DOI:
10.1039/C9RA07002H
(Paper)
RSC Adv., 2019,
9, 36690-36698
5-Acetamido-1-(methoxybenzyl) isatin inhibits tumor cell proliferation, migration, and angiogenesis†
Received
2nd September 2019
, Accepted 31st October 2019
First published on 11th November 2019
Abstract
Indole and its derivatives are widely distributed in both animals and plants. Among its array of biological activities, the anti-tumor activity of indole has garnered much attention. Furthermore, the synthesis and activity of indole derivatives, including isatin, constitute a flourishing research topic. Previously, many isatin derivatives were synthesized by our group, and 5-acetamido-1-(methoxybenzyl) isatin was screened as a candidate anti-tumor agent. In this study, we found that 5-acetamido-1-(methoxybenzyl) isatin inhibited the proliferation of several tumor cell lines, especially the human leukemia cell line K562. Morphological observation suggested that 5-acetamido-1-(methoxybenzyl) isatin induced apoptosis and caused cell cycle arrest in K562 cells. Flow cytometry revealed that 5-acetamido-1-(methoxybenzyl) isatin induced mitochondrial pathway-mediated apoptosis in K562 cells. Moreover, it downregulated Cyclin B and CDC25C and upregulated p-CDC25C and p-CDK1 (Thr14), and induced K562 cell cycle arrest in the G2/M phase. Findings from wound healing as well as transwell assay determined that 5-acetamido-1-(methoxybenzyl) isatin could suppress migration and chemotaxis in HepG2 liver cancer cells. 5-Acetamido-1-(methoxybenzyl) isatin also inhibited angiogenesis of the human umbilical vein endothelial cell line HUVEC, determined via a cell tube formation study. A clone formation study indicated that 5-acetamido-1-(methoxybenzyl) isatin can inhibit tumor cell proliferation and population dependence in a concentration-dependent manner. Thus, our findings support that 5-acetamido-1-(methoxybenzyl) isatin could be used as a potential antitumor candidate in future investigations.
Introduction
Cancer is a major health concern that causes prolonged morbidity and is a leading cause of mortality in humans.1,2 Indole can be found in most plants and aquatic animals,3 and reports have shown that derivatives of isatin, an indole derivative, possess a broad-spectrum of biological functionalities such as anticancer,4 anticonvulsant,5 antifungal,6 antidepressant,7 anti-HIV,8 and anti-inflammatory9 activities.
Sunitinib, which was approved by the Food & Drug Administration of the United States and marketed by Pfizer in 2006, is a small-molecule indole derivative with broad-spectrum anti-tumor effects.10 However, adverse reactions caused by Sunitinib involve multiple organs and systems in the body and have serious adverse reactions leading to death.11
Some antitumor drugs on the market have poor selectivity and strong cytotoxicity so that target cells develop resistance. Therefore, new types of high-efficiency anti-tumor drugs are urgently needed. 5-Acetamido-1-(methoxybenzyl) isatin (CAS registry number is 1561006-90-3) was designed as a potential anti-tumor agent by our laboratory and was screened from a series of 1,5-disubstitued isatin derivatives.12 The cytotoxic effect of 5-acetamido-1-(methoxybenzyl) isatin on multiple cancer cell lines and normal cells investigated the efficacy of 5-acetamido-1-(methoxybenzyl) isatin in vitro.
Caspases are a family of proteases and important regulators of apoptosis. Caspase-3 can increase the response intensity of Caspase-8 and Caspase-9, leading to complete suicide disintegration of cells. Cleavage of PARP can be caused by Caspase-3 activation during apoptosis, a key indicator enzyme of apoptosis. Though acting on the mitochondrial membrane and collapse of the mitochondrial membrane potential, Bcl-2 family proteins play an important role in apoptosis.13,14 In terms of mitochondrial apoptotic pathway regulation, Bcl-2 family proteins are crucial and include pro-apoptotic and anti-apoptotic proteins,15 which together affect cell proliferation and apoptosis.16 In this study, we examined the effect of 5-acetamido-1-(methoxybenzyl) isatin on the expression of these apoptosis-related proteins in cancer cells.
CyclinB1 was the first discovered cyclin, synthesized in the S phase and expressed in the G2/M phases.17 Its translation has been demonstrated to be critical for G2/M transition of the cell cycle.18 Abnormal expression of CyclinB1 is associated with tumor invasion, metastasis, and poor prognosis. CDK1 is a CDK family member and is active in many tumors. CyclinB1 and CDK1 begin to bind in the S/G2 phase, and CDK1 kinase activity appears. The CDC25 family (CDC25A, CDC25B and CDC25C) includes phosphatases that regulate the CDK complex and cell cycle progression. When in the M phase of the cell cycle, CDC25C enters the nucleus, catalyzes CDK1 dephosphorylation at Thr14/Tyr15, and activates the CDK1/CyclinB1 complex. But if CDC25C is abnormally expressed, dephosphorylation CDK1 at Thr14 and Tyr15 will be depressed in mitosis and the G2/M phase transition. Here, we examined the effect of 5-acetamido-1-(methoxybenzyl) isatin on the cell cycle distribution of cancer cells and on the expression of the relevant cell cycle regulatory proteins.
We also evaluated the effect of 5-acetamido-1-(methoxybenzyl) isatin on HepG2 cells and HUVECs by the wound healing assay, the transwell assay and the tube forming assay. The wound healing assay can determine the migration and repair capacity of cells. It is easy to operate, cheap, and initial developed experiment for evaluating directional migration in vitro.19 The transwell assay determines the cytotaxis, cell migration, and cell invasion capacities of cells.20,21 For the development and metastasis of tumor tissues, angiogenesis is the key. In that case, inhibiting angiogenesis is essential for the spread and deterioration of tumors.22 Using these assays, we examined the potential of 5-acetamido-1-(methoxybenzyl) isatin to be used as an effective anti-tumor agent in multiple aspects.
Materials and methods
Chemicals and cell culture
The isatin was the core structure of our drug design and 5-acetamido-1-(methoxybenzyl) isatin was synthesized in our laboratory. The structure of 5-acetamido-1-(methoxybenzyl) isatin is shown in Fig. 1A. The human leukemia cell line K562 and the human hepatic stellate cell line L-02 were purchased from Shanghai Cell Bank of Chinese Academy of Sciences (Shanghai, China) and cultured in RPMI-1640 supplemented with 10% fetal bovine serum, 2.05 mmol L-glutamine and 1% penicillin/streptomycin. The human umbilical vein endothelial cell line HUVEC was purchased from ATCC (American Type Culture Collection, USA) and maintained in Ham's F-12 medium supplemented with 10% fetal bovine serum, 2.05 mmol L-glutamine and 1% penicillin/streptomycin. The human colon cancer cell line HT-29, the human prostate cancer cell line PC-3, the liver cancer cell line HepG2, the non-small cell lung cancer cell line NCI-H460, the human breast cancer cell line MCF-7 and the human embryonic kidney cell line HEK-293 were stored in the Department of Drug Design and Synthesis, Tianjin University of Science and Technology. HepG2 cells were preserved in Dulbecco's Modified Eagle Medium (DMEM) supplemented with 10% fetal bovine serum, 2.05 mM glutamine and 1% penicillin/streptomycin. All cell lines were incubated at 37 °C in a humidified incubator containing with 5% CO2 and 2–3 days should be replaced fresh complete medium.
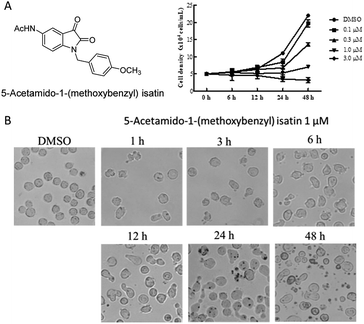 |
| Fig. 1 Morphological changes in 5-acetamido-1-(methoxybenzyl) isatin-treated K562 cells. (A) Structure and in vitro anti-cell proliferative effect of 5-acetamido-1-(methoxybenzyl) isatin. (B) Morphological changes induced by 5-acetamido-1-(methoxybenzyl) isatin treatment (1 μM). | |
Cell proliferation assay
The cell lines were taken in logarithmic growth phase and plated in 96-well culture plates at a density of 5 ×104 cells per mL. Then, cells were incubated for a while (suspended cells for 2 h and adherent cells for 24 h). After the incubation, different concentrations of 5-acetamido-1-(methoxybenzyl) isatin were added to per hole of the culture plates and incubated for 48 h. Cells were incubated for another 4 h after 48 h incubation with 5 mg mL−1 MTT (20 μL). Then, adherent cells were treated carefully by removing cell culture mediums and adding 100 μL DMSO per well to dissolved the formazan precipitate, suspension cells were treated by adding 100 μL of isopropyl hydrochloride solution into each well and mixing. MTT assay was performed using thermo microplate reader and the inhibitory concentrations (IC50) were calculated from dose–response curves of three independent assay.
Observation of cell morphology
K562 cells were cultured in 6-well plates with a density of 5 × 104 cells per mL and incubated for 2 h at 37 °C in a humidified incubator containing with 5% CO2. Then, cells were treated with 1 μM 5-acetamido-1-(methoxybenzyl) isatin for 1, 3, 6, 12, 24, 48 h and visualized by optical microscope at the corresponding time.
Quantification apoptosis by flow cytometry
The extent of apoptotic cells were monitored by the Annexin-V-FITC Apoptosis Detection Kit (Tianjin Sungene Biotech Co., Ltd.) on the basis of the manufacturer's instructions. K562 cells (5 × 104 cells per mL) were treated with DMSO or 5-acetamido-1-(methoxybenzyl) isatin (1 μM) for 0, 6, 12, 24 and 48 h respectively. After the exposure, cells were collected, washed with PBS at 461 g and gently resuspended in 1 × binding buffer. Then, the cells were incubated with Annexin-V-FITC (Annexin V-Fluorescein isothiocyanate) and PI (propidium iodide) for 10 min in dark at room temperature and evaluated by flow cytometry. Subsequently, quadrant statistics was used to analyze the fraction of cell population in different quadrants.
Measurement of mitochondrial membrane potential
Mitochondria are the key subcellular organelles for many anti-cancer drugs to induce apoptosis and the loss of mitochondrial membrane potential is a major factor in the mitochondrial induced apoptosis pathway.23 To detect whether the mitochondrial pathway is involved in apoptosis induced by 5-acetamido-1-(methoxybenzyl) isatin, K562 cells (5 × 104 cells per mL) were seeded in six-well plates and allowed to grow 2 h, then treated with DMSO or 5-acetamido-1-(methoxybenzyl) isatin (1 μM) for 0, 6, 12, 24 and 48 h respectively. Washed cells with PBS and stained with 100 nM tetramethylrhodamine methyl ester (TMRM; Invitrogen, USA), then left it in the dark for 15 min at room temperature. At the end, distinction of mitochondrial membrane potential was analyzed by flow cytometry in the FL-2 channel.
Assessing cell cycle by PI staining
To determine cell cycle distribution, K562 cells (5 × 104 cells per mL) were exposed to 5-acetamido-1-(methoxybenzyl) isatin (1 μM) for 0, 6, 12, 24 and 48 h respectively. After the treatment, K562 cells were separated by centrifugation and fixed with 1 mL of 75% ice-cold ethanol at −20 °C overnight. Then, collected the cells and washed them with PBS, incubated with 50 μg mL−1 propidium iodide (PI), 100 μg mL−1 RNase A and 0.2% Triton × 100 for 30 min protected from light at room temperature. A FACS Calibur system (version 2.0, BD) using the CELLQuest program (Becton Dickinson) was used to analyze cell cycle distribution.
Protein extraction and western blot analysis
Anti-caspase-3, anti-PARP, anti-Bcl-2, anti-Bcl-xl, and anti-Bax were bought from Santa Cruz Biotechnology (Santa Cruz, CA). Other anti-antibodies were obtained from Cell Signaling Technology (CST, Boston, MA). After treatment with DMSO or 5-acetamido-1-(methoxybenzyl) isatin for 24 h, cells were collected and lysed in lysis buffer (10 mM Hepes-Na, 150 mM Na2SO4, 1 mM EDTA, 3% CHAPS, 1 mM phenylmethylsulfonyl fluoride, 10 mg mL−1 aprotinin and 10 mg mL−1 leupeptin). The lysates were harvested by centrifuged at 20
376g at 4 °C for 10 min. After the determination of protein concentrations of cell lysates, 20 μL of each protein sample were taken and added 4 μL of 6× SB buffer and heated at 100 °C for 5 min. The samples were separated by SDS-PAGE and electrotransferred to PVDF membranes (Millipore, Bedford, MA). The membranes were probed with the antibodies and incubated with Alexa Fluor® 680 Goat Anti-Mouse IgG and Alexa Fluor® 680 Goat Anti-Rabbit IgG followed by detection using the Odyssey western blotting detection system (Amersham Pharmacia Biotech).24
Migration assay
Wound healing assay – HepG2 cells (5 × 104 cells per mL) were seeded in 6-well plates and incubated for 24 h. Then, the HepG2 cells were scratched with a 10 μL pipette tip to form straight lines as wounds. The cells were washed for three times by 1 × PBS and covered with fresh medium with DMSO or 5-acetamido-1-(methoxybenzyl) isatin (0.01 μM, 0.1 μM, 1 μM) for 0, 12, 24, and 48 h respectively. Then, assay plates were incubated at 37 °C and 5% CO2 atmosphere to permit cell migration. Photos were captured by a Nikon Eclipse Ti microscope (Nikon, Tokyo, Japan).
Transwell assay – HepG2 cells were planted in 24-well culture plates containing polycarbonate filter inserts (EMD Millipore corporation, Germany). The HepG2 cells were suspended in Dulbecco's modified Eagle's medium without fetal bovine serum with a density of 5 × 104 cells per mL and 200 μL (2 × 105 cells per well) were added to the upper compartment of the chamber. 600 μL Dulbecco's modified Eagle's medium supplemented with 10% fetal bovine serum was added to the lower chamber. After 30 minutes, the cells were exposed by DMSO or 5-acetamido-1-(methoxybenzyl) isatin (0.01 μM, 0.1 μM, 1 μM) and incubated for 24 h in 5% CO2 atmosphere at 37 °C to allow for migration across polycarbonate film. After 24 h, medium in the chamber was removed and the well of the HepG2 cells was washed for three times by 1 × PBS, fixed with methanol and then stained by DAPI. HepG2 cells remaining on the upper side of the filter were then voidede. The photographs of three horizons were taken in random. The migrated HepG2 cells to the lower side of the filter were counted. Finally, the migrated HepG2 cells were observed and photographed by the fluorescence microscope (Nikon, Tokyo, Japan) and five fields of view were record randomly.
Tube forming assay
The capillary-like network formation of HUVEC cells was investigated with Matrigel-coated 96-well culture plates. Matrigel (13.9 mg mL; BD Bioscience, San Jose, CA, USA) was thawed at 4 °C and 50 μL Matrigel was added to each well of the 24-well culture plate. Then, the assay plate incubated for 30 minute at 37 °C for polymerization. The HUVEC cells (1 × 105 cells per well) were suspended in Ham's F-12 medium containing 10% FBS, and were seeded to the wells. Cells were incubated at 37 °C in 5% CO2 for 40 minutes and then treated by DMSO or 5-acetamido-1-(methoxybenzyl) isatin (0.01 μM, 0.1 μM, 1 μM). After 12 h of culture, the formation of capillary-like tubes in each well of the culture plate was photographed by a Nikon Eclipse Ti microscope (Nikon, Tokyo, Japan).
Clone formation assay
The cell cloning rate, that is, the cell seeding survival rate, indicated that the adherent cells survived and the number of colonies formed after the cells were seeded.25 Clone formation rate reflected two important characteristics of cell proliferation and population dependence.26 Therefore, cell clone formation assay can be used to evaluate the ability of compounds to inhibit tumor cell proliferation and population dependence, it can also be used to evaluate the ability of cells to generate tumors in vivo.27
HepG2 cells were planted at a density of 1000 cells per mL in 6-well plates, with 2 mL of each well. After 24 h incubation, added 10 μL of 5-acetamido-1-(methoxybenzyl) isatin at different concentrations (0.1 μM, 0.2 μM, 0.4 μM, 0.8 μM) to each well and set the control group. The culture was terminated on the seventh day after treatment by 5-acetamido-1-(methoxybenzyl) isatin. Cell culture supernatant was discarded and cells were washed with PBS. Then, fixed the cells with 1 mL methanol at room temperature. After fixing cells for 30 min, 0.1% crystal violet was used to stain the cells. Then, supernatant of 0.1% crystal violet was abandoned and cells were carefully washed with PBS. Three random regions of each well were photographed and the number of clones formed was counted by ImageJ.
Results
Antiproliferation activities of indole derivatives
To study the effect of 5-acetamido-1-(methoxybenzyl) isatin on tumor cell proliferation, we selected six tumor cell lines originating from different tissues to perform anti-tumor spectrum testing. As shown in Table 1, 5-acetamido-1-(methoxybenzyl) isatin significantly inhibited the proliferation of human liver cancer HepG2 cells, human colon cancer HT-29 cells, and human prostate cancer PC-3 cells. The highest anti-tumor activity was observed in K562 cells, with an IC50 of 0.29 μM. These results indicate that 5-acetamido-1-(methoxybenzyl) isatin exerted potent anticancer activity against a broad spectrum of cancers.
Table 1 Antiproliferation of 5-acetamido-1-(methoxybenzyl) isatin on tumor cell lines in vitro
Cell line |
IC50 (μM) |
CPT |
5-Acetamido-1-(methoxybenzyl) isatin |
Human leukemia (K562) |
0.08 ± 0.004 |
0.29 ± 0.003 |
Human prostate cancer (PC-3) |
1.49 ± 0.08 |
0.38 ± 0.06 |
Liver cancer (HepG2) |
0.07 ± 0.02 |
0.42 ± 0.08 |
Human breast cancer (MCF-7) |
0.37 ± 0.05 |
3.37 ± 0.86 |
Non-small cell lung cancer (H460) |
0.04 ± 0.002 |
28.40 ± 14.40 |
Human colon cancer (HT-29) |
0.04 ± 0.02 |
34.37 ± 6.30 |
Next, we tested the toxicity of 5-acetamido-1-(methoxybenzyl) isatin toward normal cells, 5-acetamido-1-(methoxybenzyl) isatin showed lower toxicity than the positive control camptothecin (CPT) (Table 2).
Table 2 Toxicity of 5-acetamido-1-(methoxybenzyl) isatin on normal cells in vitro
Cell line |
IC50 (μM) |
CPT |
5-Acetamido-1-(methoxybenzyl) isatin |
Human hepatic stellate (L02) |
0.01 ± 0.002 |
5.12 ± 0.64 |
Human umbilical vein endothelial (HUVEC) |
0.25 ± 0.03 |
8.22 ± 1.36 |
Human embryonic kidney (HEK-293) |
0.54 ± 0.27 |
17.56 ± 5.07 |
After treatment with 5-acetamido-1-(methoxybenzyl) isatin (0.1, 0.3, 1.0, and 3.0 μM) for 48 h, K562 cells continued to grow but the cell growth rate was significantly lower than that in the control group. This result suggested that the inhibitory effect of 5-acetamido-1-(methoxybenzyl) isatin on the proliferation of K562 cells was dependent on both time and concentration (Fig. 1A).
Morphological changes in 5-acetamido-1-(methoxybenzyl) isatin-treated K562 cells
As shown in Fig. 1B, because of treatment by 5-acetamido-1-(methoxybenzyl) isatin (0.1 μM) for 1, 3, 6, 12, 24 and 48 h, K562 cells presented representative apoptotic morphology (cell shrinkage and/or blebbing). As opposed to DMSO-treated cells, the proportion of elongated cells among 1 μM 5-acetamido-1-(methoxybenzyl) isatin-treated cells increased as early as 6 h after treatment, and the changes in cell morphology occurred in a time-dependent manner. Therefore, we conducted further investigation to confirm whether inhibition of K562 cell proliferation was due to the effects of the compound on apoptosis and cell cycle.
5-Acetamido-1-(methoxybenzyl) isatin induced apoptosis in K562 cells
5-Acetamido-1-(methoxybenzyl) isatin induced apoptosis of K562 cells was quantitatively determined by flow cytometric analysis of cells double-labeled with Annexin V-FITC and PI. After treatment with 1 μM 5-acetamido-1-(methoxybenzyl) isatin for 6, 12, 24 and 48 h, significant apoptosis and increased number of apoptotic K562 cells were observed compared with those in control (Fig. 2A). The total apoptosis rate in K562 cells treated with 1 μM 5-acetamido-1-(methoxybenzyl) isatin for 48 h reached 51.1% (compared with 5.1% in control). Thus, we can conclude that 1 μM 5-acetamido-1-(methoxybenzyl) isatin inhibits K562 cell proliferation by inducing the apoptotic pathway and the apoptosis rate increased in a time-dependent manner.
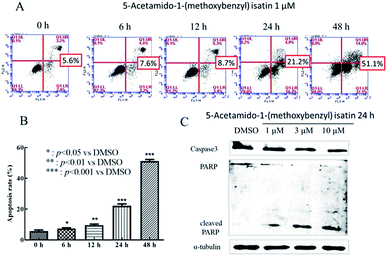 |
| Fig. 2 5-Acetamido-1-(methoxybenzyl) isatin induced apoptosis in K562 cells. (A) Flow cytometric histograms. K562 cells were treated with 1 μM 5-acetamido-1-(methoxybenzyl) isatin for 0, 6, 12, 24, 48 h and were labeled with Annexin V-FITC and PI. (B) Columns show mean values of three experiments (±SD). (C) The effect of 5-acetamido-1-(methoxybenzyl) isatin on the expression of caspase-3, PARP, and the cleavage of substrate PARP proteins. K562 cells were treated with 5-acetamido-1-(methoxybenzyl) isatin at 1, 3, 10 μM for 24 h. α-Tubulin was used as an equal loading control. | |
To further study the effect of 5-acetamido-1-(methoxybenzyl) isatin on K562 apoptosis and the molecular mechanism of apoptosis induction, changes in the expression of apoptosis-related proteins were investigated. After treatment of K562 cells with 1, 3 and 10 μM 5-acetamido-1-(methoxybenzyl) isatin for 24 h, total cellular protein was extracted.
As shown in Fig. 2C, compared with that in control, Caspase-3 activation increased gradually as the concentration of 5-acetamido-1-(methoxybenzyl) isatin increased, 89 kDa and 24 kDa fragmentation products likely resulted from PARP cleavage induced by the activated Caspase 3. Furthermore, 5-acetamido-1-(methoxybenzyl) isatin activated PARP in a concentration-dependent manner. These results demonstrate that 5-acetamido-1-(methoxybenzyl) isatin induced Caspase-3 activation and modulated the expression of apoptosis-related proteins, thereby indicating that 5-acetamido-1-(methoxybenzyl) isatin induced apoptosis in K562 cells.
Mitochondrial membrane potential measurement
Mitochondria play a significant role in the apoptotic pathway in various cancer cell lines.28 To examine mitochondrial involvement in 5-acetamido-1-(methoxybenzyl) isatin-induced apoptosis, K562 cells were treated with DMSO or 5-acetamido-1-(methoxybenzyl) isatin (1 μM) for 0, 6, 12, 24 and 48 h respectively, then stained with TMRM.
Changes in the mitochondrial membrane potential of K562 cells are shown in Fig. 3A, with a significant decline in mitochondrial membrane potential from 96.9% to 93.5% at 6 h, to 94.3% at 12 h, to 80% at 24 h and to 48% at 48 h. These results indicated that 5-acetamido-1-(methoxybenzyl) isatin can cause a decrease in the mitochondrial membrane potential, which may induce apoptosis in K562 cells through the mitochondrial pathway.
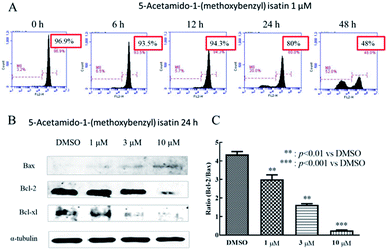 |
| Fig. 3 Mitochondrial membrane potential of K562 cells after treatment with 5-acetamido-1-(methoxybenzyl) isatin. (A) K562 cells were treated with DMSO or 5-acetamido-1-(methoxybenzyl) isatin (1 μM) for 0, 6, 12, 24, and 48 h, respectively, and then stained with 100 nM TMRM and analyzed by flow cytometry. (B) Western blot analysis of Bax, Bcl-2, and Bcl-xl proteins: K562 cells were treated with 5-acetamido-1-(methoxybenzyl) isatin at 1, 3, and 10 μM for 24 h, and α-tubulin was used as an equal loading control. (C) The effect of 5-acetamido-1-(methoxybenzyl) isatin on apoptosis-related proteins in K562 cells. | |
Expression of apoptotic mitochondrial pathway-associated proteins was further detected by western blotting. As shown in Fig. 3C, unlike in the control group, the expression of the anti-apoptotic proteins Bcl-2 and Bcl-xl was down-regulated, whereas the expression of the pro-apoptotic protein Bax was up-regulated following 5-acetamido-1-(methoxybenzyl) isatin treatment in a concentration-dependent manner. As shown in Fig. 3B, as the concentration of 5-acetamido-1-(methoxybenzyl) isatin increased, the Bcl-2/Bax expression ratio decreased. These results suggested that these three proteins associated with mitochondrial apoptosis pathways (Bcl-2, Bcl-xl, and Bax) are involved in the cell apoptosis process in 5-acetamido-1-(methoxybenzyl) isatin-treated K562 cells, indicating that 5-acetamido-1-(methoxybenzyl) isatin induces apoptosis in K562 cells through the mitochondrial apoptosis pathway.
5-Acetamido-1-(methoxybenzyl) isatin induced G2/M cell cycle phase arrest in K562 cells
DNA content was measured by flow cytometry to evaluate the cell cycle distribution of K562 cells with or without 5-acetamido-1-(methoxybenzyl) isatin (1 μM) treatment. As shown in Fig. 4A, after exposure to 5-acetamido-1-(methoxybenzyl) isatin for 1, 3, 6, 12, 24 and 48 h, the percentage of K562 cells in the G2/M phase continued to increase. At the outset, only 1.86% cells were in the G2/M phase, but as the treatment time increased, the proportion of cells in the G2/M phase increased gradually until 12 h, when the G2/M phase cell proportion peaked at 52.48%. These results indicate that 5-acetamido-1-(methoxybenzyl) isatin possesses cell proliferation inhibitory activity and can induce cell cycle arrest of K562 cells in the G2/M phase.
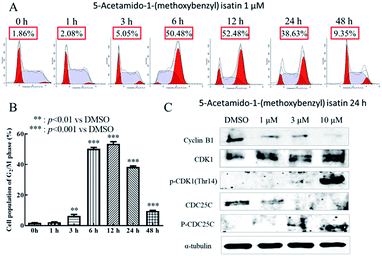 |
| Fig. 4 Effect of 5-acetamido-1-(methoxybenzyl) isatin on cell cycle of K562 cells. (A) K562 cells were treated with 5-acetamido-1-(methoxybenzyl) isatin (1 μM) for 0, 1, 3, 6, 12, 24, and 48 h, respectively and then stained with PI and analyzed by flow cytometry. (B) Bars show mean values of three experiments (±SD). (C) 5-Acetamido-1-(methoxybenzyl) isatin (1, 3, and 10 μM) gradually decreased the expression of Cyclin B and CDC25C, while gradually increasing the expression of p-CDK1 (Thr14) and p-CDC25C. | |
The expression of cell cycle-related proteins was analyzed by western blotting. The results are shown in Fig. 4C. After 24 h treatment with 5-acetamido-1-(methoxybenzyl) isatin, the total CDK1 expression in cells did not change significantly despite an increase in the compound concentration, but the expression of Cyclin B and CDC25C gradually decreased. Simultaneously, the expression of p-CDK1 (Thr14) and p-CDC25C increased gradually. Their protein levels were significantly different from those in the negative control group. These results indicate that 5-acetamido-1-(methoxybenzyl) isatin affects the expression of Cyclin B, p-CDC25C, p-CDK1 (Thr14), and CDC25C, and arrests K562 cells in the G2/M phase.
5-Acetamido-1-(methoxybenzyl) isatin inhibits HepG2 cell migration and chemotaxis
5-Acetamido-1-(methoxybenzyl) isatin exerted significant effects on the proliferation of HepG2 cells and exhibited antitumor activity at 0.42 μM after 48 h treatment. In order to further evaluate the diversity of the anti-tumor activity by 5-acetamido-1-(methoxybenzyl) isatin, the migration and chemotaxis ability of HepG2 cells was examined using wound healing and transwell assays.
After HepG2 cells were wounded, they were treated with 5-acetamido-1-(methoxybenzyl) isatin (0.01, 0.1, and 1 μM) for 0, 12, 24, and 48 h respectively. As shown in Fig. 5, 5-acetamido-1-(methoxybenzyl) isatin significantly inhibited the lateral migration and repair of HepG2 cells within a certain concentration range. As shown in Fig. 6, 5-acetamido-1-(methoxybenzyl) isatin (0.01, 0.1, and 1 μM) also markedly inhibited the migration of HepG2 cells, with similar potency as that demonstrated in the wound healing assay.
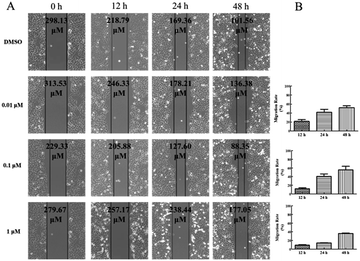 |
| Fig. 5 Effect of 5-acetamido-1-(methoxybenzyl) isatin on HepG2 cell migration assessed using a wound healing assay. (A) HepG2 cells were wounded and then treated with 5-acetamido-1-(methoxybenzyl) isatin (0.01, 0.1, and 1 μM) for 0, 12, 24, and 48 h respectively. Lateral migration and repair of HepG2 cells within a certain concentration range significantly inhibited by 5-acetamido-1-(methoxybenzyl) isatin. (B) Bars show mean values of three experiments (±SD). | |
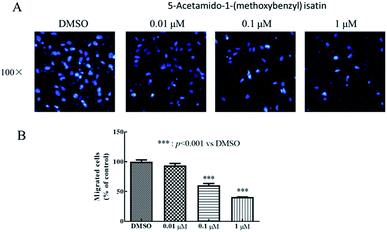 |
| Fig. 6 Effect of 5-acetamido-1-(methoxybenzyl) isatin on HepG2 cell migration assessed using the transwell assay. (A) HepG2 cells were treated with DMSO or 5-acetamido-1-(methoxybenzyl) isatin (0.01, 0.1, and 1 μM). After 24 h, medium was removed, and the cells were fixed with methanol and then stained with DAPI. HepG2 cells remaining on the upper side of the filter were then voided. The photographs of three horizons were captured in random. (B) Bars show mean values of three experiments on cells treated with 5-acetamido-1-(methoxybenzyl) isatin (±SD). | |
5-Acetamido-1-(methoxybenzyl) isatin suppresses tube formation in HUVECs
HUVECs were stimulated with several concentrations of 5-acetamido-1-(methoxybenzyl) isatin (0.01, 0.1 and 1 μM) for 12 h. Angiogenesis of the cells was observed under an inverted microscope to evaluate the effect of 5-acetamido-1-(methoxybenzyl) isatin. As shown in Fig. 7, DMSO did not affect tube formation by HUVECs, whereas 5-acetamido-1-(methoxybenzyl) isatin inhibited the angiogenesis in a dose-dependent manner.
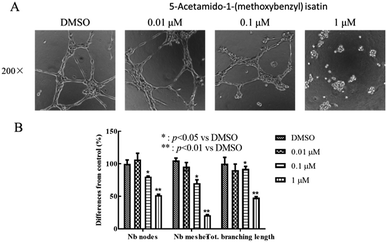 |
| Fig. 7 Suppressive effects of different concentrations of 5-acetamido-1-(methoxybenzyl) isatin on tube formation of HUVECs. (A) HUVECs cultured on matrigel-coated plates were treated with the indicated concentrations of 5-acetamido-1-(methoxybenzyl) isatin (0.01, 0.1, and 1 μM) for 12 h. Tube formation was observed by microscopy, and representative images are shown. (B) Bars show mean values of three experiments on 5-acetamido-1-(methoxybenzyl) isatin-treated HUVECs compared with the control (±SD). | |
5-Acetamido-1-(methoxybenzyl) isatin inhibits colony formation of HepG2 cells
After treatment with 0.1, 0.2, 0.4, and 0.8 μM of 5-acetamido-1-(methoxybenzyl) isatin for 7 days, we observed (Fig. 8A) that as the concentration of the 5-acetamido-1-(methoxybenzyl) isatin increased, the number of colonies formed by HepG2 cells decreased significantly. We then used ImageJ to calculate the number of clones formed. As shown in Fig. 8B, the higher the compound concentration, the lower was the number of clones formed. The results indicate that 5-acetamido-1-(methoxybenzyl) isatin inhibits tumor cell proliferation and population dependence in a concentration-dependent manner.
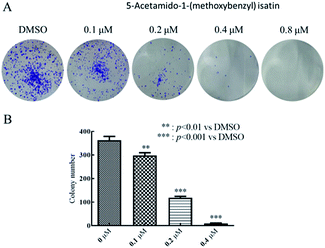 |
| Fig. 8 Effect of 5-acetamido-1-(methoxybenzyl) isatin on colony formation by HepG2 cells. (A) HepG2 cells were treated with 0.1, 0.2, 0.4, and 0.8 μM 5-acetamido-1-(methoxybenzyl) isatin for 7 days and photographed. As the concentration of 5-acetamido-1-(methoxybenzyl) isatin increased, the number of colonies formed by HepG2 cells decreased significantly. (B) 5-Acetamido-1-(methoxybenzyl) isatin inhibited tumor cell proliferation and population dependence in a concentration-dependent manner. ImageJ was used to calculate the number of clones formed. | |
Discussion
Cancer is one of the most serious diseases and the number of cancer patients continues to increase rapidly. The adverse reactions and resistance of existing drugs have resulted in a necessity for novel drugs against cancer. In addition to surgery and radiation therapy, developing targeted anti-tumor drugs will be a far-researching milestone.
Some studies indicate that the use of oxindole as a lead compound to synthesize and screen anti-tumor drugs with novel structure, stronger targeting, and stronger selectivity has broad research prospects. In 2010, Tang Peng Cho synthesized a series of potent vascular inhibitors by introducing a pyrrole-cyclocaprolactam structure at the 3-position of indole. Among these, two compounds showed good pharmacokinetics and significant effects on human colon cancer HT-29 cells transplanted in nude mice.29 In 2012, Azizian Javad synthesized a series of compounds using isatin as a starting material, and tested three human cancer cell lines to screen a highly active compound based on cytotoxicity. Formation of a lipid-soluble ring containing a hydrogen bond between the receptor and the 3-position of indole appears to be an important reason for this series of compounds to be effective VEGFR-2 inhibitors.30 In 2014, Z Ma found that the endogenous oxindole isatin could induce apoptosis in the human breast cancer cell line, MCF7, through the mitochondrial pathway, with a significant decrease in the expression of Bcl-2 and Bcl-2/Bax in cells.20
Our previous studies have revealed that 5-acetamido-1-(methoxybenzyl) isatin possesses excellent cytotoxic activity against K562 cells (IC50 = 0.29 μM). In addition, it also revealed inhibition of HepG2 cells, HT-29 cells and PC-3 cells to some extent (Table 2). These findings indicate that 5-acetamido-1-(methoxybenzyl) isatin possesses superior and broad-spectrum anticancer action against various cancers. Moreover, 5-acetamido-1-(methoxybenzyl) isatin showed lower toxicity toward normal cells than CPT. Morphological analysis indicats that 5-acetamido-1-(methoxybenzyl) isatin acted by inducing apoptosis and causing cell cycle arrest. Typical apoptotic morphology occurred in K562 cells after the treatment with 5-acetamido-1-(methoxybenzyl) isatin.31
Mitochondrial dysfunction often triggers some cellular signaling to induce apoptosis.32 Flow cytometry analysis showed that exposure to 5-acetamido-1-(methoxybenzyl) isatin significantly induced apoptosis in K562 cells, as the result of mitochondrial membrane potential collapsion. Simultaneously, 5-acetamido-1-(methoxybenzyl) isatin induced Caspase-3 activation and cleavage of its substrate PARP. After treatment with 5-acetamido-1-(methoxybenzyl) isatin, Bax was up-regulated but Bcl-2 and Bcl-xl were down-regulated. These findings indicate that 5-acetamido-1-(methoxybenzyl) isatin induced mitochondrial pathway-mediated apoptosis in K562 cells.
The cellular DNA content was measured by flow cytometry to evaluate the cell cycle distribution of K562 cells. 5-Acetamido-1-(methoxybenzyl) isatin induced cell cycle arrest of K562 cells in the G2/M phase by 12 h of treatment. Cell cycle-related protein indicated that the expression of p-CDK1 (Thr14) and p-CDC25C increased gradually, compared with negative control group. These results indicate that 5-acetamido-1-(methoxybenzyl) isatin could arrest the K562 cells in the G2/M phase by affecting the expression of cycle-related proteins.
Beyond proliferation, cell migration, directional chemotaxis, and complex tube formation are all critical, distinct, and functional components of tumor-associated angiogenesis. Wound healing and transwell experiments showed that inhibition ability of wound repairment, chemotaxis, and migration of HepG2 cells was depended on the concentration of 5-acetamido-1-(methoxybenzyl) isatin. Cell tube formation assay revealed that 5-acetamido-1-(methoxybenzyl) isatin could dose-dependently inhibit the angiogenesis of HUVECs.
Furthermore, clone formation assay expressed that 5-acetamido-1-(methoxybenzyl) isatin inhibited tumor cell proliferation and population dependence in a concentration-dependent manner.
These data support the potential application of 5-acetamido-1-(methoxybenzyl) isatin as a chemotherapeutic agent against human leukemia and human liver cancer. However, other pivotal signaling pathways induced by 5-acetamido-1-(methoxybenzyl) isatin and its antitumor pharmacological activity in vivo need to be examined in future studies.
Conclusions
The present study revealed that 5-acetamido-1-(methoxybenzyl) isatin induces apoptosis in K562 cells via the mitochondrial pathway and arrests cells in the G2/M phase by down-regulating Cyclin B and CDC25C and up-regulating p-CDC25C and p-CDK1 (Thr14). Findings on the effect of the indole derivative 5-acetamido-1-(methoxybenzyl) isatin on the migration, angiogenesis, and clone formation of cancer cells also provide a strong foundation for further studies on the antitumor activity and mechanism of action of indole compounds.
In sum up, malignant tumors seriously influence human health and put pressure on life, targeted drugs can reduce the mortality of cancer patients, and the space for demand is huge. Fortunately, the present findings prove that 5-acetamido-1-(methoxybenzyl) isatin exerts an anticancer effect in vitro. There is no doubt that in vivo research is essential, so we are preparing for further research.
Conflicts of interest
The authors declare that there are no conflicts of interest.
Acknowledgements
This work was supported jointly by “Young and middle-aged backbone innovative talents training plan” of Tianjin universities, International Science & Technology Cooperation Program of China (2013DFA31160). We would like to thank Editage for English language editing.
References
- W. Chen, R. Zheng, P. D. Baade, S. Zhang, H. Zeng, F. Bray, A. Jemal, X. Q. Yu and J. He, Ca-Cancer J. Clin., 2016, 66, 115–132 CrossRef.
- F. Bray, J. Ferlay, I. Soerjomataram, R. L. Siegel, L. A. Torre and A. Jemal, Ca-Cancer J. Clin., 2018, 68, 394–424 CrossRef PubMed.
- Q. Zhang, Y. Teng, Y. Yuan, T. Ruan, Q. Wang, X. Gao, Y. Zhou, K. Han, P. Yu and K. Lu, Eur. J. Med. Chem., 2018, 156, 800–814 CrossRef CAS.
- A. K. Rathi, R. Syed, V. Singh, H. S. Shin and R. V. Patel, Recent Pat. Anti-Cancer Drug Discovery, 2017, 12, 55–72 CrossRef CAS.
- C. F. Liu, H. J. Zhang and Z. S. Quan, Lett. Drug Des. Discovery, 2016, 13, 833–839 CrossRef CAS.
- M. Aman and R. V. Ravishankar, Biocontrol Sci. Technol., 2016, 26, 476–491 CrossRef.
- S. Wang, Y. Chen, X. Liu, X. Xu, X. Liu, B. F. Liu and G. Zhang, Arch. Pharm., 2014, 347, 32–41 CrossRef CAS.
- P. Ashok, C. L. Lu, S. Chander, Y. T. Zheng and S. Murugesan, Chem. Biol. Drug Des., 2015, 85, 722–728 CrossRef CAS.
- M. Kale, A. Narute and T. Kalyankar, Clin. Anti-Inflammatory Anti-Allergy Drugs, 2014, 1, 39–44 CrossRef CAS.
- R. J. Motzer, B. Escudier, A. Gannon and R. A. Figlin, Oncologist, 2017, 22, 41–52 CrossRef CAS.
- A. P. Zhou, Y. Bai, Y. Song, H. Luo, X. B. Ren, X. Wang, B. Shi, C. Fu, Y. Cheng, J. Liu, S. Qin, J. Li, H. Li, X. Bai, D. Ye, J. Wang and J. Ma, Oncologist, 2019, 24, e702–e708 CrossRef CAS.
- P. Yu, F. X. Liu, H. Sun, Preparation of 1,3-substituted-5-acetamidoindolinone compounds as antitumor drugs, China, CN103554008A, 2014-02-05.
- K. Tong, C. Xin and W. Chen, Oncol. Lett., 2016, 13, 518 CrossRef.
- P. K. Raghav, R. Kumar, V. Kumar and G. Raghava, Mol. Genet. Genomic Med, 2019, e910 Search PubMed.
- Y. Yan, R. Xie, Q. Zhang, X. Zhu, J. Han and R. Xia, Platelets, 2019, 30, 75–80 CrossRef CAS.
- C. T. Peng, D. S. Yi, J. Feng, H. F. Jian, L. Y. Jiang, L. Xiao, H. P. Jiang, L. L. Ya, L. Zhang and B. Hu, J. Med. Chem., 2010, 53, 8140 CrossRef.
- B. Liu, Y. Liu, Y. Wang, C. Xie, M. Gan, T. Han, J. Cao and J. Wang, Pathol., Res. Pract., 2019, 215, 152592 CrossRef.
- J. C. Xu, X. P. Zhou, X. A. Wang, M. D. Xu, T. Chen, T. Y. Chen, P. H. Zhou and Y. Q. Zhang, J. Cancer, 2019, 10, 2415–2424 CrossRef.
- L. G. Rodriguez, X. Wu and J. L. Guan, Methods Mol. Biol., 2005, 294, 23–29 Search PubMed.
- Z. Ma, L. Hou, Y. Jiang, Y. Chen and J. Song, Oncol. Rep., 2014, 32, 2111–2117 CrossRef CAS.
- X. Chang, X. Zhao, J. Wang, S. Ding, L. Xiao, E. Zhao and X. Zheng, Adv. Anticancer Agents Med. Chem., 2019 DOI:10.2174/1871520619666191023094610.
- T. Nomura, K. Hirata, T. Shimaoka, M. Yamakawa, N. Koizumi, R. Suzuki, K. Maruyama and N. Utoguchi, Biol. Pharm. Bull., 2017, 40, 1661–1668 CrossRef CAS.
- S. Hua, Y. Zhang, P. Wang, W. Dong, Z. Qian, Y. Yuan and Y. Teng, J. Tianjin Univ. Sci. Technol., 2014, 24, 591–594 Search PubMed.
- Y. Teng, L. Wang, H. Liu, Y. Yuan, Q. Zhang, M. Wu, L. Wang, H. Wang, Z. Liu and P. Yu, Chem.-Biol. Interact., 2017, 261, 103–107 CrossRef CAS.
- C. M. Yeh, J. Shay, T. C. Zeng, J. L. Chou, T. H. Huang, H. C. Lai and M. W. Chan, Int. J. Oncol., 2014, 45, 2101–2107 CrossRef CAS.
- Z. Wang, Y. I. Gao, Y. Liu, J. Chen, J. Wang, S. Gan, X. U. Danfeng and X. Cui, Int. J. Mol. Med., 2015, 36, 931–938 CrossRef CAS.
- C. P. Cheng, L. C. Huang, Y. L. Chang, C. H. Hsieh, S. M. Huang and D. Y. Hueng, OncoTargets Ther., 2016, 7, 41460–41472 Search PubMed.
- K. Tong, C. Xin and W. Chen, Oncol. Lett., 2016, 13, 518 CrossRef.
- T. P. Cho, S. Y. Dong, F. Jun, F. J. Hong, Y. J. Liang, X. Lu, P. J. Hua, L. Y. Li, Z. Lei and H. Bing, J. Med. Chem., 2010, 53, 8140 CrossRef.
- J. Azizian, K. M. Mohammadi, O. Firuzi, N. Razzaghiasl and R. Miri, Med. Chem. Res., 2011, 21, 3730–3740 CrossRef.
- B. Choudhury, R. Kandimalla, R. Bharali, J. Monisha, A. B. Kunnumakara, K. Kalita and J. Kotoky, Front. Pharmacol., 2016, 7, 3 Search PubMed.
- P. L. Ong, B. C. Weng, F. J. Lu, M. L. Lin, T. T. Chang, R. P. Hung and C. H. Chen, Food Chem. Toxicol., 2008, 46, 1535–1547 CrossRef CAS.
Footnotes |
† Electronic supplementary information (ESI) available. See DOI: 10.1039/c9ra07002h |
‡ These authors contributed equally to this work. |
|
This journal is © The Royal Society of Chemistry 2019 |
Click here to see how this site uses Cookies. View our privacy policy here.