DOI:
10.1039/C9BM01474H
(Paper)
Biomater. Sci., 2020,
8, 2289-2299
Hydrophilic titanium surfaces reduce neutrophil inflammatory response and NETosis†
Received
12th September 2019
, Accepted 8th March 2020
First published on 9th March 2020
Abstract
Biomaterial implantation triggers an immune response initially predominated by neutrophils, which activate an inflammatory cascade by producing cytokines, enzymes, immune cell recruitment chemokines, and DNA fiber networks called neutrophil extracellular traps (NETs). While the role of neutrophils has been studied extensively in infection, little is known of their role in the response to biomaterials, in this case titanium (Ti) implants. Furthermore, while implant surface modifications have been shown to attenuate pro-inflammatory polarization in other immune cells, their effects on neutrophil behavior is unknown. The aim of this study was to characterize the neutrophil response to Ti surface topography and hydrophilicity and understand how the products of biomaterial-induced neutrophil activation alters macrophage polarization. Murine neutrophils were isolated by density gradient centrifugation and plated on smooth, rough, and rough hydrophilic (rough-hydro) Ti surfaces. Neutrophils on rough-hydro Ti decreased pro-inflammatory cytokine and enzyme production as well as decreased NET formation compared to neutrophils on smooth and rough Ti. Conditioned media (CM) from neutrophils on smooth Ti enhanced pro-inflammatory macrophage polarization compared to CM from neutrophils on rough or rough-hydro Ti; pretreatment of neutrophils with a pharmacological NETosis inhibitor impaired this macrophage stimulation. Finally, co-culture of neutrophils and macrophages on Ti surfaces induced pro-inflammatory macrophage polarization compared to macrophages alone on surfaces, but this effect was ablated when neutrophils were pretreated with the NETosis inhibitor. These findings demonstrate that neutrophils are sensitive to changes in biomaterial surface properties and exhibit differential activation in response to Ti surface cues. Additionally, inhibition of NETosis enhanced anti-inflammatory macrophage polarization, suggesting NETosis as a possible therapeutic target for enhancing implant integration.
1. Introduction
Implantation of orthopedic biomaterials induces an initial immune response predominated by innate immune cells of myeloid origin.1,2 This early pro-inflammatory phase occurs as a result of cues created by osseous injury from implantation as well as from potential introduction of pathogens to a previously sterile tissue within the body.3 From here, the fate of an implant—either failure or successful integration—is decided by the microenvironment that immune cells create at the implantation site.4 Secreted signaling molecules like chemokines and cytokines not only regulate immune cell chemotaxis and activation but also stem and progenitor chemotaxis and behavior.5–7 Thus, the cells of the immune system play broad roles in determining the success of bone-dwelling implants.
Macrophages, one member of the innate immune cell class, play a critical role in osseous tissue regeneration both in general bone healing and implant integration. During the early phases of tissue injury, pro-inflammatory macrophages generate TNF-α, IL-1β, IL-6, and IL-12, which propagate inflammation by recruiting other immune cell types.8 Later, macrophages predominate the transition from inflammation to healing, adopting an anti-inflammatory phenotype (i.e. production of TGF-β, IL-10, and matrix remodeling proteins).9 In the context of implants, our group has demonstrated that orthopedic and dental implant surface modifications intrinsically promote an anti-inflammatory macrophage phenotype, suggesting macrophage phenotype is regulated by both physical and chemical cues at the injury site.10,11 In addition to regulating the immune response, macrophages also regulate the recruitment and differentiation of stem cells during healing. We previously demonstrated that ablating macrophages decreases the recruitment of inflammatory and mesenchymal stem cells (MSCs) to the implant site in bone.12 Macrophage activity therefore appears to be a critical regulatory component in the immune response to implants; however, macrophages are not the “first responders” to tissue injury.
Despite their predominance immediately following tissue injury13 and their roles in recruitment of macrophages,14 neutrophils are understudied players in tissue healing and in response to biomaterial implantation. Arriving in scores at injury sites during the minutes to hours following trauma, neutrophils exert anti-microbial activity via phagocytosis, degranulation, enzymatic release, and the production of large DNA-based fiber networks called neutrophil extracellular traps (NETs).13,15,16 Neutrophil activation is often triggered by pathogen-associated molecular patterns (PAMPs) binding to toll-like receptor-4 (TLR-4) during non-sterile inflammation.17 These events have been long understood to serve antimicrobial purposes: propagate the immune response, limit pathogen activity, and enhance clearance of bacteria. However, recent studies have shown a role for neutrophils—namely, NETosis—in sterile inflammation, which may be triggered by release of danger-associated molecular patterns (DAMPs).18 In a murine model of atherosclerosis, NETs were found to prime macrophageal release of pro-inflammatory cytokines via activation of Th-17 cells.19 Similarly, in the liver, NETosis exacerbated the inflammatory response to experimental ischemia/reperfusion injury.20 Another study found that inhibition of NETosis through pharmacological inhibition of peptidyl arginine deaminase 4 (PAD4) reduced the degree of renal injury following ischemia and reperfusion.21 Paradoxically, in other models of sterile inflammation, NETosis appears to serve an anti-inflammatory role. Aggregation of NETs in crystal-related pathologies (e.g. monosodium urate in gout) exert immunosuppressive effects by degradation of inflammatory cytokines and chemokines.22 These studies reveal that NETs serve complex roles in localized regulation and progression of the inflammatory response.
In the context of orthopedic implants, macrophages are known to serve a critical, multifaceted role in integration,9,12,23 but it is not known if this role is redundant with that of other cell types, such as neutrophils. Additionally, little is known about neutrophils’ interactions with titanium (Ti)-based materials or how surface cues alter their behavior. Here, we seek to characterize the behavior of primary murine neutrophils on modified Ti surfaces and explore the effects of neutrophilic products—cytokines and NETs—on macrophage behavior in vitro.
2. Experimental
2.1. Ti disks
Smooth, rough, and rough-hydrophilic (rough-hydro) 15 mm Ti disks were provided by Institut Straumann AG (Basel, Switzerland) and generated as previously described.10 Smooth surfaces were prepared from 1 mm thick grade 2 unalloyed Ti (Sa = 0.61 μm; Θ = 93.8°). Rough surfaces were produced by sandblasting and acid-etching (Sa = 3.22 μm; Θ = 129.8°); rough-hydrophilic surfaces were produced in a similar fashion but in a nitrogen environment, retaining surface wettability (Sa = 3.22 μm; Θ = 0°).
2.2. Neutrophil isolation
10-Week-old male C57Bl/6 mice (Jackson Laboratory, Bar Harbor, ME) were used for this study in accordance with a protocol approved by the Virginia Commonwealth University Institutional Animal Care and Use Committee (Protocol: AD10001108). Mice were euthanized by CO2 asphyxiation followed by cervical dislocation. Femurs were removed and whole bone marrow was isolated by flushing the intramedullary cavity with phosphate-buffered saline (PBS, Thermo Fisher Scientific, Waltham, MA). Erythrocytes were removed from the marrow isolate using ACK Lysing Buffer (Quality Biological Inc., Gaithersburg, MD). Neutrophils were then isolated from the remaining cells by centrifugation using Histopaque 1077 and 1119 (Sigma-Aldrich, St Louis, MO).24 Viability on isolation was measured by Trypan Blue (Sigma-Aldrich) staining and was found to be >95% for all cultures. Purity was confirmed by flow cytometry. Prior to staining, isolated cells were treated with anti-CD16/32 (Fc receptor) antibody (BioLegend, San Diego, CA) to prevent non-specific fluorescence. Next, neutrophils were identified as CD11b+/Ly6G+ using CD11b-FITC and Ly6G-PE fluorophore-conjugated antibodies (BioLegend). A purity of approximately 80% was obtained for each experiment (Fig. S1†).
2.3. Macrophage culture
Whole bone marrow was isolated and treated with lysis buffer as described above. The remaining cells were counted and plated in a 175cm2 flask at a density of 500
000 cells per mL in Dulbecco's Modified Eagle Media (DMEM) (Thermo Fisher Scientific) supplemented with 10% fetal bovine serum (Thermo Fisher Scientific), 50 U mL−1 penicillin + 50 μg mL−1 streptomycin (Thermo Fisher Scientific), and 30 ng mL−1 macrophage colony-stimulating factor (M-CSF, BioLegend). Cells were then cultured for 7 d at 37 °C, 5% CO2, and 100% humidity. Supplemented media was refreshed on day 4 of culture. On day 7, macrophages were detached with Accutase (Innovative Cell Technologies, San Diego, CA) at 37 °C, monitoring for detachment using light microscopy, followed by scraping, and seeded onto tissue culture polystyrene (TCPS) or Ti surfaces in 24-well plates for experiments.
2.4. Protein analysis
To evaluate neutrophil inflammatory cytokine and chemokine production, neutrophils were plated on TCPS or smooth, rough, or rough-hydro Ti surfaces at a density of 200
000 cells per cm2 (n = 6 per variable). After 8 hours, conditioned media (CM) was harvested, and cells were lysed in 0.05% Triton X-100. Secretion of pro-inflammatory (IL-1β, TNF-α, IL-17, IL-6, IL-12, CXCL-10), anti-inflammatory (IL-4, IL-10), and enzymatic (neutrophil elastase, myeloperoxidase [MPO]) proteins were measured in CM by enzyme-linked immunosorbent assay (ELISA, BioLegend [IL-1β, TNF-α, IL-17, IL-6, IL-12, CXCL-10, IL-4, and IL-10], and R&D systems [Neutrophil elastase, MPO], Minneapolis, MN). Protein levels were normalized to DNA content measured in cell lysate (Quant-iT™ PicoGreen dsDNA Assay, ThermoFisher).
2.5. Neutrophil morphology on surfaces
Neutrophils were seeded on surfaces at a density of 10
000 cells per surface (n = 6 per variable). Neutrophils cultured on glass with LPS served as positive NETosis controls, while untreated neutrophils served as negative controls. After 4 or 8 hours, neutrophils were fixed, and DNA was stained with 4′,6-diamidino-2-phenylindole (DAPI, ThermoFisher Scientific) and DAPI-stained nucleic acids were visualized using confocal microscopy (Zeiss, LSM 710 Laser Scanning Microscope, Oberkochen, Germany). NETosis was characterized by measuring the area and circularity of stained DNA in 60 cells per sample using ImageJ. For scanning electron microscopy (SEM), implant surfaces with adherent neutrophils were fixed with 4% paraformaldehyde and dehydrated in ethanol before being sputter coated with 40
:
60 gold palladium and observed using a scanning electron microscope (S-4000, Hitachi, Tokyo, Japan) under 5k× magnification.
2.6. Gene expression
Neutrophils were plated on TCPS, smooth, rough, or rough-hydro surfaces at a density of 200
000 cells per cm2 (n = 6 per variable). After 3 hours of culture, neutrophils were lysed in TriZol (Thermo Fisher). mRNA was isolated, and 1 μg was converted to cDNA using iScript cDNA synthesis kit (BioRad, Hercules, CA). Using PrimePCR™ primers (Biorad) and SsoAdvanced Universal SYBR green supermix (Biorad), quantitative RT-PCR (qPCR) was performed to assess expression of genes associated with macrophage recruitment (Ccl2, Ccl3, Ccl4, Ccl5, Ccl17, Ccl20). Differences were determined by 2−ΔΔCt method.
2.7. Macrophage response to neutrophil-conditioned media (CM)
Neutrophils were cultured at a density of 200
000 cells per cm2 for 8 hours on TCPS or modified Ti surfaces in the presence of 50 nM GSK484 (Cayman Chemical, Ann Arbor, MI), an inhibitor of PAD4 and consequently NETosis, or an equivalent concentration of ethanol vehicle25 (n = 6 per variable). Then, CM was collected and spun at 2000g for 30 m to remove cellular debris and transferred to macrophages cultured on TCPS at a density of 10
000 cells per cm2. After 24 hours of culture, macrophage samples were detached into single-cell suspensions using Accutase (Life Technologies) at 37 °C for 5–12 min, followed by centrifugation at 300g and resuspension in 1% bovine serum albumin in PBS (staining buffer) prior to immunostaining for flow cytometry.
2.8. Macrophage response to surface cues
Macrophages were cultured alone on TCPS or modified Ti surfaces in the presence of 50 nM GSK484 or ethanol vehicle for 24 hours at a density of 10
000 cells per cm2. Then, cells were detached into single-cell suspension as described in the previous section prior to immunostaining.
2.9. Macrophage-neutrophil co-culture
Neutrophils were cultured at a density of 200
000 cells per cm2 for 8 hours on TCPS or modified Ti surfaces in the presence of 50 nM GSK484 or ethanol vehicle. Then, macrophages cultured in TCPS flasks were added directly to the neutrophils on Ti surfaces at a density of 10
000 cells per cm2, and the cells were co-cultured for 24 hours. Finally, cells were detached into single-cell suspension as previously described before immunostaining.
2.10. Flow cytometry
Polarization of macrophages was characterized using flow cytometry. Prior to fluorescent staining, Fc receptors were blocked by incubation with anti-CD16/32 (BioLegend) to prevent non-specific binding of subsequent antibodies. Then, cells were incubated with anti-CD68-FITC (BioLegend), a pan-macrophage marker that excludes neutrophils, which do not express high surface CD68; anti-CD80-PE (BioLegend), a pro-inflammatory surface marker; and anti-CD206-APC (BioLegend), an anti-inflammatory surface marker. Pro-inflammatory macrophages were identified as CD68+/CD80+, while anti-inflammatory macrophages were identified as CD68+/CD206+. Antibody concentrations were added based on the manufacturer's recommendations. Stained cell suspensions were analyzed using a Guava ® easyCyte 6-2L Benchtop Flow Cytometer (MilliporeSigma) instrument collecting 10
000 events per sample (n = 6 per group). Results were analyzed using FlowJo software (FlowJo LLC, Ashland, OR). A schematic showing gating strategy is included in Fig. S2.†
2.11. Transwell co-culture
To determine the effect of neutrophil secreted factors on macrophage polarization, neutrophils were plated in 24-well plates at a density of 200
000 cells per well on smooth, rough, or rough-hydro Ti surfaces (n = 9 per surface) for 8 hours with 50 nM GSK484 or equivalent volume of ethanol vehicle. Additionally, macrophages were plated at 10
000 cells per cm2, on 1.0 μm transwell inserts and incubated for 24 hours. Then, neutrophils on Ti surfaces were transferred to 6-well plates (3 surfaces per well) and inserts containing macrophages were transferred to the 6 well plates and fresh medium was added (n = 6 per surface type). After 12 hours of co-culture, macrophage-laden inserts were transferred to new 6-well plates with fresh media and cultured for 12 hours. Conditioned media was then collected, and IL-1β, IL-6, IL-10, IL-12(p40), IL-12(p70), IL-18, CCL17, CCL22, CXCL1, and TGF-β1 were measured using a LEGENDplex™ multiplex ELISA kit (BioLegend). The assay was performed according to the manufacturer's instructions, and data were analyzed using software provided by the manufacturer.
2.12. Statistical analysis
Data are presented as mean ± SD. Gene expression is presented as fold-change (2−ΔΔCt) compared to Gapdh housekeeping gene (Δ1) and to untreated TCPS controls (Δ2). Protein production is normalized to DNA quantity in each sample. Statistical analysis was performed using Prism Graphpad V7 software. Single-factor, equal variance ANOVA was used to ensure samples within groups were not significantly different (α = 0.05). Once the resulting p-value within sample groups was found to be insignificant, multiple comparisons were made between groups using Tukey's HSD test. The presented data were obtained from one of two repeated experiments, with both experiments yielding comparable results.
3. Results
3.1. Biomaterial surface cues modulate neutrophil protein secretion
To understand if neutrophils differentially regulate the implant microenvironment in response to biomaterial surface cues, the secretion of several pro- and anti-inflammatory proteins were measured, as well as CXCL-10, a chemokine involved in neutrophil recruitment during inflammatory responses, and MCP-1, a macrophage recruitment chemokine. LPS-exposed neutrophils on TCPS served as a positive control for inflammatory activation. After 8 hours, culture on smooth or rough Ti surfaces increased neutrophil pro-inflammatory cytokine secretion (IL-1β, IL-6, IL-12, TNF-α, IL-17) the most (Fig. 1A). The highest levels of immune cell recruitment-associated chemokines (CXCL-10, MCP-1) were measured in media from cells on smooth or rough surfaces (Fig. 1B), while rough-hydro surfaces enhanced anti-inflammatory (IL-4, IL10) cytokine secretion (Fig. 1C). Following trends seen in cytokine activation, smooth and rough Ti surfaces enhanced secretion of the classical neutrophil activation markers neutrophil elastase and MPO (Fig. 2). These data suggest that, like macrophages, neutrophils modulate their inflammatory phenotype in response to smooth, rough, and rough-hydrophilic surface modifications.
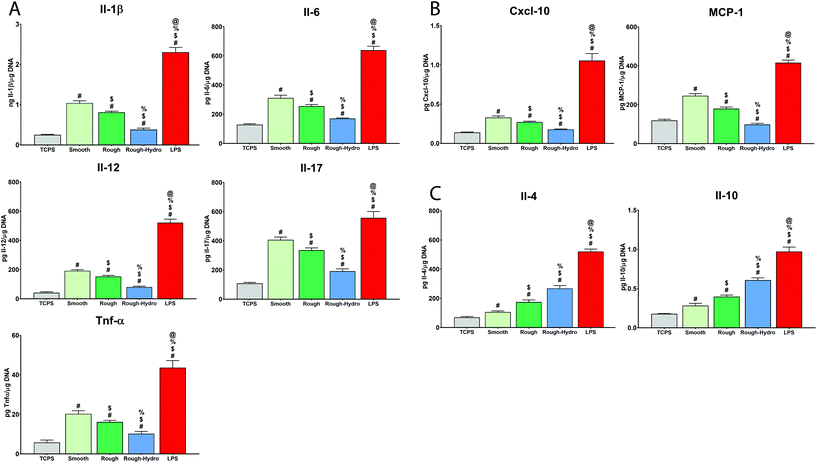 |
| Fig. 1 Neutrophil cytokine and chemokine secretion in response to surface modifications. Neutrophils were cultured for 8 hours on tissue culture polystyrene (TCPS) or Ti. Concentration of secreted (A) pro-inflammatory cytokines, (B) chemokines, and (C) anti-inflammatory cytokines was measured in conditioned media by enzyme-linked immunosorbent assay and normalized to DNA content in each sample (n = 6 per group). Lipopolysaccharide (LPS)-treated cells were used as a positive control. p < 0.05: # vs. TCPS, $ vs. smooth, % vs. rough, @ vs. rough-hydro. | |
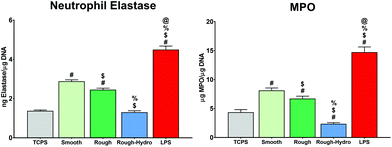 |
| Fig. 2 Neutrophil enzyme secretion in response to surface modifications. Neutrophils were cultured for 8 hours on tissue culture polystyrene (TCPS) or Ti. Concentration of secreted neutrophil elastase and myeloperoxidase (MPO) was measured by enzyme-linked immunosorbent assay and normalized to DNA content in each sample (n = 6 per group). Lipopolysaccharide (LPS)-treated cells were used as a positive control. p < 0.05: # vs. TCPS, $ vs. smooth, % vs. rough, @ vs. rough-hydro. | |
3.2. Neutrophils alter NETotic activity in response to biomaterial surface cues
To characterize the NETotic response to different surface stimuli, neutrophils were cultured on smooth, rough, or rough-hydro surfaces. By 4 hours post-plating, neutrophils on smooth surfaces showed large NETs formation. In contrast, neutrophils on rough surfaces appeared only to form small NETs at 8 hours and neutrophils on rough-hydro surfaces did not show signs of NETs formation over the time course examined (Fig. 3A). Next, distribution of DNA was quantified by shape analysis, where the wide, web-like spread of DAPI-stained DNA indicates NETosis has occurred. Confocal microscopy of DNA-stained neutrophils revealed enhanced NETosis on smooth Ti compared to the other Ti surfaces (Fig. 3B). Interestingly, DNA was even more compact in neutrophils on the rough-hydro surfaces than on glass. Similarly, SEM at 4 and 8 hours post-plating revealed similar neutrophil morphology on Ti surfaces (Fig. 4). These findings suggest that neutrophils are sensitive to Ti surface cues and adjust their NETotic activity accordingly.
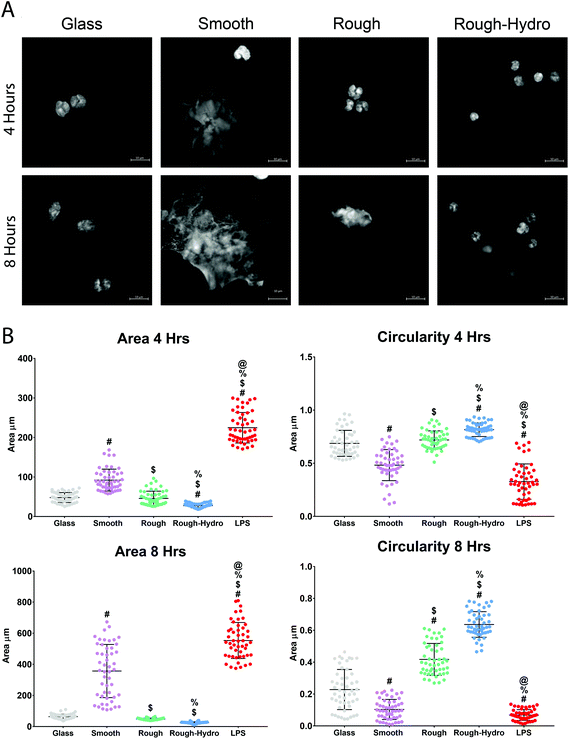 |
| Fig. 3 Differential formation of neutrophil extracellular traps (NETs) on smooth, rough, and rough-hydro surfaces. Neutrophils were seeded on surfaces at a density of 10 000 cm−2 for 4 or 8 hours prior to staining with DAPI for imaging. (A) Representative confocal microscopic images of nets on glass or Ti surfaces. (B) Characterization of net area and shape at 4 or 8 hours by quantification using ImageJ software (n = 60 per variable). Glass served as a negative control, while bacterial lipopolysaccharide (LPS), a potent activator of NETosis, served as a positive control. p < 0.05: # vs. glass, $ vs. smooth, % vs. rough, @ vs. rough-hydro. | |
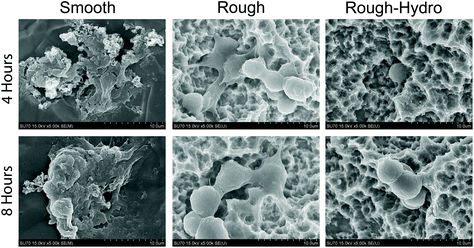 |
| Fig. 4 Neutrophil morphology on smooth, rough, and rough-hydro surfaces. neutrophils were seeded on surfaces at a density of 10 000 cm−2 for 4 (top row) or 8 hours (bottom row) prior to fixation and gold sputter-coating for SEM. | |
3.3. Surface roughness and hydrophilicity attenuate macrophage recruitment-related gene expression
Next, to explore the effect of surface modifications on immune cell recruitment, immune cell chemokine-encoding genes were evaluated at 3 hours following plating. Recruitment genes (Ccl2, Ccl3, Ccl4, Ccl5, Ccl17) were highly upregulated on smooth surfaces when compared to the modified Ti surfaces (Fig. 5). Interestingly, Ccl20 was downregulated relative to fresh neutrophils, but this chemokine motif is most strongly attractive to T-cells, suggesting that primarily innate immune recruitment chemokine genes are upregulated. These results suggest that surface properties regulate innate immune cell recruitment by neutrophils.
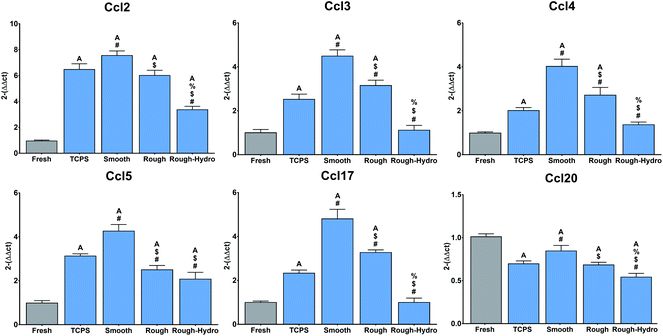 |
| Fig. 5 Regulation of macrophage recruitment-associated chemokines by neutrophils cultured on Ti surfaces. Neutrophils were cultured for 3 hours on Ti surfaces and mRNA for Ccl2, Ccl3, Ccl4, Ccl5, Ccl17, and Ccl20 measured by quantitative real time PCR. Fresh neutrophil mRNA was extracted immediately following neutrophil isolation and served as a negative control. Results are presented as fold-change (2−ΔΔCt). p < 0.05: a vs. fresh, # vs. TCPS, $ vs. smooth, % vs. rough. | |
3.4. Modified Ti surfaces decrease neutrophilic pro-inflammatory stimulation of macrophages
To evaluate the downstream consequences of surface-dependent activity on neutrophils, macrophages were exposed to CM from neutrophils treated with the PAD4 inhibitor GSK484. First, macrophages were cultured alone on Ti surfaces for 24 hours, treated with PAD4 inhibitor or ethanol vehicle. Ti surface modifications elicited changes in pro- and anti-inflammatory macrophage surface markers comparable to our previous work: smooth surfaces enhanced only pro-inflammatory markers, rough surfaces enhanced both pro- and anti-inflammatory surface markers, while rough-hydro surfaces decreased pro- and increased anti-inflammatory markers (Fig. 6, top row). Macrophages treated with the PAD4 inhibitor had a similar response to Ti surfaces as macrophages treated with vehicle only, verifying that the NETosis inhibitor did not have a direct effect on macrophages. Next, macrophages grown on TCPS were treated with CM from neutrophils cultured on Ti surfaces. CM from neutrophils on rough and rough-hydro surfaces enhanced anti-inflammatory macrophage polarization after 24 hours, as evidenced by the increase in CD206+ and decrease in CD80+ macrophages; PAD4 inhibition further enhanced the anti-inflammatory effect of CM (Fig. 6). These data suggest that neutrophils on modified Ti surfaces provide differential inflammatory stimuli through secreted molecules and that the magnitude of this effect is partially NET-dependent.
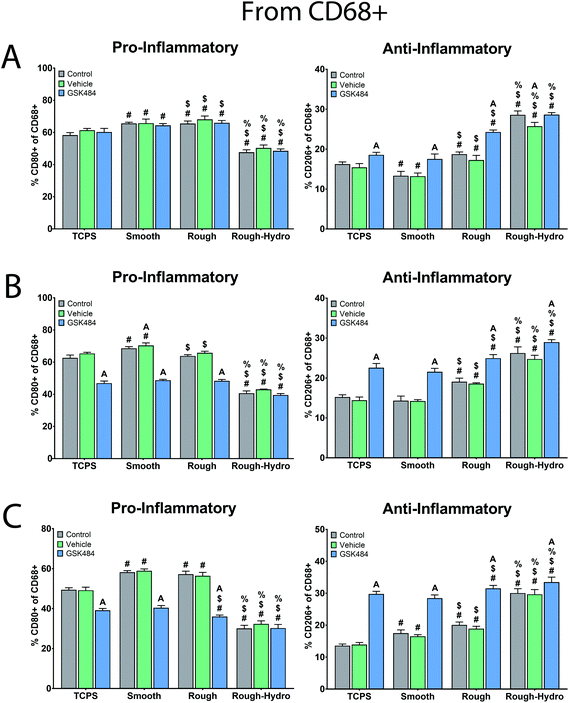 |
| Fig. 6 Effect of surface-dependent neutrophil activation on macrophage immunophenotype. Macrophages were categorized by immunostaining and flow cytometry. Pro-inflammatory macrophages were identified as CD68+/CD80+, while anti-inflammatory macrophages were identified as CD68+/CD206+. Data are presented as percentages of CD68+ cells (macrophage only). (A) Macrophages were directly plated on TCPS or Ti surfaces in the presence of GSK484 or vehicle (ethanol) and cultured for 24 hours. (B) Macrophages plated on TCPS were treated with cm from neutrophils plated on Ti surfaces with or without GSK484. (C) Neutrophils were cultured on Ti surfaces for 8 hours in the presence of GSK484 or vehicle, followed by the direct addition of macrophages to the culture. After 24 hours, macrophages were phenotyped. p < 0.05: # vs. TCPS, $ vs. smooth, % vs. rough, a vs. control. | |
Next, we examined the effect of direct neutrophil co-culture on macrophage polarization. Flow cytometric analysis of macrophages revealed increased pro-inflammatory polarization on smooth surfaces, enhanced pro- and anti-inflammatory polarization on rough, and highly anti-inflammatory polarization on rough-hydro surfaces (Fig. 6). However, pre-treatment of neutrophils with PAD4 inhibitor decreased pro-inflammatory markers and increased anti-inflammatory markers in macrophages regardless of surface modifications. To further support these findings, a transwell co-culture system was used. Analysis of macrophage CM from this system revealed enhanced pro-inflammatory cytokine and chemokine production (IL-1β, IL-6, IL-12p40 and -p70, IL-18, CCL22, CXCL1) on smooth and rough Ti, but higher anti-inflammatory and pro-resolution cytokines and chemokines (IL-10, CCL17, TGF-β1) on rough and rough-hydro Ti surfaces (Fig. 7). Consistent with previous findings, prior neutrophil inhibition with GSK484 appeared to negate the pro-inflammatory effect of neutrophils on macrophages when cultured on smooth or rough Ti. These findings indicate that surface-activated neutrophil behavior can differentially affect macrophage polarization, an effect heavily dependent on NETosis.
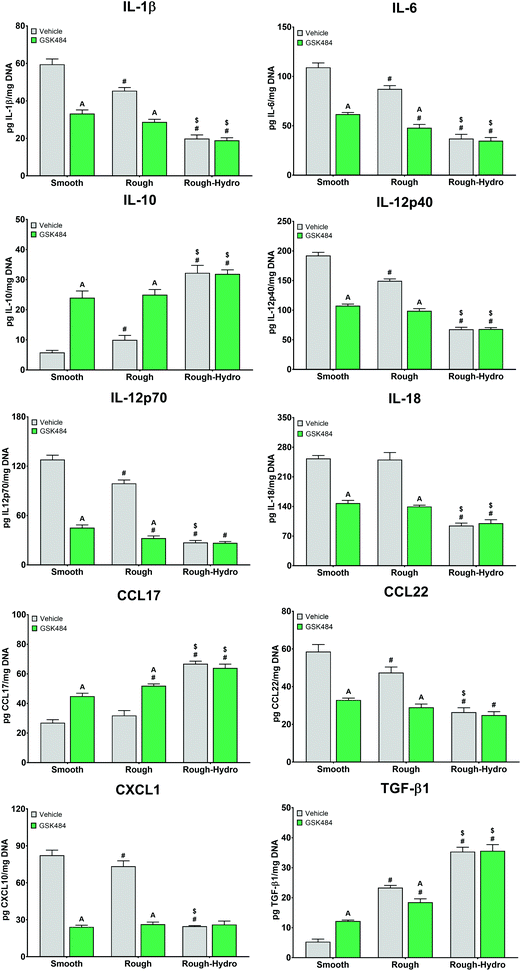 |
| Fig. 7 Effect of co-culture with neutrophils on macrophage protein secretion. Neutrophils were plated on smooth, rough, or rough-hydro Ti surfaces in the presence of GSK484 or ethanol vehicle for 8 hours. Then, macrophages in 1 μm transwell inserts were exposed to neutrophils for 12 hours, followed by 12 hours of culture in fresh media. Macrophage protein secretion was measured by ELISA. p < 0.05: # vs. smooth, $ vs. rough, a vs. respective vehicle. | |
4. Discussion
Neutrophils have long been recognized as critical early players in the physiologic response to biomaterial implantation, but surprisingly little is known about the cues modulating their behavior. Furthermore, while cytokine secretion has been used exclusively as the reporter for this characterizing neutrophil interaction with biomaterials, NETosis has yet to be explored thoroughly in this context. Here, we demonstrate that neutrophils differentially activate in response to smooth, rough, or rough-hydro Ti surfaces by secretion of inflammatory molecules, enzymes, and formation of NETs. Additionally, we show that neutrophil activation is important to macrophage polarization, underscoring the importance of neutrophil activation to healing overall.
This study is among the first to describe the effect of biomaterial physical cues on neutrophil behavior during sterile inflammation. Neutrophils have traditionally been studied in infectious inflammation, and the features distinguishing this cell type from other innate immune cells—namely, macrophages and dendritic cells—are based in this context: neutrophils are not antigen-presenting cells and generally do not survive past the early inflammatory phase.26,27 Macrophages are recognized as the primary drivers of granulation tissue formation and recruitment of stem cells during the proliferative phase of healing.28 In contrast, neutrophils have been thought only to serve to destroy infectious organisms through phagocytosis, enzymatic attack, ROS production, and amplification of the early pro-inflammatory response through cytokine and chemokine production.14,15,26,29 NETosis was initially thought to be merely another facet of this antimicrobial role, as NETs are released in response to bacterial-dependent Toll-like Receptor (TLR) or immunoglobulin-dependent Fc receptor activation.17,30,31 However, NETs have since been shown to form under various sterile inflammatory conditions, including autoimmune disease,32 crystallopathy,22 and organ injury.20,21,33 In sterile inflammation, NETs enhance the pro-inflammatory response through the cytotoxicity of free histones,34 transformation of pro-IL-1β into active IL-1β by NET-based enzymes,35 and direct action as a DAMP signal for other immune cells.18,20 NETs also serve as attachment hubs for bactericidal enzymes such as MPO, leukocyte proteases, and LL-37, an enzyme that serves both chemotactic and antimicrobial functions.36 Interestingly, MPO activity can serve as an additional amplifying component of the inflammatory response; in the lung, genetic knockdown of MPO decreased neutrophil recruitment and subsequent cytokine and chemokine production by other immune cell types.37 In this context, our findings suggest that heightened neutrophil cytokine production, enzyme secretion, and NETosis on biomaterial surfaces may adversely affect the response of other cell types, underscoring the importance of expanding the field's characterization of materials beyond how they activate macrophages.
Our results showed that smooth Ti surfaces increased neutrophil expression of chemokines responsible for the chemotaxis of other inflammatory cells such as neutrophils, monocytes, macrophages and T cells. Importantly, our results showed that neutrophils are sensitive to biomaterial physical cues, in this case surface topography, and neutrophils cultured on rough Ti surfaces showed lower levels of pro-inflammatory cytokines, enzymes and chemokines, suggesting that physical and mechanical cues are important players in neutrophil activation when not in context of infections by microorganisms. Furthermore, our results showed that the combination of surface topography/hydrophilicity caused the lowest levels of pro-inflammatory mediators and the highest increases anti-inflammatory interleukins in comparison to smooth and rough surfaces.
Under the assumption that smoothness and hydrophobicity serve as pro-inflammatory cues to neutrophils as they do to other immune cell types, the findings presented in this study are heavily supported by data demonstrating the effects of neutrophil activation on inflammation in disease. Unfortunately, while the pro-inflammatory effect of smooth or hydrophobic relative to rough-hydrophilic titanium has been observed in numerous studies with macrophage or macrophage-like cell lines,9–12,38,39 the mechanism of this phenomenon has yet to be definitively identified. Interestingly, as NETs formed even in the in vitro culture of only neutrophils, NETosis appears to occur directly as a consequence of biomaterial surface characteristics. Identifying the mechanism of NET activation in response to biomaterial surface characteristics (e.g. Toll-like receptor activation), or whether suicidal or vital NETosis predominated in our model, is beyond the scope of this paper.
While a recent study identified a NETotic response to roughened Ti implant surfaces,40 our study demonstrates that the neutrophil response depends on the type of surface modification and that these cues alter neutrophil inflammatory response. We have previously demonstrated a similar effect in macrophages, which respond to roughness and hydrophilicity with an enhanced anti-inflammatory profile.10–12 Since then, others have shown that this anti-inflammatory macrophage phenotype is more capable of driving osteogenesis than naïve or pro-inflammatory macrophage phenotypes.41,42 Here, we see that, when exposed to rough-hydrophilic surfaces, neutrophils secrete fewer pro-inflammatory molecules and do not undergo NETosis. This effect may contribute positively to osseointegration by decreasing the pro-inflammatory polarization of macrophages in response to interactions with NETs. While neutrophils do not persist into the proliferative phase of osseointegration, it would nevertheless be valuable to explore neutrophil/NET-MSC interactions, as an anti-osteogenic effect on MSCs would underscore the importance of their clearance from the implant surface prior to healing. Interestingly, pharmacologic ablation of NETosis enhanced the anti-inflammatory effect of surface modifications during macrophage-neutrophil co-culture, suggesting that inhibition of NETosis may enhance healing and subsequent bone formation around titanium implants. Indeed, a number of studies have shown that NETosis adversely affects biological systems requiring tolerogenesis, instead greatly exacerbating inflammation. One study found that mice deficient in PAD4 experienced significantly lower loss of pregnancy compared to control mice in an antiangiogenic model of pregnancy loss; the effect was found to be mediated by lower inflammatory activation and thrombotic response.43 Perhaps more relevantly, in an experimental autoimmune arthritis model, Padi4−/− mice experienced decreased severity, with lower cytokine production and inflammatory gene expression in response to type 2 collagen immunization.44 Our results suggest that the inflammatory response to clinically-relevant Ti biomaterials is similarly modulated by neutrophil activity—and that inhibition of NETosis may serve to hasten the resolution of inflammation with implants.
5. Conclusions
In this study we demonstrated that modified Ti surfaces decrease pro-inflammatory neutrophil behavior as measured by decreased cytokine production, decreased enzyme production, and attenuated NETosis. Furthermore, conditioned media from neutrophils grown on modified Ti surfaces lead to anti-inflammatory macrophage polarization, an effect further enhanced by pre-treatment of neutrophils with a pharmacologic NETosis inhibitor. Finally, the anti-inflammatory macrophage response to surface modifications is preserved even during co-culture with neutrophils, and NETosis inhibition enhances this anti-inflammatory effect. These findings emphasize the importance of the neutrophil response to implantable materials during design as well as identifying NETosis as a possible therapeutic target for enhancing integration of implanted biomaterials.
Conflicts of interest
The authors declare no conflict of interest.
Acknowledgements
Ti surfaces were supplied by Institut Straumann AG, Basel, Switzerland. Funding for this project has been provided partially through the Innovation in Implant Sciences Award by the International Association for Dental Research/Academy of Osseointegration.
References
- J. M. Anderson, ASAIO Trans., 1988, 34, 101–107 CrossRef CAS PubMed.
- K. Donath, M. Laass and H. J. Günzl, Virchows Arch. A: Pathol. Anat. Histopathol., 1992, 420, 131–137 CrossRef CAS PubMed.
- S. N. Christo, K. R. Diener, A. Bachhuka, K. Vasilev and J. D. Hayball, BioMed Res. Int., 2015, 2015, 342304 CrossRef PubMed.
- N. J. Hallab, Spine, 2016, 41, S30–S31 CrossRef PubMed.
- E. Ferreira, R. M. Porter, N. Wehling, R. P. O'Sullivan, F. Liu, A. Boskey, D. M. Estok, M. B. Harris, M. S. Vrahas, C. H. Evans and J. W. Wells, J. Biol. Chem., 2013, 288, 29494–29505 CrossRef CAS PubMed.
- Z. X. Yang, G. X. Mao, J. Zhang, X. L. Wen, B. B. Jia, Y. Z. Bao, X. L. Lv, Y. Z. Wang and G. F. Wang, Adv. Clin. Exp. Med., 2017, 26, 201–206 CrossRef PubMed.
- S. L. Hyzy, R. Olivares-Navarrete, S. Ortman, B. D. Boyan and Z. Schwartz, Tissue Eng., Part A, 2017, 23, 1132–1141 CrossRef CAS PubMed.
- J. Kzhyshkowska, A. Gudima, V. Riabov, C. Dollinger, P. Lavalle and N. E. Vrana, J. Leukocyte Biol., 2015, 98, 953–962 CrossRef CAS PubMed.
- J. Wang, F. Meng, W. Song, J. Jin, Q. Ma, D. Fei, L. Fang, L. Chen, Q. Wang and Y. Zhang, Int. J. Nanomed., 2018, 13, 4029–4043 CrossRef CAS PubMed.
- K. M. Hotchkiss, G. B. Reddy, S. L. Hyzy, Z. Schwartz, B. D. Boyan and R. Olivares-Navarrete, Acta Biomater., 2016, 31, 425–434 CrossRef CAS PubMed.
- K. M. Hotchkiss, N. B. Ayad, S. L. Hyzy, B. D. Boyan and R. Olivares-Navarrete, Clin. Oral Implants Res., 2017, 28, 414–423 CrossRef PubMed.
- K. M. Hotchkiss, N. M. Clark and R. Olivares-Navarrete, Biomaterials, 2018, 182, 202–215 CrossRef CAS PubMed.
- J. Wang, Cell Tissue Res., 2018, 371, 531–539 CrossRef CAS PubMed.
- T. A. Butterfield, T. M. Best and M. A. Merrick, J. Athl. Train., 2006, 41, 457–465 Search PubMed.
- G. S. Selders, A. E. Fetz, M. Z. Radic and G. L. Bowlin, Regener. Biomater., 2017, 4, 55–68 CrossRef CAS PubMed.
- L. Lauková and B. Konečná, J. Appl. Biomed., 2018, 16, 1–9 CrossRef.
- A. B. Al-Khafaji, S. Tohme, H. O. Yazdani, D. Miller, H. Huang and A. Tsung, Mol. Med., 2016, 22, 621–631 CAS.
- T. Iba, M. Murai, I. Nagaoka and Y. Tabe, Acute Med. Surg., 2014, 1, 2–9 CrossRef PubMed.
- A. Warnatsch, M. Ioannou, Q. Wang and V. Papayannopoulos, Science, 2015, 349, 316–320 CrossRef CAS PubMed.
- H. Huang, S. Tohme, A. B. Al-Khafaji, S. Tai, P. Loughran, L. Chen, S. Wang, J. Kim, T. Billiar, Y. Wang and A. Tsung, Hepatology, 2015, 62, 600–614 CrossRef CAS PubMed.
- W. M. Raup-Konsavage, Y. Wang, W. W. Wang, D. Feliers, H. Ruan and W. B. Reeves, Kidney Int., 2018, 93, 365–374 CrossRef CAS PubMed.
- Y. Li, X. Cao, Y. Liu, Y. Zhao and M. Herrmann, Front. Immunol., 2018, 9, 1559 CrossRef PubMed.
- K. A. Alexander, M. K. Chang, E. R. Maylin, T. Kohler, R. Müller, A. C. Wu, N. Van Rooijen, M. J. Sweet, D. A. Hume, L. J. Raggatt and A. R. Pettit, J. Bone Miner. Res., 2011, 26, 1517–1532 CrossRef CAS PubMed.
- M. Swamydas and M. S. Lionakis, J. Visualized Exp., 2013, e50586 Search PubMed.
- Y. Zhou, L.-L. An, R. Chaerkady, N. Mittereder, L. Clarke, T. S. Cohen, B. Chen, S. Hess, G. P. Sims and T. Mustelin, Sci. Rep., 2018, 8, 15228 CrossRef PubMed.
- H. L. Malech, F. R. DeLeo and M. T. Quinn, Methods Mol. Biol., 2020, 2087, 3–10 CrossRef PubMed.
- S. I. Simon and M.-H. Kim, Blood, 2010, 116, 511–512 CrossRef CAS PubMed.
- P. Krzyszczyk, R. Schloss, A. Palmer and F. Berthiaume, Front. Physiol., 2018, 9, 419 CrossRef PubMed.
- V. Delgado-Rizo, M. A. Martínez-Guzmán, L. Iñiguez-Gutierrez, A. García-Orozco, A. Alvarado-Navarro and M. Fafutis-Morris, Front. Immunol., 2017, 8, 81 Search PubMed.
- E. Pieterse, N. Rother, C. Yanginlar, L. B. Hilbrands and J. van der Vlag, Front. Immunol., 2016, 7, 484 Search PubMed.
- E. Aleyd, M. W. M. van Hout, S. H. Ganzevles, K. A. Hoeben, V. Everts, J. E. Bakema and M. van Egmond, J. Immunol., 2014, 192, 2374–2383 CrossRef CAS PubMed.
- J.-M. Berthelot, B. Le Goff, A. Neel, Y. Maugars and M. Hamidou, Jt., Bone, Spine, 2017, 84, 255–262 CrossRef PubMed.
- E. Lefrançais, B. Mallavia, H. Zhuo, C. S. Calfee and M. R. Looney, JCI Insight, 2018, 3, e98178 CrossRef PubMed.
- R. Allam, S. V. R. Kumar, M. N. Darisipudi and H.-J. Anders, J. Mol. Med., 2014, 92, 465–472 CrossRef CAS PubMed.
- D. M. Clancy, C. M. Henry, G. P. Sullivan and S. J. Martin, FEBS J., 2017, 284, 1712–1725 CrossRef CAS PubMed.
- M. J. Kaplan and M. Radic, J. Immunol., 2012, 189, 2689–2695 CrossRef CAS PubMed.
- A. Haegens, P. Heeringa, R. J. van Suylen, C. Steele, Y. Aratani, R. J. J. O'Donoghue, S. E. Mutsaers, B. T. Mossman, E. F. M. Wouters and J. H. J. Vernooy, J. Immunol., 2009, 182, 7990–7996 CrossRef CAS PubMed.
- M. A. Alfarsi, S. M. Hamlet and S. Ivanovski, J. Biomed. Mater. Res., Part A, 2014, 102, 60–67 CrossRef PubMed.
- M. A. Alfarsi, S. M. Hamlet and S. Ivanovski, Clin. Implant Dent. Relat. Res., 2015, 17, 1036–1047 CrossRef PubMed.
- L. Vitkov, W.-D. Krautgartner, A. Obermayer, W. Stoiber, M. Hannig, M. Klappacher and D. Hartl, PLoS One, 2015, 10, e0121359 CrossRef PubMed.
- Y. Zhang, T. Böse, R. E. Unger, J. A. Jansen, C. J. Kirkpatrick and J. J. J. P. van den Beucken, Cell Tissue Res., 2017, 369, 273–286 CrossRef CAS PubMed.
- C. L. Yang, Y. H. Sun, W. H. Yu, X. Z. Yin, J. Weng and B. Feng, Eur. Cells Mater., 2018, 36, 15–29 CrossRef CAS PubMed.
- L. Erpenbeck, C. S. Chowdhury, Z. K. Zsengellér, M. Gallant, S. D. Burke, S. Cifuni, S. Hahn, D. D. Wagner and S. A. Karumanchi, Biol. Reprod., 2016, 95, 132 CrossRef PubMed.
- A. Suzuki, Y. Kochi, H. Shoda, Y. Seri, K. Fujio, T. Sawada, R. Yamada and K. Yamamoto, BMC Musculoskeletal Disord., 2016, 17, 205 CrossRef PubMed.
Footnote |
† Electronic supplementary information (ESI) available. See DOI: 10.1039/c9bm01474h |
|
This journal is © The Royal Society of Chemistry 2020 |