DOI:
10.1039/C8SC03837F
(Edge Article)
Chem. Sci., 2019,
10, 1070-1074
Brønsted acid-catalyzed aromatic annulation of alkoxyallenes with naphthols: a reaction sequence to larger π-conjugated naphthopyrans with aggregation-induced emission characters†
Received
28th August 2018
, Accepted 1st November 2018
First published on 2nd November 2018
Abstract
A practical and readily scalable reaction sequence was developed for the straightforward synthesis of a new family of larger π-conjugated naphthopyrans by a Brønsted acid-catalyzed aromatic annulation of alkoxyallenes with inert naphthols. The cascade pathway involves allylation/cyclization/debenzyloxylation/isomerization/dehydration. The new class of solid state diphenylmethylene substituted naphthopyrans are fluorescent emissive and proved to have aggregation-induced emission (AIE) behavior.
Naphthopyran represents a unique unit in a wide family of natural products;1 it has been widely studied in photochromic chromenes (Scheme 1, A) and applied to commercially available photochromic lenses.2 For examples, the natural product B was isolated from the roots of Pentas bussei, a plant in Kenya that was used as a remedy for gonorrhea, syphilis, and dysentery by decoction of the roots. Conocurvone C, an extract from the endemic Australian shrub Conospermum sp. (Proteaceae), can inhibited the cytopathic effects of HIV-1 infection. Despite these important synthetic advances in strong acid-promoted cyclizations and transition metal-catalyzed annulations, the available methods are extensively limited to provide 3,3-disubstituted naphthopyrans (such as A and B).3 In principle, the extension of the conjugated π-system might endow materials with extraordinary properties in luminescence,4 organic electronics,5 and photovoltaic devices.6 Therefore, the development of efficient methods for the discovery of a new family of naphthopyrans with a larger conjugated π-system (Scheme 1, D) would be of great significance, yet remains challenging, probably owing to the unavoidable obstacles to compose these molecules.7 Recently, we realized a phosphoric acid-catalyzed exclusively linear allylation of alkoxyallenes with reactively enolated pyrazolones through a hydrogen-bonding interaction (Scheme 1, eqn (1)).8 Nevertheless, the transformation between alkoxyallenes and inert nucleophiles is still unknown reasonably on account of the limitation of their reactivity and reaction mode.9 Exploitation of straightforward and efficient access to achieve synthetic efficiency has always been a primary goal for the chemical sciences. Combining different kinds of reactions into cascades is one of the most effective strategies to enable expeditious synthesis of complex molecules.10 As is well documented, benzhydrol motifs are very versatile in Brønsted acid-catalyzed transformations by virtue of the high reactivity via hydroxyl release.11 We anticipate that implantation of such a reactive moiety into alkoxyallenes might lead to novel cascades by reaction with other nucleophiles bearing multiple reactive sites. The cascade reaction might provide practical and rapid access to larger π-conjugated aromatic compounds. Herein, we present an unprecedented allylation/cyclization/debenzyloxylation/isomerization/dehydration cascade reaction between benzhydrol alkoxyallenes and inert naphthols by a simple Brønsted acid catalysis under mild reaction conditions, affording a series of larger π-conjugated diphenylmethylene substituted naphthopyrans (Scheme 1, eqn (2)). The new class of naphthopyrans have aggregation-induced emission (AIE)12 and luminescence in the solid state ranging from the yellow to the near-infrared region. Most of those compounds are quite inert to UV light irradiation, which is promising for their potential utilization for long term and precise tracking.
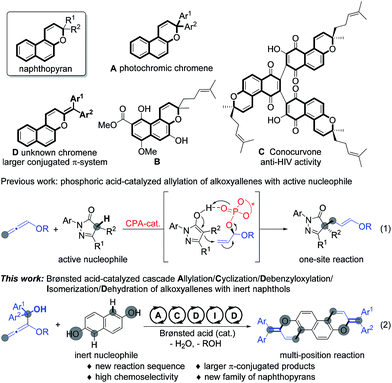 |
| Scheme 1 Typical naphthopyran structures and Brønsted acid-catalyzed addition of alkoxyallenes to nucleophiles. | |
Initially, alkoxyallene 1a and naphthol 2a were selected to optimize the reaction conditions (Table 1). Different Brønsted acids as the catalyst, including phosphoric acid, trifluoroacetic acid, and TsOH, gave disappointing results in 1,2-dichloroethane (DCE) at 25 °C (entries 1–3). Gratifyingly, the desired product 3aa was isolated in 56% yield from an unidentifiable mixture in the presence of 5.0 mol% phosphoric acid by raising the reaction temperature up to 80 °C (entries 4, 5). Other solvents were detrimental to the reaction results (entries 6–9). We speculated that the temperature was too high for the allylation/cyclization steps, which led to the above unidentifiable mixture. The treatment of the reaction mixture at 25 °C for 30 minutes followed by heating at 80 °C for 8 h improved the yield up to 88% (entry 10). Increasing the loading of acid to 10.0 mol% furnished the product in almost quantitative yield (entry 11). Additionally, CF3CO2H and TsOH as the catalyst under the optimal reaction conditions gave complicated results, probably owing to their strong acidity leading to the decomposition of 1a (entries 12, 13). As expected, no reaction occurred without an acid catalyst (entry 14).
Table 1 Optimization of the reaction conditions

|
Entrya |
Acid-cat. |
Solvent |
T (°C) |
Yieldb |
Reaction conditions: to the reaction mixture of allene 1a (0.15 mmol, 1.5 equiv.) and naphthol 2a (0.1 mmol) in solvent (1.0 mL) was added acid (5.0 mol%), and continued to stir for 8 h.
The yield was determined by 1H NMR spectroscopy.
n.r. means no reaction.
The reaction mixture was stirred at 25 °C for 30 min, then heated at 80 °C for 8 h.
10.0 mol% of phosphoric acid was used; the isolated product yield is given in parentheses.
|
1 |
(PhO)2PO2H |
DCE |
25 |
Trace |
2 |
CF3COOH |
DCE |
25 |
n.r.c |
3 |
TsOH |
DCE |
25 |
n.r.c |
4 |
(PhO)2PO2H |
DCE |
60 |
5% |
5 |
(PhO)2PO2H |
DCE |
80 |
56% |
6 |
(PhO)2PO2H |
Toluene |
80 |
Trace |
7 |
(PhO)2PO2H |
CH3CN |
80 |
Trace |
8 |
(PhO)2PO2H |
THF |
80 |
n.r.c |
9 |
(PhO)2PO2H |
EtOH |
80 |
n.r.c |
10d |
(PhO)2PO2H |
DCE |
80 |
88% |
11d,e |
(PhO)2PO2H |
DCE |
80 |
98% (93%) |
12d,e |
CF3COOH |
DCE |
80 |
Complicated |
13d,e |
TsOH |
DCE |
80 |
Complicated |
14 |
|
DCE |
80 |
n.r.c |
Under the optimized reaction conditions, the substrate scope with respect to both alkoxyallenes and naphthols was investigated to evaluate the generality of the reaction. As shown in Scheme 2, the reaction sequence could tolerate a wide range of naphthols. For reaction with 1a, besides 2a, both electron-donating and electron-withdrawing substituents at the aromatic rings are quite applicable to the optimal reaction conditions, leading to the corresponding products 3ab–an in good yields of 50–90%. Notably, the cascade transformation can be easily scaled up to 2.0 gram scale without an appreciable decrease in product yields (3ah). Triphenylamine (TPA) is extensively utilized in organic electroluminescent materials, special dye synthesis, and organic solar cells.13 Inspiringly, compound 3ak bearing a TPA moiety can be assembled in good yield with naphthol 2k as the substrate. Widely studied photochromism molecule naphthopyran 2l was also amenable to this reaction system, readily resulting in the corresponding product 3al in 85% yield. Treatment of more challenging substrates indol-5-ol 2o and 3,5-dimethoxyphenol 2p into the reaction afforded the products 3ao and 3ap in 75% and 60% yields, respectively. The regioselectivity of 2o took place exclusively at the 4-position. Dinaphthols could also be employed to deliver the double-annulation products 3aq–au in acceptable yields of 58–80%. Notably, the chiral 1,1′-bi-2-naphthol (BINOL) derivative 3ar could be obtained in 58% yield followed by “one-pot” demethylation with BBr3, which provided a new skeleton for the potential chiral ligand excavation.14 The weak disulfide bond was also compatible with the acid-catalyzed cascade process. Accordingly, the reaction of 2u with 1a facilely assembled the product 3au in 76% yield. Compounds 3ae and 3al were characterized by X-ray crystallographic analysis.15 To our delight, the scope of this reaction was further extended with a series of alkoxyallenes 1b–g and the desired products 3ba–ga were obtained in high yields of 67–84%, no matter whether symmetric alkoxyallenes 1b–d or asymmetric ones 1e–g were used as the starting materials.
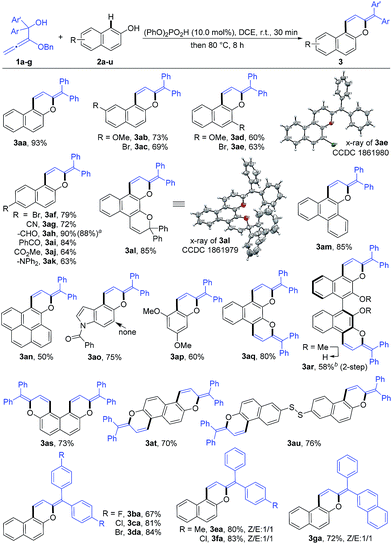 |
| Scheme 2 Reaction conditions: to the reaction mixture of 1 (0.75 mmol, 1.5 equiv.) and 2 (0.5 mmol) in DCE (5 mL) was added phosphoric acid (10.0 mol%), stirred at 25 °C for 30 min, then heated at 80 °C for 8 h. Yield is that of the isolated product. a2.0-gram scale. b10 equiv. of 1a was used and the yield is that of isolated 1,1′-bi-2-naphthol product after “one-pot” demethylation by BBr3 at room temperature. | |
In order to confirm the cascade reaction, a series of control reactions for the intermediates were executed. As demonstrated in Scheme 3, the initial allylation and cyclization were very fast and facile. Allylated product 4 was isolated in 63% yield by the reaction of 1a and 2a at 0 °C for only 5 minutes in the presence of 5.0 mol% phosphoric acid and 4 could be entirely cyclized just by warming to 25 °C for 30 minutes. Chromene 5 was also smoothly obtained in 95% yield by the direct reaction of 1a and 2a at the same conditions. Further increasing the temperature from 25 °C to 50 °C and heating for 20 minutes led to the reactive debenzyloxylated products 6a/6b in 31% yield with a ratio of 2
:
1. Final product 3aa was formed in quantitative yield by directly treating 5 to the optimized reaction conditions (Table 1, entry 11), and 6a/6b were monitored by TLC during the reaction. The cascade reaction could be completely blocked before dehydration if the alkoxyallenes 1h/h′ bearing a cyclohexyl group instead of biaryl substitutes were employed.
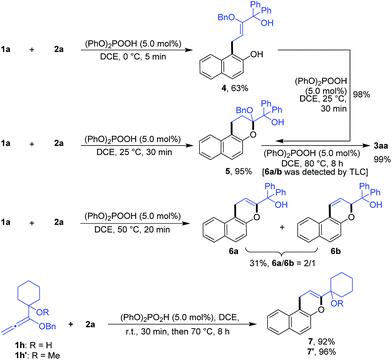 |
| Scheme 3 Investigation of intermediates. | |
These findings strongly support the reaction sequence via allylation/cyclization/debenzyloxylation/isomerization/dehydration with high efficiency (Scheme 4).
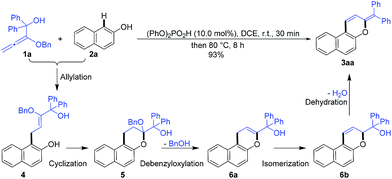 |
| Scheme 4 Proposed reaction sequence. | |
As a new family of larger π-conjugated naphthopyrans was obtained, we went on to investigate their photophysical properties. The absorptions and emissions were measured in the solid state under nitrogen at ambient temperature. With regard to the exemplified compounds 3ac, 3ad, 3ba and 3da, there are overall three absorption bands that are common in both solution and solid states (Table 2 and Fig. S1†). The strong absorption bands below 300 nm are normally assigned to local π–π* transitions of aryl rings. Bands in the 300–400 nm region are from the composited π systems corresponding to the naphthopyran16 or diphenylmethylene moiety,17 separately. The last absorption bands at 400–500 nm are assigned to the whole conjugated molecule system. Remarkably, those compounds exhibit solid-state photoluminescence in the visible region from yellow to red (Fig. 1). Compounds 3ae and 3al, involving similar half-side structure of 2-(diphenylmethylene)-2H-chromene with two pendent phenyls staggered with each other (Scheme 2, see crystal structures), have similar absorption and emission vibronic structures in their spectra (Fig. S1†). However, 3al emits red light, whereas 3ae gives red-color emission (Fig. 1). Those results showed that the emission colors can be well tuned via increasing π conjugation at the benzopyran site. Furthermore, compound 3aq emits light in the near-infrared range with a peak at 660 nm, which indicates a distinct packing morphology (Fig. S1†).18 The decaying lifetimes, measured via transient spectrometer, were a few tenths of nanoseconds to a few nanoseconds, indicating a typical fluorescence feature rather than a phosphorescent decay process (Table 2 and Fig. S2†).
Table 2 Photophysical properties of selected compounds
Compound |
λ
abs
(nm) |
λ
em
(nm) |
τ
(ns) |
Absorption peaks (λabs) were measured in neat film at room temperature.
Emission peaks were measured in powdery samples at room temperature.
Decaying lifetimes (τ) of these emitters in neat films.
|
3al
|
252/290/366/464 |
604 |
3.1 |
3an
|
273/358/473 |
557 |
0.99 |
3ao
|
326/443 |
533 |
0.58 |
3at
|
220/290/372/498 |
603 |
1.37 |
3ba
|
273/349/432 |
587 |
2.53 |
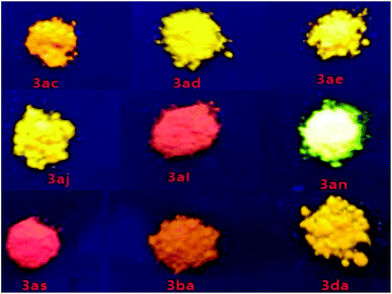 |
| Fig. 1 Selected illuminant compounds under UV irradiation at 365 nm. | |
Unlike the photochromic character of naphthopyrans,3f those compounds are inert to UV light irradiation and no structural or conformational variation was found on 3an and 3at when exposed under the intensity of 12 μW cm2 365 nm UV light over 10 hours. This should be ascribed to the stabilization effects of p–π hyperconjugation from the neighbouring diphenylmethylene group to the photosensitive C–O bonds, since natural naphthopyran3 structures involving a non-conjugated quaternary carbon centre are highly sensitive to light and heat.19 Comparing to their almost non-luminance in homogeneous THF solution, we deemed that those light emissive compounds have the AIE feature in conformity with the mechanism of the AIE molecule tetraphenylethene (Fig. 2), since both have diarylmethylene AIEgens.203an and 3at shined bright yellow and orange light under lighting from a 365 UV lamp, and became brighter with more aggregates in well dispersed suspension. The solution-suspension luminescent efficiency ΦFs of 3an in 1
:
8 THF
:
H2O (v/v) and 3at in 1
:
1.7 THF
:
H2O (v/v) are 1.9% and 2.3%, respectively. The results exhibit distinct photophysical functions compared to the natural naphthopyran molecules.
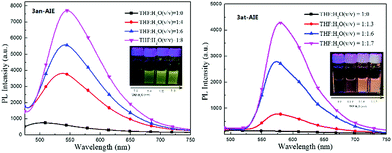 |
| Fig. 2 Photoluminescence spectra of 3an (left) and 3at (right) in THF/H2O mixtures. | |
Conclusions
In summary, we developed a practical and readily scalable reaction sequence for the straightforward synthesis of a new family of larger π-conjugated naphthopyrans by a Brønsted acid-catalyzed aromatic annulation of alkoxyallenes with inert naphthols. The cascade pathway involves allylation/cyclization/debenzyloxylation/isomerization/dehydration. The new class of diphenylmethylene substituted naphthopyrans was demonstrated to be AIEgenic with luminescent behavior in solid state. Those compounds are quite stable to UV light irradiation, which is promising for their potential utilization for long term and precise tracking. Given the importance of naphthopyrans in natural products and biocompatible photochromic materials, the method reported herein is of high importance as it provides access to hitherto unknown larger π-conjugated naphthopyran compounds whose optical properties investigation and underlying exploitation are highly warranted.
Conflicts of interest
There are no conflicts to declare.
Acknowledgements
Financial support from the Hundred Talent Program of Chinese Academy of Sciences (CAS), the National Natural Science Foundation of China (21202175 and 21602231), and the Natural Science Foundation of Jiangsu Province (BK20160396) is gratefully acknowledged.
Notes and references
-
(a) J. R. Cannon, K. R. Joshi, I. A. McDonald, R. W. Retallack, A. F. Sierakowki and L. C. H. Wong, Tetrahedron Lett., 1975, 16, 2795–2798 CrossRef;
(b) L. A. Decosterd, I. C. Parsons, K. R. Gustafson, J. H. Cardellina II, J. B. McMahon, G. M. Cragg, Y. Murata, L. K. Pannell, J. R. Steiner, J. Clardy and M. R. Boyd, J. Am. Chem. Soc., 1993, 115, 6673–6679 CrossRef CAS;
(c) J. F. Bukuru, T. N. Van, L. V. Puyvelde, S. G. Mathenge, F. P. Mudida and N. D. Kimpe, J. Nat. Prod., 2002, 65, 783–785 CrossRef CAS PubMed.
-
(a)
B. V. Gemert, Benzo and Naphthopyrans (Chromenes), in Organic Photochromic and Thermochromic Compounds, ed. J. C. Crano and R. Guglielmetti, Plenum Press, New York, 1999, vol. 1, ch. 3 Search PubMed;
(b) E. Bundgaard and F. C. Krebs, Sol. Energy Mater. Sol. Cells, 2007, 91, 954–985 CrossRef CAS;
(c)
I. F. Perepichka and D. F. Perepichka, Handbook of Thiophene-based Materials: Applications in Organic Electronics and Photonics, Wiley-VCH, Weinheim, 2009 CrossRef.
- For selected examples, see:
(a) K. Tanaka, H. Aoki, H. Hosomi and S. Ohba, Org. Lett., 2000, 2, 2133–2134 CrossRef CAS PubMed;
(b) W. Zhao and E. M. Carreira, Org. Lett., 2003, 5, 4153–4154 CrossRef CAS PubMed;
(c) M. Rawat, V. Prutyanov and W. D. Wulff, J. Am. Chem. Soc., 2006, 128, 11044–11053 CrossRef CAS PubMed;
(d) M. Frigoli, F. Maurel, J. Berthet, S. Delbaere, J. Marrot and M. M. Oliveira, Org. Lett., 2012, 14, 4150–4153 CrossRef CAS PubMed;
(e) H. Zhang, K. Wang, B. Wang, H. Yi, F. Hu, C. Li, Y. Zhang and J. Wang, Angew. Chem., Int. Ed., 2014, 53, 13234–13238 CrossRef CAS PubMed;
(f) K. Arai, Y. Kobayashia and J. Abe, Chem. Commun., 2015, 51, 3057–3060 RSC;
(g) J. Kong, T. Meng and J. Su, Org. Process Res. Dev., 2015, 19, 681–683 CrossRef CAS;
(h) J. M. Gil-Negrete, J. P. Sestelo and L. A. Sarandeses, Org. Lett., 2016, 18, 4316–4319 CrossRef CAS PubMed;
(i) V. Dimakos, T. Singh and M. S. Taylor, Org. Biomol. Chem., 2016, 14, 6703–6711 RSC;
(j) K. Tanaka, M. Sukekawa, Y. Shigematsu, Y. Hoshino and K. Honda, Tetrahedron, 2017, 73, 6456–6464 CrossRef CAS;
(k) V. Vece, S. Jakkepally and S. Hanessian, Org. Lett., 2018, 20, 4277–4280 CrossRef CAS PubMed.
- W. W. H. Lee, Z. Zhao, Y. Cai, Z. Xu, Y. Yu, Y. Xiong, R. T. K. Kwok, Y. Chen, N. L. C. Leung, D. Ma, J. W. Y. Lam, A. Qin and B. Z. Tang, Chem. Sci., 2018, 9, 6118–6125 RSC.
-
(a) C. Wang, Y. Xu, Y. Fang, M. Zhou, L. Liang, S. Singh, H. Zhao, A. Schober and Y. Lei, J. Am. Chem. Soc., 2015, 137, 3124–3130 CrossRef CAS PubMed;
(b) H. Liu, J. Zeng, J. Guo, H. Nie, Z. Zhao and B. Z. Tang, Angew. Chem., Int. Ed., 2018, 57, 9290–9294 CrossRef CAS PubMed.
- C. Yan, S. Barlow, Z. Wang, H. Yan, A. K.-Y. Jen, S. R. Marder and X. Zhan, Nat. Rev. Mater., 2018, 3, 18003 CrossRef CAS.
- H. J. Bestmann, G. Schmid, D. Sandmeier, G. Schade and H. Oechsner, Chem. Ber., 1985, 118, 1709–1719 CrossRef CAS.
- H. Zhou, Z. Wei, J. Zhang, H. Yang, C. Xia and G. Jiang, Angew. Chem., Int. Ed., 2017, 56, 1077–1081 CrossRef CAS PubMed.
- For selected examples, see:
(a) B. M. Trost, C. Jäkel and B. Plietker, J. Am. Chem. Soc., 2003, 125, 4438–4439 CrossRef CAS PubMed;
(b) P. Renaud, F. Beaufils, L. Feray and K. Schenk, Angew. Chem., Int. Ed., 2003, 42, 4230–4233 CrossRef CAS PubMed;
(c) B. M. Trost and J. Xie, J. Am. Chem. Soc., 2008, 130, 6231–6242 CrossRef CAS PubMed;
(d) B. M. Trost, J. Xie and J. D. Sieber, J. Am. Chem. Soc., 2011, 133, 20611–20622 CrossRef CAS PubMed;
(e) H. Kim, W. Lim, D. Im, D. G. Kim and Y. H. Rhee, Angew. Chem., Int. Ed., 2012, 51, 12055–12058 CrossRef CAS PubMed;
(f) H. Kim and Y. H. Rhee, J. Am. Chem. Soc., 2012, 134, 4011–4014 CrossRef CAS PubMed;
(g) W. Lim, J. Kim and Y. H. Rhee, J. Am. Chem. Soc., 2014, 136, 13618–13621 CrossRef CAS PubMed. For reviews, see:
(h) M. Brasholz, H.-U. Reissig and R. Zimmer, Acc. Chem. Res., 2009, 42, 45–56 CrossRef CAS PubMed;
(i) R. Zimmer and H.-U. Reissig, Chem. Soc. Rev., 2014, 43, 2888–2903 RSC.
-
(a) L. F. Tietze, Chem. Rev., 1996, 96, 115–136 CrossRef CAS PubMed;
(b) K. C. Nicolaou, D. J. Edmonds and P. G. Bulger, Angew. Chem., Int. Ed., 2006, 45, 7134–7186 CrossRef CAS PubMed;
(c) C. Grondal, M. Jeanty and D. Enders, Nat. Chem., 2010, 2, 167–178 CrossRef CAS PubMed;
(d) C. M. R. Volla, I. Atodiresei and M. Rueping, Chem. Rev., 2014, 114, 2390–2431 CrossRef CAS PubMed.
-
(a) C.-C. Hsiao, H.-H. Liao and M. Rueping, Angew. Chem., Int. Ed., 2014, 53, 13258–13263 CrossRef CAS PubMed;
(b) J. Jin, Y. Zhao, A. Gouranourimi, A. Ariafard and P. W. H. Chan, J. Am. Chem. Soc., 2018, 140, 5834–5841 CrossRef CAS PubMed.
-
(a) Y. Hong, J. W. Y. Lam and B. Z. Tang, Chem. Soc. Rev., 2011, 40, 5361–5388 RSC;
(b) J. Mei, Y. Hong, J. W. Y. Lam, A. Qin, Y. Tang and B. Z. Tang, Adv. Mater., 2014, 26, 5429–5479 CrossRef CAS PubMed;
(c) J. Mei, N. L. C. Leung, R. T. K. Kwok, J. W. Y. Lam and B. Z. Tang, Chem. Rev., 2015, 115, 11718–11940 CrossRef CAS PubMed.
- For selected examples, see:
(a) J. Zhang, G. Wu, C. He, D. Deng and Y. Li, J. Mater. Chem., 2011, 21, 3768–3774 RSC;
(b) M.-C. Tang, D. P.-K. Tsang, Y.-C. Wong, M.-Y. Chan, K. M.-C. Wong and V. W.-W. Yam, J. Am. Chem. Soc., 2014, 136, 17861–17868 CrossRef CAS PubMed;
(c) K. Kawasumi, T. Wu, T. Zhu, H. S. Chae, T. V. Voorhis, M. A. Baldo and T. M. Swager, J. Am. Chem. Soc., 2015, 137, 11908–11911 CrossRef CAS PubMed;
(d) Z. Li, Z. Zhu, C.-C. Chueh, S. B. Jo, J. Luo, S.-H. Jang and K.-Y. Jen, J. Am. Chem. Soc., 2016, 138, 11833–11839 CrossRef CAS PubMed;
(e) C. Li, R. Duan, B. Liang, G. Han, S. Wang, K. Ye, Y. Liu, Y. Yi and Y. Wang, Angew. Chem., Int. Ed., 2017, 56, 11525–11529 CrossRef CAS PubMed;
(f) X. Tao, L. Li, Y. Zhou, X. Qian, M. Zhao, L. Cai and X. Xie, Chin. J. Chem., 2017, 35, 1749–1754 CrossRef CAS;
(g) W.-C. Chen, Y. Yuan, Z.-L. Zhu, Z.-Q. Jiang, S.-J. Su, L.-S. Liao and C.-S. Lee, Chem. Sci., 2018, 9, 4062–4070 RSC.
-
(a) Y. Chen, S. Yekta and A. K. Yudin, Chem. Rev., 2003, 103, 3155–3212 CrossRef CAS PubMed;
(b) J. M. Brunel, Chem. Rev., 2005, 105, 857–898 CrossRef CAS PubMed;
(c) P. W. N. M. van Leeuwen, P. C. J. Kamer, C. Claver, O. Pàmies and M. Diéguez, Chem. Rev., 2011, 111, 2077–2118 CrossRef CAS PubMed;
(d)
S.-F. Zhu and Q.-L. Zhou, Privileged Chiral Ligands and Catalysts, ed. Q.-L. Zhou, Wiley-VCH, Weinheim, 2011 CrossRef;
(e) D. Parmar, E. Sugiono, S. Raja and M. Rueping, Chem. Rev., 2014, 114, 9047–9153 CrossRef CAS PubMed.
- CCDC 1861980 (3ae) and CCDC 1861979 (3al) contain the supplementary crystallographic data for this paper.†.
- R. S. Becker, G. Favaro, A. Romani, P. L. Gentili and F. M. B. Dias, Chem. Phys., 2005, 316, 108–116 CrossRef CAS.
- L. Ding, L. Lin, C. Liu, H. Li, A. Qin, Y. Liu, L. Song, H. Zhang, B. Z. Tang and Y. Zhao, New J. Chem., 2011, 35, 1781–1786 RSC.
- C. Zheng, Q. Zang, H. Nie, W. Huang, Z. Zhao, A. Qin, R. Hu and B. Z. Tang, Mater. Chem. Front., 2018, 2, 180–188 RSC.
- G. Favaro, A. Romani and F. Ortica, Photochem. Photobiol. Sci., 2003, 2, 1032–1037 RSC.
- J. Mei, Y. Hong, J. W. Y. Lam, A. Qin, Y. Tang and B. Z. Tang, Adv. Mater., 2014, 26, 5429–5479 CrossRef CAS PubMed.
Footnote |
† Electronic supplementary information (ESI) available. CCDC 1861979 and 1861980. For ESI and crystallographic data in CIF or other electronic format see DOI: 10.1039/c8sc03837f |
|
This journal is © The Royal Society of Chemistry 2019 |