DOI:
10.1039/C9RA06664K
(Paper)
RSC Adv., 2019,
9, 42360-42366
The antioxidant and tyrosinase inhibition properties of essential oil from the peel of Chinese Torreya grandis Fort.
Received
24th August 2019
, Accepted 9th December 2019
First published on 20th December 2019
Abstract
The antioxidant and tyrosinase inhibition properties of essential oil from the peel of Chinese Torreya grandis Fort. (CTGF oil) were investigated. The antioxidant properties of CTGF oil were evaluated via 2,2-diphenyl-1-picrylhydrazyl (DPPH) radical scavenging tests, and it showed an IC50 value of 0.88 ± 0.06 μg mL−1 compared to VC with a value of 1.0 ± 0.1 μg mL−1 and BHT with a value of 2.9 ± 0.1 μg mL−1. CTGF oil had relatively significant DPPH scavenging activity (p < 0.05), which could be compared to other natural oils as follows: cassia oil (92.4%) > peppermint oil (89.1%) > clove leaf oil (87.7%) > nutmeg oil (80.1%) > CTGF oil (42.6%) > lemon oil (25.5%). Furthermore, changes in the peroxide values of different treatment groups during storage for 60 days were estimated. CTGF oil displayed better antioxidant activity than lemon oil, with activity similar to that of BHT for the reduction of the peroxide value. Moreover, CTGF oil effectively inhibited the oxidation of 3,4-dihydroxy-L-phenylalanine (L-DOPA) through tyrosinase (P < 0.05). The essential oil obtained after hydrodistillation from the peel of Chinese Torreya grandis Fort. could be potentially utilized as a good new alternative source of natural antioxidants for the food and cosmetics industries.
Introduction
Antioxidants have direct effects on the minimization of oxidative deterioration through preventing radical chain reactions, thus prolonging shelf lives and retaining nutritional properties and sensory quality. Hence, they have gained the attention of numerous researchers from the food, cosmetics and pharmaceutical industries.1–3 Currently, the most common antioxidants applied in food products are chemically synthetic antioxidants such as butylated hydroxyanisole (BHA) and butylated hydroxytoluene (BHT).4 However, the use of these synthetic antioxidants is strictly restricted by local or national legislative rules in certain places due to their potentially toxic and carcinogenic effects.5
Recently, there has been exponentially growing interest in exploring antioxidants from natural resources for further use in the food and medicine domains; one example is polyphenols, which can be extracted via mixtures of organic solvents from tea or other plants around the world, via thermal treatment from natural sources, or via microwave-assisted methods from grape seeds.6–9 Compared with polyphenols, essential oils are another main antioxidant obtained from plants in the form of secondary metabolites synthesized by all plant organs.10 They are complex mixtures consisting of diverse compounds, for example, therapeutic chemicals, plant hormones, highly volatile aromatics, etc. They can be obtained from all sorts of plant sources through different methods, such as steam distillation,11–13 organic solvents,14,15 a combination of the two aforementioned methods,16 microwaves,17 and supercritical carbon dioxide.18 It is particularly worth noting that some essential oils and/or polyphenols exhibit similar or even stronger antioxidant activities than traditional synthetic antioxidants (BHT and BHA), illustrating that natural antioxidants are good candidates for use in food preservation, pharmaceutical products, alternative medicine and natural therapies because of their health benefits.
Torreya grandis Fort. ex. Lindl. is a kind of economically useful tree widely grown in the south of China. It is generally used for construction and industrial applications. The seeds of Torreya grandis Fort. are usually processed into snack foods in China. In contrast, the thick layers of peel surrounding the surfaces of the seeds of Torreya grandis Fort. are always discarded during snack food preparation. In recent years, the annual yield of Torreya grandis Fort. seeds in Zhejiang province, China, has reached approximately 1500 tons, generating a large amount of waste peel.19 Therefore, the utilization of the peel of Chinese Torreya grandis Fort. in value-added products is a fundamentally significant area for researchers from economic and environmental points of view.
In our previous work, the chemical composition of essential oil from the peel of Chinese Torreya grandis Fort. was determined via GC and GC/MS.19 However, the bioactive properties and antioxidant properties of essential oil obtained from the peel of Chinese Torreya grandis Fort. under the optimal extraction conditions still remains unclear. The purpose of this study was to investigate the antioxidant activity and tyrosinase inhibition activity of essential oil from the peel of Chinese Torreya grandis Fort., explore the potential value of Torreya grandis pericarp, and provide some basis and ideas for the development of natural antioxidants for food and cosmetics purposes.
Materials and methods
Materials
Iodized salt (food grade) was obtained from China National Salt Industry Corporation (Shanghai, China). 2,2-Diphenyl-1-picrylhydrazyl (DPPH), 3,4-dihydroxy-L-phenylalanine (L-DOPA), and tyrosinase were purchased from Sigma-Aldrich (Shanghai, China). Diethyl ether, disodium hydrogen phosphate, monosodium phosphate, anhydrous ethyl alcohol, dimethyl sulfoxide, potassium bichromate, potassium iodide, chloroform, and acetic acid were purchased from Sinopharm (Shanghai, China). Instant cornstarch was obtained from Shanghai Rongshi (Shanghai, China). 2,6-Di-tert-butyl-4-methylphenol (BHT) and ascorbic acid (vitamin C) were purchased from Yingyuan (Shanghai, China) and Fine Chemicals Institute (Shanghai, China), respectively. All commercial essential oils were purchased from Pan'an Tianlan fragrance Co., Ltd (Zhejiang, China), manufactured via steam distillation. All commercial chemicals were used without further purification.
Preparation of plant materials
Torreya grandis Fort. fruit was generously donated by Zhaojia Town Company, (Zhuji City, Zhejiang, China). The freshly cut peel was dried to a constant weight in an electric heated air-blowing drier (104A-OS, Shanghai Jingsheng Scientific Instrument Co., Ltd, Shanghai, China) at 40 °C. Then, the dried peel was quickly crushed into powder with a size from 40 mesh to 60 mesh. Finally, the powder was collected and packed into paper bags with N2 in a desiccator at ambient temperature.
Isolation of essential oil
Essential oil from the samples was obtained using standard hydrodistillation methodology from the literature with minor modifications.11,19 3n−1 half fractional factorial design was used to determine the main factor during extraction. The selected factors in this approach were the ratio of the mass of peel to water (A), the mass of salt (B), and the treatment time (C). The extract was condensed in cooling vapour to collect the exacted oil from the peel powder. Afterwards, the essential oil was dried using purified N2. All essential oil samples were maintained at freezing temperature (−18 °C). The extraction rate of essential oil was calculated as follows: |
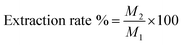 | (1) |
where M1 is the mass of essential oil from the peel of Chinese Torreya grandis Fort. (g) and M2 is the mass of dried Chinese Torreya grandis pericarp (g).
Antioxidant activity measurement
Effects of the antioxidant capacity of essential oil on lipids. 0.02 g of essential oil from the peel of Chinese Torreya grandis Fort., lemon essential oil, and BHT, respectively, were introduced to 100 g of lard oil, salad oil and palm oil, respectively. The mixed samples were stored at 60 °C in an oven for 54 days, and the samples were tested every day. 100 g of lard oil, salad oil and palm oil without the employment of antioxidants were used as controls for each experiment. Peroxide values were measured via the official AOAC method (AOAC, 1995) mentioned in some publications in terms of the ability of the peroxides20,21 formed by oil oxidation to oxidise the iodide ions of KI and free iodine, titrated using 0.01 N sodium thiosulphate solution.
DPPH assay. The hydrogen atom-or-electron donation abilities of the corresponding essential oils were measured via the change in color of a purple ethanol solution of DPPH into light yellow, following the method previously described in other publications with minor modifications.13,22 1 mL of DPPH solution (100 mM in ethanol/water, 1
:
1 v/v) was mixed vigorously with 1 mL of extracted essential oil. After 30 minutes of incubation at room temperature in the dark, the absorbance was recorded at 517 nm against a blank. For the control study, all reaction conditions were identical to the test samples except that essential oil was not introduced in the sample. Each assay was carried out in triplicate. The inhibition of the free radical DPPH, in percent, (IP%) was calculated using the following equation: |
 | (2) |
where A1 is the absorbance of the test sample, and A0 is the absorbance of the control.
Tyrosinase inhibition
The tyrosinase inhibition abilities of essential oil were determined using a modified published method with L-DOPA as the substrate, and they were compared with other tyrosinase inhibition agents: lemon essential oil, vitamin C and BHT.23 Samples were diluted in DMSO (1
:
1, v/v). Subsequently, phosphate buffer (0.1 M, pH 6.8), tyrosinase (31 units per mL) and L-DOPA (2.5 mM) were added into the diluted sample at a phosphate buffer
:
tyrosinase
:
L-DOPA
:
sample v/v ration of 2
:
1
:
1
:
1
:
1. After mixing gently, the sample was analyzed at 475 nm, using absorbance at 700 nm as a reference. The blank contained all the chemicals except L-DOPA. The percentage of tyrosinase inhibition (TIP%) was calculated as follows: |
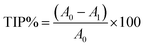 | (3) |
where A1 is the absorbance of the test sample, and A0 is the absorbance of the control.
Determination of peroxide values
0.02 g of antioxidant dissolved in 3 mL of ethyl alcohol was slowly introduced into 100 g of salad oil, lard oil and palm oil, respectively. Subsequently, samples were stored in an oven at 60 ± 2 °C for 60 days. The peroxide values of samples were regularly determined following the AOCS official method. This method evaluates all substances according to the milliequivalents of peroxide per 1000 g of sample that oxidize potassium iodide. The amount of oxidized potassium iodide was determined via a standardized sodium thiosulphate titration. Analyses were performed in duplicate.
Statistical analysis
Averages and standard deviations (SDs) from two measurements of each sample were reported. All extractions and determinations were performed in duplicate. Data are expressed as mean ± SD. Analysis of variance (ANOVA) was used for analysis of the test results (least significant difference) at the p-value < 0.05 significance level using SAS software (SAS Institute, Cary, NC, USA).
Results and discussion
Optimization of the extraction conditions for essential oils
Methods for the extraction of plant materials include the traditional approach (hydrodistillation) and novel methods (organic solvents, microwaves, and supercritical carbon dioxide). Compared with the traditional approach, the new methods exhibit apparent drawbacks, such as the utilization of expensive and hazardous solvents, labour intensive operations, and the inability to scale-up production.
In this study, hydrodistillation was applied for the extraction of essential oils, since hydrodistillation protects the extracted essential oil somewhat with the surrounding water serving as a hurdle to prevent the oil from overheating. At the same time, some studies have found that adding a small amount of inorganic salt can effectively improve the demulsification efficiency of the emulsion and increase the volatility of flavour substances.40–42 32 fractional factorial design was used to optimize the factors strongly associated with the extraction process. The matrix and results of the experiments are presented in Table 1. In this work, three factors, namely the material ratio (mass/water), mass of salt (g) and treatment time (h), were screened for their effects on the yield of essential oil (%; response). Table 1 demonstrates the factors and levels used in the 32 fractional factorial design, and the experimental range for each factor was determined from preliminary experiments. A total of 9 experimental runs were performed to complete the design. The highest yield (1.82 ± 0.02%, w/w) was achieved using a peel to water ratio of 1
:
4 with 4 g of sodium chloride for 5 h. Madhumita et al. also optimized the extraction conditions of essential oil from betel nut leaves via steam distillation. They found that the optimum extraction conditions were an extraction time of 140 min, an extraction temperature of 100 °C and a leaf to water ratio of 1
:
1.5 g L−1. The yield of betel nut leaf essential oil was only 0.48%.12
Table 1 The conditions used and results from essential oil extractions based on fractional factorial (32) experimental design
Run |
Ratio (mass/water) |
Mass of salt (g) |
Treatment time (h) |
Yielda (%) |
Data are given as mean ± SD. SD reflects the standard deviation of two replicates. |
1 |
1 : 3 |
3 |
3 |
1.23 ± 0.04 |
2 |
1 : 3 |
4 |
4 |
1.18 ± 0.03 |
3 |
1 : 3 |
5 |
5 |
1.60 ± 0.06 |
4 |
1 : 4 |
3 |
4 |
1.59 ± 0.06 |
5 |
1 : 4 |
4 |
5 |
1.82 ± 0.02 |
6 |
1 : 4 |
5 |
3 |
1.52 ± 0.07 |
7 |
1 : 5 |
3 |
5 |
0.84 ± 0.07 |
8 |
1 : 5 |
4 |
3 |
1.25 ± 0.03 |
9 |
1 : 5 |
5 |
4 |
1.64 ± 0.05 |
Antioxidant properties of essential oil from the peel of Chinese Torreya grandis Fort.
The antioxidant activity is associated with multiple mechanisms, mainly consisting of free radical scavenging, the decomposition of peroxides, the prevention of chain initiation, the prevention of continued hydrogen abstraction, the reducing capacity, and the binding of transition metal ion catalysts.24 The free radical scavenging activity is the most common mechanism related to the antioxidant activity of antioxidants.22
The free radical scavenging activities of essential oils were assessed using DPPH, as shown in Fig. 1. Essential oil from the peel of Chinese Torreya grandis Fort. can reduce the stable radical DPPH to yellow-colored diphenyl picrylhydrazine with an IC50 value of 0.88 ± 0.06 μg mL−1, compared to vitamin C with a value of 0.99 ± 0.12 μg mL−1 and BHT with a value of 2.9 ± 0.1 μg mL−1. The free radical scavenging activity of essential oil from the peel of Chinese Torreya grandis Fort. was slightly lower than that of synthetic antioxidant vitamin C but much lower than that of BHT, partly due to there being 62 kinds of compounds present in the essential oil, all having a wide variety of physical and chemical properties, accounting for a lower purity antioxidant compared to pure vitamin C and BHT.19
 |
| Fig. 1 The DPPH scavenging efficiency of essential oils obtained from different natural resources. * refers to essential oil from the peel of Chinese Torreya grandis Fort. The error bars reflect the standard deviation of two replicates. | |
To further investigate the antioxidant activity of essential oil from the peel of Chinese Torreya grandis Fort., the effects of several essential oils derived from different natural resources on DPPH scavenging efficiency are shown in Fig. 1. The order of the DPPH scavenging efficiency of different essential oils is as follows: cassia oil (92.4%) > peppermint oil (89.1%) > clove leaf oil (87.7%) > nutmeg oil (80.1%) > CTGF oil (42.6%) > lemon oil (25.5%). Except for lemon oil, all other essential oils had significant.
DPPH scavenging activity (P < 0.05). In our previous study, limonene (35.6–37.1%), α-pinene (20.1–24.1%), δ-carene (3.3–3.9%) and γ-carene (3.8–3.9%) were the major constituents of CTGF oil.19 Also of importance, other scientists have reported that limonene,29 α-pinene30 and carene31,32 exhibit good antioxidant activities, therefore enabling extracted essential oils to show antioxidant activity. However, the amounts of these antioxidant components in essential oil from the peel of Chinese Torreya grandis Fort. were not much, resulting in the DPPH scavenging efficiency of the essential oil being only 42.6%. Of note, the DPPH scavenging efficiency of essential oil from the peel of Chinese Torreya grandis Fort. was higher than those of Salvia officinalis essential oil,26 Piper carpunya (Piperaceae) essential oil27 and essential oil from Sardinian Santolina corsica Jord. & Fourr.22 Furthermore, these results from this study are comparable to earlier works on essential oil from date palm,25 the leaves of Eucalyptus globulus28 and the roots of Jatropha pelargoniifolia Courb.,39 which revealed prominent antioxidant properties.
The changes in the peroxide values of different treatment groups (CTGF oil, lemon oil, BHT and a control in lard oil, salad oil and palm oil, respectively) during storage are shown in Fig. 2. The peroxide values of all the groups in this section followed an identical trend: control > lemon oil > CTGF oil > BHT. Indeed, the antioxidant effects of different groups did not vary significantly between the groups during the initial period of storage (P > 0.05). After several days, different samples showed antioxidant effects at various degrees and levels. As expected, the peroxide values exhibited significant increases in the control samples and the samples treated with lemon oil (P < 0.05) as the storage period progressed, despite the fact that the peroxide values of the lemon-oil-treated samples in salad oil (Fig. 2(a)), lard oil (Fig. 2(b)), and palm oil (Fig. 2(c)) were slightly lower than those of the controls. Lemon oil had no significant effect on the antioxidation of salad oil when compared to the control sample, as shown in Fig. 2(b) (P > 0.05). However, all samples treated with CTGF oil and BHT showed remarkable resistance to oxidation, possessing low POVs throughout the later storage period (P < 0.001), as shown in Fig. 2. Although CTGF oil had a lower impact, to a small extent, in comparison with BHT, they both maintained significant antioxidant activity over the storage period (P < 0.05), consistent with previous results in the literature. Based on the peroxide value assays, the essential oil showed a stronger antioxidant effect when compared to the control and lemon oil groups (P < 0.05). Some researchers from Turkey have examined the antioxidant effects of essential oils from rosemary (Rosmarinus officinalis), clove (Syzygium aromaticum) and cinnamon (Cinnamomum zeylanicum).4 Their results indicated that BHA was more effective than the essential oils in retarding the lipid oxidation of crude oils;4 in addition, no antioxidative effect was exhibited during the initial phase of storage,4 highly consistent with the results in this report. Therefore, CTGF oil can be regarded as a sort of promising and effective antioxidant used for controlling lipid oxidation during storage.
 |
| Fig. 2 Changes in the peroxide values of different groups treated with BHT, CTGF oil, and lemon oil in (a) salad oil, (b) lard oil, and (c) palm oil over a storage period of 60 days in an oven at 60 ± 2 °C; BHT: unfilled triangles; CTGF oil: unfilled squares; lemon oil: filled triangles; control: filled squares. The control refers to samples treated without the employment of any antioxidant during storage; CTGF oil refers to essential oil from the peel of Chinese Torreya grandis Fort. The error bars reflect the standard deviation of two replicates. | |
The tyrosinase inhibition activity of essential oil from the peel of Chinese Torreya grandis Fort.
Tyrosinase (EC 1.14.18.1), belonging to the monophenol monooxygenase family, catalyses the hydroxylation of monophenols, including tyrosine and dopamine, to o-diphenols and can oxidize o-diphenols into o-quinones. Well-distributed tyrosinase in plant and animal tissues plays a vital role in the production of melanin.33 Mutations in the melanogenesis of tissue have assumedly been linked with malignant melanomas, cancer that is fast increasing in incidence.34 Therefore, tyrosinase inhibitors could possibly contribute to clinical cures for skin cancers and some dermatological disorders caused by melanin hyperpigmentation.35
As depicted in Fig. 3, different essential oils at 1.443 mg mL−1 were analysed for tyrosinase inhibition activity using a modified dopachrome method, with L-DOPA as the substrate. Tyrosinase inhibitory effects followed the trend vitamin C (31.95%) > CTGF oil (25.95%) > lemon oil (10.05%) > BHT (4.50%). It appeared that vitamin C and CTGF oil effectively inhibited the oxidation of L-DOPA by tyrosinase (P < 0.05). In contrast, lemon oil and BHT had relatively small effects on the tyrosinase inhibitory activity. The tyrosinase inhibitory activity of CTGF oil agreed with results obtained using Cinnamomum cassia essential oil.36
 |
| Fig. 3 The tyrosinase inhibitory activity of essential oils obtained from different resources; control: sample treated without the employment of any antioxidant; * refers to essential oil from the peel of Chinese Torreya grandis Fort.; the error bars reflect the standard deviation of two replicates. | |
Table 2 compares the Michaelis–Menten kinetics properties of tyrosinase inhibition by different antioxidants. The Michaelis constant (KM) and maximum activity (Vmax) values were determined from Lineweaver–Burk plots of tyrosinase and L-DOPA with different concentrations of antioxidants. The value of KM indicates the affinity of the enzyme (tyrosinase) toward the substrate (L-DOPA). The greater the value of KM, the lower the affinity of tyrosinase toward L-DOPA.37 As can be seen from Table 2, vitamin C showed the strongest inhibition of tyrosinase, followed by CTGF oil, lemon oil and BHT. Herein vitamin C, CTGF oil and lemon oil displayed significantly greater tyrosinase inhibition activity (P < 0.05). Conversely, BHT showed slight tyrosinase inhibition effects, highly consistent with other works.38 Therefore, CTGF oil showed strong anti-tyrosinase activity and may become a potential research area in the cosmetics industry.
Table 2 A summary of the Michaelis–Menten kinetics properties of tyrosinase inhibited by different antioxidantsa
Type |
Concentration (mg mL−1) |
KMb (mol L−1) |
Vmaxb (OD per min) |
PB: phosphate buffer solution; DMSO: dimethyl sulfoxide; CTGF oil: essential oil from the peel of Chinese Torreya grandis Fort.; BHT: butylated hydroxy toluene; Vc: vitamin C; NA: not available. Data are given as mean ± SD, SD reflects the standard deviation of two replicates. |
Control (PB)* |
NA |
8.76 × 10−4 ± 0.06 × 10−4 |
1.13 × 10−1 ± 0.08 × 10−1 |
Control (DMSO)** |
NA |
4.17 × 10−4 ± 0.03 × 10−4 |
7.72 × 10−2 ± 0.03 × 10−2 |
CTGF oil |
1.00 |
1.61 × 10−3 ± 0.02 × 10−3 |
1.09 × 10−1 ± 0.07 × 10−1 |
0.50 |
1.74 × 10−3 ± 0.09 × 10−3 |
9.93 × 10−2 ± 0.07 × 10−2 |
0.25 |
3.03 × 10−2 ± 0.06 × 10−2 |
1.13 ± 0.03 |
BHT |
1.00 |
8.75 × 10−4 ± 0.01 × 10−4 |
8.57 × 10−2 ± 0.09 × 10−2 |
0.50 |
1.22 × 10−3 ± 0.01 × 10−3 |
1.04 × 10−1 ± 0.05 × 10−1 |
0.25 |
5.51 × 10−3 ± 0.09 × 10−3 |
2.43 × 10−1 ± 0.02 × 10−1 |
Vc |
1.00 |
1.89 × 10−2 ± 0.03 × 10−2 |
7.51 × 10−3 ± 0.06 × 10−3 |
0.50 |
5.85 ± 0.07 |
1.49 ± 0.07 |
0.25 |
190.60 ± 0.03 |
22.86 ± 0.02 |
Lemon oil |
1.00 |
6.77 × 10−4 ± 0.02 × 10−4 |
6.64 × 10−2 ± 0.02 × 10−2 |
0.5 |
1.37 × 10−3 ± 0.02 × 10−3 |
9.52 × 10−2 ± 0.03 × 10−2 |
0.25 |
1.09 × 10−3 ± 0.02 × 10−3 |
1.02 × 10−1 ± 0.06 × 10−1 |
Conclusions
This study demonstrates that the essential oil obtained after distillation from the peel of Chinese Torreya grandis Fort. showed certain levels of antioxidant activity and tyrosinase inhibition. In particular, the IC50 value of CTGF oil was 0.88 ± 0.06 μg mL−1, CTGF oil had relatively significant DPPH scavenging activity (p < 0.05), and the DPPH scavenging efficiency was 42.6%. In addition, it was estimated that the antioxidant activity of CTGF oil was better than that of lemon oil according to changes in the peroxide values of different treatment groups during storage for 60 days, and it had similar antioxidant activity to BHT in reducing the peroxide value. Moreover, CTGF oil effectively inhibited the oxidation of L-DOPA by tyrosinase (P < 0.05). Therefore, essential oil from the peel of Chinese Torreya grandis Fort. may become a potential research area for the cosmetics and food industries.
Conflicts of interest
The authors declare that there are no conflicts of interest.
Acknowledgements
Financial support was kindly supplied by the start-up fund of Shanghai Institute of Technology.
References
- A. G. P. Samaranayaka and E. C. Y. Li-Chan, Food-derived peptidic antioxidants: a review of their production, assessment, and potential applications, J. Funct. Foods, 2011, 3(4), 229–254 CrossRef CAS.
- B. Turan, Role of antioxidants in redox regulation of diabetic cardiovascular complications, Curr. Pharm. Biotechnol., 2010, 11(8), 819–836 CAS.
- O. I. Aruoma, Free radicals, oxidative stress, and antioxidants in human health and disease, J. Am. Oil Chem. Soc., 1998, 75(2), 199–212 CrossRef CAS.
- M. M. Özcan and D. Arslan, Antioxidant effect of essential oils of rosemary, clove and cinnamon on hazelnut and poppy oils, Food Chem., 2011, 129(1), 171–174 CrossRef.
- R. Kahl and H. Kappus, Toxicology of the synthetic antioxidants BHA and BHT in comparison with the natural antioxidant vitamin E, Eur. Food Res. Technol., 1993, 196(4), 329–338 CAS.
- L. Song, X. Wang, X. Zheng and D. Huang, Polyphenolic antioxidant profiles of yellow camellia, Food Chem., 2011, 129(2), 351–357 CrossRef CAS PubMed.
- Y. Sone, J. K. Moon, T. T. Mai, N. N. Thu, E. Asano, K. Yamaguchi, Y. Otsuka and T. Shibamoto, Antioxidant/anti-inflammatory activities and total phenolic content of extracts obtained from plants grown in Vietnam, J. Sci. Food Agric., 2011, 91(12), 2259–2264 CAS.
- M. Bandyopadhyay, R. Chakraborty and U. Raychaudhuri, Antioxidant activity of natural plant sources in dairy dessert (Sandesh) under thermal treatment, LWT--Food Sci. Technol., 2008, 41(5), 816–825 CrossRef CAS.
- Y. Li, G. K. Skouroumounis, G. M. Elsey and D. K. Taylor, Microwave-assistance provides very rapid and efficient extraction of grape seed polyphenols, Food Chem., 2011, 129(2), 570–576 CrossRef CAS PubMed.
- F. Bakkali, S. Averbeck, D. Averbeck and M. Idaomar, Biological effects of essential oils-a review, Food Chem. Toxicol., 2008, 46(2), 446–475 CrossRef CAS PubMed.
- A. L. Dawidowicz, E. Rado and D. Wianowska, Static and dynamic superheated water extraction of essential oil components from Thymus vulgaris L, J. Sep. Sci., 2015, 32(17), 3034–3042 CrossRef PubMed.
- M. Madhumita, P. Guha and A. Nag, Optimization of the exhaustive hydrodistillation method in the recovery of essential oil from fresh and cured betel leaves (Piper betle L.) using the Box–Behnken design, J. Food Process. Preserv., 2019, 43(11), 14196–14210 Search PubMed.
- F. N. Muanda, R. Soulimani, B. Diop and A. Dicko, Study on chemical composition and biological activities of essential oil and extracts from Stevia rebaudiana Bertoni leaves, LWT--Food Sci. Technol., 2011, 44(9), 1865–1872 CrossRef CAS.
- D. S. Jk, E. H. A. Andrade, M. J. Kato, L. M. M. Carreira, E. F. Guimaraes and J. G. S. Maia, Antioxidant capacity and larvicidal and antifungal activities of essential oils and extracts from piper krukoffii, Nat. Prod. Commun., 2011, 6(9), 1361 Search PubMed.
- J. Xie, B. Sun, S. Wang and Y. Ito, Isolation and purification of nootkatone from the essential oil of fruits of Alpinia oxyphylla Miquel by high-speed counter-current chromatography, Food Chem., 2009, 117(2), 375–380 CrossRef CAS PubMed.
- S. Tsai, S. Huang, S. Lo, T. Wu, P. Lian and J. Mau, Flavour components and antioxidant properties of several cultivated mushrooms, Food Chem., 2009, 113(2), 578–584 CrossRef CAS.
- L. Wang, Z. Wang, H. Zhang, X. Li and H. Zhang, Ultrasonic nebulization extraction coupled with headspace single drop microextraction and gas chromatography-mass spectrometry for analysis of the essential oil in Cuminum cyminum L, Anal. Chim. Acta, 2009, 647(1), 72–77 CrossRef CAS PubMed.
- F. Sahena, I. S. M. Zaidul, S. Jinap, A. A. Karim, K. A. Abbas, N. A. N. Norulaini and A. K. M. Omar, Application of supercritical CO2 in lipid extraction
– a review, J. Food Eng., 2009, 95(2), 240–253 CrossRef CAS.
- T. Feng, J. Cui, Z. Xiao, H. Tian, F. Yi and X. Ma, Chemical Composition of Essential Oil from the Peel of Chinese Torreya grandis Fort., Org. Chem. Int., 2011, 2011, 1–5 CrossRef.
- C. M. Asensio, V. Nepote and N. R. Grosso, Chemical stability of extra-virgin olive oil added with oregano essential oil, J. Food Sci., 2011, 76(7), S445–S450 CrossRef CAS PubMed.
- B. E. Enriquezfernandez, L. Al. D. L. C. Y. Yanez and M. E. Sosamorales, Comparison of the stability of palm olein and a palm olein/canola oil blend during deep-fat frying of chicken nuggets and French fries, Int. J. Food Sci. Technol., 2011, 46(6), 1231–1237 CrossRef CAS.
- M. Foddai, M. Marchetti, A. Ruggero, C. Juliano and M. Usai, Evaluation of chemical composition and anti-inflammatory, antioxidant, antibacterial activity of essential oil of Sardinian Santolina corsica Jord. & Fourr, Saudi J. Biol. Sci., 2019, 26(5), 930–937 CrossRef CAS PubMed.
- T. Y. Lim, Y. Y. Lim and C. M. Yule, Evaluation of antioxidant, antibacterial and anti-tyrosinase activities of four Macaranga species, Food Chem., 2009, 114(2), 594–599 CrossRef CAS.
- I. Bettaieb, S. Bourgou, W. A. Wannes, I. Hamrouni, F. Limam and B. Marzouk, Essential oils, phenolics, and antioxidant activities of different parts of cumin (Cuminum cyminum L.), J. Agric. Food Chem., 2010, 58(19), 10410–10418 CrossRef CAS PubMed.
- N. S. Alzoreky and A. Y. Altaher, In vitro and in situ inhibition of some food-borne pathogens by essential oils from date palm (Phoenix dactylifera L.) spathe, Int. J. Food Microbiol., 2019, 299, 64–70 CrossRef CAS PubMed.
- S. K. El Euch, D. B. Hassine, S. Cazaux, N. Bouzouita and J. Bouajila, Salvia officinalis essential oil: chemical analysis and evaluation of anti-enzymatic and antioxidant bioactivities, S. Afr. J. Bot., 2018, 120, 253–260 CrossRef.
- J. L. Ballesteros, M. Tacchini, A. Spagnoletti, A. Grandini, G. Paganetto, L. M. Neri, A. Marengo, L. Angiolella, A. Guerrini and G. Sacchetti, Rediscovering medicinal amazonian aromatic plants: Piper carpunya (piperaceae) essential oil as paradigmatic study, J. Evidence-Based Complementary Altern. Med., 2019, 2019, 1–10 CrossRef PubMed.
- M. Adnan, Bioactive potential of essential oil extracted from the leaves of Eucalyptus globulus (Myrtaceae), J. Pharmacogn. Phytochem., 2019, 8(1), 213–216 CAS.
- A. Wei and T. Shibamoto, Antioxidant activities and volatile constituents of various essential oils, J. Agric. Food Chem., 2007, 55(5), 1737–1742 CrossRef CAS PubMed.
- M. Y. Dar, W. A. Shah, M. A. Rather, Y. Qurishi, A. Hamid and M. A. Qurishi, Chemical composition, in vitro cytotoxic and antioxidant activities of the essential oil and major constituents of Cymbopogon jawarancusa (Kashmir), Food Chem., 2011, 129(4), 1606–1611 CrossRef CAS.
- N. Gourine, M. Yousfi, I. Bombarda, B. Nadjemi and E. M. Gaydou, Seasonal variation of chemical composition and antioxidant activity of essential oil from pistacia atlantica desf. leaves, J. Am. Oil Chem. Soc., 2010, 87(2), 157–166 CrossRef CAS.
- J. Koudou, L. Obame, B. S. Kumulungui, P. Edou, G. Figueredo, J. C. Chalchat and A. S. Traore, Volatile constituents and antioxidant activity of Aucoumea klaineana Pierre essential oil, Afr. J. Pharm. Pharmacol., 2009, 3(6), 323–326 CAS.
- K. Park, Y. Park, J. Lee, H. Hahn, S. Lee, C. Bae, J. Yang, D. Kim and M. Hahn, Inhibition kinetics of mushroom tyrosinase by copper-chelating ammonium tetrathiomolybdate, Biochim. Biophys. Acta, 2005, 1726(1), 115–120 CrossRef CAS PubMed.
- C. Hsu, C. Chang, H. Lu and Y. Chung, Inhibitory effects of the water extracts of Lavendula sp. on mushroom tyrosinase activity, Food Chem., 2007, 105(3), 1099–1105 CrossRef CAS.
- B. Yang, M. Zhao and Y. Jiang, Optimization of tyrosinase inhibition activity of ultrasonic-extracted polysaccharides from longan fruit pericarp, Food Chem., 2008, 110(2), 294–300 CrossRef CAS PubMed.
- C. T. Chang, W. L. Chang, J. C. Hsu, Y. Shih and S. T. Chou, Chemical Composition and Tyrosinase Inhibitory Activity of Cinnamomum Cassia Essential Oil, Bot. Stud., 2013, 54, 1–10 CrossRef PubMed.
- Z. Aumeeruddy-Elalfi, A. Gurib-Fakim and M. F. Mahomoodally, Kinetic studies of tyrosinase inhibitory activity of 19 essential oils extracted from endemic and exotic medicinal plants, S. Afr. J. Bot., 2016, 103, 89–94 CrossRef CAS.
- A. Manosroi, K. Boonpisuttinant, S. Winitchai, W. Manosroi and J. Manosroi, Free radical scavenging and tyrosinase inhibition activity of oils and sericin extracted from Thai native silkworms (Bombyx mori), Pharm. Biol., 2010, 48(8), 855–860 CrossRef.
- H. Aati, A. A. Elgamal and O. Kayser, Chemical composition and biological activity of the essential oil from the root of Jatropha pelargoniifolia Courb. native to Saudi Arabia, Journal of The Saudi Pharmaceutical Society, 2019, 27(1), 88–95 CrossRef PubMed.
- L. Xia, S. Lu and G. Cao, Salt-assisted microwave demulsification, Chem. Eng. Commun., 2004, 191(8), 1053–1063 CrossRef CAS.
- K. Seebunrueng, Y. Santaladchaiyakit and S. Srijaranai, Vortex-assisted low density solvent liquid–liquid microextraction and salt-induced demulsification coupled to high performance liquid chromatography for the determination of five organophosphorus pesticide residues in fruits, Talanta, 2015, 132, 769–774 CrossRef CAS PubMed.
- M. Fortuny, C. B. Z. Oliveira, R. L. F. V. Melo, M. Nele, R. C. C. Coutinho and A. F. Santos, Effect of Salinity, Temperature, Water Content, and pH on the Microwave Demulsification of Crude Oil Emulsions, Energy Fuels, 2007, 21(3), 1358–1364 CrossRef CAS.
|
This journal is © The Royal Society of Chemistry 2019 |