DOI:
10.1039/C8SC03315C
(Edge Article)
Chem. Sci., 2019,
10, 161-166
Transition-metal free C–C bond cleavage/borylation of cycloketone oxime esters†
Received
26th July 2018
, Accepted 28th September 2018
First published on 2nd October 2018
Abstract
An efficient transition-metal free C–C bond cleavage/borylation of cycloketone oxime esters has been described. In this reaction, the B2(OH)4 reagent not only served as the boron source but also acted as an electron donor source through formation of a complex with a DMAc-like Lewis base. This complex could be used as an efficient single electron reductant in other ring-opening transformations of cycloketone oxime esters. Free-radical trapping, radical-clock, and DFT calculations all suggest a radical pathway for this transformation.
Introduction
Alkylboronic esters are very important building blocks in organic synthesis as well as in medicinal chemistry and materials science (Fig. 1).1,2 For instance, they have been widely used in Suzuki–Miyaura coupling reactions.3 Thus, many chemists have devoted their efforts to develop facile and efficient methods for the synthesis of alkylborons.4 The classical method relies on the trapping of highly reactive alkyl-Li or alkyl-Mg reagents with suitable boron compounds.5 However, this procedure suffers from strict conditions as well as poor functional-group tolerance. In recent years, a number of C–B bond formation reactions, including transition-metal catalyzed borylation of alkyl halides, decarboxylative borylation of aliphatic esters,6 hydroboration of alkenes and others have emerged as attractive alternatives to classical methods.7 Nevertheless, noble metal catalysts, strong bases and ligands are usually required in these reactions. Therefore, developing mild borylation reactions to access functionalized alkylboron compounds is still desirable and highly in demand.4
 |
| Fig. 1 Biologically active compounds containing alkylboronic moieties. | |
Since the pioneering work of Zard and Uemura, C–C bond cleavage of cycloketone oxime derivatives has emerged as an attractive strategy to construct C–C and C–Y (Y = O, S, Se, Te, or X) bonds.8–10 Recently, an array of radical C–C bond cleavages of cycloketone oxime derivatives have been developed under different catalytic systems including transition-metal catalysis and visible-light photocatalysis (Fig. 2, route a).9a–h In addition, the Castle group reported a microwave-promoted C–C bond cleavage of cycloketone oxime ethers.9i In this regard, our group presented a series of iron and copper catalyzed C–C bond cleavages of cycloketone oxime esters.10 These established protocols provided efficient approaches to incorporate the versatile cyanoalkyl moieties into structurally diverse molecules, wherein the iminyl radical was the pivotal intermediate. Although remarkable advances have been made, the C–C bond cleavage/borylation of cycloketone oxime derivatives remains unexplored. Very recently, some elegant transition metal-free borylation reactions have been reported by different research groups.11 Intriguingly, the diboron species not only served as a boron source but also acted as an electron donor source to generate reactive radical intermediates.11,12 Inspired by these studies and our previous results, we wish to explore a novel catalytic system to form a reactive iminyl radical and simultaneously to construct C(sp3)–B bonds (Fig. 2, route b). Herein, we report a transition metal free C–C bond cleavage/borylation of cyclobutanone oxime esters through using a diboron reagent as the boron source and activator. This protocol provided a straightforward access to cyanoalkyl boronic esters, a class of versatile building blocks in organic synthesis,2,13 in good yields.
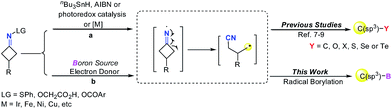 |
| Fig. 2 Transformation of cyclobutanone oxime derivatives. | |
Results and discussion
To test our hypothesis, cyclobutanone oxime ester 1a was treated with 1.2 equiv. of bis(pinacolato)diboron (B2pin2) in DMAc at room temperature under the irradiation of a 23 W CFL bulb. To our delight, the desired pinacol cyanoalkyl boronic ester 2a was obtained in 7% yield (Table 1, entry 1). When bis(catecholato)diboron (B2cat2) was used instead of B2pin2, the yield of 2a was increased to 54% after workup with pinacol and NEt3 (entry 2). However, the yield of 2a decreased dramatically when the reaction was conducted in the dark. Satisfactorily, tetrahydroxydiboron B2(OH)4 showed better reaction efficiency and gave the desired product 2a in 61% yield (entry 4). Surprisingly, it was found that photoactivation was not essential for the reaction with B2(OH)4. Treatment of 1a with 2.0 equiv. of B2(OH)4 still resulted in a 60% yield of 2a even in the dark (entry 5). A similar result was also observed under ambient light (entry 6). These results indicated that the reaction was not triggered by a light source. Notably, increasing the amount of B2(OH)4 to 3.0 equiv. improved the yield of 2a to 77%, while further increasing the amount of B2(OH)4 did not improve the yields of 2a (entries 7 and 8). Solvent screening indicated that other amide-based solvents such as NMP and DMF were less effective (entries 9 and 10). Additionally, other types of solvents such as DCM, acetone and MeCN all furnished worse yields (entries 11–13). It should be noted that the reaction in DCM using 1.0 equiv. of DMAc as the additive only afforded a trace amount of 2a (entry 14). Finally, other additives such as 4-phenylpyridine, 4-cyanopyridine, DMAP and Cs2CO3 were also tested, but none of them gave better results than DMAc alone (entries 15–18).
Table 1 Optimization of reaction conditionsa
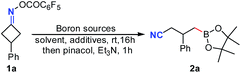
|
Entry |
Boron sources (equiv.) |
Solvent |
Additives (equiv.) |
Yieldb (%) |
Reaction conditions: 1a (0.20 mmol, 1.0 equiv.), boron sources (2.0–4.0 equiv.), additives (1.0 equiv.), in 2.0 mL of solvent at room temperature under N2 for 16 h; then pinacol (0.8 mmol, 4.0 equiv.) dissolved in Et3N (0.7 mL) was added to the reaction mixture and stirred for 1 h.
NMR yields by using CH2Br2 as the internal standard.
Reaction by irradiation with a 23 W compact fluorescent light (CFL) bulb.
Without addition of pinacol and Et3N.
The reaction was conducted in the dark.
n.r. = no reaction.
3-Phenylbutanenitrile was observed as the major product.
|
1 |
B2pin2 (2.0) |
DMAc |
|
7c,d |
2 |
B2Cat2 (2.0) |
DMAc |
|
54c |
3 |
B2Cat2 (2.0) |
DMAc |
|
28e |
4 |
B2(OH)4 (2.0) |
DMAc |
|
61c |
5 |
B2(OH)4 (2.0) |
DMAc |
|
60e |
6 |
B2(OH)4 (2.0) |
DMAc |
|
61 |
7
|
B
2
(OH)
4
(3.0)
|
DMAc
|
|
77
|
8 |
B2(OH)4 (4.0) |
DMAc |
|
73 |
9 |
B2(OH)4 (3.0) |
NMP |
|
66 |
10 |
B2(OH)4 (3.0) |
DMF |
|
52 |
11 |
B2(OH)4 (3.0) |
MeCN |
|
20 |
12 |
B2(OH)4 (3.0) |
Acetone |
|
15 |
13 |
B2(OH)4 (3.0) |
DCM |
|
n.r.f |
14 |
B2(OH)4 (3.0) |
DCM |
DMAc (1.0) |
Trace |
15 |
B2(OH)4 (3.0) |
DMAc |
4-Phenylpyridine (1.0) |
56 |
16 |
B2(OH)4 (3.0) |
DMAc |
4-Cyanopyridine (1.0) |
Traceg |
17 |
B2(OH)4 (3.0) |
DMAc |
DMAP (1.0) |
63 |
18 |
B2(OH)4 (3.0) |
DMAc |
Cs2CO3 (1.0) |
26 |
With the optimal conditions in hand, the generality and limitations of cyclobutanone oxime esters were examined (Table 2). A variety of 3-aryl, benzyl and alkyl substituted oxime esters were efficiently engaged in this ring-opening/borylation reaction to provide the corresponding boronic esters 2a–2q in moderate to good isolated yields. It was found that the nature of the substituents on the aromatic ring has a significant effect on the reaction efficiency (2e–2gvs.2h and 2i). Satisfactorily, functional groups including halogen (2d, 2h, 2j), ester (2i, 2o, 2p) and ether (2m, 2q) in the oxime esters were well-tolerated. Cyclobutanone oxime ester 1r without any substituent furnished the desired product 2r in 85% yield. The 3,3-disubstituted substrates 1s and 1t also afforded the target products 2s and 2t in 76% and 34% yields, respectively.
Table 2 Scope of cyclobutanone oxime estersa
1 (0.2 mmol), B2(OH)4 (0.6 mmol), DMAc (2 mL) at room temperature under N2 for 16 h; then workup with pinacol (0.8 mmol) and Et3N (0.7 mL), isolated yields.
|
|
Notably, besides primary boronic esters, this procedure was also applicable to provide the secondary ones under modified conditions. The 2,3-disubstituted oxime esters 1u and 1v underwent the ring-opening/borylation process regioselectively to afford the desired products 2u and 2v in acceptable yields by using 1.2 equiv. of B2cat2 as the boron source at 80 °C under irradiation with 23 W CFL bulbs. It should be noted that the light irradiation, heating and boron source are all important for these reactions, implying that direct photolysis of B2Cat2 accounts partly for the formation of 2u and 2v (eqn (1) and (2)). The tricyclo[5.2.1.0(2,6)]decan-8-one oxime ester 1w also gave the anticipated secondary boronic ester 2w as a single diastereomer under the modified conditions, albeit in somewhat low yield (eqn (3)). Unfortunately, the oxime ester derived from 2-substituted cyclopentanone or cyclohexanone could not afford the desired product under the present conditions.
|  | (1) |
| 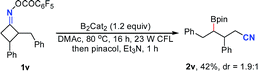 | (2) |
| 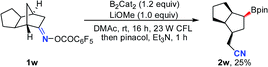 | (3) |
Satisfactorily, this metal free C–C cleavage/borylation reaction of cyclobutanone oxime esters could be scaled up. For instance, the reaction of 1a on a 2.0 mmol scale gave the product 2a in 67% isolated yield. Furthermore, the product of this reaction was not limited to pinacol boronic ester. Using methyl iminodiacetic acid (MIDA) instead of pinacol for the reaction workup, the corresponding product 3a was obtained in 60% yield. Moreover, the product 2a could be oxidized by H2O2 followed by hydrolysis to deliver the alcohol 4a in 80% yield (Scheme 1). Finally, treatment of cyanoalkyl boronic ester 2r with 4-trifluoromethylbromobenzene in the presence of a palladium catalyst afforded the coupling product 4b in 28% yield (without optimization).
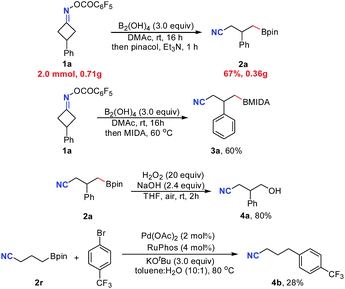 |
| Scheme 1 Synthesis of 2a on a gram scale and derivatization of the product. | |
To gain some understanding of the reaction, several control experiments were conducted (Fig. 3). When TEMPO, a typical radical scavenger was subjected to the reaction conditions, only trace amount of 2a was observed, along with the cyanoalkyl-TEMPO adduct 5a which was isolated in 50% yield, implying that a radical intermediate was involved in this transformation. In contrast, without B2(OH)4 or DMAc, the reaction of 1a with TEMPO did not take place, suggesting that both the diboron reagent and amide-based solvent play a critical role in this ring-opening process (Fig. 3, eqn (4)). Furthermore, the radical-clock substrates 6a and 6b furnished the cyclized products 7a and 7bvia a ring-opening/cyclization/borylation cascade, wherein no linear coupling product was detected (Fig. 3, eqn (5) and (6)). Treatment of 6c under the standard conditions led to the product 7c in 58% yield as the sole product (Fig. 3, eqn (7)). These results also support a radical pathway.
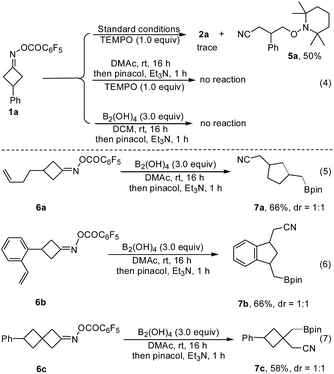 |
| Fig. 3 Control experiments. | |
Based upon the preliminary results and previous reports, a possible mechanism was proposed for this reaction with the further aid of DFT calculation (Scheme 2, for details, see the ESI†). This borylation reaction probably proceeds through a radical chain propagation mechanism. First, cyclobutanone oxime ester 1a gives the DMAc-stabilized radical intermediate I through thermal cleavage of the B–B bond of B2(OH)4·DMAc complex.11,12 Second, N–O bond cleavage of radical I affords the iminyl radical II, which delivers radical III through a β-carbon elimination process.7–9 Afterward, the alkyl radical III reacts with DMAc-ligated B2(OH)4 to produce the precursor of the desired product 2a′ and DMAc-stabilized boryl radical IV.11,12,14 Finally, the resulting boryl radical IV might propagate a radical chain process. It is worth mentioning that ArCOOB(OH)2 can be detected by LCMS during the reaction, which also provided evidence for our proposed catalytic cycle. On the other hand, based on the above result and UV-vis spectrum of 1u and B2Cat2 in DMAc, direct light-triggered homolysis of B2Cat2 might be involved in the reaction of 1u with B2Cat2.
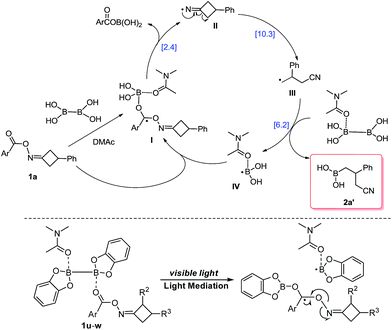 |
| Scheme 2 Proposed mechanism. | |
Remarkably, this simple B2(OH)4/DMAc system was also applicable to other C–C bond cleavage/C–C bond formations (Fig. 4). The reaction of 1a with quinoxalin-2(1H)-one 8a and quinine 8b also worked smoothly to give the cyanoalkylated products 9a and 9b in 52% and 32% yields, respectively (without optimization).10a,b These results suggested that this new transition metal free system could be used instead of transition metal catalytic systems, further indicating their potential applications in radical chemistry.
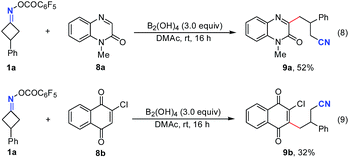 |
| Fig. 4 Reductive cleavage reactions employing the B2(OH)4/DMAc system. | |
Conclusions
In summary, we have demonstrated the first transition-metal free C–C bond cleavage/borylation of cyclobutanone oxime esters. This protocol is amenable to a variety of cyclobutanone oxime esters, thus providing a facile access to cyanoalkyl boronic esters in good yields. Primary mechanism studies revealed that B2(OH)4 not only serves as a boron source but also plays a crucial role in the C–C bond cleavage process. Further studies on the mechanistic details are currently underway in our laboratory.
Conflicts of interest
There are no conflicts to declare.
Acknowledgements
Financial support from Natural Science Basic Research Plan in Shaanxi Province of China (No. 2016JZ002), and the Fundamental Research Funds of the Central Universities (No. zrzd2017001 and xjj2016056) is greatly appreciated. We thank Prof. Pengfei Li in XJTU for a helpful discussion. We also thank Miss Lu at the Instrument Analysis Center of Xi'an Jiaotong University for her assistance with HRMS analysis.
Notes and references
-
(a) R. Jana, T. P. Pathak and M. S. Sigman, Chem. Rev., 2011, 111, 1417 CrossRef CAS;
(b) A. Rudolph and M. Lautens, Angew. Chem., Int. Ed., 2009, 48, 2656 CrossRef CAS;
(c) A. C. Frisch and M. Beller, Angew. Chem., Int. Ed., 2005, 44, 674 CrossRef CAS;
(d)
P. C. Trippier and C. McGuigan, Preparation and Applications in Organic Synthesis and Medicine, Wiley, Weinheim, 2005 Search PubMed;
(e) P. C. Trippier and C. McGuigan, MedChemComm, 2010, 1, 183 RSC;
(f)
A. Draganov, D. Wang and B. Wang, The future of boron in medicinal chemistry: therapeutic and diagnostic applications, Springer, 2014 Search PubMed;
(g) M. A. Beenen, C. An and J. A. Ellman, J. Am. Chem. Soc., 2008, 130, 6910 CrossRef CAS;
(h) L. J. Milo, J. H. Lai, W. Wu, Y. Liu, H. Maw, Y. Li, Z. Jin, Y. Shu, S. E. Poplawski, Y. Wu, D. G. Sanford, J. L. Sudmeier and W. W. Bachovchin, J. Med. Chem., 2011, 54, 4365 CrossRef CAS;
(i) W. L. A. Brooks and B. S. Sumerlin, Chem. Rev., 2016, 116, 1375 CrossRef CAS.
- For selected examples on biologically active compounds containing alkylboronic moieties, see:
(a) N. Suzuki, T. Suzuki, Y. Ota, T. Nakano, M. Kurihara, H. Okuda, T. Yamori, H. Tsumoto, H. Nakagawa and N. Miyata, J. Med. Chem., 2009, 52, 2909 CrossRef CAS;
(b) Y. Zhu, G. Wu, X. Zhu, Y. Ma, X. Zhao, Y. Li, Y. Yuan, J. Yang, S. Yu, F. Shao and M. Lei, J. Med. Chem., 2010, 53, 8619 CrossRef CAS;
(c) J. D. Ashley, J. F. Stefanick, V. A. Schroeder, M. A. Suckow, T. Kiziltepe and B. Bilgicer, J. Med. Chem., 2014, 57, 5282 CrossRef CAS;
(d) E. Caselli, C. Romagnoli, R. Vahabi, M. A. Taracila, R. A. Bonomo and F. Prati, J. Med. Chem., 2015, 58, 5445 CrossRef CAS;
(e) C. Nitsche, L. Zhang, L. F. Weigel, J. Schilz, D. Graf, R. Bartenschlager, R. Hilgenfeld and C. D. Klein, J. Med. Chem., 2017, 60, 511 CrossRef CAS PubMed. Cyanoalkylboronic esters are also applied to modify pharmaceutically active compounds through Suzuki–Miyaura couplings, for examples, see:
(f)
V. Maurizio, T. Massimiliano, M. Giacomo, L. Cesari, S. Galeazzi, A. Caricasole, A. Chiumiento and M. Salerno, US Pat., 20140005164A1, 2014;
(g)
J. Ravi, J.-R. Roland and W. Juergen, US Pat., 20160016963A1, 2016;
(h)
L. Wu, B. Shen, J. Li, Z. Li, K. Liu, F. Zhang and W. Yao, US Pat., 20170107216A1, 2017.
-
(a) A. Suzuki, Angew. Chem., Int. Ed., 2011, 50, 6722 CrossRef CAS;
(b)
A. Suzuki, in Metal-Catalyzed Cross-Coupling Reactions, Wiley, Weinheim, 1998 Search PubMed;
(c) N. Miyaura and A. Suzuki, Chem. Rev., 1995, 95, 2457 CrossRef CAS;
(d) A. Suzuki, J. Organomet. Chem., 1999, 576, 147 CrossRef CAS.
- For selected reviews, see:
(a) I. A. I. Mkhalid, J. H. Barnard, T. B. Marder, J. M. Murphy and J. F. Hartwig, Chem. Rev., 2010, 110, 890 CrossRef CAS;
(b) E. Hartmann, D. J. Vyas and M. Oestreich, Chem. Commun., 2011, 47, 7917 RSC;
(c) A. Ros, R. Fernández and J. M. Lassaletta, Chem. Soc. Rev., 2014, 43, 3229 RSC;
(d) E. C. Neeve, S. J. Geier, I. A. I. Mkhalid, S. A. Westcott and T. B. Marder, Chem. Rev., 2016, 116, 9091 CrossRef CAS;
(e) K. Kubota, H. Iwamoto and H. Ito, Org. Biomol. Chem., 2017, 15, 285 RSC;
(f) B. S. L. Collins, C. M. Wilson, E. L. Myers and V. K. Aggarwal, Angew. Chem., Int. Ed., 2017, 56, 11700 CrossRef CAS;
(g) A. B. Cuenca, R. Shishido, H. Ito and E. Fernández, Chem. Soc. Rev., 2017, 46, 415 RSC;
(h) K. Chen, L. Wang, G. Meng and P. Li, Synthesis, 2017, 49, 4719 CrossRef CAS;
(i) G. Yan, D. Huang and X. Wu, Adv. Synth. Catal., 2018, 360, 1040 CrossRef CAS.
-
(a) G. Zweifel and H. C. Brown, Org. React., 1963, 13, 1 CAS;
(b)
H. C. Brown, Organic Synthesis via Organoboranes, Wiley, New York, 1975 Search PubMed;
(c) H. C. Brown and T. E. Cole, Organometallics, 1983, 2, 1316 CrossRef CAS.
-
(a) A. Bej, D. Srimani and A. Sarkar, Green Chem., 2012, 14, 661 RSC;
(b) J. Yi, J.-H. Liu, J. Liang, J.-J. Dai, C.-T. Yang, Y. Fu and L. Liu, Adv. Synth. Catal., 2012, 354, 1685 CrossRef CAS;
(c) A. Joshi-Pangu, X. Ma, M. Diane, S. Iqbal, R. J. Kribs, R. Huang, C.-Y. Wang and M. R. Biscoe, J. Org. Chem., 2012, 77, 6629 CrossRef CAS;
(d) A. S. Dudnik and G. C. Fu, J. Am. Chem. Soc., 2012, 134, 10693 CrossRef CAS;
(e) C. Kleeberg, L. Dang, Z. Lin and T. B. Marder, Angew. Chem., Int. Ed., 2009, 48, 5350 CrossRef CAS;
(f) C.-T. Yang, Z.-Q. Zhang, H. Tajuddin, C.-C. Wu, J. Liang, J.-H. Liu, Y. Fu, M. Czyzewska, P. G. Steel, T. B. Marder and L. Liu, Angew. Chem., Int. Ed., 2012, 51, 528 CrossRef CAS;
(g) H. Ito and K. Kubota, Org. Lett., 2012, 14, 890 CrossRef CAS PubMed;
(h) S. K. Bose, K. Fucke, L. Liu, P. G. Steel and T. B. Marder, Angew. Chem., Int. Ed., 2014, 53, 1799 CrossRef CAS PubMed;
(i) T. C. Atack, R. M. Lecker and S. P. Cook, J. Am. Chem. Soc., 2014, 136, 9521 CrossRef CAS;
(j) M. Jiang, H. Yang and H. Fu, Org. Lett., 2016, 18, 5248 CrossRef CAS PubMed;
(k) T. C. Atack and S. P. Cook, J. Am. Chem. Soc., 2016, 138, 6139 CrossRef CAS;
(l) C. Li, J. Wang, L. M. Barton, S. Yu, M. Tian, D. S. Peters, M. Kumar, A. W. Yu, K. A. Johnson, A. K. Chatterjee, M. Yan and P. S. Baran, Science, 2017, 356, 7355 CrossRef;
(m) D. Hu, L. Wang and P. Li, Org. Lett., 2017, 19, 2770 CrossRef CAS.
- For selected examples, see:
(a) C. M. Vogels and S. A. Westcott, Curr. Org. Chem., 2005, 9, 687 CrossRef CAS;
(b) K. S. Lee, A. R. Zhugralin and A. H. Hoveyda, J. Am. Chem. Soc., 2009, 131, 7253 CrossRef CAS;
(c) J. A. Schiffner, K. Müther and M. Oestreich, Angew. Chem., Int. Ed., 2010, 49, 1194 CrossRef CAS;
(d) K. M. Logan, S. R. Sardini, S. D. White and M. K. Brown, J. Am. Chem. Soc., 2018, 140, 159 CrossRef CAS;
(e) Z. Liu, H.-Q. Ni, T. Zeng and K. M. Engle, J. Am. Chem. Soc., 2018, 140, 3223 CrossRef CAS.
-
(a) J. Boivin, E. Fouquet and S. Z. Zard, J. Am. Chem. Soc., 1991, 113, 1055 CrossRef CAS;
(b) J. Boivin, E. Fouquet and S. Z. Zard, Tetrahedron Lett., 1991, 32, 4299 CrossRef CAS;
(c) A.-C. Callier-Dublanchet, B. Quiclet-Sire and S. Z. Zard, Tetrahedron Lett., 1997, 38, 2463 CrossRef CAS;
(d) T. Nishimura and S. Uemura, J. Am. Chem. Soc., 2000, 122, 12049 CrossRef CAS;
(e) T. Nishimura, Y. Nishiguchi, Y. Maeda and S. Uemura, J. Org. Chem., 2004, 69, 5342 CrossRef CAS PubMed;
(f) T. Nishimura, T. Yoshinaka, Y. Nishiguchi, Y. Maeda and S. Uemura, Org. Lett., 2005, 7, 2425 CrossRef CAS.
-
(a) H.-B. Yang and N. Selander, Chem.–Eur. J., 2017, 23, 1779 CrossRef CAS;
(b) B. Zhao and Z. Shi, Angew. Chem., Int. Ed., 2017, 56, 12727 CrossRef CAS;
(c) W. Ai, Y. Liu, Q. Wang, Z. Lu and Q. Liu, Org. Lett., 2018, 20, 409 CrossRef CAS;
(d) L. Li, H. Chen, M. Mei and L. Zhou, Chem. Commun., 2017, 53, 11544 RSC;
(e) H. Jiang and A. Studer, Angew. Chem., Int. Ed., 2017, 56, 12273 CrossRef CAS;
(f) J. Davies, N. S. Sheikh and D. Leonori, Angew. Chem., Int. Ed., 2017, 56, 13361 CrossRef CAS;
(g) X.-Y. Yu, J.-R. Chen, P.-Z. Wang, M.-N. Yang, D. Liang and W.-J. Xiao, Angew. Chem., Int. Ed., 2018, 57, 738 CrossRef CAS;
(h) E. M. Dauncey, S. P. Marcillo, J. J. Douglas, N. S. Sheikh and D. Leonori, Angew. Chem., Int. Ed., 2018, 57, 744 CrossRef CAS;
(i) M. M. Jackman, S. Im, S. R. Bohman, C. C. L. Lo, A. L. Garrity and S. L. Castle, Chem.–Eur. J., 2018, 24, 594 CrossRef CAS.
-
(a) Y.-R. Gu, X.-H. Duan, L. Yang and L.-N. Guo, Org. Lett., 2017, 19, 5908 CrossRef CAS;
(b) L. Yang, P. Gao, X.-H. Duan, Y.-R. Gu and L.-N. Guo, Org. Lett., 2018, 20, 1034 CrossRef CAS PubMed;
(c) J. Wu, J.-Y. Zhang, P. Gao, S.-L. Xu and L.-N. Guo, J. Org. Chem., 2018, 83, 1046 CrossRef CAS PubMed;
(d) J.-Y. Zhang, X.-H. Duan, J.-C. Yang and L.-N. Guo, J. Org. Chem., 2018, 83, 4239 CrossRef CAS;
(e) J.-F. Zhao, P. Gao, X.-H. Duan and L.-N. Guo, Adv. Synth. Catal., 2018, 360, 1775 CrossRef CAS;
(f) J.-F. Zhao, X.-H. Duan, Y.-R. Gu, P. Gao and L.-N. Guo, Org. Lett., 2018, 20, 4614 CrossRef CAS PubMed.
- For recent examples concerning metal-free radical C(sp2)-B bond formation reactions, see:
(a) F. Mo, Y. Jiang, D. Qiu, Y. Zhang and J. Wang, Angew. Chem., Int. Ed., 2010, 49, 1846 CrossRef CAS PubMed;
(b) K. Chen, M. S. Cheung, Z. Lin and P. Li, Org. Chem. Front., 2016, 3, 875 RSC;
(c) A. M. Mfuh, J. D. Doyle, B. Chhetri, H. D. Arman and O. V. Larionov, J. Am. Chem. Soc., 2016, 138, 2985 CrossRef CAS PubMed;
(d) K. Chen, S. Zhang, P. He and P. Li, Chem. Sci., 2016, 7, 3676 RSC;
(e) A. M. Mfuh, V. T. Nguyen, B. Chhetri, J. E. Burch, J. D. Doyle, V. N. Nesterov, H. D. Arman and O. V. Larionov, J. Am. Chem. Soc., 2016, 138, 8408 CrossRef CAS PubMed;
(f) L. Zhang and L. Jiao, J. Am. Chem. Soc., 2017, 139, 607 CrossRef CAS;
(g) L. Candish, M. Teders and F. Glorius, J. Am. Chem. Soc., 2017, 139, 7440 CrossRef CAS;
(h) W.-M. Cheng, R. Shang, B. Zhao, W.-L. Xing and Y. Fu, Org. Lett., 2017, 19, 4291 CrossRef CAS;
(i) W. Liu, X. Yang, Y. Gao and C.-J. Li, J. Am. Chem. Soc., 2017, 139, 8621 CrossRef CAS PubMed;
(j) L. Zhang and L. Jiao, Chem. Sci., 2018, 9, 2711 RSC; for C(sp3)-B bond formation, see:
(k) A. Fawcett, J. Pradeilles, Y. Wang, T. Mutsuga, E. L. Myers and V. K. Aggarwal, Science, 2017, 357, 283 CrossRef CAS PubMed;
(l) Y. Cheng, C. Mück-Lichtenfeld and A. Studer, J. Am. Chem. Soc., 2018, 140, 6221 CrossRef CAS.
- For selected examples using diboron reagents for single-electron reductant, see:
(a) J. Cid, H. Gulyas, J. J. Carbo and E. Fernández, Chem. Soc. Rev., 2012, 41, 3558 RSC;
(b) R. D. Dewhurst, E. C. Neeve, H. Braunschweig and T. B. Marder, Chem. Commun., 2015, 51, 9594 RSC;
(c) G. Wang, H. Zhang, J. Zhao, W. Li, J. Cao, C. Zhu and S. Li, Angew. Chem., Int. Ed., 2016, 55, 5985 CrossRef CAS;
(d) G. Wang, J. Cao, L. Gao, W. Chen, W. Huang, X. Cheng and S. Li, J. Am. Chem. Soc., 2017, 139, 3904 CrossRef CAS;
(e) Y. Katsuma, H. Asakawa and M. Yamashita, Chem. Sci., 2018, 9, 1301 RSC;
(f) J. Cao, G. Wang, L. Gao, X. Cheng and S. Li, Chem. Sci., 2018, 9, 3664 RSC;
(g) A. Guo, J. Han and X. Tang, Org. Lett., 2018, 20, 2351 CrossRef CAS.
-
(a)
Chemistry of the Cyano Group, ed. Z. Pappoport and S. Patai, Wiley, London, 1970 Search PubMed;
(b) F. F. Fleming, Nat. Prod. Rep., 1999, 16, 597 RSC;
(c) E. L. May, A. E. Jacobson, M. V. Mattson, J. R. Traynor, J. H. Woods, L. S. Harris, E. R. Bowman and M. D. Aceto, J. Med. Chem., 2000, 43, 5030 CrossRef CAS;
(d) F. F. Fleming and Q. Wang, Chem. Rev., 2003, 103, 2035 CrossRef CAS;
(e) F. F. Fleming and Z.-Y. Zhang, Tetrahedron, 2005, 61, 747 CrossRef CAS;
(f) F. F. Fleming, L. Yao, P. C. Ravikumar, L. Funk and B. C. Shook, J. Med. Chem., 2010, 53, 7902 CrossRef CAS;
(g) R. López and C. Palomo, Angew. Chem., Int. Ed., 2015, 54, 13170 CrossRef.
- For selected examples on the stabilization of a boryl radical by ligands, see:
(a) C. Ollivier and P. Renaud, Chem. Rev., 2001, 101, 3415 CrossRef CAS;
(b) P. P. Power, Chem. Rev., 2003, 103, 789 CrossRef CAS PubMed;
(c) A. Staubitz, A. P. M. Robertson, M. E. Sloan and I. Manners, Chem. Rev., 2010, 110, 4023 CrossRef CAS;
(d) D. Lu, C. Wu and P. Li, Org. Lett., 2014, 16, 1486 CrossRef CAS PubMed;
(e) A. J. Rosenthal, M. Devillard, K. Miqueu, G. Bouhadir and D. Bourissou, Angew. Chem., Int. Ed., 2015, 54, 9198 CrossRef CAS;
(f) J. W. Taylor, A. McSkimming, C. F. Guzman and W. H. Harman, J. Am. Chem. Soc., 2017, 139, 11032 CrossRef CAS and references cited therein.
Footnote |
† Electronic supplementary information (ESI) available: Experimental procedures and characterization of new compounds are provided. See DOI: 10.1039/c8sc03315c |
|
This journal is © The Royal Society of Chemistry 2019 |
Click here to see how this site uses Cookies. View our privacy policy here.