Removal of contaminants from canal water using microwave synthesized zero valent iron nanoparticles†
Received
22nd February 2020
, Accepted 19th August 2020
First published on 2nd September 2020
Abstract
Nanoscale zero valent iron nanoparticles (nZVI-NPs) have been used for the remediation of a wide variety of environmental contaminants. Here, nZVI-NPs were synthesized using a green method involving leaf extract of Mentha piperita as the reducing agent and microwave treatment in place of conventional heating. The resulting NP composition, morphology, surface charge and size were studied using UV-visible spectrophotometry (UV-vis), scanning electron microscopy coupled with energy dispersive X-ray spectroscopy (SEM-EDX) and dynamic light scattering (DLS), respectively. The plasmon resonance spectrum of the synthesized nZVI-NPs had a peak at 292 nm. The synthesized nZVI-NPs were spherical in shape, with sizes between 5 and 10 nm, and negatively charged with zeta potential of −27.9 ± 2.19 mV. The nZVI-NPs with a high reactive surface area were successfully utilized to remove phosphate, lead, ammonia, nitrate and chloride from water samples taken from the Worcester and Birmingham canal at the University of Birmingham, United Kingdom. A successful rapid removal of dissolved agricultural contaminants (phosphate, ammonia, nitrate, lead and chloride) by 10 μg/100 mL nZVI-NPs was observed during reaction times of 12–24 hours, confirming the use of the NPs as a viable remediation strategy. The nZVI-NPs adsorbed 85.01% of phosphate, 99.51% of ammonia, 86.33% of nitrate, and 83.4% of chloride present in the samples initially, as well as removing 79.33% of the lead present within 24 hours.
Water impact
This work demonstrates the use of low energy (microwave) synthesized zero valent iron nanoparticles, reduced and stabilised via a plant extract, for single-step removal of a range of dissolved agricultural contaminants (phosphate, ammonia, nitrate, lead and chloride) from canal water. While lab solutions are a long way from utilisation in the real world, the multi-pollutant remediation using cheap and sustainable materials is an important first step.
|
Introduction
Globally, 80% of municipal wastewater is discharged into the environment untreated, while industry is responsible for dumping millions of tons of heavy metals, toxic sludge and other wastes into water bodies annually.1,2 The presence of organic and inorganic pollutants in water and food is one of the most serious environmental issues currently due to the widespread utilization of pesticides and fertilizers in agriculture.3 Around 115 million tons of mineral nitrogen fertilizers are applied to croplands each year, of which 20% accumulates in soils and biomass, while 35% enters waterways. Water pollution remains a global challenge for both developed and developing countries, threatening human and environmental health, with serious efforts needed to prevent further deterioration of water quality.4 While human settlements, agriculture and industry are major sources of water pollution, agriculture is considered to be the biggest polluter.5 Treatment of effluents compromising heavy metals has become an important topic due to the environmental and sanitary problems arising, and increasingly restrictive legislation. These heavy metals are not only carcinogenic and teratogenic but also cause deleterious health effects in humans and the environment throughout the food chain.6,7 Currently, more than 700 emerging pollutants, their metabolites and transformation products, are listed as being present in the European aquatic environment, with potentially harmful effects on human health and ecosystems.8
The development of nanotechnology at the end of 20th century has widened the variety of adsorbents available for remediation of contaminated sites. The unique properties of nanomaterials including high surface area, particle size, reactivity, shape and size, as well as the range of transformations they undergo in the environment, including agglomeration and dissolution, enables a broad range of possible applications in different fields.9,10 The distinct physical and chemical properties of NPs, such as nano-size, surface characteristics, reactivity, conductivity, and optical properties, are often related with negative ecotoxicological effects in the environment and unexpected health hazards.11–13 NPs have been cited as one of the most advanced processes for waste water treatment as nano-adsorbents, nano-catalysts and nano-membranes for the removal of pollutants.14–16 Over the last 20 years, nano-catalysts like iron oxide have gained considerable attention in the field of wastewater treatment, where they have been used to degrade pollutants because of their unique catalytic properties and outstanding stability.17
NPs' potential as adsorbents arises due to their small size and large surface area, which enhances their chemical activity and adsorption capacity.18,19 NPs used for heavy metal removal must be non-toxic to avoid introduction of additional contaminants into the environment, and must have high and selective adsorption capacity and the ability to adsorb at low concentrations (or particle and analyte), and allow easy removal of the organic, inorganic or toxic pollutants from the environment.20 Iron NPs (nZVI-NPs) undergo various reactions including adsorption, reduction, precipitation, oxidation, co-precipitation, etc. which makes them suitable for use in the removal of metals, as shown schematically in Fig. 1.24 nZVI-NPs can be directly used to remediate contaminated environments with low chance of secondary contamination due to their ecofriendly nature and adsorptive properties.21,22 Gupta et al. 2015, reported the modification of iron nano-adsorbents showing high removal affinity of different heavy metal pollutants such as Cr3+, Co2+, Ni2+, Cu2+, Cd2+, Pb2+ and As3+ from wastewater.23
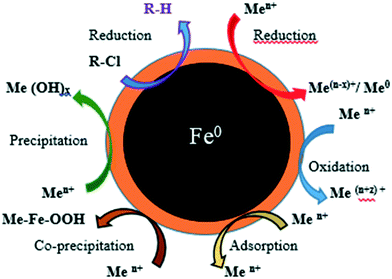 |
| Fig. 1 The structure of nZVI-NPs showing various mechanisms for removal of metals and chlorinated compounds. Adapted from O'Carroll et al. 2013.24 | |
nZVI-NPs are inexpensive and their main applications utilize their electron donating properties, which make nZVI-NPs fairly reactive in water and enables them to serve as excellent electron donors, thereby making them a versatile remediation material.24,25
| Fe0 → Fe2+ + 2e− E0 = −0.41 | (1) |
However, in aquatic environments, nZVI-NPs react with water to form a layer of oxy-hydroxide, and thus many strategies for surface modification and protection have been proposed in the literature.
26,27 Indeed, many of the ligands used to modify the surface of metallic or nZVI-NPs (Fe
0) are also utilized as part of the reaction to form the particles, by acting as reducing agents, which can react with dissolved oxygen and to some extent with water. The classical electrochemical/corrosion reactions take place by which iron is oxidized from exposure to water and oxygen. There are several such examples already in the literature, often utilizing plant extracts as both stabilizing agents and reducing species, wherein detailed analysis of these structures shows a hydroxy-polyphenol layer surrounding the iron core, which also enhances their anti-oxidant properties.
28 Prasad
et al. (2014) found that functional groups such as –NH, –C
![[double bond, length as m-dash]](https://www.rsc.org/images/entities/char_e001.gif)
O, –C
![[double bond, length as m-dash]](https://www.rsc.org/images/entities/char_e001.gif)
N and –C
![[double bond, length as m-dash]](https://www.rsc.org/images/entities/char_e001.gif)
C, present in
Mentha piperita extract, were involved in the synthesis process of nZVI-NPs.
29
Conventional methods of synthesis of NPs have several limitations viz. high temperature and pressure and energy requirements, relatively expensive processes and low production rates, as well as having additional drawbacks including contamination of precursor and release of harmful by-products due to toxic solvents.30 Hence, the production of NPs by green methods is advantageous due to the lower energy requirements, decreased reaction times, their ecofriendly nature arising from the use of less toxic synthesis precursors and routes, leading to greater sustainability of the resulting materials and their applications in environment remediation.31 Biosynthesis of NPs, i.e. synthesis using reducing agents extracted from plants or microbes, has been acquiring attention due to the vast range of biomolecules available, and the fact that several also have therapeutic or antimicrobial functions, such that multi-functional NPs can also be designed at low cost.32,33
Early research, assessing the application of NPs for remediation and removal of environmental contaminants was limited to remediation of one pollutant at a time, focusing on either organic or inorganic pollutants.34,35 However, in recent studies, NPs such as nZVI have emerged as a new option for the treatment of contaminated soil and ground water targeting multiple pollutants including chlorinated organic contaminants (solvents, pesticides, chlorinated ethenes etc.)36,37 and for reduction of inorganic anions such as nitrate to ammonia,37 or perchlorate to chloride.38 The objective of the present work was thus to evaluate the efficiency of biosynthesized nZVI-NPs in removing different contaminants, arising from agricultural and urban pollution such as nitrate, phosphate, ammonia lead and chloride, from canal water. Leaf extract of Mentha piperita was used as the reducing agent to synthesize nZVI-NPs via microwave heating for just 3 minutes at 48 °C. Water samples were collected from the canal that passes through the University of Birmingham, United Kingdom. Initial concentrations of the target pollutants were determined, and the final concentrations following incubation for 12 or 24 hours with 1 mg L−1Mentha piperita stabilized nZVI-NPs were used to demonstrate the effectiveness of the green-synthesized nZVI-NPs for multi-contaminate freshwater remediation.
2. Materials and methods
2.1 Preparation of leaf extract
20 grams of fresh Mentha piperita leaves were collected from the Hazara University, Mansehra, Pakistan and washed with tap water to remove the sand and soil particles. Extract was prepared using the procedure described by Wang et al.39 Briefly, leaves were washed with de-ionized water, cut into small pieces and boiled at 20 g L−1 in deionized water at 48 °C (medium low setting) for 5 minutes in a conventional microwave oven {INPUT: 230–240 V∼50 Hz 1200 W (MICRO), OUTPUT: 700 W 2450 MHz (Boss international company)}, and filtered using Whatman filter paper (diameter: 12.5 cm).
To attain greater stability, maximum yield, maximum production and controlled size of NPs, different parameters including mixing ratio of precursor salt, temperature and time of heating were optimized.
2.2 Phytochemical screening tests
Various active and bioactive compounds detection tests were conducted to identify substances like phenols, alkaloids, flavonoids, tannins, saponins, tannins, terpenoids, quinones, etc. in the plant extract using the method described by Ugochukwu, SC (2013).40 Summary details of the tests are shown in Table S1,† providing insights into the functional groups available for adsorption of pollutants.
2.3 Synthesis of the nZVI-NPs
nZVI-NPs were synthesized using a nontoxic and cost-effective approach, under controlled conditions following optimization of the synthesis parameters. The NPs were prepared using 10 mL of 1 mM FeCl3 to 20 mL of L. Mentha piperita extract at a volume ratio of 1
:
6 and heated in the microwave for 3 minutes at 48 °C (medium low). The reduction of Fe ions was observed by carefully monitoring an aliquot (1 mL) at different time intervals (1 to 5 minutes) by UV-visible spectrophotometer using a lambda 25 (Perkin Elmer, Shelton, CT 06484, USA). A spectral scanning analysis between 200–800 nm was performed, and the absorption maximum for the as synthesized nZVI-NPs was found to span the range 250–370 nm. The reaction equation is shown below.
FeCl3·6H2O + Extract → nZVI-NPs + Byproducts (Salt) (Plant leaves). |
The overall reduction of Fe2+ ions was confirmed by the visible color change from greenish to blackish in the reaction mixture, indicating the formation of nZVI-NPs (Table S2†).
After about 3 minutes of heating in the microwave oven at 48 °C (i.e. medium low setting) the reaction is complete. About 0.144 g of dried nZVI-NPs were synthesized from a 1
:
6 mixture of the Mentha piperita leaf extract and the Fe precursor mixture, as shown in Fig. S1.† The mechanism of biosynthesis of the metallic nZVI-NPs is shown schematically in Fig. S2 in the ESI,† where the secondary metabolites (tannins, saponins, glycosides, terpenoids and flavonoids) reduce the FeCl3·6H2O salt into nZVI-NPs. These metabolites not only act as stabilizing agents but also help to prevent the oxidation of the NPs.
2.4 Characterization of nZVI-NPs
In additional to UV-visible characterization, dynamic light scattering (DLS) and scanning electron microscopy equipped with an energy dispersive spectrophotometer (SEM-EDS) were applied to determine the size, elemental composition and morphology of the resulting NPs. For the DLS analysis, the refractive index (RI) was 1.33 and the dispersant was DI water. Malvern Zeta sizer Nano-ZS software was used for data collection and analysis. To study the Zeta potential of the FeNPs, 0.1 μg of particles were dispersed in 50 mL of deionized water (media) and sonicated for 5 minutes to prevent agglomeration of the particles before measuring at 25 °C.
2.5 Water sample collection
Water samples were collected from the Worcester and Birmingham canal at the University of Birmingham, United Kingdom. About 1 liter of the sample was taken from the canals and kept in clean and dried bottles. The samples were labelled and filtered using glass micro fibers (GFC) filters (Whatmann, diameter 47 mm, CAT no 1822-047). Before filtration, filter paper was weighed and kept in the oven for 30 minutes to dry the moisture content already present in it. The filter paper was weighted again after drying and then used for filtration. ESI† Fig. S2 shows the as-collected and filtered waters, respectively.
2.6 Adsorption, optimization and determination of contaminant concentrations
Filtrated water samples were analyzed for contaminants which were identified using individual portable photometers based on light emitting diodes (LED) of HANNIA Instruments specific for each contaminant. The collected sample was tested for the presence of each of the target pollutants (nitrate, lead and chloride) individually. The HI96713 phosphate low range photometer was used to study the phosphate in the water sample while the HI96753 Chloride photometer was used for chlorine detection and the same specific photometers were used throughout the work.
Once the presence of the target pollutants was confirmed, the adsorption optimization was done on the water samples by adding 10 μg of nZVI-NPs to 100 mL canal water samples and shaking for 10 minutes. All the samples were checked after the time interval of 12 to 24 hours whereupon quantification of pollutant adsorption was carried out. Triplicate samples were prepared for each optimization.
3. Results and discussion
3.1 Phytochemical screening results on Mentha piperita leaf extract
Tannins, phenols, terpenoids are present whereas saponins, phlobatannins (phenolic substances) were absent as shown in Table S3.† These phytochemical compounds have different reducing properties which play a role in the synthesis of the NPs by reducing the precursor.41–43 As measured, low to moderate levels of phenolic with antioxidant activity were already reported from Mentha piperita.39 The capping biomolecules on the surface of plant extract stabilized nZVI-NPs consist of negatively charged groups and are also responsible for stability of the extract mediated ZVI-NPs.44
Bare metal NPs are highly chemically reactive and can be easily oxidized in air resulting in a loss of magnetism and dispersibility. Thus, from an application point of view, it is essential to formulate protection strategies to stabilize the naked NPs. Thus there is considerable interest in use of natural and biodegradable polymers (polyphenols) to cap these particles to prevent oxidation and agglomeration. As a result, the selection of the plant extracts used for synthesis of the NPs should be made carefully. For example, it was observed that hydrolysable tannins (plant polyphenols) were the major metabolites (79%) existing in leaf extract compared with other phytochemicals. Hydrolyzable tannins can inhibit hydroxyl radical formation from the Fenton reaction through complexation of ferrous ions.45 As such, polyphenol anions assisted the reduction of Fe3+ and Fe2+ ions to Fe0 (nZVI-NPs). The hydroxyl and carboxylic groups of polyphenolic compounds contained in the leaf extract are deprotonated and are strong complexing and reducing agents for iron ions. Thus, iron ions and hydroxyl groups are converted into carbonyl groups during the reduction reaction as the iron ions are concurrently reduced into the nZVI-NPs.
3.2 Characterization of nZVI-NPs using UV-visible spectrophotometry
The biosynthesis of the nZVI-NPs was observed through UV-visible double beam spectrophotometry, with a representative spectrum shown in Fig. 2. The peak obtained for the synthesized nZVI-NPs spans the range of 250–370 nm, which is identical to the characteristic UV peak of metallic iron. The absorption peak obtained is in a good agreement with previous literature.46 The maximum wavelength (λ) of the absorbance peak for the nZVI-NPs was found at 292 nm with an absorbance (A) of 1.158, confirming the formation of nZVI-NPs as this is a signature band. Indeed, the black coloration of the solution confirmed the synthesis of nZVI-NPs as a result of the oscillation of the conduction band electrons, known as the surface plasmon resonance (SPR). The reduction of Fe3+ ions to Fe0 NPs is indicated by the change in color due to excitation of electrons, as shown in ESI† Fig. S1 and Table S2.
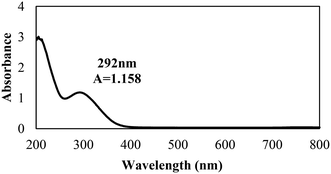 |
| Fig. 2 UV-visible spectrophotometric spectrum of Mentha piperita leaf extract stabilized nZVI-NPs. | |
3.2.1 SEM-EDX characterization of nZVI-NPs.
A representative SEM image and its corresponding EDX spectrum are shown in Fig. 3. The SEM image confirms the formation of nano-sized Fe particles in the range of 5–10 nm. Uniformly distributed, spherical shaped NPs are clearly seen in the SEM image, with some agglomeration. EDX spectroscopy was used for the elemental analysis. The strong peaks at 6.5 and 7.2 KeV confirmed the presence of Fe, and the atomic percentage of Fe was quantified as 100% (Table 1). Thus, we can have confidence that the repeated centrifugation steps following the synthesis leads to the complete purification of the NPs, and that the Mentha piperita leaf extract reducing and antioxidant agents such as phenolic compounds, protect the nZVI-NPs from oxidation prior to their application for remediation,47 as there is no evidence of O present in the EDX spectra. It is worth noting also that the nZVI-NPs remained in solution throughout the synthesis and application steps, thus limiting their contact with air.
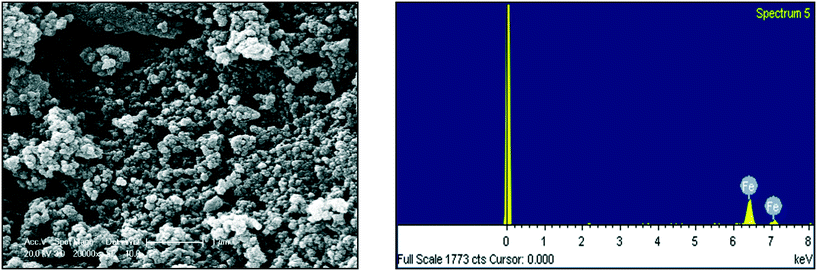 |
| Fig. 3 SEM image of biosynthesized nZVI-NPs and their corresponding EDX spectra. | |
Table 1 The atomic% of the microwave synthesized nZVI-NPs
Elements |
Weight% |
Atomic% |
Fe |
100.00 |
100.00 |
Total |
100.00 |
|
3.2.2 Zeta potential analysis through dynamic light scattering (DLS).
DLS analyzes velocity distribution movement of particles from dynamic fluctuations of light scattering intensity which is due to the Brownian motion of particles. The z-average particle size was determined to be 52.7 ± 61.1 nm and the polydispersity index (PDI) by zeta sizer software was found to be 0.29 ± 0.040. The larger size of NPs indicated agglomeration of the ZVI-NPs due to their magnetic property, as the DLS measured the hydrodynamic size. The zeta potential for NPs was found to be 27.9 ± 2.19 mV confirming the electrostatic stability of nZVI-NPs.
3.3 Treatment of canal water
The detected contaminant levels in the filtered as-collected canal water are shown in Table 2, which were compared with the recommended levels for of the Organization for Economic Co-operation and Development (OECD).48–52
Table 2 Constituents present in canal water initially (observed results) and comparison to the EPA recommendations and UK guidelines for drinking water
No |
Constituents |
EPA level in drinking water |
UK drinking waterQuality regulation |
Contaminant level found in Birmingham Canal water |
1. |
Phosphate |
0.01–0.03 mg L−1 (ref. 32) |
1 mg L−1 (ref. 53) |
0.74 mg L−1 |
2. |
Ammonia |
0.25–32.5 mg L−1 (ref. 33) |
0.5 mg L−1 (ref. 54) |
76.7 mg L−1 |
3. |
Lead |
0.00 mg L−1 (ref. 34) |
0.01 mg L−1 (ref. 55) |
46.6 mg L−1 |
4. |
Nitrate |
10 mg L−1 (ref. 35) |
50 mg L−1 (ref. 56) |
55.6 mg L−1 |
5. |
Chloride |
1.0 mg L−1 (ref. 36) |
5 mg L−1 (ref. 57) |
46.6 mg L−1 |
3.3.1 Removal of phosphate.
Phosphate is an important element naturally present in fresh and drinking water and its concentration ranges from 0.01 to 0.03 mg L−1. Excess phosphate (>0.1 mg L−1) can speed up eutrophication which is a reduction of dissolved oxygen in waterbodies due to excessive algal growth.58 Extensive work has already been done on phosphate removal, among which iron-based materials were considered most effective.59 nZVI-NPs are active in the removal of phosphate and other contaminants, due to their extensive highly reactive surface area.60–62
The % adsorption is calculated using the following equation:
|
| (2) |
The % adsorption of phosphate in the first 12 hours of incubation of 100 mL of the filtered canal water with 10 μg of biosynthesized nZVI-NPs was 63%, whereas after 24 hours, 85% of the initial phosphate was removed, as shown in
Table 3. The results presented here confirm previous findings, that NPs have good absorbing capability for phosphorus, and that the
Mentha piperita leaf extract stabilized nZVI-NPs are highly effective.
Table 3 Removal efficiency of nZVI-NPs at different time periods (12–24 h)
Constituents |
Concentration remaining after 12 h incubation (mg L−1) |
Concentration remaining after 24 h incubation (mg L−1) |
% adsorption (12 h) |
% adsorption(24 h) |
Phosphate |
0.27 |
0.11 |
63.01 |
85.01 |
Ammonia |
45.3 |
0.37 |
40.9 |
99.51 |
Lead |
27.3 |
9.7 |
41.4 |
79.18 |
Nitrate |
31.1 |
7.6 |
44.06 |
86.33 |
Chloride |
32.3 |
7.9 |
30.68 |
83.04 |
Removal of phosphate is totally based on point of zero charge (PZC), as when the solution pH is lower than the PZC the surface of the NPs is positively charged, making the surface suitable for absorption of anions such as PO43−, enhancing the adsorption of phosphate. The initial pH of the canal water was 4 and after incubating with the Mentha piperita leaf extract stabilized nZVI-NPs for 24 hours, it was found to be 6.1, such that the NPs would be positively charged, which is why effective removal of phosphate was achieved. The present study showed excellent removal of phosphate by our biosynthesized nZVI-NPs as compared to EL-FeNPs which removed 30.4% of total P (eucalyptus leaf extract FeNPs).37
3.3.2 Ammonia/nitrate removal.
Agricultural production as well as industrial and domestic waste dumping into surface water are the main sources of contamination of ammonia and nitrate. The initial concentration of ammonia in the canal water was found to be 76.7 mg L−1, while that of nitrate was 55.6 mg L−1 initially. During the first 12 hours of incubation of 100 mL of the canal water with 10 μg of biosynthesized nZVI-NPs about 40.9% of the ammonia and 44.06% of the nitrate initially present was removed, whereas 99.51% of ammonia and 86.33% nitrate removal was observed after 24 hours of incubation with the Mentha piperita leaf extract stabilized nZVI-NPs.
Previous researchers have also demonstrated successful removal of nitrate (NO3−) utilizing nZVI-NPs. According to these studies, the reduction efficiency was enhanced by increasing the solid–liquid ratio or decreasing the pH. The NO3− received electrons directly from Fe0 by enhancing Fe2+ in the aqueous phase.63–65 In this study, the biosynthesized nZVI showed excellent removal of ammonia after 12 to 24 hours, during which the pH of the water shifted from 4 towards 6.1 implying that less acidic conditions may provide better efficiency of ammonia removal, due to lower formation of the ammonium ion (NH4+) at the closer to neutral conditions, and thus the greater amount of the ammonia being in the neutral form that can bind to the slightly positively charged nZVI-NPs (note that the point of zero charge of magnetite is pH 6.1). Reaction kinetics were not measured in the present study, due to the simultaneous removal of several pollutants. The results shown in Table 3 indicate that nZVI-NPs are effective for both ammonia and nitrate removal from canal water samples.
3.3.3 Removal of lead (Pb).
Lead, the 34th most common element in the lithosphere, is toxic to humans and wildlife as it affects the structure and functions of vertebrate's bone, central nervous system, kidney and hematopoietic systems by effecting reproductive, biochemical, histopathological, neuro-physical systems, as well as being toxic to reproduction and development.66 Lead leaches into groundwater through smelting, mining, iron and steel foundries, combustion of fossil fuels in automobiles, and cement industries.
The initial concentration of the Pb2+ in the canal water was determined to be 46.6 mg L−1. High lead removal was obtained due to the presence of large numbers of active sites for adsorption process to occur in the presence of the nZVI-NPs. The results indicate that Pb2+ removal followed a similar trend as phosphate, ammonia and nitrate, with 41.4% of the initial concentration of Pb removed by the Mentha piperita leaf extract stabilized nZVI-NPs in the first 12 hours and 79.18% after 24 hours (Table 3).
3.3.4 Removal of chloride (Cl).
Chlorine is the number 1 contaminant found in drinking water, especially since it is added intentionally in order to purify water and kill microorganisms and other pathogens. Research has shown that people exposed to chlorinated water for long periods have a greater risk of contracting bladder cancer, though more research is needed.65 Carcinomas of the large intestines are one of the most common across the globe. Around 1.4 million cases of carcinomas were diagnosed in 2012, while the risk of colorectal cancer increases with age, affecting men more than women.66,67
Excess chlorine (>80 μ L−1) reacts with water and minerals to create trihalomethane (THMs), which causes asthma, heart disease, eczema and cancer. Other by-products such as aldehydes, haloacetic acids (HAAs), chloramines are also formed due to the complex reaction between chlorine and organic matter (humic acid, amino-acids and carbohydrates, etc.).65 The EPA declared that chlorine is safe to drink in water in smaller amounts but above the 0.080 mg L−1 average level can cause a severe problem. The permissible level of Cl− in drinking water is up to 4 mg L−1 by EPA and that of WHO is 5 mg L−1.66,67 nZVI-NPs synthesized from the Mentha piperita extract indicate strong antibacterial and antioxidant activities.67 The interaction of Cl− ions with the Mentha piperita leaf extract stabilized nZVI-NPs (10 μg of biosynthesized nZVI-NPs incubated in 100 mL of canal water) are shown in Table 3 and Fig. 4, indicating that these NPs are very effective for removal of excess chlorine from water after 24 hours, although some further optimization is needed for the 12 hours timeframe.
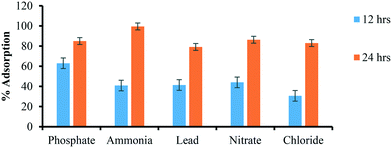 |
| Fig. 4 The % adsorption of different constituents of Birmingham Canal water by the nZVI-NPs. | |
Conclusion
nZVI-NPs have the unique characteristics of nZVI-NPs, including high surface area and anti-oxidant properties, make them effective for removing toxic metal ions, and organic and inorganic solutes from surface water. Removal of heavy metals from the Birmingham canal water using nZVI-NPs as a nano-adsorbent has been described. The presence of various phytochemicals in the Mentha piperita extract facilitates the reduction process and made it an effective capping agent on nZVI-NPs. The polyphenols and other secondary metabolites have stabilizing and antioxidant effect which makes Mentha piperita an ideal candidate for the synthesis of nZVI-NPs. Experimental studies concluded the % removal of phosphate, ammonia, nitrate, lead and chloride to be 85.01%, 99.51%, 86.33%, 79.33% and 83.04% of the initial constituents, respectively, within 24 h. The pH during the studies ranged between 4 to 8 and no buffer or other chemical was added. The synthesized nZVI-NPs are favorable for the treatment of agriculturally contaminated water and represent an environmentally friendly treatment process for simultaneous removal of 5 typical agricultural and urban pollutants. This laboratory demonstration represents the first step towards developing this green, rapid and low-energy consuming (microwave synthesis in just 3 minutes) synthesis process for nZVI-NPs for use in environmental remediation of agriculturally polluted waterways.
Conflicts of interest
The authors declare no conflict of interest.
Acknowledgements
The authors are thankful to the Higher education Commission (HEC) Pakistan for granting an IRSIP Scholarship to Salma Shad, and to the School of Geography, Earth and Environmental Sciences, University of Birmingham, United Kingdom for hosting Salma Shad during her research visit and for the technical support and all characterization. We thank Katie Reilly and David Tubbs from the School of Geography, Earth and Environmental Sciences, University of Birmingham, United Kingdom for their helpful suggestions regarding sampling and measurements. Consumables support was provided from the NanoFASE project (Grant Agreement No. 642007).
References
- T. Sato, M. Qadir, S. Yamamoto, T. Endo and M. Zahoor, Global, regional, and country level need for data on wastewater generation, treatment, and use, Agric. Water Manag., 2013, 130, 1–13, DOI:10.1016/j.agwat.2013.08.007.
-
J. Mateo-Sagasta, L. Raschid-Sally and A. Thebo. Global wastewater and sludge production, treatment and use, in Wastewater – economic asset in an urbanizing world, ed. P. Drechsel, M. Qadir and D. Wichelns, Springer, The Netherlands, 2015, DOI:10.1007/978-94-017-9545-6_2.
- UNESCO, 2017, UN World Water Development Report: Wastewater, the Untapped Resource, https://unesdoc.unesco.org/ark:/48223/pf0000247153_eng.
- FAO, More people, more food, worse water? a global review of water pollution from agriculture, 2018, http://www.fao.org/3/ca0146en/CA0146E.pdf.
- Boxall ABA. New and emerging water pollutants arising from agriculture, 2012, Paris, OECD, http://www.oecd.org/greengrowth/sustainable-agriculture/49848768.pdf.
- S. Bagchi, Arsenic threat reaching global dimensions, Can. Med. Assoc. J., 2007, 177(11), 1344–1345, DOI:10.1503/cmaj.071456.
- P. B. Tchounwou, C. G. Yedjou, A. K. Patlolla and D. J. Sutton, Heavy metals toxicity and the environment, Exper. Suppl., 2012, 101, 133–164, DOI:10.1007/978-3-7643-8340-4_6.
- The Project on emerging nanotechnologies, http://www.nanotechproject.org/.
- A. Ito, M. Shinkai, H. Honda and T. Kobayashi, Medical application of functionalized magnetic nanoparticles, J. Biosci. Bioeng., 2005, 100, 1–11, DOI:10.1263/jbb.100.1.
- E. Semenzin, E. Lanzellotto, D. Hristozov, A. Critto, A. Zabeo, E. Giubilato and A. Marcomini, Species sensitivity weighted distribution for ecological risk assessment of engineered nanomaterials: the n-TiO2 case study, Environ. Toxicol. Chem., 2015, 34, 2644–2659, DOI:10.1002/etc.3103.
- X. He, W. G. Aker, P. P. Fu and H.-M. Hwang, Toxicity of engineered metal oxide nanomaterials mediated by nano- bio- eco- interactions: A review and perspective, Environ. Sci.: Nano, 2015, 2, 564–582, 10.1039/C5EN00094G.
- I. A. Mudunkotuwa and V. H. Grassian, Biological and environmental media control oxide nanoparticle surface composition: The roles of biological components (proteins and amino acids), inorganic oxyanions and humic acid, Environ. Sci.: Nano, 2015, 2, 429–439, 10.1039/C4EN00215F.
- C. B. Park, J. W. Jung, M. Baek, B. Sung, J.-W. Park, Y. Seol and Y. J. Kim, Mixture toxicity of metal oxide nanoparticles and silver ions on Daphnia magna, J. Nanopart. Res., 2019, 21, 166, DOI:10.1007/s11051-019-4606-2.
- Q. Zhang, R. Xu, P. Xu, R. Chen, Q. He, J. Zhong and X. Gu, Performance study of ZrO2 ceramic micro-filtration membranes used in pretreatment of DMF wastewater, Desalination, 2014, 346, 1–8, DOI:10.1016/j.desal.2014.05.006.
- Y. Zhang, L. Yan, W. Xu, X. Guo, L. Cui, L. Gao, Q. Wei and B. Du, Adsorption of Pb (II) and Hg (II) from aqueous solution using magnetic CoFe2O4 reduced graphene oxide, J. Mol. Liq., 2014, 191, 177–182, DOI:10.1016/j.molliq.2013.12.015.
- X. Tang, Q. Zhang, Z. Liu, K. Pan, Y. Dong and Y. Li, Removal of Cu (II) by loofah fibers as a natural and low-cost adsorbent from aqueous solutions, J. Mol. Liq., 2014, 199, 401–407, DOI:10.1016/j.molliq.2013.11.034.
- A. K. Dutta, S. K. Maji and B. Adhikary, γ-Fe2O3 nanoparticles: an easily recoverable effective photo-catalyst for the degradation of rose Bengal and methylene blue dyes in the waste-water treatment plant, Mater. Res. Bull., 2014, 49, 28–34, DOI:10.1016/j.materresbull.2013.08.024.
- O. E. Gutierrez-Muñiz, G. García-Rosales, E. Ordoñez-Regil, M. T. Olguin and A. Cabral-Prieto, Synthesis, characterization and adsorptive properties of carbon with iron nanoparticles and iron carbide for the removal of As (V) from water, J. Environ.
Manage., 2013, 114, 1–7, DOI:10.1016/j.jenvman.2012.09.027.
- X. Yang, T. Zhoum, B. Ren, Z. Shi and A. Hursthouse, Synthesis, characterization, and adsorptive properties of Fe3O4/GO nanocomposites for antimony removal, J. Anal. Methods Chem., 2017, 3012364, DOI:10.1155/2017/3012364.
-
T. E. Cloete, M. de Kwaadsteniet and M. Botes, Nanotechnology in water treatment Applications, ed. J. M. Lopez-Romero, Caister Academic Press, New York, USA, 2010, p. 196, ISBN-13: 9781904455660 Search PubMed.
- Y. H. Li, J. Ding, Z. Luan, Z. Di, Y. Zhu, C. Xu, D. Wu and B. Wei, Competitive adsorption of Pb2+, Cu2+ and Cd2+ ions from aqueous solutions by multi walled carbon nanotubes, Carbon, 2003, 41, 2787–2792, DOI:10.1016/S0008-6223(03)00392-0.
- Y. H. Li, J. Ding, Z. Luan, Z. Di, Y. Zhu, C. Xu and D. Wu, Adsorption of cadmium (II) from aqueous solution by surface oxidized carbon nanotubes, Carbon, 2003, 41, 1057–1062, DOI:10.1016/S0008-6223(02)00440-2.
- V. K. Gupta, I. Tyagi, H. Sadegh, R. Shahryari-Ghoshekand, A. S. H. Makhlouf and B. Maazinejad, Nanoparticles as adsorbent; a positive approach for removal of noxious metal ions, a review, Science, Technology and Development, 2015, 34, 195, DOI:10.3923/std.2015.195.214.
- D. O'Carroll, B. Sleep, M. Krol, H. Boparai and C. Kocur, Nanoscale zero valent iron and bimetallic particles for contaminated site remediation, Adv. Water Resour., 2013, 51, 104–122, DOI:10.1016/j.advwatres.2012.02.005.
-
W. Stumm and J. J. Morgan, Aquatic Chemistry: Chemical Equilibria and Rates in Natural Waters, John Wiley & Sons, Inc., New York, 1996, 3rd edn, ISBN: 978-0-471-51185-4 Search PubMed.
- N. Zhu, H. Ji, P. Yu, J. Niu, M. U. Farooq, M. W. Akram, I. O. Udego, H. Li and X. Niu, Surface modification of magnetic iron oxide nanoparticles, Nanomaterials, 2018, 8, 10810, DOI:10.3390/nano8100810.
- A. Gankanda, N. S. Rentz and L. F. Greenlee, Influence of Ligand Size and Chelation Strength on Zerovalent Iron Nanoparticle Adsorption and Oxidation Behavior in the Presence of Water Vapor and Liquid Water, J. Phys. Chem. C, 2019, 123(4), 2474–2487, DOI:10.1021/acs.jpcc.8b07850.
- B. Desalegn, M. Megharaj, Z. Chen and R. Naidu, Green synthesis of zero valent iron nanoparticle using mango peel extract and surface characterization using XPS and GC-MS, Heliyon, 2019, 5(5), e01750, DOI:10.1016/j.heliyon.2019.e01750.
- K. S. Prasad, P. Gandhi and K. Selvaraj, Synthesis of green nano iron particles (GnIP) and their application in adsorptive removal of As (III) and As (V) from aqueous solution, Appl. Surf. Sci., 2014, 317, 1052–1059, DOI:10.1016/j.apsusc.2014.09.042.
- K. N. Thakkar, S. S. Mhatre and R. Y. Parikh, Biological synthesis of metallic nanoparticles, Nanomedicine, 2010, 6, 257–262, DOI:10.1016/j.nano.2009.07.002.
- S. S. Shankar, A. Rai, A. Ahmad and M. Sastry, Rapid synthesis of Au, Ag, and bimetallic Au core–Ag shell nanoparticles using Neem (Azadirachta indica) leaf broth, J. Colloid Interface Sci., 2004, 275, 496–502, DOI:10.1016/j.jcis.2004.03.003.
- S. S. Shankar, A. Rai, B. Ankamwar, A. Singh, A. Ahmad and M. Sastry, Biological synthesis of triangular gold nanoprisms, Nat. Mater., 2004, 3, 482–488, DOI:10.1038/nmat1152.
- V. Armendariz, I. Herrera, J. R. Peralta-Videa, M. Jose-Yacaman, H. Troiani and P. Santiago, Gardea-Torresdey JL Size controlled gold nanoparticle formation by Avena sativa biomass: use of plants in Nano biotechnology, J. Nanopart. Res., 2004, 6, 377–382, DOI:10.1007/s11051-004-0741-4.
- D. Cao, X. Jin, L. Gan, T. Wang and Z. Chen, Removal of phosphate using iron oxide nanoparticles synthesized by eucalyptus leaf extract in the presence of CTAB surfactant, Chemosphere, 2016, 159, 23–31, DOI:10.1016/j.chemosphere.2016.05.080.
- B. Desalegn, M. Megharaj, Z. Chen and R. Naidu, Green synthesis of zero valent iron nanoparticles using mango peel extract and surface characterization using XPS and GC-MS, Heliyon, 2019, 5(5), e01750, DOI:10.1016/j.heliyon.2019.e01750.
- N. C. Mueller, J. Braun, J. Bruns, M. Černík, P. Rissing, D. Rickerby and B. Nowack, Application of nanoscale zero valent iron (NZVI) for groundwater remediation in Europe, Environ. Sci. Pollut. Res., 2012, 19(2), 550–558, DOI:10.1007/s11356-011-0576-3.
- G. G. Valiyeva, I. Bavasso, L. Di Palma, S. R. Hajiyeva, M. A. Ramazanov and F. V. Hajiyeva, Synthesis of Fe/Ni Bimetallic Nanoparticles and Application to the Catalytic Removal of Nitrates from Water, Nanomaterials, 2019, 9(8), 1130, DOI:10.3390/nano9081130.
- J. Cao, D. Elliott and W. Zhang, Perchlorate reduction by nanoscale iron particles, J. Nanopart. Res., 2005, 7, 499–506, DOI:10.1007/s11051-005-4412-x.
- T. Wang, X. Jin, Z. Chen, M. Megharaj and R. Naidu, Green synthesis of Fe nanoparticles using eucalyptus leaf extracts for treatment of eutrophic wastewater, Sci. Total Environ., 2014, 466-467, 210–213, DOI:10.1016/j.scitotenv.2013.07.022.
- S. C. Ugochukwu, A. I. Uche and O. Ifeanyi, Preliminary phytochemical screening of different solvent extracts of stem bark and roots of Dennetia tripetala G Baker, Asian J. Plant Sci. Res., 2013, 3, 10–13 Search PubMed.
- G. E. Hoag, J. B. Collins, J. L. Holcomb, J. R. Hoag, M. N. Nadagouda and R. S. Varma, Degradation of bromothymol blue by ‘greener’ nanoscale zero-valent iron synthesize during tea polyphenols, J. Mater. Chem., 2009, 19, 8671–8677, 10.1039/B909148C.
- K. S. Mukunthan and S. Balaji, Silver nanoparticles shoot up from the root of Daucus carrota (L.), Int. J. Green Nanotechnol., 2012, 4, 54–61, DOI:10.1080/19430892.2012.654745.
- F. Luo, Z. Chen, M. Megharaj and R. Naidu, Biomolecules in grape leaf extract involved in one-step synthesis of iron-based nanoparticles, RSC Adv., 2014, 4, 53467–53474, 10.1039/C4RA08808E.
- M. N. Nadagouda, A. B. Castle, R. C. Murdock, S. M. Hussain and R. S. Varma, In vitro biocompatibility of nanoscale zerovalent iron particles (NZVI) synthesized using tea polyphenols, Green Chem., 2010, 12, 114, 10.1039/B921203P.
- S. Eslami, M. A. Ebrahimzadeh and P. Biparva, Green synthesis of safe zero valent iron nanoparticles by Myrtus communis leaf extract as an effective agent for reducing excessive iron in iron-overloaded mice, a thalassemia model, RSC Adv., 2018, 8(46), 26144–26155, 10.1039/C8RA04451A.
- M. Pattanayak and P. L. Nayak, Ecofriendly green synthesis of iron nanoparticles from various plants and spices extract, Int. J. Plant, Anim. Environ. Sci., 2013, 1, 2231–4490 Search PubMed.
- S. Najafian, M. Moradi and M. Sepehrimanesh, Polyphenolic contents and antioxidant activities of two medicinal plant species, Mentha piperita and Stevia rebaudiana, cultivated in Iran, Comp. Clin. Pathol., 2016, 25(4), 743–747, DOI:10.1007/s00580-016-2258-5.
- Isotopic fingerprint of phosphorus in drinking water (British), https://www.bgs.ac.uk/research/groundwater/dating/phosphorusIsotopes.html.
- Properties and contaminants of water- Drinking water- research, http://dwi.defra.gov.uk/private-watersupply/installations/Micro-and-Chem.pdf.
- Lead in drinking water - Thames Water, https://www.thameswater.co.uk/-/media/SiteContent/Thames-Water/Help-andAdvice/Download-our-lead-fact-pack.pdf.
- Drinking Water Inspectorate. Nitrate, http://dwi.defra.gov.uk/consumers/advice-leaflets/nitrate.pdf.
- Drinking Water Inspectorate. Chlorine, http://dwi.defra.gov.uk/consumers/advice-leaflets/chlorine.pdf.
-
B. Oram, Phosphate on the environment, https://water-research.net/index.php/phosphate-in-water.
- T. Almeelbi and A. N. Bezbaruah, Aqueous phosphate removal using nanoscale zerovalent iron, J. Nanopart. Res., 2012, 14(7), 1–14, DOI:10.1007/s11051-012-0900-y.
- W. X. Zhang, Nanoscale iron particles for environmental remediation: An overview, J. Nanopart. Res., 2003, 5, 323–332, DOI:10.1023/A:1025520116015.
- Y. H. Huang, Y. J. Shih, C. C. Chang and S. H. Chuang, A comparative study of phosphate removal technologies using adsorption and fluidized bed crystallization process, Desalin. Water Treat., 2011, 32(1–3), 351–356, DOI:10.5004/dwt.2011.2721.
- A. N. Bezbaruah, S. Krajangpan, B. J. Chisholm, E. Khan and J. J. Elorza Bermudez, Entrapment of iron nanoparticles in calcium alginate beads for groundwater remediation applications, J. Hazard. Mater., 2009, 166, 1339–1343, DOI:10.1016/j.jhazmat.2008.12.054.
- Y. H. Hwang, D. G. Kim and H. S. Shin, Mechanism study of nitrate reduction by nano zero valent iron, J. Hazard. Mater., 2011, 185, 1513–1521, DOI:10.1016/j.jhazmat.2010.10.078.
- T. Suzuki, M. Moribe, Y. Oyama and M. Niinae, Mechanism of nitrate reduction by zero-valent Iron: Equilibrium and kinetics studies, Chem. Eng. J., 2012, 183, 271–277, DOI:10.1016/j.cej.2011.12.074.
- J. Duggan and A. Dhawan, Speciation and vertical distribution of lead and lead shot in soil at a recreational firing range, Soil Sediment Contam., 2007, 16, 351–369, DOI:10.1080/15320380701404425.
- N. Farnad, R. Heidari and B. Aslanipour, Phenolic composition and comparison of antioxidant activity of alcoholic extracts of Peppermint (Mentha piperita), J. Food Meas. Charact., 2014, 8(2), 113–121, DOI:10.1007/s11694-014-9171-x.
- The Harmful Effects of Chlorine in Water, http://www.filterwater.com/articles.harmfuleffectsofchlorine.aspx.
-
N. Howlader, A. M. Noone, M. Krapcho, J. Garshell, D. Miller, S. F. Altekruse, C. L. Kosary, M. Yu, J. Ruhl and Z. Tatalovich, et al. SEER Cancer Statistics Review, 1975-2012, https://seer.cancer.gov/archive/csr/1975_2012/.
- Y.-L. Lin, P.-C. Chiang and E.-E. Chang, Removal of small trihalomethane precursors from aqueous solution by nanofiltration, J. Hazard. Mater., 2007, 146, 20–29, DOI:10.1016/j.jhazmat.2006.11.050.
- US EPA (2018). Edition of the drinking water standards and health advisories, EPA 822-R-04-005, Office of Water, U.S. Environmental Protection Agency, Washington, DC, 2004, https://www.epa.gov/sites/production/files/2018-03/documents/dwtable2018.pdf.
- WHO, Guidelines for Drinking-Water Quality, WHO, 3rd ed., 2003. ISBN: 978–92–4-154995-0.
- R. Singh, M. A. Shushni and A. Belkheir, Antibacterial and antioxidant activities of Mentha piperita L, Arabian J. Chem., 2015, 8(3), 322–328, DOI:10.1016/j.arabjc.2011.01.019.
Footnote |
† Electronic supplementary information (ESI) available. See DOI: 10.1039/d0ew00157k |
|
This journal is © The Royal Society of Chemistry 2020 |
Click here to see how this site uses Cookies. View our privacy policy here.