DOI:
10.1039/C9CC10057A
(Communication)
Chem. Commun., 2020,
56, 3011-3014
Visible-light photoredox-catalyzed dual C–C bond cleavage: synthesis of 2-cyanoalkylsulfonylated 3,4-dihydronaphthalenes through the insertion of sulfur dioxide†
Received
30th December 2019
, Accepted 22nd January 2020
First published on 23rd January 2020
Abstract
An efficient novel visible-light photoredox-catalyzed dual carbon–carbon bond cleavage of methylenecyclopropanes and cycloketone oximes for the synthesis of 2-cyanoalkylsulfonated 3,4-dihydronaphthalenes through the insertion of sulfur dioxide is established. This dual cleavage of carbon–carbon bonds involves a radical pathway and goes through a sequence of iminyl radical formation, carbon–carbon bond cleavage, sulfur dioxide insertion, sulfonyl radical addition, another carbon–carbon bond cleavage, and intramolecular cyclization.
Sulfur-containing derivatives are important synthons in organic synthesis and drug synthesis.1,2 Sulfones, a class of useful organosulfur derivatives, have already been widely used in the chemical industry, agrochemicals, pharmaceuticals, organic synthesis and inflaming retarding materials.3,4 Due to the extensive application of sulfones, the approaches for the construction of sulfones have attracted organic chemists’ attention and many novel strategies have been established.5,6 Among them, the insertion of sulfur dioxide was the most convenient way for the synthesis of sulfones. DABSO [DABCO·(SO2)2],7 sodium sulfites8a–d and thiourea dioxide8e,f were usually used as sulfur dioxide sources to prepare sulfones.
Alkylnitriles are an important class of organic skeletons encountered in many nitrile-containing pharmaceuticals and natural products.9 Moreover, cyanoalkyl groups could easily be transformed into other functional groups.10 Thus, the development of efficient and convenient ways to access alkylnitriles has become interesting. Typical strategies to synthesize alkylnitriles include dehydrogenation of amines,11a–c dehydration of aldoximes11d–g or amides,12a–c and cyanation of alkyl halides.12d–g Recently, the functionalization of α-C–H bonds in inert alkylnitriles has also been developed.13 The cleavage of carbon–carbon σ-bonds in cyclobutanone oximes has also emerged as a convenient route to import cyanoalkyl groups, which bear longer aliphatic chains, into organic molecules.14–16 In 1991, Zard et al.14a developed the first radical carbon–carbon bond cleavage of cyclobutanone sulphenylimines or carboxymethyl oximes to prepare alkylnitriles. Afterwards, many organic chemists, for example, Uemura's,14b,c Selander's,14d,e Xiao's,14f–j Guo's,15a–c Yu's,15d,e Wu's,15f,g Li's15h and other groups,16 have reported similar ring-opening reactions of cyclobutanone oxime derivatives. Most of these methods employed cyanoalkyl radicals, which came from ring-opening of cyclobutanone oximes, to directly react with other reaction partners. However, the methods which used cyanoalkyl radicals to capture SO216e,f and then formed sulfonyl radicals were very few (eqn (1), Scheme 1).
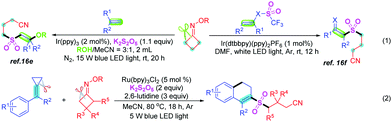 |
| Scheme 1
In situ SO2-capture reactions of cycloketone oxime esters. | |
MCPs (methylenecyclopropanes) were usually used to react with diverse free radicals,17 such as CF3,17a SCF3,17b alkyl,17c acyl,17dα-carbonyl17e and sulfonyl17f–h-containing radicals. In the reaction between MCPs and sulfonyl radicals, the sulfonyl radicals usually came from sulfonyl chlorides and sodium sulfinates. However, strategies for ring-opening and cyclization of MCPs with cyanoalkylsulfonyl radicals, which formed from cyclobutanone oximes and sulfur dioxide sources, are lacking.
Visible-light-catalysis, as a convenient and simple tool, is widely used in organic synthesis because of its safety, mild reaction conditions, availability, and high efficiency.18 Herein, we develop a visible-light photoredox-catalyzed difunctionalization of carbon–carbon σ-bonds to construct 2-cyanopropylsulfonyl-substituted 3,4-dihydronaphthalenes from MCPs, cyclobutanone oximes and K2S2O5, which features the cleavage of two carbon–carbon σ-bonds and the formation of one new carbon–carbon bond and two carbon-sulfur bonds in one-pot (eqn (2), Scheme 1).
We chose 1-(benzyloxy)-2-(cyclopropylidenemethyl)benzene 1a and cyclobutylidenemethyl 4-(trifluoromethyl)benzoate 2a as model substrates to clarify the standard reaction conditions. To our surprise, the reaction employing Ru(bpy)3Cl2 as a photocatalyst, K2S2O5 as a sulfur dioxide source, 2,6-lutidine as base, and CH3CN as solvent at 80 °C and irradiated with a 5 W blue LED light source for 18 h could afford the product 4-((8-(benzyloxy)-3,4-dihydronaphthalen-2-yl)sulfonyl)butanenitrile 3aa in 81% yield. Various sulfur dioxide sources, cyclobutanone O-benzoyl oximes, photocatalysts, solvents, visible-light sources, temperatures and reaction times were successively examined, and the optimized conditions were as follows: 1-(benzyloxy)-2-(cyclopropylidenemethyl)benzene 1a (0.2 mmol), cyclobutylidenemethyl 4-(trifluoromethyl)benzoate 2a (0.3 mmol, 1.5 equiv.), Ru(bpy)3Cl2 (0.01 mmol, 5 mol%), 2,6-lutidine (0.6 mmol, 3 equiv.), K2S2O5 (0.4 mmol, 2 equiv.), CH3CN (2 mL) at 80 °C and irradiated with a 5 W blue LED light source for 18 h (see Table S1 in the ESI†).
Based on the optimal conditions, we initially investigated the scope of MCPs 1 and cycloketone oxime esters 2 (Table 1). A series of substituted cycloketone oxime esters 2 were examined in the presence of MCPs 1a under the standard conditions. The results revealed that 3-substituted cyclobutanone oximes 2b–j bearing benzyl, benzyloxy, aryl and naphthalen-1-ylmethyl groups were all suitable substrates. The corresponding products 3ab–aj could be obtained in moderate yields. Employing 3,3-disubstituted cyclobutanone oximes 1k and 1l in the reaction could provide the sulfonated products 3ak and 3al in 79% and 72% yields, respectively. The 2-substituted cycloketone oxime ester 2m was examined and the target product 3am could be obtained in 69% yield. Then, our attention was turned to research the scope of MCPs 1 in the presence of cycloketone oxime esters 2a, Ru(bpy)3Cl2, K2S2O5, 2,6-lutidine and CH3CN. A variety of mono-substituted MCPs, which bear one substituent at the ortho-, meta- or para-position on the aryl ring, were examined. All of them could undergo the dual carbon–carbon bond cleavage/sulfonylation reaction smoothly and afforded the corresponding 2-cyanoalkylsulfonylated 3,4-dihydronaphthalenes 3 in moderate yields (3aa–na). The reaction yields indicated that both the hindrance and electronic effect of the substituents had obvious influences on the transformation: the reactivity order is ortho- < meta- < para- and electron-withdrawing < electron-donating. However, the meta-substituted MCP 1e gave products 3ea and 3ea′ (3
:
1) in 83% yield. Subsequently, di-substituted MCPs 1o–r and poly-substituted MCP 1s were also explored and all of them were suitable for this reaction (products 3oa–sa). Halogen substituted MCPs 1p–s were suitable for this transformation, which provided opportunities for further modification of the products (products 3pa–sa). Additionally, phenyl-substituted MCP 1t was also a good candidate for this reaction. However, the four-membered substrate 1u could not install the target product 3ua. Substrate 1v, which connected a phenyl group and a methyl, could react well with 2a to deliver the sulfonylated product 3va in 72% yield. The naphthyl-substituted MCP 1w could also deliver product 3wa under the standard conditions (product 3wa). Finally, a series of MCPs 1x–aa connecting two aryl rings at the terminal of the carbon–carbon double bond also worked well and afforded the ring-opening and cyclization products 3xa–3aaa in good yields. However, 1-Cbz-3-azetidinone, oxetan-3-one and cyclopentanone derived O-acyl oximes 2n, 2o and 2p could not give the target products 3 under the standard conditions.
Table 1 Scope of cycloketone oxime esters (2) and MCPs (1)a
Reaction conditions: 1 (0.2 mmol), 2a (0.3 mmol, 1.5 equiv.), K2S2O5 (0.4 mmol, 2 equiv.), Ru(bpy)3Cl2 (5 mol%), 2,6-lutidine (0.6 mmol, 3 equiv.), CH3CN (2 mL) at 80 °C under an argon atmosphere and 5 W blue LED irradiation for 18 h.
Most of the substrate 1 was decomposed.
|
|
To gain further understanding of the reaction mechanism, three radical trapping experiments were carried out. The yields of MCPs 1a with cycloketone oxime esters 2a and K2S2O5 were very low when these reactions were carried out by using TEMPO, hydroquinone and 1,1-diphenylethene as radical inhibitors (eqn (1)–(3), respectively, Scheme 2). Additionally, using TEMPO (2,2,6,6-tetramethylpiperidine-1-oxyl) in the transformation could afford 4-((2,2,6,6-tetramethylpiperidin-1-yl)oxy)butanenitrile 4 (eqn (1), Scheme 2). The trapping product 4 could be detected by GC-MS. Using 1,1-diphenylethene in the sulfonylation reaction could give cyanoalkyl group-trapping product 6,6-diphenylhex-5-enenitrile 5 and cyanoalkylsulfonyl group-trapping product 4-((2,2-diphenylvinyl)sulfonyl)butanenitrile 6 in 11% and 27% yields, respectively (eqn (3), Scheme 2). These experimental results suggested that this transformation definitely contained a radical process. Additionally, the cyano group could be transformed into a series of other functional groups, such as carboxyl,14g,15b,16a amide,16a ester,16a and tetrazole15b groups. The cyano group in the sulfonylated product 3aa could be converted into an ester group in the presence of ZnCl2 and n-butyl alcohol (eqn (4), Scheme 2).
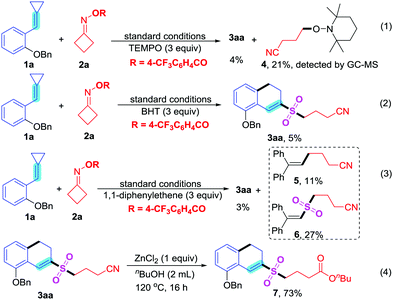 |
| Scheme 2 Control experiments. | |
According to the literature14–18 and above results, we proposed a mechanism for the visible-light photoredox-catalyzed dual cleavage of C–C bonds (Scheme 3). Initially, [Ru(bpy)3]2+ was transformed into [Ru(bpy)3]2+* photoexcited under irradiation with visible-light.17f,18c Subsequently, the reduction of cycloketone oxime 2a by [Ru(bpy)3]2+* provided iminyl radical A, which underwent cleavage of the carbon–carbon bond to deliver cyanoalkyl radical B. Next, intermediate B trapped sulfur dioxide, which came from K2S2O5, to give cyanoalkylsulfonyl radical C. Both the cyanoalkyl radical B and cyanoalkylsulfonyl radical C could be trapped by 1,1-diphenylethene to give products 5 and 6, respectively. Then, the addition of cyanoalkylsulfonyl radical C to the carbon–carbon double bond in substrate 1a afforded radical D, which went through another cleavage of the carbon–carbon bond to install radical E. The intramolecular cyclization of intermediate E assembled radical F. Finally, radical F underwent SET by [Ru(bpy)3]3+ and deprotonation by 2,6-lutidine to provide the target product 3aa, and [Ru(bpy)3]3+ was reverted to ground-state [Ru(bpy)3]2+.
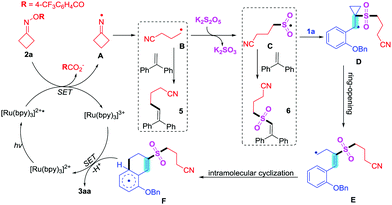 |
| Scheme 3 Possible reaction mechanism. | |
In summary, we have developed a facile and convenient route to diverse 2-cyanoalkylsulfonated 3,4-dihydronaphthalenes via visible-light photoredox-catalyzed dual C–C bond cleavage of methylenecyclopropanes and cycloketone oxime esters by the insertion of sulfur dioxide. This reaction follows a radical pathway and goes through a sequence of iminyl radical formation, C–C bond cleavage, sulfur dioxide insertion, sulfonyl radical addition, another C–C bond cleavage, and cyclization. Both the cyanoalkyl radical and cyanoalkylsulfonyl radical were trapped by radical inhibitors. Further research and application of this dual C–C bond cleavage reaction are currently underway in our laboratory.
We thank Hunan Provincial Innovation Foundation for Postgraduates (CX20190921), the Natural Science Foundation of Hunan Province (No. 2018JJ3208) and the National Natural Science Foundation of China (No. 21602056, 51874132 and 21805081) for financial support.
Conflicts of interest
There are no conflicts to declare.
Notes and references
-
(a) M. Wang and X.-F. Jiang, Top. Curr. Chem., 2018, 376, 14 CrossRef PubMed
;
(b) H. Min, G.-H. Xiao, W.-J. Liu and Y. Liang, Org. Biomol. Chem., 2016, 14, 11088 RSC
;
(c) C. Wu, L.-H. Lu, A.-Z. Peng, G.-K. Jia, C. Peng, Z. Cao, Z. Tang, W.-M. He and X. Xu, Green Chem., 2018, 20, 3683 RSC
;
(d) P. Dang, Z.-L. Zheng and Y. Liang, J. Org. Chem., 2017, 82, 2263 CrossRef CAS PubMed
;
(e) Z.-J. Qiao and X.-F. Jiang, Org. Lett., 2016, 18, 1550 CrossRef CAS PubMed
;
(f) Y.-M. Li, M. Wang and X.-F. Jiang, ACS Catal., 2017, 7, 7587 CrossRef CAS
;
(g) W.-J. Liu, H. Min, X.-M. Zhu, G.-B. Deng and Y. Liang, Org. Biomol. Chem., 2017, 15, 9804 RSC
.
-
(a) Y.-M. Li, W.-S. Xie and X.-F. Jiang, Chem. – Eur. J., 2015, 21, 16059 CrossRef CAS PubMed
;
(b) G.-Z. Li, H. Xie, J.-J. Chen, Y.-J. Guo and G.-J. Deng, Green Chem., 2017, 19, 4043 RSC
;
(c) Y.-H. Zhang, Y.-M. Li, X.-M. Zhang and X.-F. Jiang, Chem. Commun., 2015, 51, 941 RSC
;
(d) Z.-J. Qiao, N.-Y. Ge and X.-F. Jiang, Chem. Commun., 2015, 51, 10295 RSC
;
(e) Y.-J. Xie, X.-G. Chen, Z. Wan, H.-W. Huang, B. Yi and G.-J. Deng, Green Chem., 2017, 19, 4294 RSC
.
-
(a) S. Shaaban, S. Liang, N.-W. Liu and G. Manolikakes, Org. Biomol. Chem., 2017, 15, 1947 RSC
;
(b) C. M. Rayner, Contemp. Org. Synth., 1994, 1, 191 RSC
;
(c) D.-C. Meadows and J. Gervay-Hague, Med. Res. Rev., 2006, 26, 793 CrossRef CAS PubMed
;
(d) Y. M. Markitanov, V.-M. Timoshenko and Y.-G. Shenko, J. Sulfur Chem., 2014, 35, 188 CrossRef CAS
;
(e) H. Fukuda, F. J. Frank and W. E. Truce, J. Org. Chem., 1963, 28, 1420 CrossRef CAS
;
(f) Y.-Y. Fang, Z.-G. Luo and X.-M. Xu, RSC Adv., 2016, 6, 59661 RSC
;
(g) A. N. R. Alba, X. Companyóa and R. Rios, Chem. Soc. Rev., 2010, 39, 2018 RSC
.
-
(a) W.-H. Rao, B.-B. Zhan, K. Chen, P.-X. Ling, Z.-Z. Zhang and B.-F. Shi, Org. Lett., 2015, 17, 3552 CrossRef CAS PubMed
;
(b) M.-J. CabreraAfonso, Z.-P. Lu, C.-B. Kelly, S.-B. Lang, R. Dykstra, O. Gutierrez and G.-A. Molander, Chem. Sci., 2018, 9, 3186 RSC
;
(c) R.-J. Song, Y. Liu, Y.-Y. Liu and J.-H. Li, J. Org. Chem., 2011, 76, 1001 CrossRef CAS PubMed
;
(d) L.-Y. Xie, S. Peng, F. Liu, G.-R. Chen, W. Xia, X.-Y. Yu, W.-F. Li, Z. Cao and W.-M. He, Org. Chem. Front., 2018, 5, 2604 RSC
;
(e) F.-H. Xiao, C. Liu, S.-S. Yuan, H.-W. Huang and G.-J. Deng, J. Org. Chem., 2018, 83, 10420 CrossRef CAS PubMed
;
(f) B. A. Howell and Y. G. Daniel, J. Fire Sci., 2018, 36, 518 CrossRef CAS
.
- For reviews:
(a)
D. Zheng and J. Wu, Sulfur Dioxide Insertion Reactions for Organic Synthesis, Nature Springer, Berlin, 2017 CrossRef
;
(b) J.-D. Liu and L.-Y. Zheng, Adv. Synth. Catal., 2019, 361, 1710 CrossRef CAS
;
(c) S.-Q. Ye, G.-Y.-S. Qiu and J. Wu, Chem. Commun., 2019, 55, 1013 RSC
;
(d) G.-Y.-S. Qiu, L.-F. Lai, J. Cheng and J. Wu, Chem. Commun., 2018, 54, 10405 RSC
;
(e) G.-Y.-S. Qiu, K.-D. Zhou, L. Gao and J. Wu, Org. Chem. Front., 2018, 5, 691 RSC
;
(f) J. Zhu, W.-C. Yang, X.-D. Wang and L. Wu, Adv. Synth. Catal., 2018, 360, 386 CrossRef CAS
;
(g) G. Liu, C.-B. Fan and J. Wu, Org. Biomol. Chem., 2015, 13, 1592 RSC
.
-
(a) Y.-C. Wu, S.-S. Jiang, S.-Z. Luo, R.-J. Song and J.-H. Li, Chem. Commun., 2019, 55, 8995 RSC
;
(b) X.-Y. He, X. Yue, L. Zhang, S. Wu, M. Hu and J.-H. Li, Chem. Commun., 2019, 55, 3517 RSC
;
(c) J. Yang, Y.-Y. Liu, R.-J. Song, Z.-H. Peng and J.-H. Li, Adv. Synth. Catal., 2016, 358, 2286 CrossRef CAS
;
(d) W. Su, Tetrahedron Lett., 1994, 35, 4955 CrossRef CAS
;
(e) M. Rahimizadeha, G. Rajabzadehb, S.-M. Hossein and E. AliShiri, J. Mol. Catal. A: Chem., 2010, 323, 59 CrossRef
;
(f) A. Rostami and J. Akradi, Tetrahedron Lett., 2010, 51, 3501 CrossRef CAS
.
-
(a) Y. Wang, L.-L. Deng, J. Zhou, X.-C. Wang, H.-B. Mei, J.-L. Han and Y. Pan, Adv. Synth. Catal., 2018, 360, 1060 CrossRef CAS
;
(b) A. S. Deeming, C. J. Russell, A. J. Hennessy and M. C. Willis, Org. Lett., 2014, 16, 150 CrossRef CAS PubMed
;
(c) H.-B. Zhu, Y.-J. Shen, Q.-Y. Deng, C.-Y. Huang and T. Tu, Chem. – Asian J., 2017, 12, 706 CrossRef CAS PubMed
;
(d) A. S. Deeming, C. J. Russell and M. C. Willis, Angew. Chem., Int. Ed., 2016, 55, 747 CrossRef CAS PubMed
;
(e) H. Woolven, C. González-Rodríguez, I. Marco, A. L. Thompson and M. C. Willis, Org. Lett., 2011, 13, 4876 CrossRef CAS PubMed
;
(f) V. Vedovato, E. P. A. Talbot and M. C. Willis, Org. Lett., 2018, 20, 5493 CrossRef CAS PubMed
.
-
(a) X.-X. Gong, X.-F. Li, W.-L. Xie, J. Wu and S.-Q. Ye, Org. Chem. Front., 2019, 6, 1863 RSC
;
(b) Y.-W. Li, T. Liu, G.-Y.-S. Qiu and J. Wu, Adv. Synth. Catal., 2019, 361, 1154 CrossRef CAS
;
(c) X.-X. Gong, J.-H. Chen, L.-F. Lai, J. Cheng, J.-T. Sun and J. Wu, Chem. Commun., 2018, 54, 11172 RSC
;
(d) M. Wang, S.-H. Chen and X.-F. Jiang, Org. Lett., 2017, 19, 4916 CrossRef CAS PubMed
;
(e) S. Kumar, S. Verma, S. L. Jain and B. Sain, Tetrahedron Lett., 2011, 52, 3393 CrossRef CAS
;
(f) S.-Q. Ye, Y.-W. Li, J. Wu and Z.-M. Li, Chem. Commun., 2019, 55, 2489 RSC
.
-
(a) F. F. Fleming, Nat. Prod. Rep., 1999, 16, 597 RSC
;
(b) F. F. Fleming, L. Yao, P. C. Ravikumar, L. Funk and B. C. Shook, J. Med. Chem., 2010, 53, 7902 CrossRef CAS PubMed
;
(c) X.-S. Wu, J. Riedel and V. M. Dong, Angew. Chem., Int. Ed., 2017, 56, 11589 CrossRef CAS PubMed
;
(d) Y.-H. He, Y.-Q. Wang, J. Gao, S.-S. Li, W. Wang, X.-H. Zheng, S.-Y. Zhang, L.-J. Gu and G.-P. Li, Chem. Commun., 2018, 54, 7499 RSC
;
(e) Y. Li, J.-Q. Shang, X.-X. Wang, W.-J. Xia, T. Yang, Y.-C. Xin and Y.-M. Li, Org. Lett., 2019, 21, 2227 CrossRef CAS PubMed
;
(f) W. Zhang, Y.-L. Pan, C. Yang, X. Li and B. Wang, Org. Chem. Front., 2019, 6, 2765 RSC
.
-
(a) R. López and C. Palomo, Angew. Chem., Int. Ed., 2015, 54, 13170 CrossRef PubMed
;
(b)
A. J. Fatiadi, Preparation and Synthetic Application of Cyano Compounds, ed. S. Patai and Z. Rappaport, Wiley, New York, 1983 Search PubMed
;
(c)
R. C. Larock, Comprehensive Organic Transformations: A Guide to Functional Group Preparations, Wiley-VCH, New York, 2nd edn, 1988 Search PubMed
.
-
(a) K.-N. T. Tseng, A. M. Rizzi and N. K. Szymczak, J. Am. Chem. Soc., 2013, 135, 16352 CrossRef CAS PubMed
;
(b) I. Dutta, S. Yadav, A. Sarbajna, S. De, M. Hölscher, W. Leitner and J. K. Bera, J. Am. Chem. Soc., 2018, 140, 8662 CrossRef CAS PubMed
;
(c) L. V. A. Hale, T. Malakar, K.-N. T. Tseng, P. M. Zimmerman, A. Paul and N. K. Szymczak, ACS Catal., 2016, 6, 4799 CrossRef CAS
;
(d) L. Yu, H.-Y. Li, X. Zhang, J.-Q. Ye, J.-P. Liu, Q. Xu and M. Lautens, Org. Lett., 2014, 16, 1346 CrossRef CAS PubMed
;
(e) Y.-L. Ban, J.-L. Dai, X.-L. Jin, Q.-B. Zhang and Q. Liu, Chem. Commun., 2019, 55, 9701 RSC
;
(f) X. Zhang, J.-J. Sun, Y.-H. Ding and L. Yu, Org. Lett., 2015, 17, 5840 CrossRef CAS PubMed
;
(g) R. Ding, Y.-G. Liu, M.-R. Han, W.-Y. Jiao, J.-Q. Li, H.-Y. Tian and B.-G. Sun, J. Org. Chem., 2018, 83, 12939 CrossRef CAS PubMed
.
-
(a) M. H. Al-Huniti and M. P. Croatt, Asian J. Org. Chem., 2019, 8, 1791 CrossRef CAS
;
(b) S.-L. Zhou, D. Addis, S. Das, K. Junge and M. Beller, Chem. Commun., 2009, 4883 RSC
;
(c) Y.-Y. Wang, L.-Y. Fan, H.-M. Qi, S.-W. Chen and Y.-H. Li, Asian J. Org. Chem., 2018, 7, 367 CrossRef CAS
;
(d) G. P. Ellis and T. M. Romney-Alexander, Chem. Rev., 1987, 87, 779 CrossRef CAS
;
(e) Y. Ueda, N. Tsujimoto, T. Yurino, H. Tsurugi and K. Mashima, Chem. Sci., 2019, 10, 994 RSC
;
(f) A.-Y. Xia, X. Xie, H.-Y. Chen, J.-D. Zhao, C.-L. Zhang and Y.-H. Liu, Org. Lett., 2018, 20, 7735 CrossRef CAS PubMed
;
(g) L. Yang, Y.-T. Liu, Y. Park, S.-W. Park and S. Chang, ACS Catal., 2019, 9, 3360 CrossRef CAS
.
-
(a) Y. Li, B. Liu, H.-B. Li, Q. Wang and J.-H. Li, Chem. Commun., 2015, 51, 1024 RSC
;
(b) X.-H. Ouyang, M. Hu, R.-J. Song and J.-H. Li, Chem. Commun., 2018, 54, 12345 RSC
;
(c) S.-S. Jiang, Y.-C. Wu, S.-Z. Luo, F. Teng, R.-J. Song, Y.-X. Xie and J.-H. Li, Chem. Commun., 2019, 55, 12805 RSC
.
-
(a) J. Boivin, E. Fouquet and S. Z. Zard, J. Am. Chem. Soc., 1991, 113, 1055 CrossRef CAS
;
(b) T. Nishimura, T. Yoshinaka, Y. Nishiguchi, Y. Maeda and S. Uemura, Org. Lett., 2005, 7, 2425 CrossRef CAS PubMed
;
(c) T. Nishimura and S. Uemura, J. Am. Chem. Soc., 2000, 122, 12049 CrossRef CAS
;
(d) H.-B. Yang and N. Selander, Chem. – Eur. J., 1779, 2017, 23 Search PubMed
;
(e) H.-B. Yang, S. R. Pathipati and N. Selander, ACS Catal., 2017, 7, 8441 CrossRef CAS
;
(f) X.-Y. Yu, J.-R. Chen, P.-Z. Wang, M.-N. Yang, D. Liang and W.-J. Xiao, Angew. Chem., Int. Ed., 2018, 57, 738 CrossRef CAS PubMed
;
(g) X.-Y. Yu, P.-Z. Wang, D.-M. Yan, B. Liu, J.-R. Chen and W.-J. Xiao, Adv. Synth. Catal., 2018, 360, 3601 CrossRef CAS
;
(h) B.-Q. He, X.-Y. Yu, P.-Z. Wang, J.-R. Chen and W.-J. Xiao, Chem. Commun., 2018, 54, 12262 RSC
;
(i) B. Lu, Y. Cheng, L.-Y. Chen, J.-R. Chen and W.-J. Xiao, ACS Catal., 2019, 9, 8159 CrossRef CAS
;
(j) X.-Y. Yu, Q.-Q. Zhao, J. Chen, J.-P. Chen and W.-J. Xiao, Angew. Chem., Int. Ed., 2018, 57, 15505 CrossRef CAS PubMed
.
-
(a) Y.-Q. Tang, J.-C. Yang, L. Wang, M.-J. Fan and L.-N. Guo, Org. Lett., 2019, 21, 5178 CrossRef CAS PubMed
;
(b) J. Wu, J.-Y. Zhang, P. Gao, S.-L. Xu and L.-N. Guo, J. Org. Chem., 2018, 83, 1046 CrossRef CAS
;
(c) J.-J. Zhang, X.-H. Duan, Y. Wu, J.-C. Yang and L.-N. Guo, Chem. Sci., 2019, 10, 161 RSC
;
(d) J. Lou, Y. He, Y.-L. Li and Z.-K. Yu, Adv. Synth. Catal., 2019, 361, 3787 CrossRef CAS
;
(e) Y. He, J. Lou, K.-K. Wu, H.-M. Wang and Z.-K. Yu, J. Org. Chem., 2019, 84, 2178 CrossRef CAS
;
(f) Z.-P. Yin, J. Rabeah, A. Brückner and X.-F. Wu, ACS Catal., 2018, 8, 10926 CrossRef CAS
;
(g) Z.-P. Yin, J. Rabeah, A. Brückner and X.-F. Wu, Org. Lett., 2019, 21, 1766 CrossRef CAS PubMed
;
(h) J.-X. Xu, F. Teng, J.-N. Xiang, W. Deng and J.-H. Li, Org. Lett., 2019, 21, 9434 CrossRef PubMed
.
-
(a) W. Zhang, Y.-L. Pan, C. Yang, L. Chen, X. Li and J.-P. Cheng, J. Org. Chem., 2019, 84, 7786 CrossRef CAS PubMed
;
(b) X. Shen, J.-J. Zhao and S.-Y. Yu, Org. Lett., 2018, 20, 5523 CrossRef CAS
;
(c) L. Tian, S.-Q. Guo, R. Wang, Y. Li, C.-L. Tang, L.-L. Shi and J.-K. Fu, Chem. Commun., 2019, 55, 5347 RSC
;
(d) M.-H. Xu, K.-L. Dai, Y.-Q. Tu, X.-M. Zhang, F.-M. Zhang and S.-H. Wang, Chem. Commun., 2018, 54, 7685 RSC
;
(e) J. Zhang, X.-F. Li, W.-L. Xie, S.-Q. Ye and J. Wu, Org. Lett., 2019, 21, 4950 CrossRef CAS PubMed
;
(f) M. Zheng, G.-G. Li and H.-J. Lu, Org. Lett., 2019, 21, 1216 CrossRef CAS PubMed
.
-
(a) Z.-Z. Zhu, K. Chen, L.-Z. Yu, X.-Y. Tang and M. Shi, Org. Lett., 2015, 17, 5994 CrossRef CAS PubMed
;
(b) M.-T. Chen, X.-Y. Tang and M. Shi, Org. Chem. Front., 2017, 4, 86 RSC
;
(c) Y. Liu, Q.-L. Wang, C.-S. Zhou, B.-Q. Xiong, P.-L. Zhang, C.-A. Yang and K.-W. Tang, J. Org. Chem., 2017, 82, 7394 CrossRef CAS PubMed
;
(d) Y. Liu, Q.-L. Wang, C.-S. Zhou, B.-Q. Xiong, P.-L. Zhang, C.-A. Yang and K.-W. Tang, J. Org. Chem., 2018, 83, 4657 CrossRef CAS PubMed
;
(e) Y. Liu, Z. Chen, Q.-L. Wang, C.-S. Zhou, B.-Q. Xiong, C.-A. Yang and K.-W. Tang, J. Org. Chem., 2019, 84, 9984 CrossRef CAS PubMed
;
(f) Y. Liu, Q. L. Wang, Z. Chen, Q. Zhou, H. Li, C.-S. Zhou, B.-Q. Xiong, P.-L. Zhang and K.-W. Tang, J. Org. Chem., 2019, 84, 2829 CrossRef CAS PubMed
;
(g) Y. Liu, Q. L. Wang, Z. Chen, Q. Zhou, C.-S. Zhou, B.-Q. Xiong, P.-L. Zhang, C.-A. Yang and K.-W. Tang, Adv. Synth. Catal., 2019, 361, 2315 CrossRef
;
(h) Y. Liu, Q. L. Wang, Z. Chen, Q. Zhou, C.-S. Zhou, B.-Q. Xiong, P.-L. Zhang, C.-A. Yang and K.-W. Tang, Org. Biomol. Chem., 2019, 17, 1365 RSC
.
-
(a) Y. Liu, Q. L. Wang, Z. Chen, Q. Zhou, B.-Q. Xiong, P.-L. Zhang and K.-W. Tang, Chem. Commun., 2019, 55, 12212 RSC
;
(b) B.-G. Cai, Z.-L. Chen, G.-Y. Xu, J. Xuan and W.-J. Xiao, Org. Lett., 2019, 21, 4234 CrossRef CAS PubMed
;
(c) Q. L. Wang, W.-Z. Zhang, Q. Zhou, C.-S. Zhou, B.-Q. Xiong, K.-W. Tang and Y. Liu, Org. Biomol. Chem., 2019, 17, 7918 RSC
;
(d) H.-H. Zhang, J.-J. Zhao and S.-Y. Yu, J. Am. Chem. Soc., 2018, 140, 16914 CrossRef CAS PubMed
;
(e) J.-J. Zhao, H.-H. Zhang, X. Shen and S.-Y. Yu, Org. Lett., 2019, 21, 913 CrossRef CAS PubMed
;
(f) Q.-Q. Zhao, M. Li, X.-S. Xue, J.-R. Chen and W.-J. Xiao, Org. Lett., 2019, 21, 3861 CrossRef CAS PubMed
;
(g) X. Wang, Y.-F. Han, X.-H. Ouyang, R.-J. Song and J.-H. Li, Chem. Commun., 2019, 55, 14637 RSC
;
(h) Y. Liu, Q. L. Wang, C.-S. Zhou, B.-Q. Xiong, P.-L. Zhang, C.-A. Yang and K.-W. Tang, J. Org. Chem., 2018, 53, 2210 CrossRef PubMed
.
Footnote |
† Electronic supplementary information (ESI) available. See DOI: 10.1039/c9cc10057a |
|
This journal is © The Royal Society of Chemistry 2020 |
Click here to see how this site uses Cookies. View our privacy policy here.