showed an intense accumulation of bound Tc-99m along the flow path of the liquid in the filtration unit. The inlet and the bottom of the membrane adsorbed the radioactive Tc-99m, whereas the remaining parts of the membrane especially the outlet, did not show any signs of radioactivity. The radioactivity of the water after filtration was below the level of detection and thus could be considered as non-radioactive. These findings indicate a very high affinity of the amyloid–carbon hybrid membrane of the tested compound Tc-99m pertechnetate and demonstrate that this method is easily scalable for large scale wastewater treatment.
3 Conclusions
In summary, we have demonstrated that amyloid–carbon hybrid membranes are capable of removing clinically relevant radioactive substances from hospital wastewater by adsorption mechanism. The approach presented here is scalable, sustainable and of very low cost in that it relies on very affordable raw materials. Importantly, the membranes have proven to bear the potential to be used for the purification of radioactive wastewater on a large scale. For example, while the metastable Tc-99m tested here, is a short-lived (half-life about 6 hours) nuclear isotope used in nuclear medicine produced from molybdenum-99, long-lived technetium isotopes, notably Tc-99, with a half-life of over 210
000 years, are contributing components of nuclear reactor effluents, which must be controlled and for which liquid storage is unthinkable. Since the metal–ligand binding characteristics between radionuclides and amyloids are fully independent on the nuclear details of the isotopes, it can safely be stated that any technetium isotopes will be removed with outstanding efficiency by this technology. Similar considerations apply to the release of the long-lived I-129. Other long-lived fission products, such as Zr-93 remain to be evaluated, but our results for the nuclides considered point to a universal approach in radioactive wastewater treatment and warrant further investigation into filtration systems for liquid nuclear waste and nuclear energy incidents remediation. The possibility of converting large volumes of radioactive liquid waste into low volumes of solid radioactive waste is a key requirement in the nuclear and clinical radioactive wastewater treatment. The authors plan to conduct future studies at pilot scale level in other Swiss hospitals to assess the long-term performance of the technology with additional clinically relevant radioactive wastewater compounds, the adsorption capacity, as well as the economic and sustainability footprint of this technology on radioactive waste. Our vision is to extend these studies to further relevant radionuclides used in nuclear medicine and nuclear industry and to pave the way to an efficiently and sustainable radioactive wastewater management.
Conflicts of interest
RM and SB are inventors of an ETH-related patent. BluAct Technologies GmbH has an exclusive licence of this patent from ETH.
Acknowledgements
Support from the Innosuisse innovation cheque 36689.1 INN0-EE. Is acknowledged. Michael Diener is acknowledged for the schematic preparation of the syringe-aided filtration setup. The authors acknowledge the support of the ScopeM/Swiss Federal Institute of Technology ETHZ.
References
- F. A. Mettler, W. Huda, T. T. Yoshizumi and M. Mahesh, Effective doses in radiology and diagnostic nuclear medicine: A catalog, Radiology, 2008, 248, 254–263 CrossRef.
- M. Hamoudeh, M. A. Kamleh, R. Diab and H. Fessi, Radionuclides delivery systems for nuclear imaging and radiotherapy of cancer, Adv. Drug Delivery Rev., 2008, 60, 1329–1346 CrossRef CAS.
- M. D. Wang, D. M. Shin, J. W. Simons and S. Nie, Nanotechnology for targeted cancer therapy, Expert Rev. Anticancer Ther., 2007, 7, 833–837 CrossRef CAS.
-
R. E. Coleman, Holland-Frei Cancer Medicine, 6th edn, 2003 Search PubMed.
- S. Banerjee, M. R. Ambikalmajan Pillai and N. Ramamoorthy, Evolution of Tc-99m in diagnostic radiopharmaceuticals, Semin. Nucl. Med., 2001, 31, 260–277 CrossRef CAS.
-
Medical Imaging Methods: Recent Trends, ed. A. K. Shukla, Springer Singapore, 2019 Search PubMed.
- ICRP, Recommendations of the International Commission on Radiological Protection, Br. J. Radiol., 1955, 6 Search PubMed.
- The Radiation protection Rules (RPR) 1971, under Section 30 of The Atomic Energy Act, 1962.
- International Commission on Radiation Units and Measurement (ICRU) Recommendations. 1980.
- IAEA. Management of Waste from the Use of Radioactive Material in Medicine, Industry, Agriculture, Research and Education. IAEA Safety Guide WS-G-2.7 International Atomic Energy Agency 2005.
- Release of Patients After Radionuclide Therapy, IAEA Safety reports series 63, International Atomic Energy Agency 2009.
- S. Menon and V. Kumar, Weaponizing radioactive medical waste - The looming threat, International Journal of Nuclear Security, 2019, 5 Search PubMed.
- S. Khan, A. Syed, R. Ahmad, T. A. Rather, M. Ajaz and F. Jan, Radioactive waste management in a hospital, Int. J. Health Sci., 2010, 4, 39–46 Search PubMed.
- S. Bolisetty, M. Peydayesh and R. Mezzenga, Sustainable technologies for water purification from heavy metals: review and analysis, Chem. Soc. Rev., 2019, 48, 463–487 RSC.
-
D. B. Schweiz, SR 814.56 Verordnung vom 26. April 2017 über die Gebühren im Strahlenschutz (GebV-StS).
- R. O. A. Rahman, H. A. Ibrahium and Y.-T. Hung, Liquid radioactive wastes treatment: A review, Water, 2011, 3, 551–565 CrossRef.
-
J. McCurry, The Guardian, 2019 Search PubMed.
- S. Bolisetty and R. Mezzenga, Amyloid-carbon hybrid membranes for universal water purification, Nat. Nanotechnol., 2016, 11, 365–371 CrossRef CAS.
- S. Bolisetty, N. Reinhold, C. Zeder, M. N. Orozco and R. Mezzenga, Efficient purification of arsenic-contaminated water using amyloid–carbon hybrid membranes, Chem. Commun., 2017, 53, 5714–5717 RSC.
- Q. Zhang, S. Bolisetty, Y. Cao, S. Handschin, J. Adamcik, Q. Peng and R. Mezzenga, Selective and efficient removal of fluoride from water: In situ engineered amyloid fibril/ZrO 2 hybrid membranes, Angew. Chem., Int. Ed., 2019, 58, 6012–6016 CrossRef CAS.
- M. Peydayesh, M. K. Suter, S. Bolisetty, S. Boulos, S. Handschin, L. Nyström and R. Mezzenga, Amyloid fibrils aerogel for sustainable removal of organic contaminants from water, Adv. Mater., 2020, 32, 1907932 CrossRef CAS.
- J. G. Mcafee, C. F. Fueger, H. S. Stern, H. N. Wagner and T. Migita, Tc99m pertechnetate for brain scanning, J. Nucl. Med., 1964, 5, 811–827 CAS.
- J. Weaver, C. Z. Soderquist, N. M. Washton, A. S. Lipton, P. L. Gassman, W. W. Lukens, A. A. Kruger, N. A. Wall and J. S. McCloy, Chemical trends in solid alkali pertechnetates, Inorg. Chem., 2017, 56, 2533–2544 CrossRef CAS.
- A. MacDonald and S. Burrell, Infrequently performed studies in nuclear medicine: part 1, J. Nucl. Med. Technol., 2008, 36, 132–143 CrossRef , quiz 145.
- H.-M. Park, 123 I: Almost a designer radioiodine for thyroid scanning, J. Nucl. Med., 2002, 43, 77–78 CAS.
-
Practical Nuclear Medicine, ed. P. F. Sharp, H. G. Gemmell and A. D. Murray, Springer-Verlag, London, 2005 Search PubMed.
- A. Jalilian, An overview on Ga-68 radiopharmaceuticals for positron emission tomography applications, Iran. J. Nucl. Med., 2016, 24, 1–10 CAS.
- M. P. M. Stokkel, D. Handkiewicz Junak, M. Lassmann, M. Dietlein and M. Luster, EANM procedure guidelines for therapy of benign thyroid disease, Eur. J. Nucl. Med. Mol. Imaging, 2010, 37, 2218–2228 CrossRef.
- WHO | Health risk assessment from the nuclear accident after the 2011 Great East Japan earthquake and tsunami, based on a preliminary dose estimation.
- L. Emmett, K. Willowson, J. Violet, J. Shin, A. Blanksby and J. Lee, Lutetium 177 PSMA radionuclide therapy for men with prostate cancer: a review of the current literature and discussion of practical aspects of therapy, J. Med. Radiat. Sci., 2017, 64, 52–60 CrossRef.
- M. Peydayesh, S. Bolisetty, T. Mohammadi and R. Mezzenga, Assessing the binding performance of amyloid–carbon membranes toward heavy metal ions, Langmuir, 2019, 35, 4161–4170 CrossRef CAS.
Footnotes |
† Electronic supplementary information (ESI) available. See DOI: 10.1039/d0ew00693a |
‡ These two authors contributed equally. |
|
This journal is © The Royal Society of Chemistry 2020 |
Click here to see how this site uses Cookies. View our privacy policy here.