DOI:
10.1039/C8SC05581E
(Edge Article)
Chem. Sci., 2019,
10, 3765-3769
Chiral phosphoric acid-catalyzed enantioselective construction of structurally diverse benzothiazolopyrimidines†
Received
14th December 2018
, Accepted 6th February 2019
First published on 7th February 2019
Abstract
A highly efficient catalytic enantioselective [4+2] cycloaddition was developed between 2-benzothiazolimines and enecarbamates. A wide range of benzothiazolopyrimidines bearing three contiguous stereogenic centers was obtained in high to excellent yields and with excellent diastereo- and enantioselectivities (d.r. > 98
:
2 and up to >99% ee). Furthermore, this chiral phosphoric acid-catalyzed strategy was scalable and enabled access to a new class of optically pure Lewis base isothiourea derivatives.
Introduction
Isothioureas constitute an important class of organocatalysts due to their highly nucleophilic nature.1 Recent efforts have been directed to the development of chiral derivatives capable of promoting enantioselective transformations, which are difficult to control using traditional nucleophilic catalysts. Among the several promising examples, benzothiazolopyrimidine, better known as homobenzotetramisole (HBTM), has assumed a leading position as a chiral catalyst. Chiral HBTMs have been successfully used in a number of asymmetric transformations1a including the kinetic resolution of secondary alcohols and carboxylic acids,2 desymmetrization,3 acyl transfer,4 annulation processes,5 and lactonisation,6 among others.7 Nevertheless, despite the interest in optically active HBTMs,1a there have been only limited reports on their enantioselective synthesis.8 In 2008, Birman et al. disclosed the first asymmetric synthesis of HBTMs following a seven-step sequence involving a key SNAr reaction between 2-chlorobenzo[d]thiazole and amino-alcohols prepared from the chiral pool of amino acids (Scheme 1a).2f Chiral amino-alcohols, produced by catalytic enantioselective procedures, were later engaged in similar routes as reported by the same group as well as by Smith et al. and Okamoto et al. (Scheme 1a).4a,b,9 More recently, Enders et al. established an efficient asymmetric synthesis of precursors of HBTMs by the N-heterocyclic carbene-catalyzed Mannich/lactamization domino reaction of 2-benzothiazolimines and α-chloroaldehydes (Scheme 1b).10 Despite these examples, no catalytic asymmetric approach has been reported so far to directly construct these heterocycles. An attractive direct access relying on the aza-Diels–Alder approach has been reported by Mellor et al. for the synthesis of homobenzotetramisole in an achiral stoichiometric context.9,11 The requirement of a full equivalent of the acid to perform the reaction is self-evident due to the presence of the basic isothiourea that might capture the acid during the formation of HBTMs.2f,12 Turning this process into a catalytic enantioselective approach would be highly appealing, not only to access new potent chiral Lewis bases but also for the generation of novel biologically active molecules.13
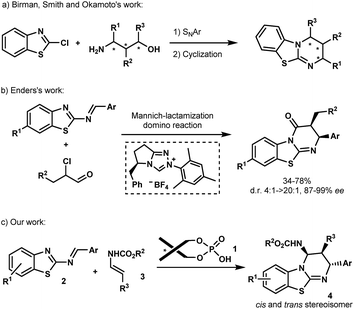 |
| Scheme 1 Previous studies and our strategy. | |
In the past few years, chiral phosphoric acids have been widely recognized as powerful catalysts for the synthesis of various heterocycles containing nitrogen atoms.14 In this context, we have recently documented phosphoric acid-catalyzed enantioselective aza-Diels–Alder reactions14,15 of various azadienes with enecarbamates as dienophiles.16 Based on these studies, we envisioned that this Brønsted acid 1 may be capable of promoting the [4+2] cycloaddition between 2-benzothiazolimines 2 and enecarbamates 3 (Scheme 1c) allowing the development of an enantioselective synthesis of benzothiazolopyrimidines 4.
Because of the formation of the basic isothiourea during the process, catalyst poisoning might lead to difficulties in developing a catalytic version. Nevertheless, we reasoned that tuning the catalyst or using additives to induce a hydrogen shuttle could help in overcoming the problem.17
Results and discussion
With this proposal in mind, we examined the aza-Diels–Alder reaction of 2-benzothiazolimine182a with (E)-N-benzyl propenylcarbamate (3a) in the presence of chiral phosphoric acids 1. Gratifyingly, in dichloromethane at room temperature, the reaction promoted by 1a afforded benzothiazolopyrimidine 4a as a single diastereomer, with an enantiomeric excess of 95% albeit with a low yield (Table 1, entry 1). Obviously, while yields remained low, we were delighted to observe that no full catalyst inhibition was occurring. Encouraged by this result, we screened various reaction conditions to search for the optimal reaction conditions. Performing the reaction at 50 °C in DCE slightly improved the kinetics (16 h instead of 24 h) even if overall yields were not superior and significant erosion of enantioselectivity was observed.19 Then, a series of phosphoric acids were screened in DCE at 50 °C (entries 2–4), and we found that the catalyst structure had a marked impact on the reaction efficiency. For instance, the use of chiral phosphoric acid 1c having two bulky 3,3′-(2,4,6-triisopropylphenyl) groups improved the yield, presumably by facilitating the catalyst turnover. A further survey of reaction conditions revealed that the optimal conditions consisted in performing the reaction with 3 equivalents of 3a in the presence of 10 mol% of 1c, delivering 4a in 90% yield with almost perfect diastereo- and enantioselectivity (entry 5).
Table 1 Optimization of the enantioselective [4+2] cycloadditiona
With optimized reaction conditions in hand, the scope of this chiral phosphoric acid-catalyzed enantioselective aza-Diels-Alder reaction was investigated and the results are summarized in Scheme 2. First, 2-benzothiazolimines bearing various substituents on the aromatic rings were evaluated. Pleasingly, the reaction was insensitive to the electronic nature of the substituents on the benzothiazole rings. For instance, the benzothiazole bearing either electron-donating or electron-withdrawing groups, such as methyl, halogen and trifluoromethyl groups, provided the corresponding benzothiazolopyrimidine 4b–f in high yields and excellent enantioselectivities (up to 99% ee). An unsubstituted benzothiazole ring proceeded uneventfully to give cycloadduct 4g with almost perfect enantioselectivity (up to 99% ee). It is worth noting that the reactions performed on a 1 mmol scale displayed the same results as the ones on a 0.10 mmol scale, further indicating the practicality of this protocol. On the other hand, the electronic properties of aromatic rings that came from aldehydes had no significant impact on the enantiomeric excess, though the reaction with the electron-rich imine gave a slightly lower yield (4hvs.4a). When the reaction was conducted with imine, which had bromine at the meta-position, the desired cycloadduct 4k was obtained in 80% yield and 98% ee. Notably, 4k can be enriched to >99% ee by single recrystallization, which is very interesting in view of their use as organocatalysts. Moreover, a relative and absolute configuration was unambiguously determined by single crystal X-ray analysis (see the ESI†). Finally, the reaction with imine 2l derived from 2-thiophenecarboxaldehyde proceeded smoothly to give the cycloadduct 4l in almost quantitative yields with 98% ee. This novel catalytic enantioselective cycloaddition was also applied to representative enecarbamates (Scheme 2). Notably, a variety of protecting groups bonded to the nitrogen atom of 3 (including Boc, Alloc and 4-(trifluoromethyl)benzylcarbamate) were well tolerated and the corresponding benzothiazolopyrimidines (4m–q) were, in all cases, isolated in excellent yields and excellent stereoselectivities. It is noteworthy that the catalyst loading was successfully reduced to 5.0 mol% (4q, entry 10). A comparable enantioselectivity (97%) was still achieved with a slightly lower chemical yield (70%).
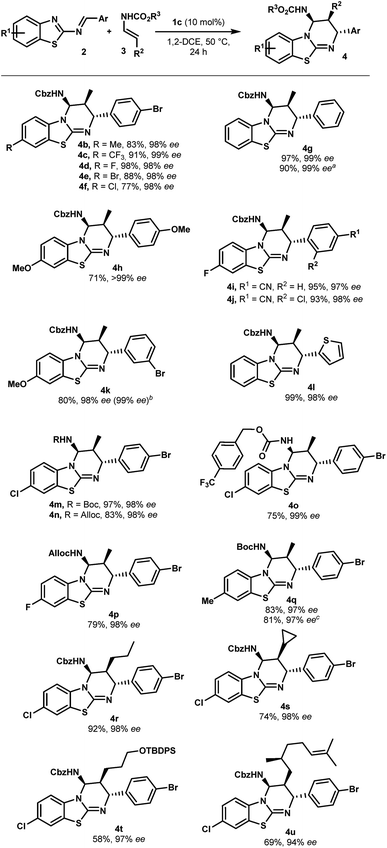 |
| Scheme 2 Scope of the enantioselective synthesis of benzothiazolopyrimidines 4. a General reaction conditions: 2 (0.1 mmol), 3 (0.3 mmol), 1c (10 mol%), 1,2-DCE (1 mL, 0.1 M), 50 °C, and 24 h. Yields refer to chromatographically purified compounds. The d.r. values were determined by 1H NMR analysis to be higher than 98 : 2 in all cases. The ee values were determined by HPLC on a chiral stationary phase: see the ESI† for details. a From 1.0 mmol of 2g. b After recrystallization. c From 0.3 mmol of 2b. d With 5 mol% of 1c. | |
In addition, β-substituted enecarbamates bearing different alkyl groups reacted efficiently to deliver expected trisubstituted-cycloadducts 4r–u in good to excellent yields with high selectivities (94 to 98% ee). The reaction with 3c or 3d containing a cyclopropyl or silyl ether group formed products 4s or 4t with a slight decrease in the yield without affecting asymmetric induction.
The stereoselective issue of the reaction ((E)-enecarbamates to the cis product) raises questions about the mechanism. In order to get insight, we computationally evaluated the free energy of both diastereoisomers of the product 4g (Fig. 1A). The trans form was found favoured by more than 5.2 kcal mol−1 over its cis sibling (see the ESI†). This result together with the fact that the cis isomer is actually observed suggests that the cycloaddition occurs under kinetic control. A stepwise process was established by performing a control experiment in the presence of 3 equivalents of ethanol, which evidenced the formation of an N-Cbz-iminium intermediate (5, Fig. 1B).
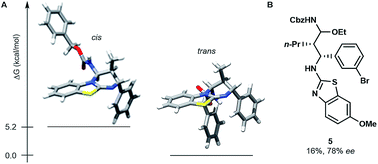 |
| Fig. 1 (A) Most stable cis and trans conformers of 4g found at the B3LYP/6-31G(d) level. (B) Structure of intermediate 5 obtained in the presence of 3.0 equiv. of ethanol on the way to 4k. | |
Based on this, as well as our previous work and other published reports,14,16,20 a possible catalytic mechanism was proposed in Scheme 3. We hypothesized that 1 would form a hydrogen network with the nitrogen Lewis basic sites of 2-benzothiazolimine 2 and the acidic site of enecarbamate 3, leading to complex 6. Mediated by the phosphoric acid double activation, subsequent bond formation would deliver complex 7. The aromatic and R residue would be positioned in a trans situation as found in the product, but the pseudo equatorial arrangement would create substantial strain. Maintained in the chiral cavity by the double hydrogen anchors, rotation of the backbone would furnish a sterically less-congested conformer 8. Finally the intramolecular nucleophilic addition of benzothiazole to iminium will give the observed cis–trans substituted benzothiazolopyrimidine 4.21 Heating, rapid formation of hydrogen-bonded complex 6 or use of excess 3 may all facilitate the decomplexation of 9 and ensure the catalyst turnover.
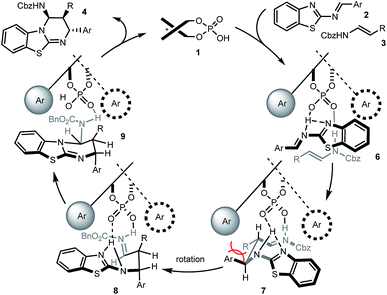 |
| Scheme 3 Plausible mechanism and transition states. | |
As a further illustration of the utility of this novel catalytic cycloaddition, we developed conditions to convert the carbamate group into other functional groups. The N-Boc group of 4q was removed by mild acidic hydrolysis to produce free alcohol 10 (a key intermediate to install additional molecular diversity) with no erosion of enantiopurity (Scheme 4). The absolute configuration was confirmed without ambiguity by X-ray diffraction analysis (see the ESI†).
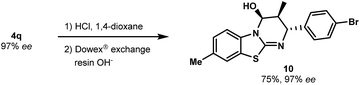 |
| Scheme 4 Derivatization of the benzothiazolopyrimidine product 4q. | |
Conclusions
We have developed an efficient enantioselective [4+2] cycloaddition organocatalyzed by chiral phosphoric-acid for the synthesis of benzothiazolopyrimidines bearing three contiguous stereogenic centers, which were unreported before. Involving 2-benzothioazolimines and enecarbamates as substrates, this scalable strategy furnished a wide range of compounds with very high yields and enantioselectivity in a diastereospecific manner. The obtained cycloadducts were successfully converted into corresponding free alcohol heterocycles without any loss of enantioselectivity. Further investigations are in progress concerning the catalytic activity of these new compounds as chiral nucleophilic organocatalysts.
Conflicts of interest
There are no conflicts to declare.
Acknowledgements
We thank ICSN and CNRS for financial support; L. J. acknowledges Saclay University for the doctoral fellowship. D. J. acknowledges the French Embassy and an RBI fellowship for financial support. The IT department of Paris-Sud University is acknowledged for providing computing resources.
Notes and references
-
(a) J. Merad, J.-M. Pons, O. Chuzel and C. Bressy, Eur. J. Org. Chem., 2016, 5589 CrossRef
;
(b) S. Vellalath and D. Romo, Angew. Chem., Int. Ed., 2016, 55, 13934 CrossRef CAS PubMed
;
(c) J. E. Taylor, S. D. Bull and J. M. J. Williams, Chem. Soc. Rev., 2012, 41, 2109 RSC
;
(d) N. De Rycke, F. Couty and O. R. P. David, Chem.–Eur. J., 2011, 17, 12852 CrossRef CAS PubMed
.
- For selected examples, see:
(a) M. D. Greenhalgh, S. M. Smith, D. M. Walden, J. E. Taylor, Z. Brice, E. R. T. Robinson, C. Fallan, D. B. Cordes, A. M. Z. Slawin, H. C. Richardson, M. A. Grove, P. H.-Y. Cheong and A. D. Smith, Angew. Chem., Int. Ed., 2018, 57, 3200 (
Angew. Chem.
, 2018
, 130
, 3254
) CrossRef CAS PubMed
;
(b) K. Nakata, K. Gotoh, K. Ono, K. Futami and I. Shiina, Org. Lett., 2013, 15, 1170 CrossRef CAS PubMed
;
(c) I. Shiina, K. Nakata, K. Ono, L. Y.-S. Onda and M. Itagaki, J. Am. Chem. Soc., 2010, 132, 11629 CrossRef CAS PubMed
;
(d) I. Shiina, K. Nakata, K. Ono, M. Sugimoto and A. Sekiguchi, Chem.–Eur. J., 2010, 16, 167 CrossRef CAS PubMed
;
(e) X. Yang and V. B. Birman, Adv. Synth. Catal., 2009, 351, 2301 CrossRef CAS PubMed
;
(f) V. B. Birman and X. Li, Org. Lett., 2008, 10, 1115 CrossRef CAS PubMed
.
- For selected recent examples, see:
(a) J. Merad, P. Borkar, F. Caijo, J.-M. Pons, J.-L. Parrain, O. Chuzel and C. Bressy, Angew. Chem., Int. Ed., 2017, 56, 16052 (
Angew. Chem.
, 2017
, 129
, 16268
) CrossRef CAS PubMed
;
(b) J. Merad, P. Borkar, T. B. Yenda, C. Roux, J.-M. Pons, J.-L. Parrain, O. Chuzel and C. Bressy, Org. Lett., 2015, 17, 2118 CrossRef CAS PubMed
;
(c) V. B. Birman, H. Jiang and X. Li, Org. Lett., 2007, 9, 3237 CrossRef CAS PubMed
.
- For selected examples, see:
(a) C. Joannesse, C. P. Johnson, C. Concellon, C. Simal, D. Philp and A. D. Smith, Angew. Chem., Int. Ed., 2009, 48, 8914 (
Angew. Chem.
, 2009
, 121
, 9076
) CrossRef CAS PubMed
;
(b) Y. Zhang and V. B. Birman, Adv. Synth. Catal., 2009, 351, 2525 CrossRef CAS PubMed
;
(c) V. B. Birman, E. W. Uffman, H. Jiang, X. Li and C. J. Kilbane, J. Am. Chem. Soc., 2004, 126, 12226 CrossRef CAS PubMed
.
- For representative examples, see:
(a) S. Wang, C. Rodríguez-Escrich and M. A. Pericàs, Angew. Chem., Int. Ed., 2017, 56, 15068 (
Angew. Chem.
, 2017
, 129
, 15264
) CrossRef CAS PubMed
;
(b) S. L. Wang, J. Izquierdo, C. Rodriguez-Escrich and M. A. Pericàs, ACS Catal., 2017, 7, 2780 CrossRef CAS
;
(c) E. R. T. Robinson, D. M. Walden, C. Fallan, M. D. Greenhalgh, P. H.-Y. Cheong and A. D. Smith, Chem. Sci., 2016, 7, 6919 RSC
;
(d) E. R. T. Robinson, C. Fallan, C. Simal, A. M. Z. Slawin and A. D. Smith, Chem. Sci., 2013, 4, 2193 RSC
.
- For selected examples, see:
(a) W. Kong and D. Romo, J. Org. Chem., 2017, 82, 13161 CrossRef CAS PubMed
;
(b) L. C. Morrill, J. Douglas, T. Lebl, A. M. Z. Slawin, D. J. Fox and A. D. Smith, Chem. Sci., 2013, 4, 4146 RSC
;
(c) C. Simal, T. Lebl, A. M. Z. Slawin and A. D. Smith, Angew. Chem., Int. Ed., 2012, 51, 3653 (
Angew. Chem.
, 2012
, 124
, 3713
) CrossRef CAS PubMed
;
(d) D. Belmessieri, L. C. Morrill, C. Simal, A. M. Z. Slawin and A. D. Smith, J. Am. Chem. Soc., 2011, 133, 2714 CrossRef CAS PubMed
;
(e) C. A. Leverett, V. C. Purohit and D. Romo, Angew. Chem., Int. Ed., 2010, 49, 9479 (
Angew. Chem.
, 2010
, 122
, 9669
) CrossRef CAS PubMed
;
(f) V. C. Purohit, A. S. Matla and D. Romo, J. Am. Chem. Soc., 2008, 130, 10478 CrossRef CAS PubMed
.
- For further examples, see:
(a) M. E. Abbasov, B. M. Hudson, D. J. Tantillo and D. Romo, Chem. Sci., 2017, 8, 1511 RSC
;
(b) J. N. Arokianathar, A. B. Frost, A. M. Z. Slawin, D. Stead and A. D. Smith, ACS Catal., 2018, 8, 1153 CrossRef CAS
;
(c) M. E. Abbasov, B. M. Hudson, D. J. Tantillo and D. Romo, J. Am. Chem. Soc., 2014, 136, 4492 CrossRef CAS PubMed
;
(d) P.-P. Yeh, D. S. B. Daniels, D. B. Cordes, A. M. Z. Slawin and A. D. Smith, Org. Lett., 2014, 16, 964 CrossRef CAS PubMed
;
(e) L. C. Morrill, L. A. Ledingham, J.-P. Couturier, J. Bickel, A. D. Harper, C. Fallan and A. D. Smith, Org. Biomol. Chem., 2014, 12, 624 RSC
;
(f) D. G. Stark, L. C. Morrill, P.-P. Yeh, A. M. Z. Slawin, T. J. C. O'Riordan and A. D. Smith, Angew. Chem., Int. Ed., 2013, 52, 11642 (
Angew. Chem.
, 2013
, 125
, 11856
) CrossRef CAS PubMed
.
- Examples of racemic synthesis:
(a) T. Raj, H. Sharma, Mayank, A. Singh, T. Aree, N. Kaur, N. Singh and D. O. Jang, ACS Sustainable Chem. Eng., 2017, 5, 1468 CrossRef CAS
;
(b) S. Okamoto, Y. Sakai, S. Watanabe, S. Nishi, A. Yoneyama, H. Katsumata, Y. Kosaki, R. Sato, M. Shiratori, M. Shibuno and T. Shishido, Tetrahedron Lett., 2014, 55, 1909 CrossRef CAS
;
(c) B. Ranieri, O. Robles and D. Romo, J. Org. Chem., 2013, 79, 6291 CrossRef PubMed
.
- B. Viswambharan, T. Okimura, S. Suzuki and S. Okamoto, J. Org. Chem., 2011, 76, 6678 CrossRef CAS PubMed
.
- Q. Ni, X. Song, J. Xiong, G. Raabe and D. Enders, Chem. Commun., 2015, 51, 1263 RSC
.
- J. M. Mellor and H. Rataj, Tetrahedron Lett., 1996, 37, 2619 CrossRef CAS
.
-
(a) B. Maji, C. Joannesse, T. A. Nigst, A. D. Smith and H. Mayr, J. Org. Chem., 2011, 76, 5104 CrossRef CAS PubMed
;
(b) M. Kobayashi and S. Okamato, Tetrahedron Lett., 2006, 47, 4347 CrossRef CAS
.
-
(a) S. Gupta, N. Ajmera, N. Gautam, R. Sharma and D. Gautam, Indian J. Chem., 2009, 48B, 853 CAS
;
(b) G. Shukla, A. K. Tiwari, V. K. Singh, A. Bajpai, H. Chandra and A. K. Mishra, Chem. Biol. Drug Des., 2008, 72, 533 CrossRef CAS PubMed
;
(c) R. A. Glennon, S. M. Tejani-Butt, W. Padgett and J. W. Daly, J. Med. Chem., 1984, 27, 1364 CrossRef CAS PubMed
;
(d) J. Yevich, D. Temple, R. Covington, D. Owens, R. Seidehamel and K. Dungan, J. Med. Chem., 1982, 25, 864 CrossRef CAS PubMed
.
- Recent reviews on chiral phosphoric acid catalysis:
(a) J. Merad, C. Lalli, G. Bernadat, J. Maury and G. Masson, Chem.–Eur. J., 2018, 24, 3925 CrossRef CAS PubMed
;
(b) D. Parmar, E. Sugiono, S. Raja and M. Rueping, Chem. Rev., 2017, 117, 10608 (
Chem. Rev.
, 2014
, 114
, 9047
) CrossRef CAS PubMed
;
(c) S. Schenker, A. Zamfir, M. Freund and S. B. Tsogoeva, Eur. J. Org. Chem., 2011, 2209 CrossRef CAS
;
(d) M. Rueping, A. Kuenkel and I. Atodiresei, Chem. Soc. Rev., 2011, 40, 4539 RSC
;
(e) D. Kampen, C. M. Reisinger and B. List, Top. Curr. Chem., 2010, 291, 395 CrossRef CAS PubMed
;
(f) M. Terada, Bull. Chem. Soc. Jpn., 2010, 83, 101 CrossRef CAS
;
(g) M. Terada, Synthesis, 2010, 1929 CrossRef CAS
;
(h) M. Terada, Chem. Commun., 2008, 4097 RSC
;
(i) T. Akiyama, Chem. Rev., 2007, 107, 5744 CrossRef CAS PubMed
;
(j) T. Akiyama, J. Itoh and K. Fuchibe, Adv. Synth. Catal., 2006, 348, 999 CrossRef CAS
.
-
(a) G. Masson, C. Lalli, M. Benohoud and G. Dagousset, Chem. Soc. Rev., 2013, 42, 902 RSC
;
(b) X. Jiang and R. Wang, Chem. Rev., 2013, 113, 5515 CrossRef CAS PubMed
;
(c) D. Bello, R. Ramón and R. Lavilla, Curr. Org. Chem., 2010, 14, 332 CrossRef CAS
;
(d) V. V. Kouznetsov, Tetrahedron, 2009, 65, 2721 CrossRef CAS
;
(e) P. Buonora, J.-C. Olsen and T. Oh, Tetrahedron, 2001, 57, 6099 CrossRef CAS
.
-
(a) H. Liu, G. Dagousset, G. Masson, P. Retailleau and J. Zhu, J. Am. Chem. Soc., 2009, 131, 4598 CrossRef CAS PubMed
;
(b) G. Dagousset, J. Zhu and G. Masson, J. Am. Chem. Soc., 2011, 133, 14804 CrossRef CAS PubMed
;
(c) G. Dagousset, P. Retailleau, G. Masson and J. Zhu, Chem.–Eur. J., 2012, 18, 5869 CrossRef CAS PubMed
;
(d) L. He, M. Bekkaye, P. Retailleau and G. Masson, Org. Lett., 2012, 14, 3158 CrossRef CAS PubMed
;
(e) L. He, G. Laurent, P. Retailleau, B. Folléas, J.-L. Brayer and G. Masson, Angew. Chem., Int. Ed., 2013, 52, 11088 (
Angew. Chem.
, 2013
, 125
, 11294
) CrossRef CAS PubMed
;
(f) J. Brioche, T. Courant, L. Alcaraz, M. Stocks, M. Furber, J. Zhu and G. Masson, Adv. Synth. Catal., 2014, 356, 1719 CrossRef CAS
;
(g) J. Pous, T. Courant, G. Bernadat, B. I. Iorga, F. Blanchard and G. Masson, J. Am. Chem. Soc., 2015, 137, 11950 CrossRef CAS PubMed
;
(h) A. Dumoulin and G. Masson, J. Org. Chem., 2016, 81, 10154 CrossRef CAS PubMed
;
(i) L. Jarrige, F. Blanchard and G. Masson, Angew. Chem., Int. Ed., 2017, 56, 10573 (
Angew. Chem.
, 2017
, 129
, 10709
) CrossRef CAS PubMed
.
- For selected examples, see:
(a) M. Rueping and C. Azap, Angew. Chem., Int. Ed., 2006, 45, 7832 (
Angew. Chem.
, 2006
, 118
, 7996
) CrossRef CAS PubMed
;
(b) G. Li and J. C. Antilla, Org. Lett., 2009, 11, 1075 CrossRef CAS PubMed
;
(c) S. Müller, M. J. Webber and B. List, J. Am. Chem. Soc., 2011, 133, 1853 Search PubMed
;
(d) L. Hong, W. Sun, D. Yang, G. Li and R. Wang, Chem. Rev., 2016, 116, 4006 CrossRef CAS PubMed
.
- Selected examples using 2-benzothiazolimines in catalytic enantioselective reaction:
(a) M. Frías, A. C. Carrasco, A. Fraile and J. Alemán, Chem.–Eur. J., 2018, 24, 3117 CrossRef PubMed
;
(b) H.-X. He, W. Yang and D.-M. Du, Adv. Synth. Catal., 2013, 355, 1137 CrossRef CAS
;
(c) L. Li, B. Song, P. S. Bhadury, Y. Zhang, D. Hu and S. Yang, Eur. J. Org. Chem., 2011, 4743 CAS
.
- Addition of AcOH to improve catalyst turnover (see ref. 17) leads to substantial degradation..
-
(a) J. P. Reid and J. M. Goodman, Chem.–Eur. J., 2017, 23, 14248 CrossRef CAS PubMed
;
(b) J. P. Reid, L. Simón and J. M. Goodman, Acc. Chem. Res., 2016, 49, 1029 CrossRef CAS PubMed
;
(c) L. Simón and J. M. Goodman, J. Org. Chem., 2011, 76, 1775 CrossRef PubMed
.
- G. Fraenkel, J. Foos, C. C. Ho, Y. Liang, S. Q. A. Rizvi, H. Stucki and F. Steel, Tetrahedron Lett., 1978, 19, 327 CrossRef
.
Footnote |
† Electronic supplementary information (ESI) available. CCDC 1815484 and 1851627. For ESI and crystallographic data in CIF or other electronic format see DOI: 10.1039/c8sc05581e |
|
This journal is © The Royal Society of Chemistry 2019 |