DOI:
10.1039/C8SC04681F
(Edge Article)
Chem. Sci., 2019,
10, 2228-2235
Benzene construction via Pd-catalyzed cyclization of 2,7-alkadiynylic carbonates in the presence of alkynes†
Received
20th October 2018
, Accepted 18th December 2018
First published on 19th December 2018
Abstract
A palladium-catalyzed highly regio- and chemo-selective cyclization of 2,7-alkadiynylic carbonates with functionalized alkynes to construct 1,3-dihydroisobenzofuran and isoindoline derivatives under mild conditions has been developed. Functional groups such as alcohol, sulfonamide, and indoles could be well tolerated. After careful mechanistic studies, a mechanism involving oxidative addition and regioselectivity-defined double alkyne insertions has been proposed.
Introduction
Benzocyclopentane derivatives, especially those containing oxygen and nitrogen heterocycles, exist widely in natural products and biologically active molecules:1 the phthalan (1,3-dihydroisobenzofuran) and isoindoline skeletons are representative examples (Fig. 1),2 which are also important building blocks in organic synthesis.3 Common approaches for the construction of phthalan and isoindoline structures include: (1) [2 + 2 + 2] cycloaddition reactions of 1,6-diynes with alkynes;4–11 (2) tetradehydro-Diels–Alder reaction of eneynes and alkynes or hexadehydro-Diels–Alder reaction of 1,3,8-triynes and electrophiles;12 (3) domino reaction consisting of Heck couplings and consecutive 6π-electrocyclizations or Sonogashira couplings and sequenced Garratt–Braverman cyclization of alkenyl halides and 1,6-diynes.13
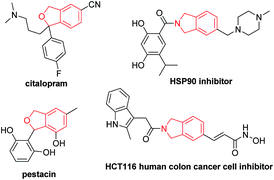 |
| Fig. 1 Some typical natural or bioactive phthalans and isoindolines compounds. | |
Among all these methods, transition metal-catalyzed [2 + 2 + 2] cyclization of 1,6-diynes and alkynes is the most straightforward one.4–11 However, there is an issue of regioselectivity when non-symmetric 1,6-diynes and non-symmetric alkynes were applied (Scheme 1, eqn (1)).4–11 Based on our previous explorations in the tandem reactions between 2,7-alkadiynyl carbonates 3 and various allenes to construct fused tricycles,14 we envisioned a new approach to benzocyclopentanes by applying Pd-catalyzed tandem reaction of 2,7-alkadiynyl carbonates 3 with functionalized terminal or non-terminal alkynes 4, in which the selectivity issue may be addressed by starting the cyclization from the oxidative addition of the propargylic carbonate unit to afford allenylpalladium intermediate A. Then the defined exo-insertion of the intramolecular alkyne and the subsequent regio-selective insertion of the intermolecular alkyne would produce benzylpalladium intermediate C, which underwent β-H elimination to give the final alkenyl benzene product (Scheme 1, eqn (2)). Here we wish to report the realization of such a concept.
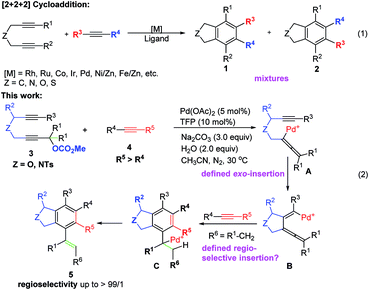 |
| Scheme 1 The transition metal-catalyzed [2 + 2 + 2] cyclotrimerization and cyclization of 2,7-alkadiynyl carbonate 3 in the presence of functionalized alkynes 4. | |
Results and discussion
Initially we conducted the reaction of 2,7-alkadiynylic carbonate 3a (0.3 mmol) and 4-methyl-N-(prop-2-yn-1-yl)benzenesulfonamide 4a (1.2 equiv.) under the catalysis of Pd(OAc)2 (5 mol%) and TFP (10 mol%) in the presence of K2CO3 (3.0 equiv.) and H2O (2.0 equiv.) at 70 °C in CH3CN. Interestingly, a pair of regioisomers 5aa and 6aa were observed in 81% and 3% yields, respectively, demonstrating a high regioselectivity. In addition, 2% yield of the cyclization product 7aa was obtained (entry 1, Table 1). Encouraged by this exciting observation, the influence of the critical reaction parameters was investigated. Firstly, considering of our previous works,14b,15 an appropriate amount of water may increase the solubility of K2CO3 in CH3CN, the effect of water was tested: the reaction failed to give better results when more water or no water were added (entries 2 and 3, Table 1). After screening a series of mono-phosphine ligand such as PPh3, LB-Phos·HBF4,16 and Gorlos-Phos·HBF4,17 it was found that TFP was still the best (entries 4–6, Table 1). The effect of base was also investigated: the reactions using NaOH or NEt3 as the base produced 5aa in lower yields with a poorer selectivity (entries 7 and 8, Table 1). Na2CO3 was slightly better than K2CO3, resulting in 82% yield of 5aa (entry 9, Table 1).
Table 1 The effect of water, ligand, and basea
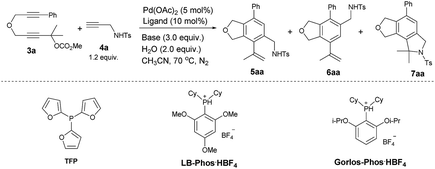
|
Entry |
Ligand |
Base |
Time (h) |
Yield of 5aa/6aa/7aab (%) |
Recovery of 3ab (%) |
Reaction condition: 3a (0.3 mmol), 4a (1.2 equiv.), Pd(OAc)2 (5 mol%), ligand (10 mol%), base (3.0 equiv.), and H2O (2.0 equiv.) in CH3CN (3.0 mL) at 70 °C unless otherwise noted.
Determined by the 1H NMR analysis of the crude product using mesitylene as the internal standard.
H2O (4.0 equiv.) were added.
No H2O was added.
|
1 |
TFP |
K2CO3 |
2 |
81/3/2 |
0 |
2c |
TFP |
K2CO3 |
2 |
76/3/3 |
0 |
3d |
TFP |
K2CO3 |
2 |
70/4/3 |
0 |
4 |
PPh3 |
K2CO3 |
26 |
37/5/5 |
14 |
5 |
LB-Phos·HBF4 |
K2CO3 |
24 |
19/3/2 |
43 |
6 |
Gorlos-Phos·HBF4 |
K2CO3 |
24 |
31/4/2 |
27 |
7 |
TFP |
NaOH |
2 |
64/5/4 |
0 |
8 |
TFP |
Et3N |
2 |
77/4/2 |
0 |
9
|
TFP
|
Na
2
CO
3
|
2
|
82/3/2
|
0
|
Further solvent screening showed that the reactions in dioxane, DMSO, DMF, or DCE all delivered poorer results than those in CH3CN (entries 1–4, Table 2). It is worth mentioning that the efficiency, yield, and selectivity could be kept at the same level when the reaction was conducted at a lower temperature of 30 °C (entries 5–7, Table 2). The reaction at 10 °C is sluggish (entry 8, Table 2). Based on these studies, the optimal mild conditions have been defined as follows: Pd(OAc)2 (5 mol%), TFP (10 mol%), Na2CO3 (3.0 equiv.), and H2O (2.0 equiv.) in CH3CN at 30 °C.
Table 2 The effect of solvent and temperaturea

|
Entry |
Solvent |
Temp. (°C) |
Time (h) |
Yield of 5aa/6aa/7aab (%) |
Recovery of 3ab (%) |
Reaction condition: 3a (0.3 mmol), 4a (1.2 equiv.), Pd(OAc)2 (5 mol%), TFP (10 mol%), Na2CO3 (3.0 equiv.), and H2O (2.0 equiv.) in solvent (3.0 mL).
Determined by the 1H NMR analysis of the crude product using mesitylene as the internal standard.
|
1 |
Dioxane |
70 |
2 |
72/6/4 |
0 |
2 |
DMSO |
70 |
26 |
48/3/3 |
25 |
3 |
DMF |
70 |
2 |
76/3/5 |
0 |
4 |
DCE |
70 |
6 |
49/7/3 |
0 |
5 |
CH3CN |
80 |
2 |
80/3/3 |
0 |
6 |
CH3CN |
60 |
2 |
80/3/3 |
0 |
7
|
CH
3
CN
|
30
|
2
|
82/3/2
|
0
|
8 |
CH3CN |
10 |
26 |
68/2/4 |
3 |
The scope of the terminal alkynes was examined by using methyl (2-methyl-5-((3-phenylprop-2-yn-1-yl)oxy)pent-3-yn-2-yl) carbonate (3a) as the model substrate on a 1 mmol scale (Table 3). The reaction of 3a with propargyl tosylamide 4a afforded 5aa/6aa in 78% yield with a selectivity of 97/3. When propargyl alcohol 4b was used, 5ab/6ab was obtained in 72% yield with the same selectivity. In light of the fact that the indole skeletons are very common in natural products and biologically active molecules,18 it is interesting to note that the reactions with a series of N-propargyl indole derivatives (4c–4f) also worked, affording the phthalan derivatives bearing an indole ring 5ac/6ac–5af/6af in 66–69% yields with a ratio of 95/5 ∼> 99/1. Synthetically useful groups such as methyl, formyl, bromo could be introduced at different positions in the indole ring. The structure of product was unambiguously established by the X-ray single crystal diffraction analysis of 5ae (Fig. 2).19
Table 3 Scope of terminal alkynes 4.a,b,c
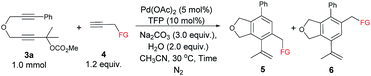
|
Reaction conditions: 3a (1.0 mmol), 4 (1.2 equiv.), Pd(OAc)2 (5 mol%), TFP (10 mol%), Na2CO3 (3.0 equiv.), and H2O (2.0 equiv.) in CH3CN (10 mL) at 30 °C.
Combined isolated yield of 5 and 6.
The ratio of 5 and 6 was determined by 1H NMR analysis of the isolated product.
|
|
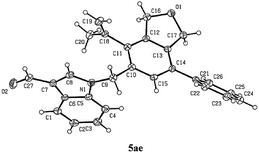 |
| Fig. 2 ORTEP representation of 5ae. | |
Next, the reactivity of various oxygen- or nitrogen-tethered 2,7-alkadiynylic carbonates was examined with different functionalized terminal alkynes (Table 4). In addition to being methyl groups, the two R1 groups could be a five- or six-membered ring. The corresponding products 5ba/6ba and 5ja/6ja were isolated in 79% yield with a selectivity of 98/2 and 53% yield with a selectivity of 90/10, respectively. The substrates with the Ar group bearing either electron-rich or electron-deficient groups at the 8-position could also be applied, producing the expected products 5cb/6cb–5fg/6fg in 59%–85% yields with the ratio of 93/7–97/3. This method could be extended to 8-(3′-thienyl), 8-nBu, 8-TMS and 6-propyl substituted 2,7-alkadiynylic carbonates to afford 5ga/6ga–5ha/6ha in moderate to good yields with the selectivity of 96/4 to >99/1. Nitrogen-tethered 2,7-alkadiynylic carbonate 3i also worked and the isoindoline derivatives 5ia/6ia were obtained in 67% yield with a selectivity of 98/2.
Table 4 The reaction of 2,7-alkadiynylic carbonates 3 with functionalized terminal alkynes 4a,b,c
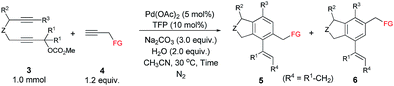
|
Reaction conditions: 3 (1.0 mmol), 4 (1.2 equiv.), Pd(OAc)2 (5 mol%), TFP (10 mol%), Na2CO3 (3.0 equiv.), H2O (2.0 equiv.) in CH3CN (10 mL) at 30 °C.
Combined yield of 5 and 6.
The ratio of 5 and 6 was determined by 1H NMR analysis of the isolated product.
The reaction was conducted at 50 °C and 11% of 3j was recovered.
0.5 mmol scale.
|
|
The reaction of 3a with 4a could be easily conducted on a gram-scale synthesis, resulting in the isolation of 1.42 g (85%) of 5aa/6aa with a selectivity of 97/3 together with a 1% yield of 7aa (eqn (3)).
| 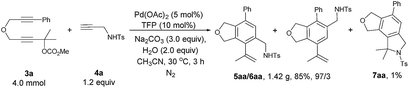 | (3) |
Some control experiments were conducted to obtain further information concerning the regioselectivity (Scheme 2). When phenyl or n-butyl substituted terminal alkyne 4h or 4i was used, the corresponding bicyclic product could still be formed with a decent regioselectivity, albeit in a much lower yield (Scheme 2a). Furthermore, the reaction of 3a with internal propargylic alcohol 4j could give 22% yield of the product 5aj exclusively (Scheme 2b). X-ray single crystal diffraction analysis of 5aj showed that the regioselectivity was reversed-the CH2OH group was at the ortho-position of the phenyl group from 3a (Fig. 3).20 The reaction of 3a with 1-phenylpropyne 4k also gave 5ak/6ak in 11% yield with the same regioselectivity as 4j, indicating that the hydroxy group should have nothing to do with the regioselectivity (Scheme 2b). The structure of 5ak was also confirmed by X-ray single crystal diffraction analysis (Fig. 3).21 However, the reaction did not work with 1,2-diphenylethyne 4l (Scheme 2b). Thus, the regioselectivity obviously depends on the size of two groups of the alkyne: the larger group of the alkyne was more likely to stay away from the phenyl group originating from 3a. The failure of the reaction of 3a-OH with 4a under the standard conditions indicated that the reaction may not proceed the [2 + 2 + 2] cycloaddition of the diyne unit in 3a with the C–C triple bond in 4a (Scheme 2c).
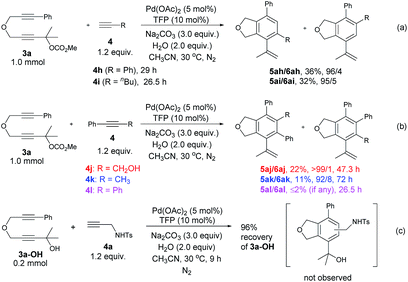 |
| Scheme 2 Control experiments. | |
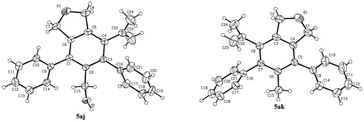 |
| Fig. 3 ORTEP representations of 5aj and 5ak. | |
Based on these experimental results, a possible mechanism is shown in Scheme 3 by taking the reaction of 3a and 4a as an example: oxidative addition of 3a with the catalytically active species Pd(0) would give the allenylpalladium intermediate IN-1,22 which undergoes intramolecular exo-mode insertion of the C–C triple bond to generate the alkenylpalladium species IN-2.23 The species IN-2 would undergo intermolecular carbopalladation of the C
C bond of 4a to generate a new alkenylpalladium intermediate IN-3 (path a) or IN-5 (path b). Then intramolecular carbopalladation of allene would form a new benzylpalladium intermediate IN-4 or IN-6. In the presence of a base, the key intermediate IN-4 could undergo the intramolecular nucleophilic attack or β-H elimination forming tricyclic product 7aa or the phthalan derivative 5aa and regenerating the catalytically active Pd(0). As a comparison, the key intermediate IN-6 could only go through the β-H elimination to deliver the phthalan derivative isomer 6aa. Obviously, there is a strong steric interaction between the phenyl group and –CH2NHTs moiety in IN-5, which make the reaction more likely to go through path a.
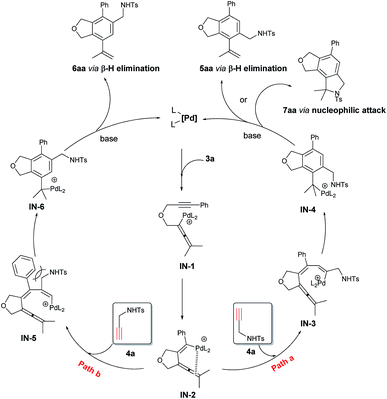 |
| Scheme 3 The proposed mechanistic pathways. | |
An alternative mechanism involving a Sonogashira coupling (path c) of IN-2 has been presented in Scheme 4. The resulted intermediate IN-8 could go through the Garratt–Braverman cyclization to deliver 5aa.24
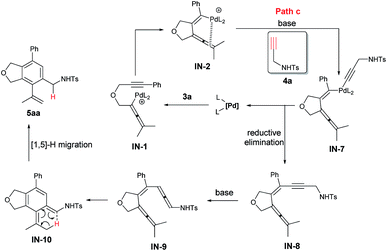 |
| Scheme 4 An alternative mechanism involving a Sonogashira coupling of IN-2. | |
There should be a possibility of [1,5]-H migration process in path c. Thus, the reaction of 3a-d6 and 4a was conducted and a mixture of 5aa-d5 and 6aa-d5 with a ratio 98/2 with no deuteration at the α-position of the NHTs group was afforded in 85% yield (eqn (4)), indicating that there is no H-migration process, thus, path c is not viable for the formation of 5aa.
|  | (4) |
We also performed some deuterium labelling experiments for the investigation of the D–H exchange of the terminal alkyne (Scheme 5). To our surprise, the reaction of 3a with D-4c under the standard conditions afforded 5ac/6ac in 84% yield with a selectivity of 95/5 without any deuterium incorporation (eqn (5)). We conjectured that the deuterium atom may be easily exchanged with the hydrogen atom under the aqueous environment. Thus, H2O (2.0 equiv.) was replaced with D2O (2.0 equiv.), which led to the formation of 25% deuterium incorporation in D-5ac (eqn (6)). Interestingly, the regioselectivity dropped from 95
:
5 to 81
:
19 (compare eqn (5) with eqn (6)), which might be explained by the steric effect of D vs. H at 30 °C.25 Of course, further attention is obviously needed. The D–H exchange was also proven by the reaction of D-4c with H2O or the reaction of 4c with 3a in the presence of D2O (eqn (7) and (8)). These experimental facts further support the mechanism shown in Scheme 3.
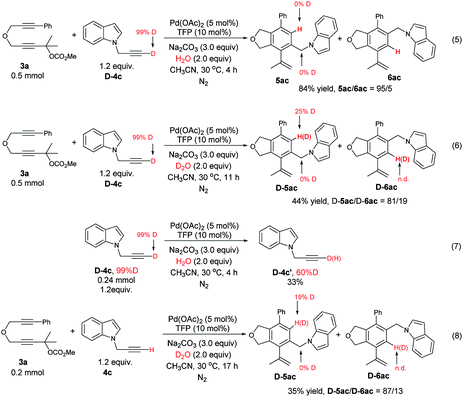 |
| Scheme 5 Deuterium labeling experiments: investigating of the D–H exchange of the terminal alkyne (n.d. = not able to be determined by 1H NMR analysis). | |
In order to show the potential of the products, some synthetic applications have been conducted (Scheme 6). The bicyclic product 5aa could be transferred to tricyclic isobenzofuro[5,4-c]azepine derivative 8aa in 57% yield after an allylation-RCM process.26 The tetracyclic product 9ba containing a spirocycle skeleton can be easily obtained through an electrophilic cyclization with NIS (1.5 equiv.).27 The Fe(NO3)3·9H2O–TEMPO–NaCl–catalyzed oxidation of 5ab proceeded smoothly to give the aryl aldehyde 10ab in 49% yield.28
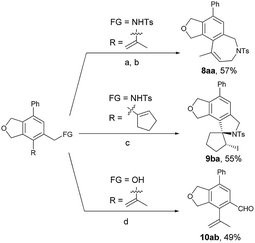 |
| Scheme 6 Synthetic applications. Reaction condition: (a) allylbromide (2.0 equiv.), K2CO3 (4.0 equiv.), CH3CN, refluxed (85 °C), 4 h; (b) Grubbs' II catalyst (10 mol%), toluene, 80 °C, 26 h; (c) NIS (1.5 equiv.), CH3CN/H2O = 15/1, rt, 27 h. (d) Fe(NO3)3·9H2O (10 mol%), TEMPO (10 mol%), NaCl (5 mol%), CH2Cl2, rt, 17.5 h. | |
Conclusions
In summary, we have developed a highly regio- and chemo-selective annulation of 2,7-alkadiynylic carbonates in the presence of functionalized alkynes to construct 1,3-dihydroisobenzofuran and isoindoline derivatives under mild conditions. Functional groups such as sulfonamide, alcohol, and indoles could be kept untouched, which provides a chance for many further transformations to more complicated polycycles. Further studies in this area are being pursued in our laboratory.
Conflicts of interest
There are no conflicts to declare.
Acknowledgements
Financial supports from the National Natural Science Foundation of China (21690063) and National Basic Research Program of China (2015CB856600) are greatly appreciated. We thank Mr Yangguangyan Zheng in this group for reproducing the preparation of 5ae, 5cb, and 5de. Shengming Ma is a Qiu Shi Adjunct Professor at Zhejiang University.
Notes and references
-
(a) S. O. Simonetti, E. L. Larghi, A. B. J. Bracca and T. S. Kaufman, Nat. Prod. Rep., 2013, 30, 941 RSC;
(b) R. Karmakar, P. Pahari and D. Mal, Chem. Rev., 2014, 114, 6213 CrossRef CAS PubMed;
(c) W. Jiaang, Y. Chen, T. Hsu, S. Wu, C. Chien, C. Chang, S. Chang, S. Lee and X. Chen, Bioorg. Med. Chem. Lett., 2005, 15, 687 CrossRef CAS PubMed;
(d) S. Van Goethem, P. Van der Veken, V. Dubois, A. Soroka, A.-M. Lambeir, X. Chen, A. Haemers, S. Scharpé, I. De Meester and K. Augustyns, Bioorg. Med. Chem. Lett., 2008, 18, 4159 CrossRef CAS PubMed;
(e) E. Pinard, D. Alberati, M. Bender, E. Borroni, V. Brom, S. Burner, H. Fischer, D. Hainzl, R. Halm, N. Hauser, S. Jolidon, J. Lengyel, H. Marty, T. Meyer, J. Moreau, R. Mory, R. Narquizian, R. D. Norcross, P. Schmid, R. Wermuth and D. Zimmerli, Bioorg. Med. Chem. Lett., 2010, 20, 6960 CrossRef CAS PubMed;
(f) S. Tso, M. Lou, C. Wu, W. Gui, J. L. Chuang, L. K. Morlock, N. S. Williams, R. M. Wynn, X. Qi and D. T. Chuang, J. Med. Chem., 2017, 60, 1142 CrossRef CAS PubMed;
(g) T. Mancilla-Percino, C. R. Trejo-Muñoz, J. A. Díaz-Gandarilla, P. Talamás-Rohana, J. E. Guzmán Ramírez, J. Cervantes and F. Ortíz, Arch. Pharm. Chem. Life Sci., 2016, 349, 175 CrossRef CAS PubMed;
(h) S. Tso, X. Qi, W. Gui, C. Wu, J. L. Chuang, I. Wernstedt-Asterholm, L. K. Morlock, K. R. Owens, P. E. Scherer, N. S. Williams, U. K. Tambar, R. M. Wynn and D. T. Chuang, J. Biol. Chem., 2014, 289, 4432 CrossRef CAS PubMed.
-
(a) B. G. Pollock, Expert Opin. Pharmacother., 2001, 2, 681 CrossRef CAS PubMed;
(b) J. K. Harper, A. M. Arif, E. J. Ford, G. A. Strobel, J. A. Porco Jr, D. P. Tomer, K. L. Oneill, E. M. Heider and D. M. Grant, Tetrahedron, 2003, 59, 2471 CrossRef CAS;
(c) J. Ren, J. Li, Y. Wang, W. Chen, A. Shen, H. Liu, D. Chen, D. Cao, Y. Li, N. Zhang, Y. Xu, M. Geng, J. He, B. Xiong and J. Shen, Bioorg. Med. Chem. Lett., 2014, 24, 2525 CrossRef CAS PubMed;
(d) M. Shultz, J. Fan, C. Chen, Y. S. Cho, N. Davis, S. Bickford, K. Buteau, X. Cao, M. Holmqvist, M. Hsu, L. Jiang, G. Liu, Q. Lu, C. Patel, J. R. Suresh, M. Selvaraj, L. Urban, P. Wang, Y. Yan-Neale, L. Whitehead, H. Zhang, L. Zhou and P. Atadja, Bioorg. Med. Chem. Lett., 2011, 21, 4909 CrossRef CAS PubMed.
-
(a) J. Almena, F. Foubelo and M. Yus, Tetrahedron, 1996, 52, 8545 CrossRef CAS;
(b) J. Almena, F. Foubelo and M. Yus, Tetrahedron, 1995, 51, 3351 CrossRef CAS;
(c) U. Azzena, S. Demartis, M. G. Fiori, G. Melloni and L. Pisano, Tetrahedron Lett., 1995, 36, 8123 CrossRef CAS;
(d) U. Azzena, S. Demartis and G. Melloni, J. Org. Chem., 1996, 61, 4913 CrossRef CAS;
(e) D. García, F. Foubelo and M. Yus, Tetrahedron, 2008, 64, 4275 CrossRef;
(f) D. Y. Li, X. S. Shang, G. R. Chen and P. N. Liu, Org. Lett., 2013, 15, 3848 CrossRef CAS PubMed;
(g) H. X. Siyang, X. R. Wu, H. L. Liu, X. Y. Wu and P. N. Liu, J. Org. Chem., 2014, 79, 1505 CrossRef CAS PubMed;
(h) J. G. Sośnicki, Monatsh. Chem., 2000, 131, 475 CrossRef;
(i) T. Ohmura, A. Kijima and M. Suginome, Org. Lett., 2011, 13, 1238 CrossRef CAS PubMed;
(j) P. Galletti, F. Funiciello, R. Soldati and D. Giacomini, Adv. Synth. Catal., 2015, 357, 1840 CrossRef CAS.
- For selected reviews, see:
(a) P. R. Chopade and J. Louie, Adv. Synth. Catal., 2006, 348, 2307 CrossRef CAS;
(b) K. Tanaka, Bull. Chem. Soc. Jpn., 2018, 91, 187 CrossRef CAS;
(c) S. Saito and Y. Yamamoto, Chem. Rev., 2000, 100, 2901 CrossRef CAS PubMed;
(d) J. A. Varela and C. Saá, Chem. Rev., 2003, 103, 3787 CrossRef CAS PubMed;
(e) B. Heller and M. Hapke, Chem. Soc. Rev., 2007, 36, 1085 RSC;
(f) G. Domínguez and J. Pérez-Castells, Chem. Soc. Rev., 2011, 40, 3430 RSC;
(g) N. Weding and M. Hapke, Chem. Soc. Rev., 2011, 40, 4525 RSC;
(h) S. Kotha, E. Brahmachary and K. Lahiri, Eur. J. Org. Chem., 2005, 4741 CrossRef CAS;
(i) S. Okamoto, Heterocycles, 2012, 85, 1579 CrossRef CAS;
(j) T. Shibata and K. Tsuchikama, Org. Biomol. Chem., 2008, 6, 1317 RSC;
(k) M. Babazadeh, S. Soleimani-Amiri, E. Vessally, A. Hosseiniand and L. Edjlali, RSC Adv., 2017, 7, 43716 RSC;
(l) S. Okamoto and Y. Sugiyama, Synlett, 2013, 24, 1044 CrossRef CAS;
(m) Y. Shibata and K. Tanaka, Synthesis, 2012, 44, 323 CrossRef CAS;
(n) Y. Yamamoto, Tetrahedron Lett., 2017, 58, 3787 CrossRef CAS.
- For selected reports on Fe-catalyzed cyclization reactions, see:
(a) D. Brenna, M. Villa, T. N. Gieshoff, F. Fischer, M. Hapke and A. J. Wangelin, Angew. Chem., Int. Ed., 2017, 56, 8451 CrossRef CAS PubMed;
(b) N. Saino, D. Kogure and S. Okamoto, Org. Lett., 2005, 7, 3065 CrossRef CAS PubMed;
(c) D. Bhatt, H. Chowdhury and A. Goswami, Org. Lett., 2017, 19, 3350 CrossRef CAS PubMed;
(d) N. Saino, D. Kogure, K. Kase and S. Okamoto, J. Organomet. Chem., 2006, 691, 3129 CrossRef CAS.
- For selected reports on Ru-catalyzed cyclization reactions, see:
(a) R. W. Foster, C. J. Tame, H. C. Hailes and T. D. Sheppard, Adv. Synth. Catal., 2013, 355, 2353 CrossRef CAS PubMed;
(b) Y. Yamamoto, R. Ogawa and K. Itoh, Chem. Commun., 2000, 549 RSC;
(c) B. Witulski, T. Stengel and J. M. Fernández-Hernández, Chem. Commun., 2000, 1965 RSC;
(d) E. Kang, C. Kang, S. Yang, E. Oks and T. Choi, Macromolecules, 2016, 49, 6240 CrossRef CAS;
(e) Y. Yamamoto, K. Kinpara, T. Saigoku, H. Nishiyama and K. Itoh, Org. Biomol. Chem., 2004, 2, 1287 RSC;
(f) R. Karmakar, K. Wang, S. Y. Yun, P. Mamidipalli and D. Lee, Org. Biomol. Chem., 2016, 14, 4782 RSC;
(g) F. Ye, M. Haddad, V. Michelet and V. Ratovelomanana-Vidal, Org. Lett., 2016, 18, 5612 CrossRef CAS PubMed.
- For selected reports on Co-catalyzed cyclization reactions, see:
(a) P. Jungk, F. Fischer and M. Hapke, ACS Catal., 2016, 6, 3025 CrossRef CAS;
(b) A. Geny, N. Agenet, L. Iannazzo, M. Malacria, C. Aubert and V. Gandon, Angew. Chem., Int. Ed., 2009, 48, 1810 CrossRef CAS PubMed;
(c) T. Sugihara, A. Wakabayashi, Y. Nagai, H. Takao, H. Imagawa and M. Nishizawa, Chem. Commun., 2002, 576 RSC;
(d) M. Wu, M. Shanmugasundaram and C. Cheng, Chem. Commun., 2003, 718 RSC;
(e) K. E. Ruhl and T. Rovis, J. Am. Chem. Soc., 2016, 138, 15527 CrossRef CAS PubMed;
(f) P. Jungk, F. Fischer, I. Thiel and M. Hapke, J. Org. Chem., 2015, 80, 9781 CrossRef CAS PubMed.
- For selected reports on Ir-catalyzed cyclization reactions, see:
(a) A. Auvinet, M. Ez-Zoubir, S. Bompard, M. R. Vitale, J. A. Brown, V. Michelet and V. Ratovelomanana-Vidal, ChemCatChem, 2013, 5, 2389 CrossRef CAS;
(b) A. Auvinet, M. Ez-Zoubir, M. R. Vitale, J. A. Brown, V. Michelet and V. Ratovelomanana-Vidal, ChemSusChem, 2012, 5, 1888 CrossRef CAS PubMed.
- For selected reports on Rh-catalyzed cyclization reactions, see:
(a) B. Witulski and T. Stengel, Angew. Chem., Int. Ed., 1999, 38, 2426 CrossRef CAS;
(b) F. Ye, M. Haddad, V. Ratovelomanana-Vidal and V. Michelet, Catal. Commun., 2018, 107, 78 CrossRef CAS;
(c) Y. Tahara, S. Obinata, K. S. Kanyiva, T. Shibata, A. Mándi, T. Taniguchi and K. Monde, Eur. J. Org. Chem., 2016, 1405 CrossRef CAS;
(d) F. E. McDonald, H. Y. H. Zhu and C. R. Holmquist, J. Am. Chem. Soc., 1995, 117, 6605 CrossRef CAS;
(e) J. Clayden and W. J. Moran, Org. Biomol. Chem., 2007, 5, 1028 RSC;
(f) R. Grigg, R. Scott and P. Stevenson, Tetrahedron Lett., 1982, 23, 2691 CrossRef CAS.
- For selected reports on Ni-catalyzed cyclization reactions, see:
(a) Y. Sato, T. Nishimata and M. Mori, J. Org. Chem., 1994, 59, 6133 CrossRef CAS;
(b) S. Ikeda, H. Watanabe and Y. Sato, J. Org. Chem., 1998, 63, 7026 CrossRef CAS;
(c) P. Turek, P. Novák, R. Pohl, M. Hocek and M. Kotora, J. Org. Chem., 2006, 71, 8978 CrossRef CAS PubMed;
(d) A. Jeevanandam, R. P. Korivi, I. Huang and C. Cheng, Org. Lett., 2002, 4, 807 CrossRef CAS PubMed.
- For selected reports on Pd-catalyzed cyclization reactions, see:
(a) Y. Yamamoto, A. Nagata, H. Nagata, Y. Ando, Y. Arikawa, K. Tatsumi and K. Itoh, Chem. - Eur. J., 2003, 9, 2469 CrossRef CAS PubMed;
(b) P. Zhou, M. Zheng, H. Jiang, X. Li and C. Qi, J. Org. Chem., 2011, 76, 4759 CrossRef CAS PubMed;
(c) E. Negishi, L. S. Harring, Z. Owczarczyk, M. M. Mohamud and M. Ay, Tetrahedron Lett., 1992, 33, 3253 CrossRef CAS;
(d) Y. Yamamoto, A. Nagata and K. Itoh, Tetrahedron Lett., 1999, 40, 5035 CrossRef CAS.
-
(a) P. Wessig and G. Müller, Chem. Rev., 2008, 108, 2051 CrossRef CAS PubMed;
(b) T. R. Hoye, B. Baire, D. W. Niu, P. H. Willoughby and B. P. Woods, Nature, 2012, 490, 208 CrossRef CAS PubMed;
(c) N. J. Kramer, T. T. Hoang and G. B. Dudley, Org. Lett., 2017, 19, 4636 CrossRef CAS PubMed;
(d) L. Lu, X.-Y. Liu, X.-Z. Shu, K. Yang, K.-G. Ji and Y.-M. Liang, J. Org. Chem., 2009, 74, 474 CrossRef CAS PubMed;
(e) B. Martín-Matute, D. J. Cárdenas and A. M. Echavarren, Angew. Chem., Int. Ed., 2001, 40, 4754 CrossRef;
(f) A. S. K. Hashmi, M. Wölfle, F. Ata, M. Hamzic, R. Salathé and W. Frey, Adv. Synth. Catal., 2006, 348, 2501 CrossRef CAS;
(g) D. Niu, T. Wang, B. P. Woods and T. R. Hoye, Org. Lett., 2014, 16, 254 CrossRef CAS PubMed;
(h) J. Chen, V. Palani and T. R. Hoye, J. Am. Chem. Soc., 2016, 138, 4318 CrossRef CAS PubMed;
(i) A. S. K. Hashmi, T. M. Frost and J. W. Bats, J. Am. Chem. Soc., 2000, 122, 11553 CrossRef CAS;
(j) A. S. K. Hashmi, M. Ghanbari, M. Rudolph and F. Rominger, Chem. - Eur. J., 2012, 18, 8113 CrossRef CAS PubMed;
(k) A. S. K. Hashmi, J. P. Weyrauch, E. Kurpejović, T. M. Frost, B. Miehlich, W. Frey and J. W. Bats, Chem. - Eur. J., 2006, 12, 5806 CrossRef CAS PubMed.
-
(a) For a selected review, see: A. D. Meijere, P. V. Zezschwitz and S. Bräse, Acc. Chem. Res., 2005, 38, 413 CrossRef PubMedFor selected reports on domino reactions of alkenyl or aryl halides with two acetylenic fragments, see:
(b) R. Shen, X. Huang and L. Chen, Adv. Synth. Catal., 2008, 350, 2865 CrossRef CAS;
(c) S. Zhu, J. Cao, L. Wu and X. Huang, J. Org. Chem., 2012, 77, 10409 CrossRef CAS PubMed;
(d) S. Torii, H. Okumoto and A. Nishimura, Tetrahedron Lett., 1991, 32, 4167 CrossRef CAS;
(e) P. J. Parsons, M. Stefinovic, P. Willis and F. Meyer, Synlett, 1992, 864 CrossRef CAS;
(f) S. S. Goh, G. Chaubet, B. Gockel, M. A. Cordonnier, H. Baars, A. W. Phillips and E. A. Anderson, Angew. Chem., Int. Ed., 2015, 54, 12618 CrossRef CAS PubMed;
(g) M. Leibeling, D. Koester, M. Pawliczek, S. Schild and D. Werz, Nat. Chem. Biol., 2010, 6, 199 CrossRef CAS PubMedFor selected reports on domino reactions of alkenyl or aryl halides with two alkynes, see:
(h) H. Kinoshita, H. Takahashi and K. Miura, Org. Lett., 2013, 15, 2962 CrossRef CAS PubMed;
(i) S. Kawasaki, T. Satoh, M. Miura and M. Nomura, J. Org. Chem., 2003, 68, 6836 CrossRef CAS PubMed.
-
(a) X. Lian and S. Ma, Angew. Chem., Int. Ed., 2008, 47, 8255 CrossRef CAS PubMed;
(b) X. Huang, W. Wu, S. Song, C. Fu and S. Ma, Adv. Synth. Catal., 2016, 358, 2791 CrossRef CAS.
- Q. Li, C. Fu and S. Ma, Angew. Chem., Int. Ed., 2012, 51, 11783 CrossRef CAS PubMed.
- B. Lü, C. Fu and S. Ma, Tetrahedron Lett., 2010, 51, 1284 CrossRef.
- B. Lü, P. Li, C. Fu, L. Xue, Z. Lin and S. Ma, Adv. Synth. Catal., 2011, 353, 100 CrossRef.
-
(a) T. Fischer, A. Najjar, F. Totzke, C. Schächtele, W. Sippl, C. Ritter and A. Hilgeroth, J. Enzyme Inhib. Med. Chem., 2017, 33, 1 Search PubMed;
(b) W. M. Eldehna, D. H. EL-Naggar, A. R. Hamed, H. S. Ibrahim, H. A. Ghabbour and H. A. Abdel-Azizb, J. Enzyme Inhib. Med. Chem., 2018, 33, 309 CrossRef CAS PubMed;
(c) W. M. Eldehna, R. I. Al-Wabli, M. S. Almutairi, A. B. Keeton, G. A. Piazza, H. A. Abdel-Aziz and M. I. Attia, J. Enzyme Inhib. Med. Chem., 2018, 33, 867 CrossRef CAS PubMed;
(d) F. Wang, Y. Fang, T. Zhu, M. Zhang, A. Lin, Q. Gu and W. Zhu, Tetrahedron, 2008, 64, 7986 CrossRef CAS.
- Crystal data for compound 5ae: C27H23NO2; MW = 393.46; orthorhombic space group P b c a; final R indices [I>2\s(I)], R1 = 0.0717, wR2 = 0.1322, R indices (all data) R1 = 0.0937, wR2 = 0.1440; a = 37.682(4) Å, b = 6.9971(4) Å, c = 15.2009(9) Å; α = 90, β = 90, γ = 90; V= 4007.9(5) Å3; T = 150.0 K; Z = 8; reflections collected/unique 23799/3672 (Rint = 0.0793); number of observations [>2\s(I)] 2837, parameters: 272. CCDC 1849157 contains the supplementary crystallographic data for this paper.†.
- Crystal data for compound 5aj: C24H22O2; MW=342.41; monoclinic space group P 1 21/n 1; final R indices [I > 2\s(I)], R1 = 0.0602, wR2 = 0.1251, R indices (all data) R1 = 0.0921, wR2 = 0.1457; a = 6.8864(5) Å, b = 33.272(3) Å, c = 8.5011(8) Å; α = 90, β = 104.932(8), γ = 90; V= 1882.0(3) Å3; T = 293.0 K; Z = 4; reflections collected/unique 7378/3441 (Rint = 0.0345); number of observations [>2\s(I)] 2370, parameters: 237. CCDC 1849158 contains the supplementary crystallographic data for this paper.†.
- Crystal data for compound 5ak: C24H22O1; MW=326.41; triclinic space group P -1; final R indices [I > 2\s(I)], R1 = 0.0624, wR2 = 0.1570, R indices (all data) R1 = 0.1055, wR2 = 0.1984; a = 8.4646(7) Å, b = 10.2366(11) Å, c = 11.8274(12) Å; α = 77.022(9), β = 69.293(8), γ = 74.924(8); V= 915.61(16) Å3; T = 296.0 K; Z = 2; reflections collected/unique 5997/3358 (Rint = 0.0336); number of observations [>2\s(I)] 2007, parameters: 228. CCDC 1855724 contains the supplementary crystallographic data for this paper.†.
- For slected reviews, see:
(a) J. Tsuji and T. Mandai, Angew. Chem., Int. Ed. Engl., 1995, 34, 2589 CrossRef CAS;
(b) L. Guo, X. Duan and Y. Liang, Acc. Chem. Res., 2011, 44, 111 CrossRef CAS PubMed;
(c) J. Ye and S. Ma, Acc. Chem. Res., 2014, 47, 989 CrossRef CAS PubMed.
-
(a) R. Grigg, R. Rasul and V. Savic, Tetrahedron Lett., 1997, 38, 1825 CrossRef CAS;
(b) F. Wang, X. Tong, J. Cheng and Z. Zhang, Chem. - Eur. J., 2004, 10, 5338 CrossRef CAS PubMed;
(c) S. Song, C. Fu, X. Huang and S. Ma, Adv. Synth. Catal., 2018, 360, 1019 CrossRef CAS.
- J. Das, S. S. Bag and A. Basak, J. Org. Chem., 2016, 81, 4623 CrossRef CAS PubMed.
- J. D. Dunitz and R. M. Ibberson, Angew. Chem., Int. Ed., 2008, 47, 4208 CrossRef CAS PubMed.
- B. Bradshaw, P. Evans, J. Fletcher, A. T. L. Lee, P. G. Mwashimba, D. Oehlrich, E. J. Thomas, R. H. Davies, B. C. P. Allen, K. J. Broadley, A. Hamrounic and C. Escargueil, Org. Biomol. Chem., 2008, 6, 2138 RSC.
- Y. Morino, I. Hidaka, Y. Oderaotoshi, M. Komatsu and S. Minakata, Tetrahedron, 2006, 62, 12247 CrossRef CAS.
- S. Ma, J. Liu, S. Li, B. Chen, J. Cheng, J. Kuang, Y. Liu, B. Wan, Y. Wang, J. Ye, Q. Yu, W. Yuan and S. Yu, Adv. Synth. Catal., 2011, 353, 1005 CrossRef CAS.
Footnote |
† Electronic supplementary information (ESI) available: Experimental section, characterization of all the compounds, and copies of 1H NMR and 13C NMR spectra. CCDC 1849157, 1849158 and 1855724. For ESI and crystallographic data in CIF or other electronic format see DOI: 10.1039/c8sc04681f |
|
This journal is © The Royal Society of Chemistry 2019 |
Click here to see how this site uses Cookies. View our privacy policy here.