Emerging per- and polyfluoroalkyl substances (PFAS) in human milk from Sweden and China †
Received
13th February 2020
, Accepted 3rd September 2020
First published on 7th September 2020
Abstract
Twenty per- and polyfluoroalkyl substances (PFAS) were determined in human milk from residents of three Chinese cities (Shanghai, Jiaxing, and Shaoxing; [n = 10 individuals per city]), sampled between 2010 and 2016. These data were compared to a combination of new and previously reported PFAS concentrations in human milk from Stockholm, Sweden, collected in 2016 (n = 10 individuals). Across the three Chinese cities, perfluorooctanoate (PFOA; sum isomers), 9-chlorohexadecafluoro-3-oxanone-1-sulfonic acid (9Cl-PF3ONS; also known as 6:2 Cl-PFESA or by its trade name “F53-B”), and perfluorooctane sulfonate (PFOS; sum isomers) occurred at the highest concentrations among all PFAS (up to 411, 976, and 321 pg mL−1, respectively), while in Stockholm, PFOA and PFOS were dominant (up to 89 and 72 pg mL−1, respectively). 3H-Perfluoro-3-[(3-methoxy-propoxy)propanoic acid] (ADONA) was intermittently detected but at concentrations below the method quantification limit (i.e. <10 pg mL−1) in Chinese samples, and was non-detectable in Swedish milk. The extremely high concentrations of F53-B in Chinese milk suggest that human exposure assessments focused only on legacy substances may severely underestimate overall PFAS exposure in breastfeeding infants.
Environmental significance
Human milk is a significant source of exposure to per- and polyfluoroalkyl substances (PFAS) in breastfed infants. While many legacy PFAS are no longer produced, there are a growing number of alternative PFAS which have been introduced as replacements. To investigate the potential for infant exposure to these chemicals, we measured legacy and emerging PFAS in human milk from three cities in China (Shanghai, Jiaxing, and Shaoxing) and one city in Sweden (Stockholm). The legacy PFAS perfluorooctanoate (PFOA), perfluorooctane sulfonate (PFOS), along with the PFOS-replacement, 9-chlorohexadecafluoro-3-oxanone-1-sulfonic acid (trade name ‘F53-B’) occurred at the highest concentrations among all PFAS, with the latter substance only detected in Chinese cities. This work suggests that human exposure assessments focused only on legacy substances may severely underestimate overall exposure to PFAS.
|
Introduction
Per- and polyfluoroalkyl substances (PFAS) are a large and diverse group of anthropogenic chemicals that have been manufactured since the 1940s.1 Approximately 5000 PFAS-related CAS numbers are known to exist globally.2 Many PFAS contain perfluoroalkyl chains which impart unique properties, including extreme stability and combined oil/water repellency. These characteristics have made PFAS attractive for use in many industrial processes and consumer products. Unfortunately, due to their widespread use and recalcitrant nature, PFAS now occur in the blood of humans globally.3
For the most widely studied PFAS (i.e. the legacy PFAS perfluorooctane sulfonate (PFOS) and perfluorooctanoate (PFOA)), the main exposure routes for non-occupationally-exposed adults include food,4–7 drinking water,8 air9 and house dust.9,10 In infants, exposure to legacy PFAS occurs primarily from placental transfer while in the womb11–14 and breast milk thereafter.15–17 Even in children up to 3–5 years of age, PFAAs obtained from breast milk as an infant represent a significant fraction of their overall PFAS burden.18,19 This is clearly a concern, given the links between adverse health effects and PFAS exposure in children.20–22
Recent investigations of human milk from Stockholm, Sweden, revealed highly contrasting PFAS profiles for the time periods 1972–1996, 2000–2012, and 2013–2016, reflecting shifting exposure over time.17,23 Of particular note was the steady increase in concentrations of certain long-chain perfluoroalkyl carboxylic acids (PFCAs) and decline in concentrations of PFOS, PFOA, and perfluorooctane sulfonamide (FOSA). The latter result likely reflects regulation and phase-out initiatives while the former may indicate ongoing use of long-chain PFCAs and/or their precursors. Similar trends have been reported in human serum from countries around the world,24–27 including Sweden.28 However, in China, where PFOS production has continued under Stockholm Convention manufacture and use exemptions, a decline in the concentrations of PFOS in human blood has not been observed.29
Relatively little is known about human exposure to emerging PFAS ‘alternatives’ which have been introduced as replacements to substances such as PFOS and PFOA. The few data which exist suggest that these substances may already occur throughout the environment. For example, 2,3,3,3-tetrafluoro-2-(1,1,2,2,3,3,3-heptafluoropropoxy)propanoic acid (HFPO-DA; trade name ‘Gen-X’) was introduced in 2010 as a PFOA alternative and has been reported in surface water globally.30–33 Similarly, the replacement emulsifier 3H-perfluoro-3-[(3-methoxy-propoxy)propanoic acid] (ADONA), which has been used as a PFOA replacement since 2008 has been reported in both surface water and human serum from Germany.33,34 In China, the chlorinated polyfluorinated ether sulfonates (Cl-PFESAs) 9-chlorohexadecafluoro-3-oxanone-1-sulfonic acid (9Cl-PF3ONS; also known as 6:2 Cl-PFESA or the trade name trade name ‘F-53B’) and 11-chloroeicosafluoro-3-oxaundecane-1-sulfonic acid (11Cl-PF3OUdS; also known as 8:2 Cl-PFESA), are also an emerging concern. F-53B is a PFOS alternative, and has been used for over 30 years as a commercial mist suppressant by the Chinese electroplating industry.35 In addition to its widespread occurrence in surface water from China,33 Cl-PFESAs were observed in >98% of metal plating workers and high fish consumers sampled from the provinces of Shandong and Hube at concentrations up to 5040 ng mL−1.36 Most recently, Cl-PFESAs have been detected in marine mammal livers from Greenland37,38 and Sweden,38 polar bear serum from Hudson Bay and the Beaufort Sea,39 and human milk from Hangzhou, China.40
To shed further light on PFAS exposure in breastfed newborns, in particular to emerging PFAS, the current study investigated 20 PFAS (including the alternatives F53-B and ADONA) in human milk from residents of three Chinese cities (Shanghai, Jiaxing, and Shaoxing), sampled between 2010 and 2016. These data were compared to a combination of new and previously reported PFAS data from human milk from Stockholm, Sweden, collected in 2016. These data provide further insight on the variability in PFAS concentrations and profiles between cities and countries.
Experimental
Sampling
Samples from Stockholm (n = 10) were purchased in 2016 from the Mothers' Milk Centre at Stockholm South General Hospital. Milk was donated anonymously from healthy native Swedish mothers, which were non-smokers and predominantly primiparous. Sampling was carried out between 2 weeks and 3 months after delivery. Data from these samples were previously reported in Nyberg et al.,17 but were re-analyzed in the present work for F53-B and ADONA. Further information is provided in Table S1 of the ESI.†. Samples from Jiaxing (n = 10) and Shanghai (n = 10) were collected from 2015–2016, while samples from Shaoxing (n = 10) were collected in 2010. All participants were informed of the objectives of the study and signed a participant information and consent form. The study was approved by, and performed in accordance with guidelines from the Ethics Committee of China National Center for Food Safety Risk Assessment. Samples were initially stored at −18 °C in polyethylene sampling bags and bottles. Upon being shipped to the Environmental Specimen Bank (ESB) at the Swedish Museum of Natural History in Stockholm Sweden and the Yangtze Environmental Specimen Bank (YESB) at the Tongji Jiaxing Research Institution in Jiaxing China, samples were thawed, transferred to pre-washed glass bottles with lids covered with aluminum foil, and stored at −20 °C prior to analysis.
Standards and reagents
Linear isomer standards of perfluoroheptanoate (PFHpA), PFOA, perfluorononanoate (PFNA), perfluorodecanoate (PFDA), perfluorundecanoate (PFUnDA), perfluorododecanoate (PFDoDA), perfluorotridecanoate (PFTrDA), perfluorotetradecanoate (PFTeDA), 3-perfluoroheptyl propanoic acid (7:3 FTCA), perfluorobutane sulfonate (PFBS), perfluorohexane sulfonate (PFHxS), PFOS, perfluorodecane sulfonate (PFDS), perfluorooctanesulfonamide (FOSA), N-methyl perfluorooctane sulfonamidoacetic acid (MeFOSAA), N-ethyl perfluorooctane sulfonamidoacetic acid (EtFOSAA), potassium 9-chlorohexadecafluoro-3-oxanonane-1-sulfonate (9Cl-PF3ONS), potassium 11-chloroeicosafluoro-3-oxaundecane-1-sulfonate (11Cl-PF3OUdS), and sodium dodecafluoro-3H-4,8-dioxanonanoate (ADONA) were obtained from Wellington Labs (Guelph, ON, Canada). Perfluoropentanoate (PFPeA) and perfluorohexanoate (PFHxA) were investigated as part of Nyberg et al.17 (i.e. Stockholm samples from 2016), but since they were not measured in the Chinese samples, they were left out of the present analysis to ensure comparability of sum PFAS concentrations between Chinese and Swedish samples. All isotopically labeled standards were obtained from Wellington Labs (a full list is provided in Table S2, ESI†). Formic acid, ammonium sulfate, and ammonium acetate were obtained from Merck. Potassium hydroxide was purchased from VWR, methyl tert-butyl ether (HPLC grade) was purchased from RATHBURN (Walkerburn, Scotland) and acetonitrile was obtained from Honeywell. Standard Reference Material (SRM) 1954: Organic Contaminants in Fortified Human Milk, was obtained from the National Institute of Standards and Technology (NIST).
Chemical analysis
Sample extraction was carried out using a previously published method.17,23 Briefly, 2 mL of each sample was acidified with formic acid and then spiked with isotopically labeled internal standards. After vortexing, the sample was fortified with 600 μL of saturated ammonium sulfate, vortexed again, then fortified with 7 mL acetonitrile, and finally placed on a mechanical shaker. After 30 min of shaking, the samples were centrifuged and the top organic layer was transferred into a polypropylene tube and evaporated at 40 °C. The extract was then diluted with 300 μL of water and vortexed before adding 500 μL of 1 M potassium hydroxide. The tube was vortexed and then supplemented with 7 mL of methyl tert-butyl ether, then placed in a mechanical shaker for 20 minutes followed by centrifugation. The organic layer was subsequently transferred into a clean polypropylene tube. The extracts were evaporated at 40 °C and then fortified with 200 μL of buffer (1
:
2
:
1 ammonium acetate
:
acetonitrile
:
water). After vortexing and centrifugation, the lower layer was transferred to an Eppendorf tube and recovery standard was added. After vortexing, the mixture was transferred to microvial for analysis by UPLC-MS/MS.
Instrumental analysis was carried out on a Waters ultra-performance liquid chromatograph (UPLC) coupled to a Waters Xevo TQ-S triple quadrupole mass spectrometer operated in negative electrospray ionization, selected reaction monitoring mode. Instrumental parameters are provided in Table S2 (ESI†). Extracts were chromatographed on a BEH C18 analytical column (2.1 × 50 mm, 1.7 μm particle size, Waters) operated at a flow rate of 0.4 mL min−1, using a mobile phase composition of 90% water/10% acetonitrile containing 2 mM ammonium acetate (solvent A) and 100% acetonitrile containing 2 mM ammonium acetate (solvent B). The gradient profile is provided in Table S3 (ESI†). A total of two precursor/product ion transitions were monitored per analyte; one for quantification and the other for qualification (Table S2, ESI†).
Quantitative determination of target compounds was carried out by isotope dilution or an internal standard approach using a linear calibration curve with 1/x weighting. Branched isomers were determined semi-quantitatively using the calibration curve for the linear isomer. In almost all cases, the most sensitive ion was used for quantification. However, for PFOA, we observed an interference in the m/z 413/369 ion; therefore, the m/z 413/169 ion was used for quantification. For PFOS, concentrations are reported as the average of m/z 499/80 and 499/99 ions for both branched and linear isomers, as recommended by Riddell et al.41 Sum (∑) PFOS concentrations were obtained by summing the concentrations of branched and linear PFOS.
Quality control
Laboratory background contamination was monitored by including procedural blanks (2 mL Milli-Q water, n = 6) during the processing of human milk samples. Blank concentrations were generally below method detection limits for all targets but PFOA and FOSA (see Tables S4–S6, ESI†). For these substances, a higher reporting limit was adopted (see Data handling section). Method accuracy and precision were assessed using replicate samples of bovine milk analyzed with a low- (1–20 ng; n = 4), or high- (4–60 ng; n = 4) spike of PFAS (Table S7, ESI†). In most cases, mean percent recoveries ranged from 68 to 103% and 70 to 101% at low- and high-spiking levels, respectively. The exceptions were for PFBS and 7:3 FTCA, which displayed lower recoveries of 34/67%, 3.3/19% (low/high fortification levels), respectively, which was attributed to a lack of exactly-matched, isotopically-labelled internal standards. Finally, external validation of the method was achieved through comparison of PFAS concentrations measured in SRM 1954 (n = 3) to those reported by Nyberg et al.17 and by Keller et al.42 (Table S5, ESI†). In general, concentrations reported in SRM 1954 were consistent with Nyberg et al.17 and at the lower end of the range reported by Keller et al. (2010).42
Data handling
The limits of detection and quantification (LOD and LOQ, respectively) were defined as the concentration produced at a signal-to-noise ratio of 3 and 10, respectively, and are similar to those reported previously for PFAS in breast milk.23,40,43,44 In most cases, concentrations are reported down to the LOD. The exceptions were for PFOA and FOSA in Chinese samples, which were detectable in blanks, and therefore higher reporting limits were used (average blank + 3× the standard deviation of the blanks). Notably, reporting limits in Nyberg et al. were slightly lower than the present work, therefore PFAS in Swedish samples are in some cases detectable despite being below reporting limits used for Chinese samples. In all cases, values below the reporting limit were replaced with 0 for calculation of sum PFAS concentrations, estimated daily intakes (EDIs) and estimated weekly intakes (EWIs). For EDI and EWI calculations we assumed an infant body weight of 7 kg and a milk consumption rate of 600 mL day−1, as described previously.15
Results and discussion
PFAS profiles
Average Σ20PFAS concentrations, together with relative profiles and the concentrations of the top 9 most frequently detected PFAS are summarized in Fig. 1. Means, ranges, and detection frequencies for all PFAS are summarized in Table 1 (raw data in ESI Tables S4–S6†). The average Σ20PFAS concentrations in human milk (HM) were highest in Jiaxing (765 pg mL−1) followed by Shanghai (462 pg mL−1), Shaoxing (430 pg mL−1), and Stockholm (130 pg mL−1). The year of sampling (2015–2016 for Shanghai and Jiaxing; 2010 for Shaoxing; and 2016 for Stockholm) as well as maternal parity (n = 1 or 2 children for individuals from Shanghai and Jiaxing and n = 1 child for individuals from Shaoxing and Stockholm) may play an important role in the observed PFAS concentrations and profiles.17,45 However, due to the small number of individuals included in this study, the importance of these factors could not be investigated in detail. Despite this potential source of variability, some similarities were observed in PFAS profiles across all cities. For example, profiles in Jiaxing, Shanghai, Shaoxing, and Stockholm were all dominated by PFOS, PFOA, and long chain PFCAs (i.e. C9–C14), but in Chinese cities, 9Cl-PF3ONS (the main component of F53-B) was also prevalent, occurring in 100% of samples at concentrations of up to 976 pg mL−1. 11Cl-PF3OUdS (a minor component of F53-B) was also detected in most samples from Jiaxing and Shaoxing (up to 25 pg mL−1) and 3 samples from Shanghai (up to 87 pg mL−1). Neither 9Cl-PF3ONS or 11Cl-PF3OUdS were observed in samples from Stockholm. The occurrence of F53-B components exclusively in Chinese samples is not surprising since this product is produced and used exclusively in China. However, the highly elevated concentrations of 9Cl-PF3ONS, which exceeded PFOS in some samples, was unexpected and highlights the extreme bioaccumulative properties of this substance.36
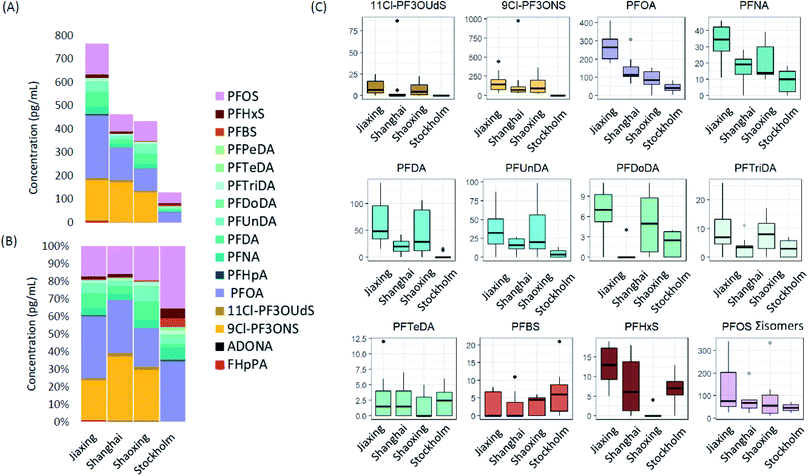 |
| Fig. 1 Sum mean concentrations (pg mL−1; Panel A) and profiles normalized to 100% (Panel B) for PFAS determined in human milk samples from Shanghai, Jiaxing, and Shaoxing (China) and Stockholm (Sweden). Box-whisker plots of individual concentrations are shown in Panel C. Boxes display 1st and 3rd quartiles bisected by the median while whiskers represent the interquartile range (excluding the median). For all panels, values below limits of detection were replaced with ‘0’. | |
Table 1 Average concentrations (pg mL−1) ± stdev and detection frequency (DF) of PFAS in mother's milk from Shanghai, Jiaxing and Shaoxing, China, and from Stockholm, Sweden. Range shown in parentheses
Analyte |
DF (%; all samples) |
Shanghai (n = 10) |
Jiaxing (n = 10) |
Shaoxing (n = 10) |
Stockholm (n = 10) |
Conc. |
DF (%) |
Conc. |
DF (%) |
Conc. |
DF (%) |
Conc. |
DF (%) |
L-PFHpA |
10 |
(<10) |
0 |
(<10–17) |
30 |
(<10) |
5 |
(<10–11) |
2.5 |
L-PFOA |
97.5 |
139 ± 36 (64–308) |
100 |
266 ± 37 (177–411) |
100 |
94 ± 21 (33–151) |
47.5 |
42 ± 12 (<2–81) |
25 |
Br-PFOA |
22.5 |
(<2–3) |
0 |
4 ± 1 (<2–8) |
50 |
2 ± 1 (<2–5) |
10 |
2 ± 1 (<2–6) |
7.5 |
L-PFNA |
90 |
17 ± 4 (<5–28) |
90 |
32 ± 6 (11–46) |
100 |
21 ± 6 (10–39) |
42.5 |
10 ± 3 (<5–18) |
17.5 |
L-PFDA |
70 |
21 ± 6 (<10–42) |
80 |
64 ± 21 (15–139) |
100 |
47 ± 21 (<10–106) |
27.5 |
<10 (<10–15) |
5 |
L-PFUnDA |
75 |
16 ± 4 (<5–27) |
80 |
38 ± 14 (<5–87) |
90 |
34 ± 16 (<5–99) |
35 |
7 ± 2 (<5–13) |
12.5 |
L-PFDoDA |
55 |
(<2–4) |
10 |
7 ± 2(2–11) |
80 |
6 ± 2 (<2–11) |
32.5 |
2 ± 1 (<2–4) |
15 |
L-PFTriDA |
70 |
4 ± 1 (<2–11) |
60 |
10 ± 4 (<2–26) |
80 |
8 ± 3 (<2–17) |
35 |
4 ± 1 (<2–7) |
15 |
L-PFTeDA |
50 |
3 ± 1 (<2–7) |
50 |
4 ± 2 (<2–12) |
50 |
3 ± 1 (<2–5) |
25 |
3 ± 1 (<2–6) |
15 |
L-PFPeDA |
10 |
(<2–3) |
10 |
(<2–4) |
10 |
2 ± 1 (<2–4) |
7.5 |
(<2) |
0 |
L-PFBS |
50 |
4 ± 1 (<4–11) |
30 |
5 ± 1 (<4–8) |
40 |
4 ± 1 (<4–6) |
32.5 |
7 ± 3 (<4–21) |
17.5 |
L-PFHxS |
65 |
8 ± 3 (<4–18) |
70 |
13 ± 3 (5–19) |
100 |
3 ± 0.3 (<4–4) |
22.5 |
7 ± 2 (<4–13) |
20 |
Br-PFHxS |
22.5 |
<2 (<2–3) |
30 |
<2 (<2–5) |
30 |
(<2) |
10 |
(<2–4) |
7.5 |
L-PFOS |
100 |
65 ± 22 (16–177) |
100 |
119 ± 52 (21–321) |
100 |
77 ± 45 (7–308) |
50 |
39 ± 7 (23–58) |
25 |
Br-PFOS |
87.5 |
8 ± 3 (<2–22) |
90 |
12 ± 4 (3–26) |
100 |
7 ± 4 (1–27) |
40 |
7 ± 2 (<1–14) |
20 |
L-PFDS |
2.5 |
(<2) |
0 |
(<2) |
0 |
(<2) |
5 |
(<2–3) |
2.5 |
Br-PFDS |
0 |
(<2) |
0 |
(<2) |
0 |
(<2) |
2.5 |
(<2) |
0 |
L-FOSA |
7.5 |
(<2–4) |
0 |
2 ± 0.5(<2–4) |
0 |
(<2–4) |
7.5 |
(<2–4) |
7.5 |
Br-FOSA |
0 |
(<2) |
0 |
(<2) |
0 |
(<2) |
2.5 |
(<2) |
0 |
L-MeFOSAA |
0 |
(<5) |
0 |
(<5) |
0 |
(<5) |
2.5 |
(<5) |
0 |
Br-MeFOSAA |
0 |
(<5) |
0 |
(<5) |
0 |
(<5) |
2.5 |
(<5) |
0 |
L-EtFOSAA |
0 |
(<5) |
0 |
(<5) |
0 |
(<5–8) |
5 |
(<5) |
0 |
Br-EtFOSAA |
0 |
(<5) |
0 |
(<5) |
0 |
(<5) |
2.5 |
(<5) |
0 |
7:3 FTCA |
20 |
(<2–4) |
10 |
7 ± 4 (<2–27) |
40 |
2 ± 1 (<2–8) |
10 |
(<2–3) |
2.5 |
ADONA |
20 |
3 ± 1 (<3–8) |
30 |
3 ± 1 (<3–8) |
30 |
(<3–5) |
7.5 |
(<3) |
0 |
9Cl-PF3ONS |
75 |
170 ± 144 (31–976) |
100 |
173 ± 65 (30–443) |
100 |
126 ± 56 (17–364) |
27.5 |
(<2) |
0 |
11Cl-PF3OUdS |
45 |
11 ± 13 (<2–87) |
30 |
10 ± 5 (<2–25) |
80 |
8 ± 4 (<2–22) |
20 |
(<2) |
0 |
Among the remaining PFAS, concentrations of PFOS, PFOA, PFNA, PFDA and PFUnDA were generally higher in Chinese cities compared to Stockholm, while concentrations of PFDoDA and PFTriDA were comparable (Fig. 1). Surprisingly, mean PFBS concentrations in Stockholm were slightly higher than Chinese cities, while PFHxS concentrations in Stockholm were comparable to Jiaxing and Shanghai and considerably higher than Shaoxing (Fig. 1). This may point to more prevalent use of PFBS and PFHxS-based formulations in Sweden compared to some locations in China. Contamination of drinking water with PFHxS and PFBS in some Swedish cities is also known to have occurred,8 which may (at least in part) explain the occurrence of these substances in Sweden. All other PFAS (i.e. PFHpA, Br-PFOA, L-PFPeDA, Br-PFHxS, PFDS, FOSA, MeFOSAA, EtFOSAA, 7:3 FTCA, and ADONA) were detected in <25% of all samples (i.e. from China and Sweden), and not exclusively in one city (Table 1). The infrequent detection of these substances precluded any firm conclusions regarding relative differences in exposure among Chinese cities, or between China and Sweden. However, it is notable that ADONA was detected (but below limits of quantification) in 8 of the 30 Chinese samples but none from Stockholm, while L-FOSA was detected in 3 of the 10 samples from Stockholm but none from China. To the best of our knowledge, this is the first observation of ADONA in human milk. However, given the very low concentrations (i.e. <8 pg mL−1), which fell below the LOQ (10 pg mL−1) this result remains tentative at this time.
To the best of our knowledge, Cl-PFESAs have only been reported in human milk once previously.40 In that work, samples from 174 woman from Hangzhou, China (2018 and 2019) contained ∑19PFAS concentrations ranging from 9–1755 pg mL−1 (mean 203 pg mL−1).40 9Cl-PF3ONS was detected in 100% of samples (consistent with the present study) while 11Cl-PF3OUdS was detected in only 20% of samples. Based on mean concentrations, PFOA was the dominant substance (87 pg mL−1) followed by PFHxA (41 pg mL; not measured in the present work), 9Cl-PF3ONS (28 pg mL−1), PFOS (25 pg mL−1), and perfluorobutanoate (24 pg mL−1; not measured in the present work). Mean concentrations of C9–C11 PFCAs ranged from 12–13 pg mL−1. Overall, the PFAS concentrations and profiles reported in Jin et al. (2020)40 are very similar to those reported in the present work for Shaoxing, which is perhaps not surprising considering the close proximity of these cities. Our work builds on this initial finding by showing that the occurrence of F-53B in human milk is widespread in China.
Estimated daily and weekly intakes and potential health risks
Mean Σ20PFAS EDIs were highest in Jiaxing (66 ng kg−1 bw d−1; range 30–116 ng kg−1 bw d−1) followed by Shanghai (40 ng kg−1 bw d−1; range 14–144 ng kg−1 bw d−1), Shaoxing (37 ng kg−1 bw d−1; range 5–71 ng kg−1 bw d−1), and finally Stockholm (11 ng kg−1 bw d−1; range 5–17 ng kg−1 bw d−1). In comparison, Winkens et al.46 reported ΣPFAS EDIs of 43 ng kg−1 bw d−1 for HM sampled from Spain (2008)47 and 29 ng kg−1 bw d−1 for HM from Sweden (2004)15 while Tao et al.48 estimated a ΣPFAS EDI of up to 87 ng kg−1 bw d−1 for HM collected in USA in 2004. The range of EDIs reported here for Shanghai are consistent with the results of Liu et al.,43 who reported a Σ6PFAS EDI of up to 129.1 ng kg−1 bw d−1 (median = 17.2 ng kg−1 d−1) for human milk sampled in 2007 from this city.
In 2008, the European Food Safety Authority (EFSA) established tolerable daily intakes (TDIs) for PFOS and PFOA of 150 and 1500 ng kg−1 bw d−1, respectively.49 These values were modified to tolerable weekly intakes (TWIs) of 13 and 6 ng kg−1 bw w−1, respectively, in 2018 (1.8 and 0.8 ng kg−1 bw d−1, respectively, assuming chronic intake),50 and most recently (2020) to a single TWI of 8 ng kg−1 bw w−1 based on the sum of four PFAS (PFOA, PFNA, PFHxS and PFOS). A comparison of the most recent TWIs to estimated weekly intakes (EWIs; based on Σ4PFAS; Table S9†) in the present work revealed that all samples exceeded the TWI of 8 ng kg−1 bw w−1. The highest EWI was for Jiaxing (average 260 ng kg−1 bw w−1; range 163–384 ng kg−1 bw w−1), followed by Shanghai (average 138 ng kg−1 bw w−1; range 63–314 ng kg−1 bw w−1), Shaoxing (average 118 ng kg−1 bw w−1; range 39–268 ng kg−1 bw w−1) and Stockholm (average 60 ng kg−1 bw w−1; range 26–89 ng kg−1 bw w−1). Unfortunately, TWIs have not been determined for most of the substances analyzed in this study. Such data are particularly important for the main components of F-53B which displayed very high concentrations in some Chinese HM samples. We note that some agencies have already started to define ΣPFAS threshold values based on a larger number of PFAS than what was used in the most recent EFSA estimates (e.g. the Swedish Drinking Water Guideline).51 Re-calculation of EWIs from the present work using Σ20PFAS concentrations revealed averages of 442, 271 and 250 ng kg−1 bw w−1 for Jiaxing, Shangahai and Shaoxing, respectively, and 73 ng kg−1 bw w−1 for Stockholm (Table 2), considerably higher than when only using Σ4PFAS concentrations.
Table 2 Range, mean, and median estimated weekly intakes (EWIs; ng kg−1 bw w−1) based on ∑20PFAS concentrations and assuming consumption of 600 mL day−1 and a body weight of 7 kg. Values below LOQ were set to 0 for EDI calculations
|
Shanghai (n = 10) |
Jiaxing (n = 10) |
Shaoxing (n = 10) |
Stockholm (n = 10) |
Range |
98–978 |
206–789 |
58–487 |
33–112 |
Mean |
271 |
447 |
255 |
75 |
Median |
204 |
391 |
227 |
72 |
Conclusions
Our analysis of human milk from China and Sweden revealed the presence of 9Cl-PF3ONS, 11Cl-PF3OUdS, and ADONA in human milk from China. To the best of our knowledge, there is only one prior study which has reported Cl-PFESAs in human milk.40 9-Cl-PF3ONS occurred at extremely high concentrations across all Chinese samples and suggest that human exposure assessments focused only on legacy substances may severely underestimate overall PFAS exposure in breastfeeding infants.
Conflicts of interest
There are no conflicts to declare.
Acknowledgements
Ms Yiwei Jiang (Yangtze ESB, Tongji Jiaxing Research Institution) is acknowledged for sampling human milk samples from Jiaxing. Mr Henrik Dahlgren (ESB, Swedish Museum of Natural History) is thanked for preparing the Swedish human milk samples. The study was financially supported by the Swedish Research Council (No. 639-2013-6913), and the Fundamental Research Funds for the Central Universities in China.
References
-
Swedish Chemicals Agency (KemI), Report 7/15, Occurrence and use of highly fluorinated substances and alternatives, 2015, accessed from: https://www.kemi.se/global/rapporter/2015/report-7-15-occurrence-and-use-of-highly-fluorinated-substances-and-alternatives.pdf Search PubMed.
-
Organisation for Economic Co-operation and Development (OECD), Toward a New Comprehensive Global Database of PFASs: Summary Report on updating the OECD 2007 List of Per- and polyfluoroalkyl substances (PFASs), 2018, Document ENV/JM/MONO(2018)7, accessed from: https://www.oecd.org/officialdocuments/publicdisplaydocumentpdf/?cote=ENV-JM-MONO(2018)7%26doclanguage=en Search PubMed.
- K. Kannan, S. Corsolini, J. Falandysz, G. Fillmann, K. S. Kumar, B. G. Loganathan, M. A. Mohd, J. Olivero, N. Van Wouwe, J. H. Yang and K. M. Aldoust, Perfluorooctanesulfonate and Related Fluorochemicals in Human Blood from Several Countries, Environ. Sci. Technol., 2004, 38(17), 4489–4495, DOI:10.1021/es0493446.
- R. Vestergren and I. T. Cousins, Tracking the Pathways of Human Exposure to Perfluorocarboxylates, Environ. Sci. Technol., 2009, 43(15), 5565–5575, DOI:10.1021/es900228k.
- L. S. Haug, S. Huber, G. Becher and C. Thomsen, Characterisation of Human Exposure Pathways to Perfluorinated Compounds--Comparing Exposure Estimates with Biomarkers of Exposure, Environ. Int., 2011, 37(4), 687–693, DOI:10.1016/j.envint.2011.01.011.
- Y. Tian, Y. Zhou, M. Miao, Z. Wang, W. Yuan, X. Liu, X. Wang, Z. Wang, S. Wen and H. Liang, Determinants of Plasma Concentrations of Perfluoroalkyl and Polyfluoroalkyl Substances in Pregnant Women from a Birth Cohort in Shanghai, China, Environ. Int., 2018, 119, 165–173, DOI:10.1016/j.envint.2018.06.015.
- G. Shan, Z. Wang, L. Zhou, P. Du, X. Luo, Q. Wu and L. Zhu, Impacts of Daily Intakes on the Isomeric Profiles of Perfluoroalkyl Substances (PFASs) in Human Serum, Environ. Int., 2016, 89–90, 62–70, DOI:10.1016/j.envint.2016.01.002.
- I. Gyllenhammar, J. P. Benskin, O. Sandblom, U. Berger, L. Ahrens, S. Lignell, K. Wiberg and A. Glynn, Perfluoroalkyl Acids (PFAAs) in Serum from 2-4-Month-Old Infants: Influence of Maternal Serum Concentration, Gestational Age, Breast-Feeding, and Contaminated Drinking Water, Environ. Sci. Technol., 2018, 52(12), 7101–7110, DOI:10.1021/acs.est.8b00770.
- K. Winkens, G. Giovanoulis, J. Koponen, R. Vestergren, U. Berger, A. M. Karvonen, J. Pekkanen, H. Kiviranta and I. T. Cousins, Perfluoroalkyl Acids and Their Precursors in Floor Dust of Children's Bedrooms - Implications for Indoor Exposure, Environ. Int., 2018, 119, 493–502, DOI:10.1016/j.envint.2018.06.009.
- S. Beesoon, S. J. Genuis, J. P. Benskin and J. W. Martin, Exceptionally High Serum Concentrations of Perfluorohexanesulfonate in a Canadian Family Are Linked to Home Carpet Treatment Applications, Environ. Sci. Technol., 2012, 46(23), 12960–12967, DOI:10.1021/es3034654.
- S. Beesoon, G. M. Webster, M. Shoeib, T. Harner, J. P. Benskin and J. W. Martin, Isomer Profiles of Perfluorochemicals in Matched Maternal, Cord, and House Dust Samples: Manufacturing Sources and Transplacental Transfer, Environ. Health Perspect., 2011, 119(11), 1659–1664, DOI:10.1289/ehp.1003265.
- K. B. Gützkow, L. S. Haug, C. Thomsen, A. Sabaredzovic, G. Becher and G. Brunborg, Placental Transfer of Perfluorinated Compounds is Selective--a Norwegian Mother and Child Sub-Cohort Study, Int. J. Hyg. Environ. Health, 2012, 215(2), 216–219, DOI:10.1016/j.ijheh.2011.08.011.
- L. Yang, Z. Wang, Y. Shi, J. Li, Y. Wang, Y. Zhao, Y. Wu and Z. Cai, Human Placental Transfer of Perfluoroalkyl Acid Precursors: Levels and Profiles in Paired Maternal and Cord Serum, Chemosphere, 2016, 144, 1631–1638, DOI:10.1016/j.chemosphere.2015.10.063.
- F. Chen, S. Yin, B. C. Kelly and W. Liu, Isomer-Specific Transplacental Transfer of Perfluoroalkyl Acids: Results from a Survey of Paired Maternal, Cord Sera, and Placentas, Environ. Sci. Technol., 2017, 51(10), 5756–5763, DOI:10.1021/acs.est.7b00268.
- A. Kärrman, I. Ericson, B. van Bavel, P. O. Darnerud, M. Aune, A. Glynn, S. Lignell and G. Lindström, Exposure of Perfluorinated Chemicals through Lactation: Levels of Matched Human Milk and Serum and a Temporal Trend, 1996–2004, in Sweden, Environ. Health Perspect., 2007, 115(2), 226–230, DOI:10.1289/ehp.9491.
- C. Kubwabo, I. Kosarac and K. Lalonde, Determination of Selected Perfluorinated Compounds and Polyfluoroalkyl Phosphate Surfactants in Human Milk, Chemosphere, 2013, 91(6), 771–777, DOI:10.1016/j.chemosphere.2013.02.011.
- E. Nyberg, R. Awad, A. Bignert, C. Ek, G. Sallsten and J. P. Benskin, Inter-Individual, Inter-City, and Temporal Trends of per- and Polyfluoroalkyl Substances in Human Milk from Swedish Mothers between 1972 and 2016, Environ. Sci.: Processes Impacts, 2018, 20(8), 1136–1147, 10.1039/c8em00174j.
- U. B. Mogensen, P. Grandjean, F. Nielsen, P. Weihe and E. Budtz-Jørgensen, Breastfeeding as an Exposure Pathway for Perfluorinated Alkylates, Environ. Sci. Technol., 2015, 49(17), 10466–10473, DOI:10.1021/acs.est.5b02237.
- E. Papadopoulou, A. Sabaredzovic, E. Namork, U. C. Nygaard, B. Granum and L. S. Haug, Exposure of Norwegian Toddlers to Perfluoroalkyl Substances (PFAS): The Association with Breastfeeding and Maternal PFAS Concentrations, Environ. Int., 2016, 94, 687–694, DOI:10.1016/j.envint.2016.07.006.
- C. C. Bach, B. H. Bech, N. Brix, E. A. Nohr, J. P. E. Bonde and T. B. Henriksen, Perfluoroalkyl and Polyfluoroalkyl Substances and Human Fetal Growth: A Systematic Review, Crit. Rev. Toxicol., 2015, 45(1), 53–67, DOI:10.3109/10408444.2014.952400.
- J. Lam, E. Koustas, P. Sutton, P. I. Johnson, D. S. Atchley, S. Sen, K. A. Robinson, D. A. Axelrad and T. J. Woodruff, The Navigation Guide - Evidence-Based Medicine Meets Environmental Health: Integration of Animal and Human Evidence for PFOA Effects on Fetal Growth, Environ. Health Perspect., 2014, 122(10), 1040–1051, DOI:10.1289/ehp.1307923.
-
National Toxicology Program (NTP), Monograph on immunotoxicity associated with exposure to perfluorooctanoic acid (PFOA) or perfluorooctane sulfonate (PFOS), 2016, accessed from: https://ntp.niehs.nih.gov/ntp/ohat/pfoa_pfos/pfoa_pfosmonograph_508.pdf Search PubMed.
- M. Sundström, D. J. Ehresman, A. Bignert, J. L. Butenhoff, G. W. Olsen, S.-C. Chang and Å. Bergman, A Temporal Trend Study (1972–2008) of Perfluorooctanesulfonate, Perfluorohexanesulfonate, and Perfluorooctanoate in Pooled Human Milk Samples from Stockholm, Sweden, Environ. Int., 2011, 37(1), 178–183, DOI:10.1016/j.envint.2010.08.014.
- E. Okada, I. Kashino, H. Matsuura, S. Sasaki, C. Miyashita, J. Yamamoto, T. Ikeno, Y. M. Ito, T. Matsumura, A. Tamakoshi and R. Kishi, Temporal Trends of Perfluoroalkyl Acids in Plasma Samples of Pregnant Women in Hokkaido, Japan, 2003-2011, Environ. Int., 2013, 60, 89–96, DOI:10.1016/j.envint.2013.07.013.
- U. Eriksson, J. F. Mueller, L.-M. L. Toms, P. Hobson and A. Kärrman, Temporal Trends of PFSAs, PFCAs and Selected Precursors in Australian Serum from 2002 to 2013, Environ. Pollut., 2017, 220(Part A), 168–177, DOI:10.1016/j.envpol.2016.09.036.
- T. H. Nøst, R. Vestergren, V. Berg, E. Nieboer, J. Ø. Odland and T. M. Sandanger, Repeated Measurements of Per- and Polyfluoroalkyl Substances (PFASs) from 1979 to 2007 in Males from Northern Norway: Assessing Time Trends, Compound Correlations and Relations to Age/Birth Cohort, Environ. Int., 2014, 67, 43–53, DOI:10.1016/j.envint.2014.02.011.
- G. W. Olsen, C. C. Lange, M. E. Ellefson, D. C. Mair, T. R. Church, C. L. Goldberg, R. M. Herron, Z. Medhdizadehkashi, J. B. Nobiletti, J. A. Rios, W. K. Reagen and L. R. Zobel, Temporal Trends of Perfluoroalkyl Concentrations in American Red Cross Adult Blood Donors, 2000-2010, Environ. Sci. Technol., 2012, 46(11), 6330–6338, DOI:10.1021/es300604p.
- A. Axmon, J. Axelsson, K. Jakobsson, C. H. Lindh and B. A. G. Jönsson, Time Trends between 1987 and 2007 for Perfluoroalkyl Acids in Plasma from Swedish Women, Chemosphere, 2014, 102, 61–67, DOI:10.1016/j.chemosphere.2013.12.021.
- M. Land, C. A. de Wit, A. Bignert, I. T. Cousins, D. Herzke, J. H. Johansson and J. W. Martin, What Is the Effect of Phasing out Long-Chain per- and Polyfluoroalkyl Substances on the Concentrations of Perfluoroalkyl Acids and Their Precursors in the Environment? A Systematic Review, Environ Evid., 2018, 7(1), 4, DOI:10.1186/s13750-017-0114-y.
- F. Heydebreck, J. Tang, Z. Xie and R. Ebinghaus, Alternative and Legacy Perfluoroalkyl Substances: Differences between European and Chinese River/Estuary Systems, Environ. Sci. Technol., 2015, 49(14), 8386–8395, DOI:10.1021/acs.est.5b01648.
- M. Sun, E. Arevalo, M. Strynar, A. Lindstrom, M. Richardson, B. Kearns, A. Pickett, C. Smith and D. R. U. Knappe, Legacy and Emerging Perfluoroalkyl Substances Are Important Drinking Water Contaminants in the Cape Fear River Watershed of North Carolina, Environ. Sci. Technol. Lett., 2016, 3(12), 415–419, DOI:10.1021/acs.estlett.6b00398.
- W. A. Gebbink, L. van Asseldonk and S. P. J. van Leeuwen, Presence of Emerging Per- and Polyfluoroalkyl Substances (PFASs) in River and Drinking Water near a Fluorochemical Production Plant in the Netherlands, Environ. Sci. Technol., 2017, 51(19), 11057–11065, DOI:10.1021/acs.est.7b02488.
- Y. Pan, H. Zhang, Q. Cui, N. Sheng, L. W. Y. Yeung, Y. Sun, Y. Guo and J. Dai, Worldwide Distribution of Novel Perfluoroether Carboxylic and Sulfonic Acids in Surface Water, Environ. Sci. Technol., 2018, 52(14), 7621–7629, DOI:10.1021/acs.est.8b00829.
- H. Fromme, M. Wöckner, E. Roscher and W. Völkel, ADONA and Perfluoroalkylated Substances in Plasma Samples of German Blood Donors Living in South Germany, Int. J. Hyg. Environ. Health, 2017, 220(2 Part B), 455–460, DOI:10.1016/j.ijheh.2016.12.014.
- S. Wang, J. Huang, Y. Yang, Y. Hui, Y. Ge, T. Larssen, G. Yu, S. Deng, B. Wang and C. Harman, First Report of a Chinese PFOS Alternative Overlooked for 30 Years: Its Toxicity, Persistence, and Presence in the Environment, Environ. Sci. Technol., 2013, 47(18), 10163–10170, DOI:10.1021/es401525n.
- Y. Shi, R. Vestergren, L. Xu, Z. Zhou, C. Li, Y. Liang and Y. Cai, Human Exposure and Elimination Kinetics of Chlorinated Polyfluoroalkyl Ether Sulfonic Acids (Cl-PFESAs), Environ. Sci. Technol., 2016, 50(5), 2396–2404, DOI:10.1021/acs.est.5b05849.
- W. A. Gebbink, R. Bossi, F. F. Rigét, A. Rosing-Asvid, C. Sonne and R. Dietz, Observation of Emerging Per- and Polyfluoroalkyl Substances (PFASs) in Greenland Marine Mammals, Chemosphere, 2016, 144, 2384–2391, DOI:10.1016/j.chemosphere.2015.10.116.
- K. Spaan, C. van Noordenburg, M. Plassmann, L. Schultes, S. D. Shaw, M. Berger, M. P. Heide-Jørgensen, A. Rosing-Asvid, S. Granquist, R. Dietz, C. Sonne, F. Rigét, A. Roos and J. Benskin, Fluorine Mass Balance and Suspect Screening in Marine Mammals from the Northern Hemisphere, Environ. Sci. Technol., 2020, 54(7), 4046–4058, DOI:10.1021/acs.est.9b06773.
- Y. Liu, E. S. Richardson, A. E. Derocher, N. J. Lunn, H.-J. Lehmler, X. Li, Y. Zhang, J. Y. Cui, L. Cheng and J. W. Martin, Hundreds of Unrecognized Halogenated Contaminants Discovered in Polar Bear Serum, Angew. Chem., Int. Ed., 2018, 57(50), 16401–16406, DOI:10.1002/anie.201809906.
- H. Jin, L. Mao, J. Xie, M. Zhao, X. Bai, J. Wen, T. Shen and P. Wu, Poly- and Perfluoroalkyl Substance Concentrations in Human Breast Milk and Their Associations with Postnatal Infant Growth, Sci. Total Environ., 2020, 713, 136417, DOI:10.1016/j.scitotenv.2019.136417.
- N. Riddell, G. Arsenault, J. P. Benskin, B. Chittim, J. W. Martin, A. McAlees and R. McCrindle, Branched Perfluorooctane Sulfonate Isomer Quantification and Characterization in Blood Serum Samples by HPLC/ESI-MS(/MS), Environ. Sci. Technol., 2009, 43(20), 7902–7908, DOI:10.1021/es901261v.
- J. M. Keller, A. M. Calafat, K. Kato, M. E. Ellefson, W. K. Reagen, M. Strynar, S. O'Connell, C. M. Butt, S. A. Mabury, J. Small, D. C. G. Muir, S. D. Leigh and M. M. Schantz, Determination of Perfluorinated Alkyl Acid Concentrations in Human Serum and Milk Standard Reference Materials, Anal. Bioanal. Chem., 2010, 397(2), 439–451, DOI:10.1007/s00216-009-3222-x.
- J. Liu, J. Li, Y. Zhao, Y. Wang, L. Zhang and Y. Wu, The Occurrence of Perfluorinated Alkyl Compounds in Human Milk from Different Regions of China, Environ. Int., 2010, 36(5), 433–438, DOI:10.1016/j.envint.2010.03.004.
- D. Lankova, O. Lacina, J. Pulkrabova and J. Hajslova, The Determination of Perfluoroalkyl Substances, Brominated Flame Retardants and Their Metabolites in Human Breast Milk and Infant Formula, Talanta, 2013, 117, 318–325, DOI:10.1016/j.talanta.2013.08.040.
- A. Ode, L. Rylander, C. H. Lindh, K. Källén, B. A. G. Jönsson, P. Gustafsson, P. Olofsson, S. A. Ivarsson and A. Rignell-Hydbom, Determinants of Maternal and Fetal Exposure and Temporal Trends of Perfluorinated Compounds, Environ. Sci. Pollut. Res., 2013, 20(11), 7970–7978, DOI:10.1007/s11356-013-1573-5.
- K. Winkens, R. Vestergren, U. Berger and I. T. Cousins, Early Life Exposure to Per- and Polyfluoroalkyl Substances (PFASs): A Critical Review, Emerging Contam., 2017, 3(2), 55–68, DOI:10.1016/j.emcon.2017.05.001.
- M. Llorca, M. Farré, Y. Picó, M. L. Teijón, J. G. Alvarez and D. Barceló, Infant Exposure of Perfluorinated Compounds: Levels in Breast Milk and Commercial Baby Food, Environ. Int., 2010, 36(6), 584–592, DOI:10.1016/j.envint.2010.04.016.
- L. Tao, K. Kannan, C. M. Wong, K. F. Arcaro and J. L. Butenhoff, Perfluorinated Compounds in Human Milk from Massachusetts, Environ. Sci. Technol., 2008, 42(8), 3096–3101, DOI:10.1021/es702789k.
- European Food Safety Authority (EFSA), Perfluoroalkylated Substances in Food: Occurrence and Dietary Exposure, EFSA J., 2012, 10(6), 2743, DOI:10.2903/j.efsa.2012.2743.
- EFSA CONTAM Panel (EFSA Panel on Contaminants in the Food Chain), H. K. Knutsen, J. Alexandere, L. Barregård, M. Bignami and B. Brüschweiler,
et al. Risk to Human Health Related to the Presence of Perfluorooctane Sulfonic Acid and Perfluorooctanoic Acid in Food, EFSA J., 2018, 16(12), 5194 Search PubMed.
-
Swedish National Food Agency, Riskhantering – PFAS i Dricksvatten Och Fisk, 2016, Last Update 2017-11-28, accessed from: https://www.livsmedelsverket.se/produktion-handel--kontroll/dricksvattenproduktion/riskhantering-pfaa-i-dricksvatten Search PubMed.
Footnote |
† Electronic supplementary information (ESI) available. See DOI: 10.1039/d0em00077a |
|
This journal is © The Royal Society of Chemistry 2020 |