DOI:
10.1039/C9SC03747K
(Edge Article)
Chem. Sci., 2019,
10, 9560-9564
Cobalt-catalyzed hydroxymethylarylation of terpenes with formaldehyde and arenes†
Received
30th July 2019
, Accepted 26th August 2019
First published on 26th August 2019
Abstract
Terpenes, consisting of isoprene monomer units, represent a family of naturally abundant compounds. The difunctionalization of terpenes is highly appealing yet remains challenging, since the multiple unbiased C
C bonds of terpenes lead to difficulty in controlling the regioselectivity. Herein, a cobalt(III)-catalyzed C–H activation strategy has been developed to facilitate hydroxymethylarylation of terpenes with formaldehyde and arenes with high chemo- and regio-selectivities. These (chemo- and regio-) selectivities are governed by the coordination abilities of isoprene, directing groups and the steric effect. This terpene difunctionalization also features high atom and step economy through a C–H addition pathway.
Introduction
Homoallylic alcohols and their derivatives are versatile synthetic intermediates that have been utilized for rapid buildup of molecular complexity.1 Additionally, the homoallylic alcohol fragment itself is found in a number of biologically active compounds and natural products (Scheme 1). For instance, the cryptophycin family consists of cytotoxins found in blue-green alga and show excellent activity against solid tumors.2 Pleuromutilins inhibit the growth of predominantly Gram-positive pathogens.3 Maoecrystal V shows potential selectivity against HeLa cell lines.4 Bryostatins exhibit remarkable biological activity against a range of cancers and other diseases such as Alzheimer's disease.5 Therefore, considerable efforts have been devoted to the catalytic synthesis of homoallylic alcohols over the past few decades.1
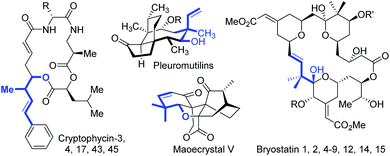 |
| Scheme 1 Representative homoallylic alcohols and their derivatives in nature. | |
Transition metal-catalyzed reductive allylation of ketones/aldehydes with 1,3-dienes has been shown as an efficient approach for the construction of homoallylic alcohols.6 Although remarkable progress has been achieved in this field, a three-component cascade reaction of arenes, dienes and carbonyls, which can be used for rapid assembly of more complex molecules, still remains limited. In particular, few reports employed naturally abundant terpenes7 as allyl precursors. Formaldehyde is an essential C1 bulk chemical that can be employed to introduce the hydroxymethyl group.8 Owing to this synthons can be produced from both petroleum and biomass, and direct transformation of basic feedstocks into value-added homoallylic alcohols would be highly appealing.
Through a transmetalation pathway, three-component additions of organometallic reagents to isoprene and aldehydes have been developed under Ni or Pt catalysis (Fig. 1a).9 From the viewpoint of atom and step economy, directed C–H bond addition to terpenes and carbonyls represents a more attractive process.10 In this regard, Ellman11 and Zhao12 recently reported an elegant Co- and Rh-catalyzed C–H bond addition to dienes and aldehydes, respectively. However, these reactions gave modest yields when involving terpenes as substrates and only highly activated aldehyde ethyl glyoxylate was demonstrated (Fig. 1b).12 Inspired by these studies, we set out to develop a methodology to achieve the hydroxymethylarylation of terpenes with formaldehyde and arenes. To realize this proposal, we had to overcome the following challenges: (1) chemoselectivity control. In terms of electrophilicity, formaldehyde is a better coupling partner than terpenes with initially formed carbon–metal species.8,13,14 (2) Regioselectivity control.15 Terpenes possess at least two unbiased C
C bonds, thus leading to a minimum of four different migratory insertion orientations with carbon–metal species (Fig. 1c). Herein, we demonstrated a Co-catalyzed three-component C–H bond addition to terpenes and formaldehyde.
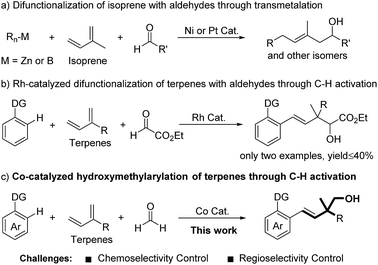 |
| Fig. 1 Catalytic difunctionalization of terpenes with aldehydes. | |
Results and discussion
We began our investigation by employing 2-phenylpyridine, isoprene, and paraformaldehyde as the model substrates (Table 1). In the presence of Cp*Co(CO)I2 (5 mol%) and AgSbF6, the desired homoallylic alcohol 4a can be obtained in 29% yield (entry 1). The evaluation of additives showed that both carboxylate salts and carboxylic acids are able to accelerate the reaction, probably by promoting the C–H activation via a concerted metallation-deprotonation pathway (entries 2–7).16 Notably, increasing the amount of HOAc to 40 mol% led to a better yield (entries 8–11). A screening of solvents suggests that dioxane is the optimal choice in terms of yield (entries 12–16).
Table 1 Optimization for hydroxymethylarylation of isprenea

|
Entry |
Additive |
x (mol%) |
Solvent |
Yieldb (%) |
Conditions: 1a (0.20 mmol), 2 (0.40 mmol), 3 (0.60 mmol), Cp*Co(CO)I2 (5 mol%), AgSbF6 (10 mol%), additive (10–50 mol%), solvent (0.50 mL), 50 °C, and 16 h.
Determined by 1H NMR with 1,3,5-trimethoxybenzene as the internal standard.
Isolated yield.
|
1 |
— |
— |
Dioxane |
29 |
2 |
PivONa |
10 |
Dioxane |
39 |
3 |
NaOAc |
10 |
Dioxane |
48 |
4 |
HCO2H |
10 |
Dioxane |
41 |
5 |
CH3CH2CO2H |
10 |
Dioxane |
58 |
6 |
PhCO2H |
10 |
Dioxane |
32 |
7 |
HOAc |
10 |
Dioxane |
57 |
8 |
HOAc |
20 |
Dioxane |
72 |
9 |
HOAc |
30 |
Dioxane |
86 |
10 |
HOAc |
40 |
Dioxane |
90(86)c |
11 |
HOAc |
50 |
Dioxane |
90 |
12 |
HOAc |
40 |
MeOH |
51 |
13 |
HOAc |
40 |
DCM |
60 |
14 |
HOAc |
40 |
PhCl |
56 |
15 |
HOAc |
40 |
THF |
74 |
16 |
HOAc |
40 |
MeCN |
14 |
With the optimized conditions in hand, we subsequently explored the substrate scope for this three-component reaction (Table 2). Subjecting benzoquinoline to standard conditions produced homoallylic alcohol 4b in a nearly quantitative yield. 2-Phenylpyrimidine was a suitable substrate as well (4c). Both electron-withdrawing and -donating groups on the pyridine motif were compatible with the process (4d–f). The substituents on the phenyl ring were further surveyed. Fluoro-substituted arenes underwent the reaction smoothly, providing the corresponding products in good yields (4g and 4h). Electron-donating MeO (4i, 4m, and 4q) and Me (4j and 4n), regardless of their positions, were all well tolerated. Notably, the protocol could be successfully extended to hydroxyl-substituted arenes (4u). The substrates bearing electron-withdrawing substituents such as acetyl (4k), chloro (4l and 4p), bromo (4o), and trifluoromethyl (4r) groups could also participate in the reactions. It is worth mentioning that only a single regioisomer was observed in all cases of meta- and para-substituted arenes. Remarkably, a 2-naphthyl derived arene could be quantitatively transformed into homoallylic alcohol 4s.
Table 2 Substrate scope for hydroxymethylarylation of isoprene
Next, we explored the scope of terpenes (Table 3). Increasing the isoprene units from 2 to 4 (myrcene 5a, farnesene 5b, and geranyl myrcene 5c) had no significant effect on the reactivity However, attempts to realize the coupling of ocimene, an isomer of myrcene 5a, with formaldehyde and arenes did not succeed even by varying different directing groups. Solanesene (5d) with nine isoprene units, owing to its poor solubility, gave the target product in a lower yield. Phytadiene (5e), derived from chlorophyll, was also applicable for the transformation. In the cases of natural terpenoids myrcenol (5f) and farnesenol (5g), the reactions occurred with high efficiency (79–81%). A bicyclic diterpene sclarene (5h) was also suitable for this three-component protocol.
Table 3 Substrate scope of terpenes
To verify the practical utility of this protocol, a gram scale reaction has been carried out to deliver hydroxymethylarylation product 6a in 84% yield (1.565 g, Fig. 2). Oxidation of 6a with mCPBA gave epoxide 9 in 54% yield accompanied by a small amount of seven-membered cyclic product 10. Notably, protic (DCM/H2O 1
:
1) solvent could promote the ring opening of epoxide 9.17 This observation inspired us to use HOTf (30 mol%) as an additive, which could facilitate the direct synthesis of 10 (37% yield) from 6a.
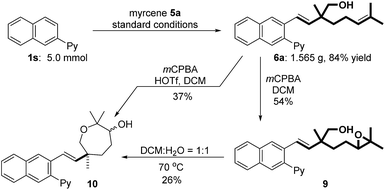 |
| Fig. 2 Scale-up experiment and further derivatization. | |
Some control experiments have been performed to explore the mechanism (Fig. 3). Kinetic isotope effect (KIE) experiments were performed with the reactions of 1a in parallel and in competition with D5-1a under standard conditions. The KIE values for the parallel and competitive reactions were determined to be 2.8 and 2.0, respectively (eqn (1)). These results imply that C–H bond cleavage might be involved in the rate-determining step. The incorporation of hydrogen atoms onto the recovered starting material indicates that there is a reversible C–H activation process under this cobalt catalysis (eqn (2)). The employment of AcOD as an additive afforded the target product in 70% yield with deuterium atoms incorporating in the methyl group (D1-4a), indicating that relatively few H-D exchange reactions were involved in the process (eqn (3)). When formaldehyde was not involved in the reaction, an oxidative coupling of isoprene with 1a occurred but gave a low yield of 7, which presumably was ascribed to the absence of an external oxidant (eqn (4)). A small amount of direct formaldehyde hydroarylation product 8 (ref. 13) could be obtained in the absence of isoprene. However, no hydroarylation product 8 was observed under standard conditions (eqn (5)). Both two-component coupling results (eqn (4) and (5)) suggest the chemoselectivity most likely originates from the difference in coordination ability between terpenes and formaldehyde toward the formed aryl-cobalt intermediate.
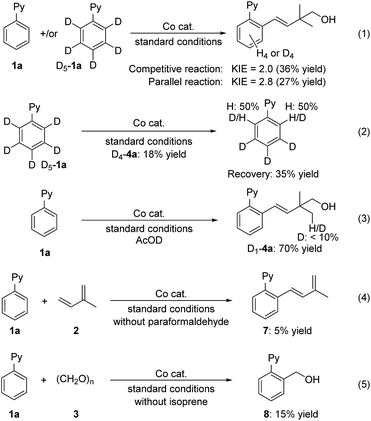 |
| Fig. 3 Mechanistic studies. | |
To better interpret the origin of regioselectivity, we provided more details about potential pathways for the formation of regiospecific homoallylic alcohol products (Fig. 4). According to different addition orientations, the addition of 5-membered Co-complex A (generated from the C–H activation step between the Co precatalyst and 1a) to isoprene may generate four addition modes (B1, B2, B3 and B4). However, due to the steric hindrance (B1 and B2) or β-elimination issue (B3), 1,2-/2,1- or 3,4-adducts should be disfavored. Instead, 4,3-adduct B4 can be smoothly produced through β-elimination to deliver Co-hydride C. Then, 1,4-insertion of Co–H into the isoprene unit gives prenyl-Co intermediate D. Finally, the directed addition of D to formaldehyde through a chair-like transition state E yields homoallylic alcohol product 4a and regenerates the cobalt catalyst.
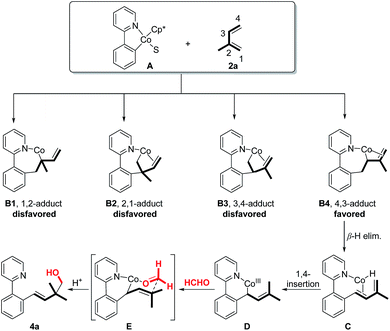 |
| Fig. 4 Proposed mechanism for regioselectivity control. | |
Conclusions
In conclusion, a three-component protocol for the hydroxymethylarylation of terpenes with paraformaldehyde and arenes has been developed via cobalt catalysis.18 The chemoselectivity results from the difference in the coordination ability between terpenes and formaldehyde. The steric hindrance and coordination abilities of isoprene and directing groups dominate the regioselectivities. Through a C–H addition pathway, this terpene difunctionalization protocol also features high atom and step economy. Further studies on the enantioselective hydroxymethylarylation of terpenes are currently underway in our laboratory.
Conflicts of interest
There are no conflicts to declare.
Acknowledgements
We thank Dr Daniel Kim (Princeton University) for helpful discussion and paper revisions. Financial support from the Dalian Institute of Chemical Physics, the National Natural Science Foundation of China (21702204 and 21801239), the Liaoning Revitalization Talents Program (XLYC1807181), and the “Thousand Youth Talents Plan” is acknowledged. This work is dedicated to the 70th anniversary of the Dalian Institute of Chemical Physics, Chinese Academy of Sciences.
Notes and references
- For representative reviews on the synthesis of homoallylic alcohols see:
(a) M. Yus, J. C. Gonzalez-Gomez and F. Foubelo, Chem. Rev., 2011, 111, 7774 CrossRef CAS PubMed;
(b) M. Yus, J. C. Gonzalez-Gomez and F. Foubelo, Chem. Rev., 2013, 113, 5595 CrossRef CAS PubMed;
(c) H.-X. Huo, J. R. Duvall, M.-Y. Huang and R. Hong, Org. Chem. Front., 2014, 1, 303 RSC;
(d) J. Feng, M. Holmes and M. J. Krische, Chem. Rev., 2017, 117, 12564 CrossRef CAS PubMed;
(e) M. Holmes, L. A. Schwartz and M. J. Krische, Chem. Rev., 2018, 118, 6026 CrossRef CAS PubMed;
(f) Y.-L. Liu and X.-T. Lin, Adv. Synth. Catal., 2019, 361, 876 CrossRef CAS.
-
(a) G. Trimurtulu, I. Ohtani, G. M. L. Patterson, R. E. Moore, T. H. Corbett, F. A. Valeriote and L. Demchik, J. Am. Chem. Soc., 1994, 116, 4729 CrossRef CAS;
(b) R. A. Barrow, T. Hemscheidt, J. Liang, S. Paik, R. E. Moore and M. A. Tius, J. Am. Chem. Soc., 1995, 117, 2479 CrossRef CAS;
(c) T. Golakoti, J. Ogino, C. E. Heltzel, L. T. Husebo, C. M. Jensen, L. K. Larsen, G. M. L. Patterson, R. E. Moore, S. L. Mooberry, T. H. Corbett and F. A. Valeriote, J. Am. Chem. Soc., 1995, 117, 12030 CrossRef CAS.
-
(a) F. Kavanagh, A. Hervey and W. J. Robbins, Proc. Natl. Acad. Sci. U. S. A., 1951, 37, 570 CrossRef CAS PubMed;
(b) S. K. Murphy, M. Zeng and S. B. Herzon, Science, 2017, 356, 956 CrossRef CAS PubMed;
(c) M. Zeng, S. K. Murphy and S. B. Herzon, J. Am. Chem. Soc., 2017, 139, 16377 CrossRef CAS PubMed.
-
(a) S. H. Li, J. Wang, X. M. Niu, Y. H. Shen, H. J. Zhang, H. D. Sun, M. L. Li, Q. E. Tian, Y. Lu, P. Cao and Q. T. Zheng, Org. Lett., 2004, 6, 4327 CrossRef CAS PubMed;
(b) P. Lu, A. Mailyan, Z. Gu, D. M. Guptill, H. Wang, H. M. L. Davies and A. Zakarian, J. Am. Chem. Soc., 2014, 136, 17738 CrossRef CAS PubMed;
(c) A. Cernijenko, R. Risgaard and P. S. Baran, J. Am. Chem. Soc., 2016, 138, 9425 CrossRef CAS PubMed . Maoecrystal V showed little to no activity against cancer cell lines according to Baran's report.
-
(a) G. R. Pettit, J. F. Day, J. L. Hartwell and H. B. Wood, Nature, 1970, 227, 962 CrossRef CAS PubMed;
(b) G. R. Pettit, C. L. Herald, D. L. Doubek, D. L. Herald, E. Arnold and J. Clardy, J. Am. Chem. Soc., 1982, 104, 6846 CrossRef CAS;
(c) B. M. Trost and G. Dong, Nature, 2008, 456, 485 CrossRef CAS PubMed.
- For selected examples on catalytic reductive coupling of dienes with carbonyl compounds see:
(a) M. Kimura, A. Ezoe, M. Mori, K. Iwata and Y. Tamaru, J. Am. Chem. Soc., 2006, 128, 8559 CrossRef CAS PubMed;
(b) F. Shibahara, J. F. Bower and M. J. Krische, J. Am. Chem. Soc., 2008, 130, 6338 CrossRef CAS PubMed;
(c) H. Y. Cho and J. P. Morken, J. Am. Chem. Soc., 2010, 132, 7576 CrossRef CAS PubMed;
(d) M. Fukushima, D. Takushima and M. Kimura, J. Am. Chem. Soc., 2010, 132, 16346 CrossRef CAS PubMed;
(e) J. R. Zbieg, E. L. McInturff, J. C. Leung and M. J. Krische, J. Am. Chem. Soc., 2011, 133, 1141 CrossRef CAS PubMed;
(f) J. R. Zbieg, J. Moran and M. J. Krische, J. Am. Chem. Soc., 2011, 133, 10582 CrossRef CAS PubMed;
(g) L. T. Kliman, S. N. Mlynarski, G. E. Ferris and J. P. Morken, Angew. Chem., Int. Ed., 2012, 51, 521 CrossRef CAS PubMed;
(h) J. R. Zbieg, E. Yamaguchi, E. L. McInturff and M. J. Krische, Science, 2012, 336, 324 CrossRef CAS PubMed;
(i) B. Y. Park, T. P. Montgomery, V. J. Garza and M. J. Krische, J. Am. Chem. Soc., 2013, 135, 16320 CrossRef CAS PubMed;
(j) K. D. Nguyen, D. Herkommer and M. J. Krische, J. Am. Chem. Soc., 2016, 138, 14210 CrossRef CAS PubMed;
(k) Y. Y. Gui, N. Hu, X. W. Chen, L. L. Liao, T. Ju, J. H. Ye, Z. Zhang, J. Li and D. G. Yu, J. Am. Chem. Soc., 2017, 139, 17011 CrossRef CAS PubMed;
(l) B. Fu, X. Yuan, Y. Li, Y. Wang, Q. Zhang, T. Xiong and Q. Zhang, Org. Lett., 2019, 21, 3576 CrossRef CAS PubMed;
(m) C. Li, R. Y. Liu, L. T. Jesikiewicz, Y. Yang, P. Liu and S. L. Buchwald, J. Am. Chem. Soc., 2019, 141, 5062 CrossRef CAS PubMed;
(n) R. Y. Liu, Y. Zhou, Y. Yang and S. L. Buchwald, J. Am. Chem. Soc., 2019, 141, 2251 CrossRef CAS PubMed.
-
(a) E. M. Davis and R. Croteau, Top. Curr. Chem., 2000, 209, 53 CrossRef CAS;
(b) K. Chen and P. S. Baran, Nature, 2009, 459, 824 CrossRef CAS PubMed;
(c) Z. G. Brill, M. L. Condakes, C. P. Ting and T. J. Maimone, Chem. Rev., 2017, 117, 11753 CrossRef CAS PubMed.
- B. Sam, B. Breit and M. J. Krische, Angew. Chem., Int. Ed., 2015, 54, 3267 CrossRef CAS.
- For the catalytic three-component reaction of isoprene and aldehydes through the transmetalation pathway see:
(a) M. Kimura, S. Matsuo, K. Shibata and Y. Tamaru, Angew. Chem., Int. Ed., 1999, 38, 3386 CrossRef CAS;
(b) M. Kimura, K. Shibata, Y. Koudahashi and Y. Tamaru, Tetrahedron Lett., 2000, 41, 6789 CrossRef CAS;
(c) J. B. Morgan and J. P. Morken, Org. Lett., 2003, 5, 2573 CrossRef CAS PubMed;
(d) M. Kimura, A. Ezoe, M. Mori and Y. Tamaru, J. Am. Chem. Soc., 2005, 127, 201 CrossRef CAS PubMed;
(e) H. Y. Cho and J. P. Morken, J. Am. Chem. Soc., 2008, 130, 16140 CrossRef CAS PubMed;
(f) Y. Ohira, M. Hayashi, T. Mori, G. Onodera and M. Kimura, New J. Chem., 2014, 38, 330 RSC;
(g) Y. Xiong and G. Zhang, J. Am. Chem. Soc., 2018, 140, 2735 CrossRef CAS.
- For representative reviews on C–H functionalization see:
(a) H. M. L. Davies and R. E. J. Beckwith, Chem. Rev., 2003, 103, 2861 CrossRef CAS;
(b) R. Giri, B.-F. Shi, K. M. Engle, N. Maugel and J.-Q. Yu, Chem. Soc. Rev., 2009, 38, 3242 RSC;
(c) D. A. Colby, R. G. Bergman and J. A. Ellman, Chem. Rev., 2010, 110, 624 CrossRef CAS;
(d) T. W. Lyons and M. S. Sanford, Chem. Rev., 2010, 110, 1147 CrossRef CAS;
(e) I. A. I. Mkhalid, J. H. Barnard, T. B. Marder, J. M. Murphy and J. F. Hartwig, Chem. Rev., 2010, 110, 890 CrossRef CAS PubMed;
(f) S. H. Cho, J. Y. Kim, J. Kwak and S. Chang, Chem. Soc. Rev., 2011, 40, 5068 RSC;
(g) H. M. L. Davies, J. Du Bois and J.-Q. Yu, Chem. Soc. Rev., 2011, 40, 1855 RSC;
(h) C.-L. Sun, B.-J. Li and Z.-J. Shi, Chem. Rev., 2011, 111, 1293 CrossRef CAS;
(i) J. Wencel-Delord, T. Droege, F. Liu and F. Glorius, Chem. Soc. Rev., 2011, 40, 4740 RSC;
(j) J. He, M. Wasa, K. S. L. Chan, O. Shao and J.-Q. Yu, Chem. Rev., 2017, 117, 8754 CrossRef CAS.
-
(a) J. A. Boerth, S. Maity, S. K. Williams, B. Q. Mercado and J. A. Ellman, Nat. Catal., 2018, 1, 673 CrossRef CAS PubMed;
(b) J. A. Boerth, J. R. Hummel and J. A. Ellman, Angew. Chem., Int. Ed., 2016, 55, 12650 CrossRef CAS PubMed;
(c) J. A. Boerth and J. A. Ellman, Angew. Chem., Int. Ed., 2017, 56, 9976 CrossRef CAS.
- R. Li, C.-W. Ju and D. Zhao, Chem. Commun., 2019, 55, 695 RSC.
- For selective examples on catalytic coupling of formaldehyde with arenes see:
(a) Y. Wu and B. Zhou, ACS Catal., 2017, 7, 2213 CrossRef CAS;
(b) G.-F. Zhang, Y. Li, X.-Q. Xie and C.-R. Ding, Org. Lett., 2017, 19, 1216 CrossRef CAS PubMed;
(c) C. Zhu, T. Pinkert, S. Gressies and F. Glorius, ACS Catal., 2018, 8, 10036 CrossRef CAS;
(d) S. H. Lee, T. Jeong, K. Kim, N. Y. Kwon, A. K. Pandey, H. S. Kim, J.-M. Ku, N. K. Mishra and I. S. Kim, J. Org. Chem., 2019, 84, 2307 CrossRef CAS;
(e)
T. Wan, S. Du, C. Pi, Y. Wang, R. Li, Y. Wu, X. Cui. ChemCatChem, 2019, 11, 3791 Search PubMed.
- For selective examples on catalytic coupling of formaldehyde with isoprene see:
(a) T. Smejkal, H. Han, B. Breit and M. J. Krische, J. Am. Chem. Soc., 2009, 131, 10366 CrossRef CAS PubMed;
(b) A. Koepfer, B. Sam, B. Breit and M. J. Krische, Chem. Sci., 2013, 4, 1876 RSC.
- Y.-C. Hu, D.-W. Ji, C.-Y. Zhao, H. Zheng and Q.-A. Chen, Angew. Chem., Int. Ed., 2019, 58, 5438 CrossRef CAS PubMed.
-
(a) L. Ackermann, Chem. Rev., 2011, 111, 1315 CrossRef CAS PubMed;
(b) P. Gandeepan, T. Mueller, D. Zell, G. Cera, S. Warratz and L. Ackermann, Chem. Rev., 2019, 119, 2192 CrossRef CAS PubMed.
- I. Vilotijevic and T. F. Jamison, Science, 2007, 317, 1189 CrossRef CAS PubMed.
- During the preparation of this manuscript, a related study was reported by Ellman's group (Just accepted online, July 16). Our work can serve as a complementary method which focuses on the reactions of terpenes and formaldehyde. D. Sun, Z. Chen and J. A. Ellman, Angew. Chem., Int. Ed., 2019, 58, 12590 CrossRef.
Footnote |
† Electronic supplementary information (ESI) available. See DOI: 10.1039/c9sc03747k |
|
This journal is © The Royal Society of Chemistry 2019 |
Click here to see how this site uses Cookies. View our privacy policy here.