DOI:
10.1039/D0AY00337A
(Paper)
Anal. Methods, 2020,
12, 2244-2252
Ion chromatography for monitoring [NTf2]− anion contaminants in pure and saline water†
Received
16th February 2020
, Accepted 12th April 2020
First published on 13th April 2020
Abstract
Hydrophobic ionic liquids containing bis(trifluoromethanesulfonyl)imide, [NTf2]−, anions partially dissolve in aqueous phases. The potential ecotoxicity of [NTf2]− means wastewater streams must be closely monitored to avoid environmental release. A new ion chromatography method is presented, which improves on existing techniques and methods by significantly decreasing analysis time and improving chromatographic peak properties. Consequently, the limit of detection is lowered to 5 μM (1.4 ppm) and limit of quantification lowered to 30 μM (8.5 ppm). The method is reproducible and has a high precision for short and medium chain length ionic liquids (RSD = 0.95%); however, microemulsion effects increase measurement errors for long chain ionic liquids (RSD = 2.32%), albeit by a relatively small amount. Hence, the method is a highly accurate analytical method for highly polarizable [NTf2]− anions, and quantification in the presence of high salinity samples, such as seawater, is readily achieved. Importantly, this method is a significant improvement on existing techniques (chromatography, NMR, UV-Vis) for many reasons, making it ideal for environmental monitoring or process design of [NTf2]− ionic liquid-based applications.
The ionic liquid research community continually seeks new applications for ionic liquids because their unusual physicochemical properties can improve existing applications in a variety of ways.1 A significant number of studies have been published showing how ionic liquids provide a truly unique opportunity and new areas are flourishing as a result. Selected examples include ionic liquids for energy storage devices,2 as solvents for lignocellulosic biomass pretreatment,3 to improve biocatalytic processes,4 or to enhance analytical separations.5 Often, applications require hydrophobic or non-coordinating ionic liquids which are prepared by ion metathesis with anions such as hexafluorophosphate ([PF6]−) or bis(trifluoromethanesulfonyl)imide ([NTf2]−).6 Unfortunately, [PF6]− anions are thermally and hydrolytically unstable and release HF as a decomposition by-product.7,8 Although alternatives are available, [NTf2]− ionic liquids are now the most popular option and their hydrophobic properties have led to a variety of aqueous biphasic applications.9–13
Despite [NTf2]− ionic liquids forming secondary phases with water, some studies have identified considerable solubilities in aqueous phases.14–19 Dissolution of [NTf2]− in water introduces a new waste stream that is rarely considered in ionic liquid process design. This problem is exacerbated by the potential (eco)toxicity of [NTf2]− anions, making wastewater monitoring a critical requirement for any industrial application. Ionic liquid toxicity is related to lipid membrane accumulation,20 chemical structure,21 and hydrophobicity,22 all of which are linked to the solubility of ionic liquids in water.23 Combined with the extremely low biodegradability of [NTf2]− containing ionic liquids,24,25 discharge of saturated wastewater into the environment could cause an ecotoxicological disaster. Ionic liquids have been compared to polychlorinated biphenyls (PCBs),26 a persistent organic pollutant (POP), because of their similar physical and chemical properties. In the UK, the drinking water inspectorate (DWI) suggests treating all organofluorine compounds as perfluorooctanesulfonic acid (PFOS) or perfluorooctanoic acid (PFOA), because of a lack of toxicological and environmental information.27 Fluorochemicals are causing increasing environmental and health concerns, leading to stringent regulations and lower emission guidelines.28 The UK Environment Agency requires waste with more than 50 mg kg−1 (50 ppm) PFOS (and similar POP derivatives) to be treated or permanently stored.29
Bacterial degradation of [NTf2]− IL wastewater is difficult because of the low biodegradability of the anion.24 Tertiary treatment options are required to remove [NTf2]− from wastewater; however, to date there is very little research in this area. Biodegradation of ionic liquids can be improved by incorporating functional groups.30 Unfortunately, functional ionic liquids often exhibit higher water solubilities and hydrophobic [NTf2]− ionic liquids can even become completely water-miscible, given enough H-bonding functionalities.31 This function-solubility relationship limits ionic liquid design by restricting the functional groups of task-specific ionic liquids. Regardless, any process requiring [NTf2]− containing ionic liquids will generate dilute wastewater through either preparation, application, or recycling of the ionic liquid phase wherever water is needed. Hence, a robust technique is necessary to analyse [NTf2]− contaminated wastewater during process development or for environmental monitoring purposes.
Ion chromatography (IC) is an essential technique for monitoring ionic trace analytes such as iodide, perchlorate, or bromate.32–36 Often, high ionic strength samples (such as drinking water or seawater) are analysed to measure low concentration analytes; however, concentrated matrices can lead to column overloading and subsequent peak distortion of the target analyte.37 This phenomenon can occur under well resolved analytical conditions and can affect peak quantification.35 Competitive Langmuir isotherm type behavior can explain the effects of eluents and analyte overloading in ion chromatography. However, it is difficult to predict how overloading can impact trace analyte peaks. Therefore, experimentally measuring the effects of eluent composition and overloaded analyte profiles can provide valuable information for method development.
The partial solubilities of hydrophobic ionic liquids in water have previously been measured by UV-vis,19,38,39 cloud point,15 total organic carbon (TOC),40 quantitative NMR spectroscopy,41 and conductivity.39 However, all techniques are limited in either ion detection (i.e. NMR requires NMR active nuclei, UV-vis requires absorbing ions), by high detection limits, problems with sample matrices (i.e. pH affects extinction coefficients for UV-vis), or an inability to differentiate anions and cations. (i.e. electrochemical, TOC, cloud point). This latter point is particularly problematic for many techniques, including common types of chromatography (i.e. HPLC).
IC has been used to analyse trace halide impurities in ionic liquid samples; however, the technique is not suited to hydrophobic anions such as [NTf2]− and chromatograms suffer from poor retention times and significant tailing.42,43 For example, even moderately polarisable anions such as [BF4]− have long retention times and non-ideal peak shapes.44 Cation IC has been used to quantify common ionic liquid cations such as imidazolium,45 pyridinium,46 and pyrrolidinium ions.47 Strong anion exchange and cation exchange resins have even been used in tandem for simultaneous separation of anions and cation.48 An alternative to IC is ion pair chromatography (IPC) which uses an ion pair reagent (IPR) to separate ions that cannot be separated by other type of chromatography.49 Ionic liquid cations can be separated and quantified by reverse phase ICP,50,51 and polarisable anions such as [OTf]−, [OTs]−, [BF4]−, and [PF6]− can be eluted in <15 min and quantified with IPRs such as [TBA][OH].52,53
Here we use a new IC method for analysing [NTf2]− in aqueous samples with a detection limit < 5 μM (1.4 ppm) in the presence of high concentrations of inorganic salts. Our method is highly accurate and capable of quantifying highly polarisable ions, unlike many other methods reported to date. In addition, our method is ideal for industrial wastewater analysis or environmental monitoring of medium-to-high salinity brine (i.e. industrial effluent or seawater) because of the high resolution between Cl− and [NTf2]−.
Experimental section
NaOH (50% w/w) and methanesulfonic acid (99%), MSA, were purchased from Fisher Scientific. Synthetic seawater (SSWS-500) was purchased from Paragon Scientific Ltd. Lithium bis(trifluoromethanesulfonyl)imide, Li[NTf2], 1,6-dibromohexane and 1,12-dibromododecane were purchased from Sigma Aldrich and used as received. 1-Methylimidazole, N-chlorobutane, and N-chlorooctane were purchased from Sigma Aldrich and distilled under reduced pressure before use. Anhydrous acetonitrile and ethyl acetate were purchased from VWR international and handled via air-free techniques. 1-Butyl-3-methylimidazolium bis(trifluoromethylsulfonyl)amide, [C4C1Im][NTf2],54 1-octyl-3-methylimidazolium bis(trifluoromethylsulfonyl)amide, [C8C1Im][NTf2],54 1,6-bis(3-methylimidazolium)hexane di[bis(trifluoromethanesulfonyl)amide], [C6(C1Im)2][NTf2],55 1,12-bis(3-methylimidazolium)dodecane di[bis(trifluoromethanesulfonyl)amide], [C12(C1Im)2][NTf2],55 tetraoctylphosphonium bis(trifluoromethylsulfonyl)amid, [P66614][NTf2],56 trihexyltetradecylphosphonium bis(trifluoromethylsulfonyl)amide, [P66614][NTf2],56 were synthesised according to existing literature procedures. All non-aqueous chemicals were stored, weighed and handled in an MBraun LABstar glovebox with <0.5 ppm H2O and <0.5 ppm O2. 1-Ethyl-3-methylimidazolium bis(trifluoromethylsulfonyl)amide (IL-02965G-0025), [C2C1Im][NTf2]; 1-dodecyl-3-methylimidazolium bis(trifluoromethylsulfonyl)amide (IL-0101-HP-0025), [C12C1Im][NTf2]; 1-butyl-1-methylpyrrolidinium bis(trifluoromethylsulfonyl)amide (IL-0035-HP-0025), [C4C1Pyrr][NTf2]; diethyl(methyl)sulfonium bis(trifluoromethylsulfonyl)amide (IL-0031-HP-0025), [S221][NTf2]; tributylmethylammonium bis(trifluoromethylsulfonyl)amide (IL-0117-HP-0025), [N4441][NTf2]; butyltriethylammonium bis(trifluoromethylsulfonyl)amide (IL-0329-HP-0025), [N4222][NTf2]; octyltriethylammonium bis(trifluoromethylsulfonyl)amide (IL-0328-HP-0025), [N8222][NTf2] were purchased from IOLITEC and used as received. Propyltrimethylammonium bis(trifluoromethylsulfonyl)amide (AM0308B), [N3111][NTf2], and hexyltrimethylammonium bis(trifluoromethylsulfonyl)amide (AM0608B1), [N6111][NTf2], were purchased from Solvionic and used as received.
Seawater ionic liquid solubility measurements were performed by combining 1.0 mL of ionic liquid with 5.0 mL of SSWS-500 in a plastic falcon tube which was agitated in a vortex mixer in a temperature-regulated laboratory at 23 ± 2 °C for 60 min. After settling, the top portion of the aqueous phase was separated, centrifuged at 14
800 rpm for 1 h, and the process was repeated to ensure all ionic liquid phase was removed. Samples were diluted to ensure 0.01–1 mM of [NTf2]− was injected into the ion chromatograph for optimum peak shape and low error; dilution factors were dependant on the ionic liquid and ranged from 10 to 25. The synthetic seawater contained NaCl (24.54 g L−1), MgCl2·6H2O (11.10 g L−1), Na2SO4 (4.09 g L−1), CaCl2·2H2O (1.54 g L−1), KCl (0.69 g L−1), NaHCO3 (0.20 g L−1), KBr (0.10 g L−1), H3BO3 (0.03 g L−1), SrCl2·6H2O (0.04 g L−1), NaF (0.003 g L−1).
Anion Chromatography (AC) was performed on a Thermofisher ICS5000 + equipped with a Dionex IonPac™ AS20 (4 × 250 mm) analytical column and AG20 (4 × 50 mm) guard column. Eluents were 18.2 MΩ cm Milli-Q® ultrapure water, HiPerSolv CHROMANORM® acetonitrile (≥99.9%), and 200 mM NaOH (from 50% w/w NaOH), which were proportioned as specified (e.g. 30% acetonitrile and 20 mM NaOH) and eluted isocratically at 1 mL min−1. All aqueous eluents were sparged with helium for 30 min before use and subsequently kept under a helium blanket to avoid CO2 ingress. An AERS500e 4 mm electrochemical analytical suppressor was used to suppress the NaOH eluent, and the suppressor was regenerated from an external supply of 18.2 MΩ cm Milli-Q® ultrapure water pumped at 1 mL min−1. Analytes were detected with a temperature-controlled conductivity detector held at 18 °C. The column and guard were housed in a temperature-controlled compartment set to 45 °C and the suppressor and conductivity detector were housed in a separate compartment set to 15 °C.
For method development, [NTf2]− standards were made by weighing anhydrous Li[NTf2] in a glovebox on an analytical balance, moving to air and dissolving in 18.2 MΩ cm Milli-Q® ultrapure water, followed by serial dilution. Samples were kept in a temperature-controlled autosampler at 23 °C, and a 10 μL injection loop was used. The Dionex IonPac™ AS20 (310 μeq per column) is a hydroxide selective column which was designed for trace analysis of perchlorate in drinking water.57,58 Resolution of the peaks was calculated using eqn (S1) in the ESI.† Asymmetry factor (As) was calculated as As = b/a, where a and b are the front and back peak half-widths at 10% peak height. Signal-to-noise (S/N) was calculated as the ratio of [NTf2]− signal height to the noise measured over a 1 minute region before [NTf2]− elution.
Two calibration curves were used for variable concentration data, one from 0.001–0.1 mM, another from 1–100 mM and R2 values of 0.999 were obtained for each. The limit of detection (LOD) was determined as S/N < 10 or as 3σi (where σi is the y-intercept value of the low concentration calibration plot). The limit of quantification (LOQ) was determined as 10σi. The ion exchange selectivity coefficient (KEluent, Analyte) for monovalent eluents and analytes was calculated from:
| 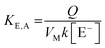 | (1) |
where
Q is column capacity in milliequivalents,
VM is the dead volume of the AS20 column (mL), [E
−] is the eluent concentration in molarity, and
k is the retention factor (eqn (S2)
†).
Results and discussion
Method development
IC is primarily a water-based chromatographic technique, and the AS20 column has ultralow hydrophobicity because the exchange resin contains quaternary ammonium alkanol functional groups. The [OH]− eluent, therefore, has a strong affinity for the stationary phase, and NaOH is thus a stronger eluent than for comparable alkylammonium based resins. Hydrophobic [NTf2]− anions have a low affinity for the mobile phase but a high affinity for the stationary ion exchange resin because of the high polarizability of the [NTf2]− anion. Consequently, high concentrations of eluent and organic solvent are required to elute [NTf2]− analytes with reasonable peak properties. In this work, eluent strength and organic solvent composition were limited by the capacity and lifetime of the suppressor. Eluent suppression has been shown to dramatically lowers background conductivity and thus reduce noise, subsequently improving detection limits. The chromatographic method for measuring [NTf2]− aqueous samples was therefore developed by balancing equipment limitations against retention times, resolution, peak asymmetry, and error (Fig. 1).
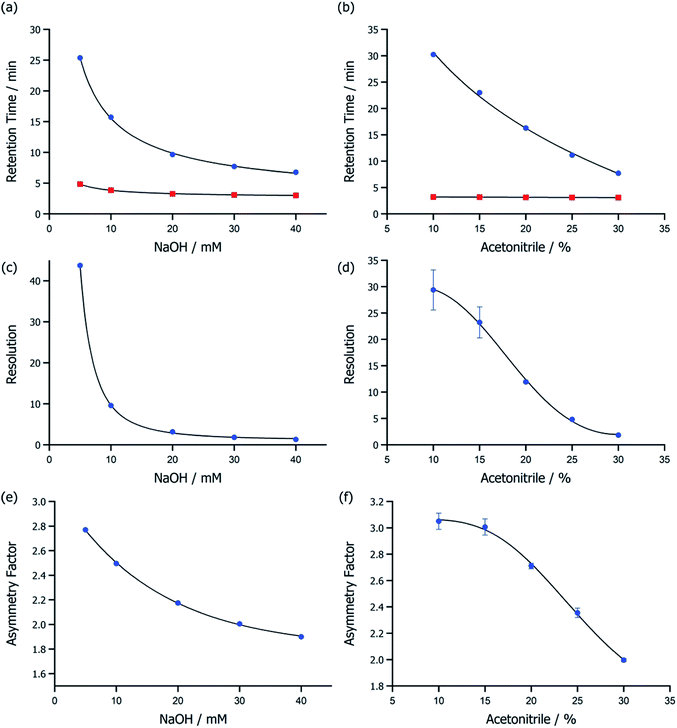 |
| Fig. 1 The retention time of 1 mM [NTf2]− (blue) and 1 mM Cl− (red) as a function of (a) NaOH total eluent strength for 30% MeCN at 1 mL min−1 and (b) acetonitrile for 30 mM NaOH at 1 mL min−1 with the calculated resolution between the components (c and d) and asymmetry factor of [NTf2]− at 10% peak height (e and f) for each NaOH and acetonitrile composition. | |
Understanding peak properties in different eluent compositions is key to finding the optimum conditions for analysis of new anionic components. Retention times of Cl− and [NTf2]− were measured for a variety of eluent strengths and acetonitrile compositions up to the tolerance of the analytical suppressor. For the 4 mm AERS500e suppressor used here, the upper limits were 40 mM NaOH (suppressed with the highest recommended current of 99 mA) and 30% MeCN. For [NTf2]−, retention times were dramatically lowered at higher eluent strengths and higher organic solvent compositions (Fig. 1a and b; ESI,†) but Cl− was relatively unaffected and rapidly eluted within 5 min at all composition. Higher NaOH and MeCN caused a significant reduction in resolution (Fig. 1c and d; ESI,†), yet peaks remained fully resolved for all compositions (resolution > 2). For NaOH ≥ 20 mM and MeCN compositions ≥ 30%, retention time was reduced to less than 10 min which was a significant improvement in analysis time and thus dramatically lowered eluent waste. Furthermore, the As of [NTf2]− was found to decrease to 2 (Fig. 1e and f, ESI,†) at the upper limit of 40 mM NaOH and 30% MeCN, which was a significant improvement on previous literature reports. Unfortunately, a reduction in eluent strength or MeCN composition caused an increase in [NTf2]− peak As, even for 1 mM of the analyte.
A solvent composition of 20 mM NaOH and 30% MeCN was selected for subsequent experiments based on method development data. The chosen composition gave an [NTf2]− retention time of 9.63 min and an As of 2.18, with 3.12 resolution from Cl−. This resolution was high enough to quantify [NTf2]− in 1000 mM NaCl matrices. The effects of increasing Cl− concentration (0–1000 mM) on Cl− and [NTf2]− resolution for different concentrations of [NTf2]− analytes were measured (Fig. S1; ESI Table S7†) for the chosen eluent composition. Importantly, quantification of a wide range of [NTf2]− concentrations (0.1–100 mM) was possible in the presence of high concentrations of NaCl, despite the conductivity detector becoming saturated with Cl− above 250 mM and [NTf2]− overloading (Fig. 2a). However, peaks shapes for <0.1 mM [NTf2]− had more Gaussian characteristic with less tailing. Overloading [NTf2]− reduced the retention time (ESI, Fig. S2†) from 9.93 min to 7.18 min (0.001 to 100 mM) which was a smaller shift compared to other high-affinity analytes with comparatively weak eluents.37
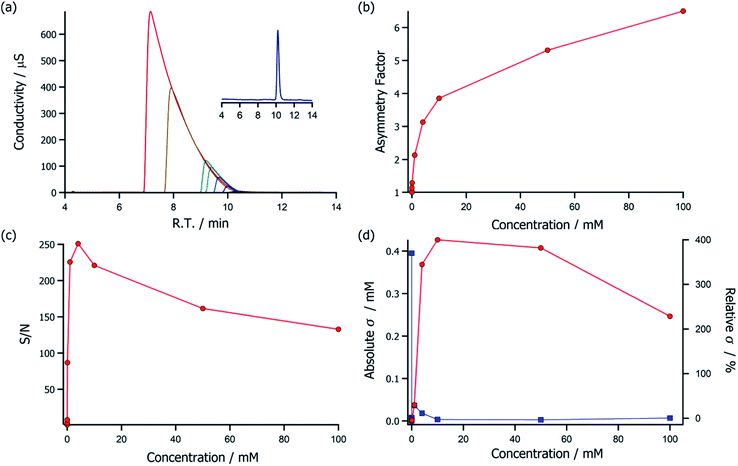 |
| Fig. 2 Concentration dependent data for 0.001 mM to 100 mM injections of [NTf2]− for 20 mM NaOH and 30% MeCN, showing (a) chromatograms overlay (increasing concentration from small to large) with inset window for 0.01 mM (b) asymmetry factor, (c) S/N, and (d) absolute (red) and relative (blue) standard deviation. | |
For example, the As of 0.1 mM analyte was 1.29, but 4 mM of analyte had a significantly higher As of 3.13. The S/N ratio was highest for 1–10 mM, and below 0.1 mM the S/N rapidly decreased. Likewise, above 10 mM the S/N decreased due to column overloading and tailing of the [NTf2]− analyte. The absolute standard deviation (σ) of [NTf2]− quantification was highest for 10 mM, which had an error of 0.43 mM (120.5 ppm). Below 1 mM [NTf2]− the absolute error was below 0.04 mM (10.6 ppm); however, the relative error increased with reduced concentration analyte. The [NTf2]− LOD was determined to be between 1–5 μM (0.3–1.4 ppm) by S/N or 18 μM (5.0 ppm) by 3σi, and the LOQ was determined to be 30 μM (8.5 ppm) from 10σi. The LOD could theoretically be further reduced by increasing the injection volume; however, the properties of [NTf2]− are ideal for use with concentrator columns which could decrease the detection limit by 2–5 orders of magnitude.36
Synthetic seawater analysis
For the purpose of this work, we chose to demonstrate the analytical method by analysing ionic liquid-saturated synthetic seawater samples with 20 different hydrophobic [NTf2]− ionic liquids (Table 1). The retention time of [NTf2]− in pure water was 9.63 ± 0.01 min and the retention time in seawater was 10.07 ± 0.17 min, showing that overloaded Cl− had a minimal retainment effect on the trace [NTf2]− analyte. Peak shape was also unaffected, indicating that IC is ideal for analysing trace [NTf2]− in concentrated brine solutions. The hydrophobicity of ionic liquid cations appeared to have a significant impact on seawater solubility, with values ranging from 66.20 mM for [C2C1Im][NTf2] to 0.22 mM for [P8888][NTf2]. Extending the alkyl-chain length of imidazolium ionic liquids significantly reduced their seawater solubility (Fig. 3a), and even a single additional carbon (i.e. C2 to C3) could reduce the solubility of [CnC1Im][NTf2] by 51.70 mM (14
471 ppm). A similar relationship was found for ammonium ionic liquids (Fig. 3b) where additional carbons could decrease the seawater solubility, although more substantial increases were needed to decrease solubility compared to imidazolium ionic liquids.
Table 1 Concentrations and dissolution volumes for [NTf2]− ionic liquids in synthetic seawater
|
Concentration (mM) |
Error (mM) |
Concentration (ppm) |
Dissolution volume (mL g−1) |
[C2C1Im][NTf2] |
66.20 |
0.66 |
18 083.0 |
38.6 |
[S221][NTf2] |
33.00 |
0.18 |
9014.4 |
78.6 |
[N1113][NTf2] |
21.71 |
0.20 |
5930.8 |
120.6 |
[C3C1Im][NTf2] |
14.50 |
0.37 |
3960.7 |
170.3 |
[N4111][NTf2] |
12.92 |
0.06 |
3529.1 |
195.4 |
[C4Py][NTf2] |
10.02 |
0.01 |
2736.3 |
240.0 |
[C4C1Im][NTf2] |
8.13 |
0.04 |
2219.7 |
293.7 |
[C4C1Pyrr] |
7.41 |
0.20 |
2024.2 |
319.7 |
[NTf2] |
[C6(C1Im)2] |
5.79 |
0.06 |
1581.5 |
427.5 |
[NTf2]2 |
[N4222][NTf2] |
4.90 |
0.04 |
1339.0 |
465.6 |
[N6111][NTf2] |
4.10 |
0.02 |
1120.5 |
574.8 |
[C6C1Im][NTf2] |
3.05 |
0.03 |
831.8 |
734.6 |
[N8222][NTf2] |
0.92 |
0.02 |
250.5 |
2206.9 |
[C8C1Im][NTf2] |
0.88 |
0.01 |
241.1 |
2384.9 |
[N4441][NTf2] |
0.86 |
0.02 |
233.7 |
2434.7 |
[C12(C1Im)2] |
0.60 |
0.01 |
164.0 |
3734.9 |
[NTf2]2 |
[N8881][NTf2] |
0.52 |
<0.01 |
142.0 |
2966.1 |
[P66614][NTf2] |
0.38 |
<0.01 |
104.3 |
3431.7 |
[C12C1Im][NTf2] |
0.37 |
0.01 |
102.1 |
5038.8 |
[P8888][NTf2] |
0.22 |
0.01 |
59.5 |
6014.2 |
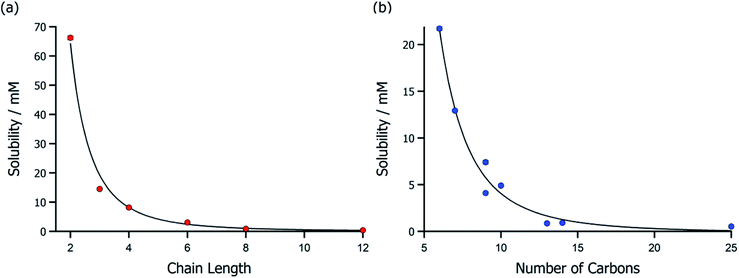 |
| Fig. 3 Ionic liquid synthetic seawater solubilities of (a) imidazolium ionic liquids with different alkyl chain lengths and (b) ammonium ionic liquids with varying numbers of carbon. | |
Different ionic head groups influenced ionic liquid solubility, albeit to a smaller extent than carbon chain lengths. For butyl-chain lengths, pyridinium > imidazolium > pyrrolidinium with only a 2.61 mM difference between the highest and lowest values. Dicationic ionic liquids had seawater solubilities lower than their symmetrical monocationic analogues (i.e. [Cn(C1Im)2][NTf2]2 and [Cn/2C1Im][NTf2]) which were 2.5 (n = 6) and 5.1 (n = 12) times lower. However, dicationic ionic liquids had higher solubilities than their equivalent length analogues (i.e. [C12(C1Im)2][NTf2]2vs. [C12C1Im][NTf2]). The lowest solubilities were measured for ionic liquids with the longest alkyl chains. For phosphonium ionic liquids symmetry affected seawater solubility; for example, [P66614][NTf2] had a solubility 0.16 mM higher than [P8888][NTf2], which has the same number of carbon atoms. This is most likely due to emulsion formation, although in this work samples were separated by two subsequent hour-long centrifuges.
Conclusions
A new anion chromatography method was developed to analyse aqueous phase concentrations of [NTf2]− by balancing peak properties and equipment limitations. Quantification in the presence of high salinity samples was possible because of the high resolution from Cl−, largely due to the high polarizability of the [NTf2]− anion. An optimised method (i.e. 20 mM NaOH and 30% MeCN) had a very low LOD (<5 μM, or 1.4 ppm) which was within 10% of the environmental quality standard (i.e. 50 ppm for fluorinated POPs), making IC a suitable analytical technique for environmental monitoring or wastewater analysis. The LOQ was determined to be slightly higher (30 μM, or 8.5 ppm), but still a significant improvement on commonly employed analytical techniques (chromatography, NMR, UV-Vis). The method was highly reproducible for short and medium chain length ionic liquids (±0.95%), but microemulsion effects of long chain ionic liquid skewed the precision by increasing error (±2.32%). Of course, this was a problem with sample preparation and microemulsions are an inherent property of long chain ionic liquids. Despite this, the method was highly accurate and was used to quantify [NTf2]− concentrations in synthetic seawater after saturation with 20 standards; hence, all process wastewater would require treatment before disposal. The lowest solubility was obtained for hydrophobic ionic liquids. The measured values showed that all ionic liquids had partial solubilities above the suggested environmental [P8888][NTf2] at 0.22 μM or 59.5 ppm. Importantly, new wastewater treatment methods will be necessary if [NTf2]− ionic liquids are to be used on an industrial scale. Other anions, such as hydrophobic fatty acids, could provide an alternative to the potentially ecotoxic [NTf2]− anion; however, this substitution will sacrifice other desirable physical properties such as thermal stability or non-coordinating ions.
We present an anion chromatography method for rapid analysis of highly polarisable [NTf2]− anions in wastewater streams with variable salinity. The ongoing development of IL-based research means that more chemical processes and technologies are being commercialised and scaled-up. Given the current lack of methods focused towards trace analysis of ecotoxic [NTf2]− anions, anion chromatographic methods such as this could become common place for wastewater analysis or environmental monitoring.
Author contributions
All experimental work, analysis, and writing was done by CJC and all authors proof-read the manuscript and have approved the final version.
Conflicts of interest
There are no conflicts to declare.
Acknowledgements
CJC would like to thank Dr Kevin Lovelock for supplying nine [NTf2]− ionic liquids and Thermo Scientific™ Dionex™ engineers at Hemel Hempstead for their help with IC. The authors thank the EPSRC (Frontier Engineering Grant EP/K038648/1) for financial support and John Televantos for sponsoring Liem Bui-Le's PhD.
References
- C. J. Clarke, W. C. Tu, O. Levers, A. Bröhl and J. P. Hallett, Green and Sustainable Solvents in Chemical Processes, Chem. Rev., 2018, 118(2), 747–800, DOI:10.1021/acs.chemrev.7b00571.
- M. Watanabe, M. L. Thomas, S. Zhang, K. Ueno, T. Yasuda and K. Dokko, Application of Ionic Liquids to Energy Storage and Conversion Materials and Devices, Chem. Rev., 2017, 7190–7239, DOI:10.1021/acs.chemrev.6b00504.
-
C. J. Clarke, W.-C. Tu, L. Weigand, A. Brandt and J. P. Hallett, Solvation Behavior of Ionic Liquids and Their Role in the Production of Lignocellulosic Biofuels and Sustainable Chemical Feedstocks, in Advanced Green Chemistry Part 1: Greener Organic Reactions and Processes, ed. I. T. Horváth and M. Malacria, World Scientific, Hong Kong, 2018, pp. 77–134. DOI:10.1142/9789813228115_0003.
- T. Itoh, Ionic Liquids as Tool to Improve Enzymatic Organic Synthesis, Chem. Rev., 2017, 10567–10607, DOI:10.1021/acs.chemrev.7b00158.
- M. D. Joshi and J. L. Anderson, Recent Advances of Ionic Liquids in Separation Science and Mass Spectrometry, RSC Adv., 2012, 2(13), 5470–5484, 10.1039/c2ra20142a.
- J. G. Huddleston, A. E. Visser, W. M. Reichert, H. D. Willauer, G. A. Broker and R. D. Rogers, Characterization and Comparison of Hydrophilic and Hydrophobic Room Temperature Ionic Liquids Incorporating the Imidazolium Cation, Green Chem., 2001, 3(4), 156–164, 10.1039/b103275p.
- C. Liu, Z. Yu, D. Neff, A. Zhamu and B. Z. Jang, Graphene-Based Supercapacitor with an Ultrahigh Energy Density, Nano Lett., 2010, 10(12), 4863–4868, DOI:10.1021/nl102661q.
- M. G. Freire, C. M. S. S. Neves, I. M. Marrucho, J. A. P. Coutinho and A. M. Fernandes, Hydrolysis of Tetrafluoroborate and Hexafluorophosphate Counter Ions in Imidazolium-Based Ionic Liquids, J. Phys. Chem. A, 2010, 114(11), 3744–3749, DOI:10.1021/jp903292n.
- H. Lai, Z. Wang and P. Wu, Structural Evolution in a Biphasic System: Poly(N-Isopropylacrylamide) Transfer from Water to Hydrophobic Ionic Liquid, RSC Adv., 2012, 2(31), 11850–11857, 10.1039/c2ra21288a.
- S. Boudesocque, A. Mohamadou and L. Dupont, Efficient Extraction of Gold from Water by Liquid–Liquid Extraction or Precipitation Using Hydrophobic Ionic Liquids, New J. Chem., 2014, 38(11), 5573–5581, 10.1039/c4nj01115e.
- Y. Wang, W. Leng, Y. Gao and J. Guo, Thermo-Sensitive Polymer-Grafted Carbon Nanotubes with Temperature-Controlled Phase Transfer Behavior between Water and a Hydrophobic Ionic Liquid, ACS Appl. Mater. Interfaces, 2014, 6(6), 4143–4148, DOI:10.1021/am405796c.
- E. Alvarez-Guerra and A. Irabien, Extraction of Lactoferrin with Hydrophobic Ionic Liquids, Sep. Purif. Technol., 2012, 98, 432–440, DOI:10.1016/j.seppur.2012.08.010.
- L. I. N. Tomé, V. R. Catambas, A. R. R. Teles, M. G. Freire, I. M. Marrucho and J. A. P. Coutinho, Tryptophan Extraction Using Hydrophobic Ionic Liquids, Sep. Purif. Technol., 2010, 72(2), 167–173, DOI:10.1016/j.seppur.2010.02.002.
- N. V. Shvedene, S. V. Borovskaya, V. V. Sviridov, E. R. Ismailova and I. V. Pletnev, Measuring the Solubilities of Ionic Liquids in Water Using Ion-Selective Electrodes, Anal. Bioanal. Chem., 2005, 381(2), 427–430, DOI:10.1007/s00216-004-3001-7.
- Y. Li, L. S. Wang and S. F. Cai, Mutual Solubility of Alkyl Imidazolium Hexafluorophosphate Ionic Liquids and Water, J. Chem. Eng. Data, 2010, 55(11), 5289–5293, DOI:10.1021/je1003059.
- L. I. N. Tomé, F. R. Varanda, M. G. Freire, I. M. Marrucho and J. A. P. Coutinho, Towards an Understanding of the Mutual Solubilities of Water and Hydrophobic Ionic Liquids in the Presence of Salts: The Anion Effect, J. Phys. Chem. B, 2009, 113(9), 2815–2825, DOI:10.1021/jp810141d.
- M. G. Freire, P. J. Carvalho, A. M. S. Silva, L. M. N. B. F. Santos, L. P. N. Rebelo, I. M. Marrucho and J. A. P. Coutinho, Ion Specific Effects on the Mutual Solubilities of Water and Hydrophobic Ionic Liquids, J. Phys. Chem. B, 2009, 113(1), 202–211, DOI:10.1021/jp8080035.
- M. G. Freire, P. J. Carvalho, R. L. Gardas, I. M. Marrucho, L. M. N. B. F. Santos and J. A. P. Coutinho, Mutual Solubilities of Water and the [Cnmim][Tf2N] Hydrophobic Ionic Liquids, J. Phys. Chem. B, 2008, 112(6), 1604–1610, DOI:10.1021/jp7097203.
- M. G. Freire, C. M. S. S. Neves, P. J. Carvalho, R. L. Gardas, A. M. Fernandes, I. M. Marrucho, L. M. N. B. F. Santos and J. A. P. Coutinho, Mutual Solubilities of Water and Hydrophobic Ionic Liquids, J. Phys. Chem. B, 2007, 111(45), 13082–13089, DOI:10.1021/jp076271e.
- R. J. Cornmell, C. L. Winder, G. J. T. Tiddy, R. Goodacre and G. Stephens, Accumulation of Ionic Liquids in Escherichia coli Cells, Green Chem., 2008, 10(8), 836–841, 10.1039/b807214k.
- S. Viboud, N. Papaiconomou, A. Cortesi, G. Chatel, M. Draye and D. Fontvieille, Correlating the Structure and Composition of Ionic Liquids with Their Toxicity on Vibrio fischeri: A Systematic Study, J. Hazard. Mater., 2012, 215–216, 40–48, DOI:10.1016/j.jhazmat.2012.02.019.
- A. G. Santos, B. D. Ribeiro, D. S. Alviano and M. A. Z. Coelho, Toxicity of Ionic Liquids toward Microorganisms Interesting to the Food Industry, RSC Adv., 2014, 4(70), 37157–37163, 10.1039/C4RA05295A.
- K. A. Kurnia, T. E. Sintra, C. M. S. S. Neves, K. Shimizu, J. N. Canongia Lopes, F. Gonçalves, S. P. M. Ventura, M. G. Freire, L. M. N. B. F. Santos and J. A. P. Coutinho, The Effect of the Cation Alkyl Chain Branching on Mutual Solubilities with Water and Toxicities, Phys. Chem. Chem. Phys., 2014, 16(37), 19952–19963, 10.1039/c4cp02309a.
-
E. Diaz, V. Monsalvo, J. Palomar and A. F. Mohedano, Ionic Liquids: Bacterial Degradation in Wastewater Treatment Plants, in Encyclopedia of Inorganic and Bioinorganic Chemistry, John Wiley & Sons, Ltd, Chichester, UK, 2016, pp. 1–12. DOI:10.1002/9781119951438.eibc2393.
- K. S. Egorova, E. G. Gordeev and V. P. Ananikov, Biological Activity of Ionic Liquids and Their Application in Pharmaceutics and Medicine, Chem. Rev., 2017, 7132–7189, DOI:10.1021/acs.chemrev.6b00562.
- G. Chatel, E. Naffrechoux and M. Draye, Avoid the PCB Mistakes: A More Sustainable Future for Ionic Liquids, J. Hazard. Mater., 2017, 324, 773–780, DOI:10.1016/J.JHAZMAT.2016.11.060.
-
A. Clare, B. Simon, H. Tom, K. Rakesh and R. Paul, Survey of the Prevalence of Perfluorooctane Sulphonate (PFOS), Perfluorooctanoic Acid (PFOA) and Related Compounds in Drinking Water and Their Sourceshttp://www.dwi.gov.uk/stakeholders/information-letters/2009/10_2009annex.pdf, accessed Aug 12, 2019 Search PubMed.
- X. Z. Lim, Tainted Water: The Scientists Tracing Thousands of Fluorinated Chemicals in Our Environment, Nature, 2019, 26–29, DOI:10.1038/d41586-019-00441-1.
-
Dispose of waste containing persistent organic pollutants (POPs) – GOV.
UK
https://www.gov.uk/guidance/dispose-of-waste-containing-persistent-organic-pollutants-pops#thresholds, accessed Aug 12, 2019 Search PubMed.
- N. Gathergood, M. T. Garcia and P. J. Scammells, Biodegradable Ionic Liquids: Part I. Concept, Preliminary Targets and Evaluation, Green Chem., 2004, 6(3), 166–175, 10.1039/b315270g.
- A. M. De Ferro, P. M. Reis, M. R. C. Soromenho, C. E. S. Bernardes, K. Shimizu, A. A. Freitas, J. M. S. S. Esperança, J. N. Canongia Lopes and L. P. N. Rebelo, Designing the Ammonium Cation to Achieve a Higher
Hydrophilicity of Bistriflimide-Based Ionic Liquids, Phys. Chem. Chem. Phys., 2018, 20(29), 19307–19313, 10.1039/c8cp03398f.
- J. Mathew, J. Gandhi and J. Hedrick, Trace Level Perchlorate Analysis by Ion Chromatography-Mass Spectrometry, J. Chromatogr. A, 2005, 1085(1), 54–59, DOI:10.1016/j.chroma.2005.05.015.
- P. E. Jackson, S. Gokhale, T. Streib, J. S. Rohrer and C. A. Pohl, Improved Method for the Determination of Trace Perchlorate in Ground and Drinking Waters by Ion Chromatography, J. Chromatogr. A, 2000, 888(1–2), 151–158, DOI:10.1016/S0021-9673(00)00557-4.
- E. Santoyo, S. Santoyo-Gutiérrez and S. P. Verma, Trace Analysis of Heavy Metals in Groundwater Samples by Ion Chromatography with Post-Column Reaction and Ultraviolet-Visible Detection, J. Chromatogr. A, 2000, 884(1–2), 229–241, DOI:10.1016/S0021-9673(00)00358-7.
- M. K. Pappoe, M. H. Naeeni and C. A. Lucy, Bromate Peak Distortion in Ion Chromatography in Samples Containing High Chloride Concentrations, J. Chromatogr. A, 2016, 1444, 57–63, DOI:10.1016/j.chroma.2016.03.045.
- K. Ito, Determination of Iodide in Seawater by Ion Chromatography, Anal. Chem., 1997, 69(17), 3628–3632, DOI:10.1021/ac9700787.
- M. F. Wahab, J. K. Anderson, M. Abdelrady and C. A. Lucy, Peak Distortion Effects in Analytical Ion Chromatography, Anal. Chem., 2014, 86(1), 559–566, DOI:10.1021/ac402624a.
- C. M. S. S. Neves, C. Held, S. Mohammad, M. Schleinitz, J. A. P. Coutinho and M. G. Freire, Effect of Salts on the Solubility of Ionic Liquids in Water: Experimental and Electrolyte Perturbed-Chain Statistical Associating Fluid Theory, Phys. Chem. Chem. Phys., 2015, 17(47), 32044–32052, 10.1039/C5CP06166K.
- K. A. Kurnia, T. E. Sintra, C. M. S. S. Neves, K. Shimizu, J. N. Canongia Lopes, F. Gonçalves, S. P. M. Ventura, M. G. Freire, L. M. N. B. F. Santos and J. A. P. Coutinho, The Effect of the Cation Alkyl Chain Branching on Mutual Solubilities with Water and Toxicities, Phys. Chem. Chem. Phys., 2014, 16(37), 19952–19963, 10.1039/c4cp02309a.
- R. Keyes and P. Scovazzo, Ammonium Ionic Liquid Solubilities in Water and Micellar Formation, J. Phys. Chem. B, 2017, 121(29), 7163–7172, DOI:10.1021/acs.jpcb.7b05109.
- V. Mazan, M. Y. Boltoeva, E. E. Tereshatov and C. M. Folden III, Mutual Solubility of Water and Hydrophobic Ionic Liquids in the Presence of Hydrochloric Acid, RSC Adv., 2016, 6(61), 56260–56270, 10.1039/C6RA06791C.
- C. Villagrán, M. Deetlefs, W. R. Pitner and C. Hardacre, Quantification of Halide in Ionic Liquids Using Ion Chromatography, Anal. Chem., 2004, 76(7), 2118–2123, DOI:10.1021/ac035157z.
- F. Hao, P. R. Haddad and T. Ruther, IC Determination of Halide Impurities in Ionic Liquids, Chromatographia, 2008, 67(5–6), 495–498, DOI:10.1365/s10337-008-0527-4.
- P. Kosobucki and B. Buszewski, Determination of Tetrafluoroborate and Chloride Anions by Capillary Isotachophoresis and Suppressed Ion Chromatography, Chem. Anal., 2008, 53(6), 895–903 CAS.
- W. Gao and H. Yu, Determination of Homologue Imidazolium Ionic Liquid Cations by Ion Chromatography Using a Carboxyl Acid Cation-Exchange Column with Direct Conductivity Detection, Anal. Lett., 2011, 44(5), 922–931, DOI:10.1080/00032711003790064.
- Q. Chen, H. Yu and J. Wang, Determination of Pyridinium Ionic Liquid Cations by Ion Chromatography with Direct Conductivity Detection, J. Liq. Chromatogr. Relat. Technol., 2012, 35(9), 1184–1193, DOI:10.1080/10826076.2011.619028.
- R. Q. Zhang, H. Yu and X. J. Sun, Separation and Determination of Pyrrolidinium Ionic Liquid Cations by Ion Chromatography with Direct Conductivity Detection, Chin. Chem. Lett., 2013, 24(6), 503–505, DOI:10.1016/j.cclet.2013.03.033.
- A. Markowska and P. Stepnowski, Simultaneous Determination of Ionic
Liquid Cations and Anions Using Ion Chromatography with Tandem Ion Exchange Columns: A Preliminary Assessment, Anal. Sci., 2008, 24, 1359–1361, DOI:10.2116/analsci.24.1359.
-
T. Cecchi, Ion Pairing Chromatography, Critical Reviews in Analytical Chemistry, Taylor & Francis Group, 2008, pp. 161–213. DOI:10.1080/10408340802038882.
- X. Jin, H. Yu and Y. Ma, Reversed-Phase Ion-Pair Chromatography of Hydroxyl Functionalized Imidazolium Ionic Liquid Cations and Its Application in Analysis of Environmental Water and Measurement of Hydrophobicity Constants, Microchem. J., 2019, 145, 988–995, DOI:10.1016/j.microc.2018.11.058.
- X. X. Zhang, Y. Q. Liu, H. Yu and R. Q. Zhang, Rapid and Simultaneous Determination of Piperidinium and Pyrrolidinium Ionic Liquid Cations by Ion Pair Chromatography Coupled with Direct Conductivity Detection, Chin. Chem. Lett., 2017, 28(1), 126–130, DOI:10.1016/j.cclet.2016.05.034.
- S. Li, H. Yu and Y. Ma, Rapid Determination of Trifluoromethanesulfonate and P-Toluenesulfonate by Ion-Pair Chromatography Using a Reversed-Phase Silica-Based Monolithic Column: Application to the Analysis of Ionic Liquids, Chromatographia, 2011, 74(11–12), 759–765, DOI:10.1007/s10337-011-2141-0.
- S. Li, H. Yu, Y. Liu and M. Li, Rapid and Simultaneous Determination of Trifluoroacetate, Trifluoromethanesulfonate, Tetrafluoroborate, and Hexafluorophosphate Organic and Inorganic Anions of Ionic Liquids by Ion-Pair Chromatography Using a Reversed-Phase Silica-Based Monolithic Column, J. Liq. Chromatogr. Relat. Technol., 2013, 36(11), 1490–1502, DOI:10.1080/10826076.2012.692146.
-
C. J. Clarke, Ionic Liquids as Designer Molecules for XPS Peak Fitting, PhD thesis, University of Nottingham, 2016.
- T. Mandai, M. Imanari and K. Nishikawa, Linker-Length Dependence of the Reorientational Dynamics and Viscosity of Bis(Imidazolium)-Based Ionic Liquids Incorporating Bis(Trifluoromethanesulfonyl) Amide Anions, Chem. Phys. Lett., 2012, 543, 72–75, DOI:10.1016/j.cplett.2012.06.026.
- R. K. Blundell and P. Licence, Quaternary Ammonium and Phosphonium Based Ionic Liquids: A Comparison of Common Anions, Phys. Chem. Chem. Phys., 2014, 16, 15278–15288, 10.1039/c4cp01901f.
- H. P. Wagner, B. V. Pepich, C. Pohl, D. Later, K. Srinivasan, R. Lin, B. DeBorba and D. J. Munch, Selective Method for the Analysis of Perchlorate in Drinking Waters at Nanogram per Liter Levels, Using Two-Dimensional Ion Chromatography with Suppressed Conductivity Detection, J. Chromatogr. A, 2007, 1155(1), 15–21, DOI:10.1016/J.CHROMA.2007.03.025.
- K. Kosaka, M. Asami, Y. Matsuoka, M. Kamoshita and S. Kunikane, Occurrence of Perchlorate in Drinking Water Sources of Metropolitan Area in Japan, Water Res., 2007, 41(15), 3474–3482, DOI:10.1016/J.WATRES.2007.05.011.
Footnotes |
† Electronic supplementary information (ESI) available: NMR, MS, and IC for the synthesized ionic liquid. Synthetic seawater chromatograms are also shown along with additional plots derived from chromatograms. See DOI: 10.1039/d0ay00337a |
‡ GSK Carbon Neutral Laboratory, University of Nottingham, UK. |
|
This journal is © The Royal Society of Chemistry 2020 |