DOI:
10.1039/C9RA06487G
(Review Article)
RSC Adv., 2019,
9, 28924-28935
Research status, industrial application demand and prospects of phenolic resin
Received
19th August 2019
, Accepted 6th September 2019
First published on 17th September 2019
Abstract
The synthesis process of the phenolic resin adhesive was developed in the 19th century, and its excellent environmental resistance and high bonding strength make it one of the main wood adhesives. With the development of industry, phenolic resin adhesive is not only used in plywood, wood processing and laminate, but also in automobile, aerospace, composite materials and other fields. Herein we review the main synthetic processes and latest research progress for phenolic resin adhesives, the capacity distribution of major domestic phenolic resin enterprises, analysis of domestic phenolic resin consumption, import and export volume and price, Chinese plywood production, and the main production and export markets. Furthermore, research and discussion on the commercial application of domestic phenolic resin adhesives in plywood were carried out, which provides a reference for development of better phenolic resin adhesives that meet the development needs of the country, along with quality and economic competitiveness.
1 Introduction
A polymeric compound obtained by polycondensation of a phenolic compound and an aldehyde compound under acidic or basic conditions generally refers to a resin synthesized from phenol and formaldehyde, and is called a phenol-formaldehyde resin (PF).1 At the end of the 1920s, phenolic resin adhesives were widely used in the production of plywood, due to its advantages of strong mechanical properties, high flame retardancy, stable molding processing performance, low cost, etc. However, the traditional phenolic resin requires a high curing temperature, which makes the curing process slow.2 In recent years, phenolic-resin-based composite materials have been developed further by improving the synthesis process and mechanical properties of phenolic resin in terms of brittleness. At the same time, many researchers have reported other focused and optimized properties related to their products and formaldehyde emission. Therefore, domestic phenolic resin is widely used in wood processing, foundry processing, electrical insulation, the automotive industry, and other fields,3 and is gradually replacing imported phenolic resin.
2 Research and industrialization of phenolic resin adhesives
2.1 Synthesis of phenolic resin
Based on the process of synthesizing a phenolic resin, the prepared resin is classified into two types: thermoplastic phenol resin and thermosetting phenol resin. The chemical structure of the raw material, the molar ratio of the phenol to the aldehyde, and the pH of the medium, and the type of catalyst are the main factors governing the type of resin.4 A thermosetting phenolic resin, also known as a resole phenolic resin, is synthesized in the presence of an excess of basic catalyst and the aldehyde. When excess acidic catalyst and phenolic substance were used, a synthetic phenolic resin, also known as a thermoplastic phenolic resin, was synthesized.
2.1.1 Synthesis principle of thermosetting phenolic resin. The synthesis process of thermosetting phenolic resin is divided into two stages: hydroxylation reaction and polycondensation reaction.5
(1) Hydroxymethylation. Under alkaline conditions, phenol reacts with catalyst NaOH upon heating to form phenoxy anion. Its resonance form suggests that phenoxy anion is a nucleophilic reagent that can attack the carbonyl group of formaldehyde to form phenol-hydroxymethyl.5 The reaction formula is shown in Fig. 1.
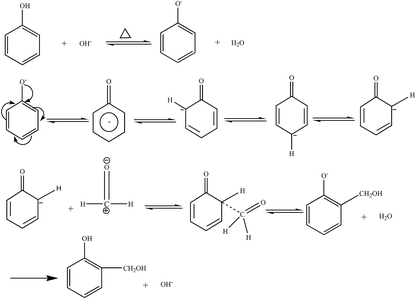 |
| Fig. 1 Formation process of phenol-hydroxymethyl. | |
The ortho and para positions on the phenol nucleus are activated by the phenolic hydroxyl group, producing ortho- or para- phenol-hydroxymethyl when reacted with formaldehyde. The methylol phenol can continue to react with formaldehyde to form dimethylol phenol and trimethylol phenol.5 The reaction formula is shown in Fig. 2.
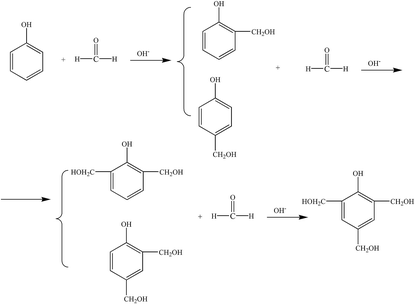 |
| Fig. 2 Addition reaction process. | |
In fact, the addition reaction product is a mixture of phenol-monomethylol and phenol-polymethylol.
(2) Polycondensation reaction. The phenol-hydroxymethyl obtained by the above addition reaction and the phenol may react with each other or undergo a polycondensation reaction with each other. When excess aldehyde and basic catalyst are used, mainly two ortho and one para-position on the phenol are replaced by hydroxymethyl groups. When heated further, two condensation reactions occur, resulting in increased relative molecular mass: the condensation between the two methylol groups forming an ether chain (–CH2OCH2–); the second, one hydroxymethyl group with an ortho or para-active hydrogen atom on a phenol reacts to form a methine chain.5 The product of the first reaction can also be converted to the product of the second reaction, as shown in Fig. 3.
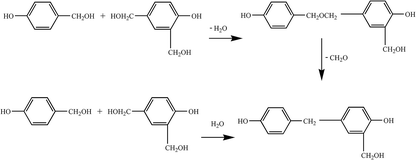 |
| Fig. 3 Polycondensation reaction process. | |
Therefore, when the degree of polycondensation is not large, the molecular structure of the product will contain more hydrophilic groups (such as the hydroxymethyl group), which will appear as a low-viscosity water-soluble liquid; when further reacted, it will lead to hydrophilic hydroxyl group. Condensation occurs between the hydrophilic groups to produce a highly viscous liquid, which is less water-soluble or immiscible, and which becomes a viable solid after washing with water.
2.1.2 Synthesis principle of thermoplastic phenolic resin. The thermoplastic phenolic resin has a linear structure, and the reaction conditions are acidic (pH 0.5–1.5, due to the presence of hydrochloric acid, sulfuric acid, oxalic acid, benzenesulfonic acid, etc.). The molar ratio of phenol to formaldehyde is 1: (0.7–0.9). In an acidic medium, the reaction between the phenol-hydroxymethyl and the hydrogen atom on the phenol ring is faster than that between the aldehyde and the phenol.5 The synthesis of the thermoplastic phenolic resin is shown in Fig. 4.
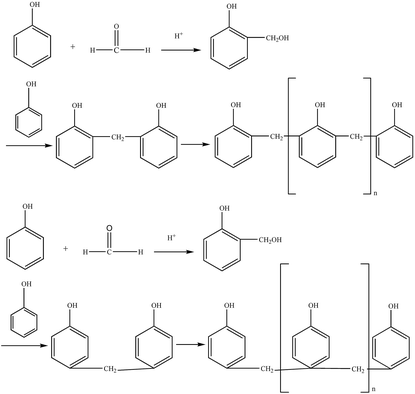 |
| Fig. 4 Synthesis of thermoplastic phenolic resin. | |
The thermoplastic phenolic resin mainly comprises methylene chains and phenolic hydroxyl groups, does not contain hydroxymethyl and ether linkages, and has poor water solubility, with its relative molecular mass ranging from 500 to 900.5
2.2 Research status of phenolic resin adhesive
Phenol-formaldehyde materials were first discovered in 1872 by the German chemist Adolph Von Baeyer. In 1907, the use of phenolic resins, also known as Bakelite, was developed by L. H. Baekeland, the “father of the phenolic resins”.6 Since the phenolic resin has the disadvantages of high brittleness, low peel strength, strong odor during curing, and high curing shrinkage, it is necessary to modify it to improve the comprehensive utilization performance. Modifications of existing common phenolic resins include measures to improve brittleness, improve heat resistance, and reduce formaldehyde emission.
2.2.1 Improve brittleness. Toughness is generally improved by the addition of an external toughening substance or an internal toughening substance. Commonly used external toughening substances are natural rubber, nitrile rubber, and thermoplastic resin, while internal toughening methods include etherification of phenolic hydroxyl groups, introduction of long methylene chains and other flexible groups between phenolic cores, and the use of glass fibers, glass cloth, asbestos, etc. to improve the brittleness of phenolic resins.7Xu Pingping et al.8 reported the co-modification of PF resin with boric acid and pyrolysis oil, by adjusting the pyrolysis oil content instead of phenol, boric acid addition, and the sodium hydroxide/phenol (NaOH/P) molar ratio during pyrolysis. When the content of the oil substitute to phenol is 20%, the boric acid addition amount is 4%, and the NaOH/P molar ratio is 0.5, the properties of the synthetic phenolic resin can be optimized as follows: the tensile strength of the film is 3.15 MPa, the elongation at break is 15.7%, and the bonding strength is 1.12 MPa. Xu Dongzhen et al.9 used different amounts of hydroxyl-terminated liquid polybutadiene (HTPB) to modify phenolic resin; when HTPB (H0711-06) with a mass fraction of 3% was added, the maximum impact strength was 3.6 kJ m−2. Feng Jingwei et al.10 obtained cardanol grafted liquid nitrile rubber (C-g-LNBR) by solution grafting. The modified PF was synthesized by using LNBR and C-g-LNBR as the toughening substance by in situ polymerization. The modified PF was used as the matrix, and the friction material was obtained by hot press forming. The thermal properties of the matrix were analyzed by thermogravimetric analysis and differential scanning calorimetry. The effects of LNBR and C-g-LNBR on the mechanical and tribological properties of PF-based friction materials were investigated. The results show that the impact strength of the 2%-C-g-LNBR/PF-based friction material is 14.8% and 7.3% higher than those of the pure PF-based and 2%-LNBR/PF-based friction materials, respectively. Rong Liping et al.11 reported that the epoxy-resin-modified phenolic resin adhesive was prepared by blending epoxy emulsion with phenolic emulsion by a room-temperature blending method, and the bonding strength and curing property of the modified phenolic resin adhesive were observed. The experimental results show that the adhesive reacts chemically with the epoxy resin and the phenolic resin during the curing process. Simultaneously, the modified phenolic resin adhesive changed from heterogeneous to homogeneous phase during the curing process, and the fracture surface of the cured product showed a certain degree of ductile fracture characteristics. When 20% epoxy resin was added, the epoxy-modified phenolic resin adhesive had a shear strength of 9.84 MPa at normal temperature and a shear strength of 9.84 MPa at 250 °C. Nie Xieming et al.12 by mixing, dissolving and adding thermosetting phenolic resin, nitrile butadiene rubber (NBR) as toughening modification material, the butadiene-phenolic resin solution was prepared. The composite prepreg was prepared by drying and coiling at 70–90 °C through impregnated with alkali-free glass cloth at room temperature. The results show that the tensile strength shear strength and peeling strength of the prepreg can reach 376 MPa, 22.96 MPa and 4.5 kN m−1 when the rubber content is about 42%.
2.2.2 Improve heat resistance. Phenolic resin is a traditional synthetic polymer material with a long history of one century.13 Adhesive is one of the main applications of phenolic resin, the main application field being wood adhesives.14 Phenolic resin adhesives have excellent properties like heat resistance, thermal stability, and flame retardancy;15 therefore, phenolic resin adhesives are also used in aerospace, automobile, composite materials, and other fields.16 With the development of the aerospace industry, there is an urgent need to improve the long-term high-temperature oxidation resistance of phenolic resins as ablative materials,17 and promote research on the heat resistance of phenolic resins. Existing reports state that the introduction of aromatic rings (like toluene, xylene, naphthalene, etc.) or melamine, coking kerosene, boride, silicone resin, molybdenum, etc. in the phenolic resin can improve the stability of the entire macromolecular chain and heat resistance. In addition, nanomaterials like boron carbide, nano-copper, and nano-silicon are used to improve the heat resistance of phenolic resins.18Wang Dongxu et al.19 reported that plasticizer rubber, a phenolic resin adhesive synthesized under catalysis by nano-alumina, has excellent heat resistance. Its shear strengths are 36.32 MPa and 4.65 MPa at room temperature and 500 °C, respectively, proving excellent bonding properties. Hu Ruofei et al.20 added different amounts of molybdenum to the synthetic phenolic resin for modification. Thermogravimetric analysis showed that when 10% ammonium molybdate was added, the thermal decomposition temperature of the phenolic resin reached a maximum of 380 °C. Zhang Y. et al.21 prepared Ti-modified phenolic resin (Ti-PF) from tetra-isopropyl titanate, phenol, and formaldehyde. The structure of the modified resin was proved by Fourier-transform infrared (FT-IR) spectroscopy. The thermal decomposition behavior of Ti-PF was investigated using a thermogravimetric analyzer. The results show that when 1% Ti was added to the phenolic resin, the decomposition rate of Ti-PF reached a maximum at 554 °C, larger 43 °C than that of the unmodified PF. The addition of Ti significantly increased the thermal stability of the phenolic resin; Zhao Lei et al.22 prepared bisphenol A phenolic resin (Bis-ANR) from bisphenol A and formaldehyde solution, and then reacted it with epoxide phenolic resin and epichlorohydrin (ECH) to obtain bisphenol A-type novolac epoxy resin (Bis-ANER). Boric-acid-modified bisphenol A phenolic resin (Bis-ABNR) was prepared by using bisphenol A, formaldehyde solution, and boric acid. Bis-ANER was cured using Bis-ABNR as curing agent, and was analyzed by using thermogravimetric analysis and differential scanning calorimetry. The heat resistance measurements of the cured product revealed that the cured product has a glass transition temperature (Tg) of 165 °C and an initial thermal decomposition temperature (Td) of 309.85 °C, showing high thermal stability; Li S. et al.23 prepared organosilicon phenolic resin by esterification of methyl trimethylsilane with novolac resin, and studied the thermal decomposition properties of the resin by using a thermogravimetric analyzer. The results showed that the initial thermal degradation temperature of the silicone-modified phenolic resin was 411.6 °C, which was 21.5 °C higher than the initial thermal degradation temperature of the unmodified PF. Chen J. et al.24 modified phenolic resin (PR) with hybrids of reduced graphene oxide (RGO)-encapsulated nano-SiO2 (SiO2-RGO) was prepared by a simple method. The synergistic effect of RGO and nano-SiO2 was achieved in the thermal decomposition of the modified PR. Thermal stability of SiO2-RGO and the modified PR was evaluated by thermogravimetric analysis (TG). With 1 mass% loading of SiO2-RGO, the maximum decomposition temperature (T-dmax) of the modified PR was increased by 32.50 °C and the residual mass at 800 °C was increased by 6.54%. Thus, SiO2-RGO can facilitate the application of PR in the fields of heat insulation and ablation resistance. Yang W. et al.25 investigated, for the first time, the role of nanosized lignin (LNP), in comparison with microlignin (LMP), when introduced at two different weight amounts (5% and 10 wt%) in bulk phenol formaldehyde resole as adhesive. The curing process has been examined by differential scanning calorimetry (DSC), while the thermal stability of the composites has been evaluated by using thermogravimetric (TGA) and thermomechanical (TMA) analysis. Results exhibited that small amount of lignin could both favor the thermal cure reaction, due to its abundance of phenylpropane units, and the initial thermal resistance could be consequently improved, especially when the nano-sized lignin was used.
2.2.3 Reduce formaldehyde emission. Existing research reports both home and abroad have shown that the use of lignin, resorcinol, cashew oil, shellac, and other materials as alternatives to modified phenolic resin adhesive, or to improve the synthesis process, can reduce the amount of formaldehyde emission, reduce production costs, and protect the environment. GB/T14732-2017 requires a free formaldehyde content of 0.3% or less. Lignin is the second most abundant natural biomass after cellulose.26 During the separation and utilization of biomass resources, lignin is mostly discarded, and its value is not fully utilized, because the structure of lignin contains a large amount of benzene rings and phenolic hydroxyl groups, now considered the main aromatic renewable resources, are the best alternatives for the manufacture of chemicals and polymers.27 Therefore, lignin is often used to replace phenol for the synthesis of phenolic resin to achieve full utilization of biomass resources. In order to successfully introduce lignin into phenolic resin as a substitute for phenol, relatively lower molar mass and better reactivity are required.28Foyer G. et al.29 have developed a new method to synthesize biobased aldehyde precursors for the synthesis of formaldehyde-free resol-type phenolic resins. We have designed a functionalization method to turn the lignin-based aldehyde precursors, i.e. 4-hydroxybenzaldehyde and vanillin, into difunctional and reactive aromatic aldehyde precursors and proved that those precursors are competitive substituents for the synthesis of resol-type phenolic resins. Those resol-type resins are synthesized without any use or release of the highly toxic and volatile formaldehyde and present close thermal stability and char yield properties. Zhang Y. et al.30 studied the work that HMF was in situ generated from glucose using chromium chlorides as catalysts and was polymerized with phenol to produce a Novolac PHMF resin. The structure was identified and the possible reaction schemes were proposed and discussed. Curing of the PHMF was realized using formaldehyde-free curing agents including OL or KL, starting at 120 °C and peaked at around 150 °C. The curing kinetic parameters for the PHMF resin were calculated. The curing mechanism was elucidated using FTIR and 13C NMR. The resin was successively applied as polymer matrix in fiberglass to produce biocomposites with good thermomechanical property. The PHMF resin has a glass transition temperature of 120–130 °C, and the fiberglass reinforced bio-composites using PHMF resin hardened with OL/KL has a Tg up to approximately 270 °C, which is similar to those of the HMTA-cured PF resin. The lignin (OL/KL) cured woven fiberglass cloth-PHMF resin composites demonstrated comparable tensile fracture strengths (∼95 MPa) to that of the HMTA cured composite. Benoit B. et al.31 used the two-step novolac strategy involves the synthesis of a cardanol nonanal pre-polymer (PCN) prior to its cross-linking in presence of a supplementary amount of nonanal hardener. The curing process was optimized by rheology measurement and thermogravimetry analysis. The alkyl chains of this phenolic network induced soft mechanical properties acting as internal plasticizers. The softening of the resulting networks did not induce a loss of thermal stability. Showing a good compromise between softness and high thermal resistance, cardanol-nonanal networks (RCN) seem to be relevant examples of an innovative and biobased approach for phenolic chemistry to design bio-sourced materials without any petro-based or hazardous molecules. Foyer G. et al.32 used a functionalization method from 4-fluorobenzaldehyde has been designed and new difunctional and bio-based aromatic aldehyde precursors were synthesized in excellent yields. Those starting materials proved to be excellent alternatives to formalde-hyde leading to bio-based resol-type phenolic resins free of formaldehyde with higher thermal stability and char yield properties. Ghorbani et al.33 studied the applicability of lignin phenolic resin (LPF) in the production of papermaking high-pressure laminates. As lignin source, pine kraft lignin and spruce sodium lignosulfonate were compared, substituting 40 wt% of phenol by lignin in each case. The synthesized resins were characterized for their viscosity development, solid content, PH, free formaldehyde as well as free phenol content and B-time. Paper-based high-pressure laminates were manufactured using the two different resins. The laminates were exposed to different test climates and were compared for boiling water resistance, thickness swelling, bending properties and impact energy. The results indicate that the pine kraft lignin (PKL-PF) and spruce sodium lignosulphonate (SSOL-PF) to replace 40 wt% of the free formaldehyde content of LPF resin were 1.7%, 1.1% (in compliance with the Chinese National Standard GB/T14732-2017: free formaldehyde content ≤0.3%), and pine kraft lignin performs superior to spruce sodium lignosulfonate for utilization in paper based laminates for outdoor usage due to a lower affinity of the pine kraft LPF laminates to water. Kalami Somayyeh et al.34 used different kinds of lignin to replace phenol to prepare phenolic resin adhesive. The DSC and ASTM standard test methods showed that corn stalk lignin with high content of hydroxyphenyl group (0.81 mmol g−1) is the best choice for 100% preparation of alternative phenolic resin adhesives. When 100% of the substituted phenol was reacted with formaldehyde, the free formaldehyde content of the phenolic resin became 0.1% (according to the requirements of GB/T14732-2017), and the amount of formaldehyde was reduced by 50%; Stücker et al.35 used enzymatic hydrolysis of poplar lignin (EHL) and alkali extraction (AEL) lignin to prepare lignin-phenol-formaldehyde resin (LPF). The results show that the free formaldehyde content of LPF synthesized by enzymatic lignin (EHL) and alkali extraction (AEL) lignin is 0.12%, which meets the requirements of GB/T14732-2010 (less than or equal to 0.3%); Zhang W. et al.36 partially replaced phenol 10–70% with lignocellulosic ethanol residues (ER) is prepared as a lignin-phenol-formaldehyde (LPF) adhesive. Characterization of the composition, functional group, and molecular weight of ER revealed that ER with more hydroxyl groups and less methoxy groups and lower molecular weight is suitable for the synthesis of LPF adhesives. The process parameters of ER-modified PF (ERPF) adhesive were optimized. The results show that the performance of ERPF adhesive prepared by using 10% ER instead of phenol is the best: its free formaldehyde content is 0.1%, free phenol content is 0.31%, and gluing strength is 1.40 MPa; Zhao M. et al.37 reported the preparation of steam explosion lignin phenolic resin (SEL-PF) adhesive by ternary stepwise copolymerization and optimization of steam explosion lignin (SEL) phenolate parameters for the preparation of adhesives. The optimized phenolate conditions were as follows: the reaction temperature of 90 °C, the reaction time of 1.5 h, the lignin/phenol weight ratio of 4
:
6, and the NaOH concentration of 8% by weight. When phenol is substituted by 10% SEL to synthesize phenolic resin adhesive, the free formaldehyde content is at least 0.19% and the bonding strength is 0.79 MPa, in compliance with the Chinese national standard GB/T14732-2006 (free formaldehyde content ≤0.3%, and bonding strength ≥0.7 MPa).
2.3 Industrial application of phenolic resin adhesive in plywood
Phenolic resins are commonly used in the industry. They are most widely used in plywood because of their excellent water resistance, heat resistance, and adhesive strength.38 The phenolic resin is the first synthetic resin in the world to be industrially produced. At present, the research on the modification of phenolic resin is vast, the synthesis mechanism is mature, and the synthetic raw materials are not limited to phenol and formaldehyde, but also raw materials such as lignin and cashew nut oil, which can be used to make the synthetic materials more extensive. In the United States, Britain and other countries, the amount of wood adhesive and phenolic resin adhesive used is much greater than the amount of urea-formaldehyde resin adhesive. The proportion of phenolic resin adhesive used in domestic wood is low.38 Therefore, the market situation of plywood and phenolic resin was investigated.
2.3.1 China's production of plywood. The plywood is a three-ply sheet formed by bonding a veneer by an adhesive. Before reform and opening, all of China's plywood enterprises were state-owned and mainly concentrated in the timber producing areas and timber semi-production and semi-sales areas. Most of the raw materials used were domestic logs, and the product sales were mainly concentrated in the Beijing–Tianjin–Shanghai region. After the implementation of the natural forest protection project, the main producing areas of plywood have been shifted to the traditional northeast, Jiangxi, and Fujian forest resources-rich areas in provinces such as Hebei, Shandong, Jiangsu, and Zhejiang, where economically developed natural forest resources are not abundant. Due to the widespread cultivation of fast-growing materials in these areas, the problem of raw materials for plywood production has been solved, and convenient transportation and extensively cheap labor have provided advantages for the development of plywood in these areas.39At present, China's plywood production areas are mainly concentrated in Hebei, Jiangsu, Shandong, Zhejiang, Guangdong, Guangxi and other provinces and are present in a number of large plywood industry clusters with regional characteristics (see Table 1).
Table 1 China's plywood industry cluster
Industrial clusters |
Core city |
Shandong plywood industry cluster |
Linyi City, Heze City, Shandong Province |
Guangxi plywood industry cluster |
Nanning City, Rong County City, Guangxi Province |
Subei plywood industry cluster |
Zhangzhou City, Jiangsu Province, Suqian City |
Hebei plywood industry cluster |
Xingtai City, Langfang City, Hebei Province |
Zhejiang plywood industry cluster |
Jiashan City, Zhejiang Province |
Anhui plywood industry cluster |
Suzhou City, Anhui Province |
In 2003, the production of Chinese plywood reached 2012.35 million cubic meters, surpassing the United States for the first time. It became the world's largest producer of plywood. In 2015, China's plywood production reached 165.462 million cubic meters, which corresponded to an increase of 10.53% over that of the previous year, accounting for 57.59% of China's wood-based panel production. Out of this, plywood production was 154.344 million cubic meters.40 The production of China's plywood in 2005–2017 is shown in Fig. 5.
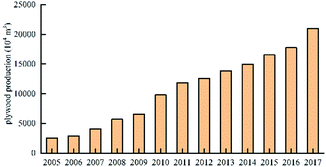 |
| Fig. 5 China's plywood production in 2005–2017. | |
2.3.2 Number of plywood exports and trade share of major export markets. In the 20th century, China was a major importer of plywood. However, after 1992, the export of plywood from China increased rapidly.41 It can be seen from Fig. 6 that before 2007, the export volume of Chinese plywood was growing. In 2008, due to the financial crisis and traditional trade barriers in other countries, the export volume of plywood decreased from 7.175 million cubic meters in 2007 to 7.185 million cubic meters in 2008, which further fell to a minimum of 5.634 million cubic meters in 2009. After this, the volume of Chinese plywood exports gradually increased as the economy recovered.42 The export volume of China's plywood in 2005–2017 is shown in Fig. 6.
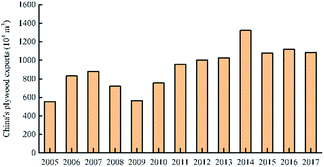 |
| Fig. 6 Statistical chart of China's plywood exports in 2005–2017. | |
The analysis of the market structure of China's plywood export suggests that the main exporting countries are the United States, South Korea, Japan, and the British.43 Fig. 7 suggests that the market share of Chinese plywood export to the United States is relatively large, and it remains the first market for Chinese plywood exports. Japan and the United Kingdom market shares are less volatile.44
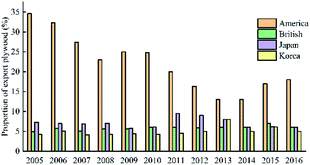 |
| Fig. 7 China's plywood export market trade share in 2005–2016. | |
2.3.3 The amount of phenolic resin adhesive used in plywood. According to the statistics of the prospective Economist, the dosage for indoor urea-formaldehyde resin (UF) in ordinary plywood is 55 kg m−3 and that of outdoor (mainly concrete template plywood) phenolic resin glue (PF) is 71.5 kg m−3. Based on past experience, it is estimated that about 10% is used outdoors and about 90% is used indoors (Fig. 8).
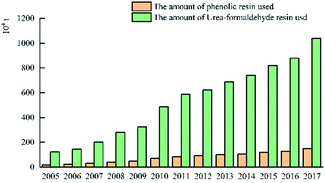 |
| Fig. 8 Resin usage in China's plywood in 2005–2017. | |
The above data shows that the use of phenolic resin in the plywood industry in China is much smaller than that of urea-formaldehyde resin, and the phenolic resin is mainly used in the oncrete form in wood. Hence, the phenolic resin is released in water resistance, bonding strength and free formaldehyde performance has to be further improved.
3 Production and market analysis of phenolic resin
3.1 China production of phenolic resin
In 2016, the annual production of phenolic resin in China was 1.018 million tons. The enterprises producing high production of phenolic resin, including Jinan Shengquan Group Co., Ltd., Shanghai Eurasian Composite Materials Co., Ltd., and Shanghai Double Tree plastic factory, are mainly concentrated in Shanghai, Zhejiang, Jiangsu, and Fujian, etc.45 The world's largest several phenolic resin production enterprises are: Hexion Specialty Chemicals, Georgia – Pacific Resins, Dynea and Arclin. The production capacity of these four companies' phenolic resin reaches 2.151 million tons per year, accounting for 42% of the world's total production capacity.46 The production of China's phenolic resin in 2005–2017 is shown in Fig. 9. The production of world phenolic resin in 2005–2011 is shown in Fig. 10. The main China's phenolic resin production enterprises and production capacity are shown in Table 2.
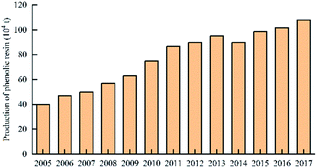 |
| Fig. 9 The production of China's phenolic resin in 2005–2017. | |
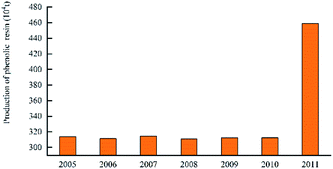 |
| Fig. 10 The production of world phenolic resin in 2005–2011. | |
Table 2 Major China's phenolic resin production enterprises and production capacity (unit: 104 ton)
Company name |
Main products |
Location |
Scale of production capacity |
Jinan Shengquan group Co., Ltd. |
Phenolic resin for organic abrasives, friction materials and refractories |
Shengquan Road, Diao Town Industrial Park, Zhangqiu District, Jinan City, Shandong Province |
35 |
Finland Taier Chemical Company |
Phenolic binder resin |
No. 59 Chongfu Road, Chemical Industrial Park, Liuhe District, Nanjing City |
15 |
Location B, Floor 1, No. 62 East Fute Road, China (Shanghai) Pilot Free Trade Zone |
1 |
San Lecot International Group |
Phenolic resin for various types of tires, refractory materials, etc. |
No. 99, Cang District South Road, Nanjing Chemical Industrial Park |
7 |
26D, Feizhou International Plaza, No. 899, Lingling Road, Shanghai |
5 |
British Huaqi (Zhangjiagang) Chemical Co., Ltd. |
Phenolic resin for tires |
No. 99 Tianba Road, Yang Zi Jiang International Chemical Industry Park, Jiangsu Province |
6 |
Asia Boden hemical (Heyuan) Co., Ltd. |
Phenolic Resin |
Heyuan, Guangdong |
5.5 |
Germany Eurasian Love Chemical Co., Ltd. |
Casting resin, paint, coated sand, etc. |
Nantong, Jiangsu |
3.6 |
Korea Kelon Chemical (Suzhou) Co., Ltd. |
Phenolic resin for refractory materials and abrasive materials |
No. 56 Qingqiu Street, Suzhou Industrial Park, Suzhou City, Jiangsu Province |
2.5 |
Shanghai Eurasian Synthetic Material Company |
Heat resistant, impact resistant, acid resistant, wear resistant phenolic molding compound |
No. 500 Jinou Road, Jinshan District, Shanghai |
2.5 |
Shandong Laiwu Runda Chemical Co., Ltd. |
Casting molding, friction materials, refractory materials, phenolic resins for abrasives |
Hengshan Road, Laicheng District, Laiwu City |
2 |
US Momentive Company and China Unicom Chemical Company Joint Venture |
Novolac, friction material, grinding material and abrasive |
Zhenjiang, Jiangsu |
1.5 |
Shanghai Shuangshu Plastic Factory |
General purpose, heat resistant, electrical phenolic molding compound |
No. 3151, Gugao Road, Pudong New Area, Shanghai |
1.5 |
Dashiqiao Resin Factory |
Phenolic resin for refractory materials |
Liaoning Yingkou City Dashi Bridge City Union |
1.2 |
Henan Bangde Chemical Co., Ltd. |
Organic abrasives, friction materials, phenolic resins for refractories |
Xingyang Yulong Town, Zhengzhou City, Henan Province, Mao Zhai Industrial Park |
1–1.5 |
Guangdong Jiangmen Kunyi Resin Materials Technology Co., Ltd. |
Friction materials, coated abrasives, abrasives, refractories, phenolic resins for insulation materials |
Yanmen Town, Xinhui District, Jiangmen City, Guangdong Province |
1 |
Changshu Southeast Plastics Co., Ltd. |
Phenolic molding compound, amino molding compound, unsaturated polyester molding compound, phenolic resin |
Shanming Road, Dayi Town, changshu city, Jiangsu Province |
0.9 |
Zhengzhou Shuangge Resin Co., Ltd |
Organic abrasives, friction materials, refractory materials, phenolic resins for insulating materials |
Xushui Town, Zhengzhou City, Henan Province, New Village Power Supply |
0.8 |
Hebei Longgang Industry and Trade Co., Ltd. |
Refractory materials, friction materials, phenolic resins for abrasives |
Zhuangke Village, Wangjun Township, Zaoqiang County, Hengshui County, Hebei Province |
0.7 |
The above data shows that the industrial cluster for the China's phenolic resin production capacity are concentrated in coastal areas such as Shanghai, Zhejiang, Jiangsu, and Fujian. There are only a handful of large-scale manufacturers, and the companies with small capacity are mostly. For example, the annual production capacity of Jinan Sheng Quan Group is 350
000 tons and that of Finland Taier Chemical Company (Nanjing) is 150
000 tons. Henan Bangde Chemical Co., Ltd. and Guangdong Jiangmen Kunyi Resin Materials Technology Co., Ltd. have an annual production capacity of about 10
000 tons.
3.2 Imports, exports and prices of phenolic resins
Fig. 11 shows that the import volume of phenolic resin fluctuates around 100
000 tons, and the export volume fluctuates around 70
000 to 80
000 tons. The import volume and export volume of China's phenolic resin in 2005–2017 are shown in Fig. 12.
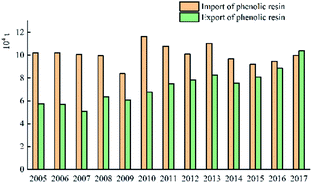 |
| Fig. 11 China's phenolic resin imports and exports in 2005–2017. | |
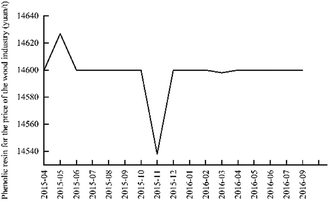 |
| Fig. 12 Price of phenolic resin in the timber industry. | |
It can be seen from Fig. 11 that the price of phenolic resin in the wood industry is basically stable, fluctuating around 14
600 yuan/ton. The price of Chinese phenolic resin for the wood industry from April 2015 to September 2016 is shown in Fig. 12.
3.3 Market consumption of phenolic resin
Phenolic resins are mainly used in the fields such as phenolic molding compounds, wood processing, friction materials, refractory insulation materials and coatings.47 The consumption of China's phenolic resin is shown in Table 3. The consumption of world phenolic resin is shown in Table 4.
Table 3 Consumption of China's phenolic resin (unit: 104 ton)
Application field |
2007 |
2008 |
2009 |
2010 |
2014 |
Phenolic molding compound |
12.4 |
12.1 |
11.9 |
12.3 |
13.5 |
Wood processing, laminate |
11.6 |
10.7 |
10.8 |
11.3 |
12.3 |
Grinding, friction material |
11 |
10 |
10 |
10.6 |
12 |
Refractory, thermal insulation material |
10.6 |
8.8 |
9 |
9.8 |
11.3 |
Casting material |
4.9 |
4.4 |
4.5 |
5 |
6 |
Coating |
2.3 |
1.9 |
1.8 |
2 |
2.3 |
Other |
2.2 |
1.7 |
1.8 |
2 |
2.7 |
Total |
55 |
49.6 |
49.8 |
53 |
60.1 |
Table 4 Consumption of world phenolic resin (unit: 104 ton)
Application field |
2007 |
2008 |
2009 |
2010 |
2014 |
Wood adhesive |
114.1 |
114.5 |
115 |
115.5 |
130 |
Phenolic molding compound |
42.6 |
43.4 |
44.5 |
47.5 |
56 |
The laminate |
37.1 |
37.3 |
37.5 |
37.5 |
43.5 |
Refractory, thermal insulation material |
37 |
37.1 |
37.2 |
37.2 |
42 |
Other |
83.1 |
84.5 |
85 |
86.5 |
95 |
Total |
314.9 |
316.8 |
319.2 |
324.2 |
366.5 |
Tables 3 and 4 show that the consumption of phenolic resin in various fields in the world is similar to that in China. In addition to the application of phenolic resin in the field of wood processing and plywood, the proportion of refractory and thermal insulation materials is high. For example, in China in 2014, the usage of phenolic molding compounds, wood processing and laminates, and refractory and thermal insulation materials were 135
000 tons, 123
000 tons, and 113
000 tons, respectively; phenolic resins were used less for foundry materials and coatings. Phenolic resins also have potential for application development such as foam materials and carbon sources.
3.4 The application of phenolic resin in other fields
In the recent years, the research and application of phenolic resin adhesives have become more in-depth with the development of the adhesive industry. The bonding principle and bonding process of adhesives are quite innovative, thus optimizing the performance and cost of adhesives. The field of application is more extensive. Li B. et al.48 successfully prepared bio-based phenol formaldehyde (BPF) with high phenol substitution rate (30–50% wt%) by using low temperature/low pressure depolymerization and hydrolysis of lignin (DHL, Mw ≈ 2000 g mol−1). FTIR measurements indicated that the foamable BPF resoles and foams had structures very similar to the phenol formaldehyde (PF) resole and foam. The prepared 30% BPF foam had similar apparent density (40 kg m−3), compressive strength (0.152 MPa), elastic modulus (2.16 MPa) and thermal conductivity (0.033 W m−1 K−1) with the PF foam. However, 50% BPF foam showed higher apparent density (108 kg m−3), higher compressive strength (0.405 MPa) and elastic modulus (7.56 MPa) than the PF foam. All BPF foams exhibited satisfactory thermal conductivity (0.033–0.040 W m−1 K−1) and cell structure. TGA analysis demonstrated that the BPF foams had excellent thermal stability at temperatures less than 200 °C. Thus, the BPF foams could be utilized as the insulation and fire resistant materials. Jiongjiong Li et al.49 prepared cork powder reinforced LTPFs (CLTPFs) with larch tannin-based rigid phenolic foam plastics (LTPFs) and cork powder as fillers. The chemical structure, cell morphology, compressive mechanical properties, friability, thermal properties, and flame retardancy of the foams were evaluated. The results indicated that the LTPFs showed inferior cell morphology, compressive strength and thermal properties, high friability and high flame retardancy as compared with the neat PF. LTPF10 presented the maximal limiting oxygen index (LOI) of 47.8%. As compared with the unreinforced LTPFs, CLTPFs possessed excellent cell morphology, high compressive strength, low thermal conductivity, low friability, and good thermal properties. At a cork powder dosage of 1 wt%, the compressive strength and modulus of CLTPFs increased by 14.84% and 16.18%, respectively; the pulverization ratio and thermal conductivity of CLTPFs decreased by 18.4% and 32.29%, respectively, as compared with those of the LTPF. In addition, the introduction of cork powder improved the thermal stability of LTPFs. The LOI values of the CLTPFs were slightly lower than those of LTPFs. LTPF reinforced with 1 wt% cork powder exhibited the best combination of the features. Zhao G. et al.50 successfully prepared a phenolic resin dispersed particle gel (PDPG) from a bulk gel by mechanical shearing, using a Waring blender at a shear rate of 1000 rpm to simulate shear degradation at shear time. After 15 min, the viscosity retention rate of PDPG particles was as high as 90%. After 24 h of the shear time, the viscosity retention rate of the PDPG particles was over 90%. The results showed that PDPG particles had good shear resistance and swelling ability, which improved the application performance of phenolic resin in oil fields. The injection of PDPG particles was successfully applied to Chang Qing Oilfield in China for the first time, providing a reference for the controlled production from other similar low-permeability mature oilfields. Mousavi A. et al.51 modified SiO2 with a silane coupling agent (3-aminopropyl) triethoxysilane (APTES) to obtain SiO2–NH2 and then attached to the surface of furfural-modified go (GOFA) to obtain SGOFA. The novolac composite was prepared by incorporating SGOFA into 4 wt% and 8 wt% novolac resin matrices. The results show that the degradation temperature of novolac increased from 445.4 °C to 460.0 °C and 467.4 °C by adding 4 wt% and 8 wt% SGOFA, respectively, which significantly improved the degradation temperature of phenolic resin varnish. Yang G. et al.52 provided a method for the preparation of three-dimensional (3D) graphene/phenolic resin-based porous carbon composites with enhanced conductivity by activation of carbonized 3D graphene/phenolic resin composites and potassium hydroxide material. The 3D graphene/phenolic resin-based porous carbon composite exhibits a high specific capacity of 215.3 F g−1 at 0.1 A g−1, a good specific capacity of 72.9%, and excellent cycle stability in the range 0.1–5 A g−1 (after 5000 cycles, the specific capacitance was decreased to only 3.4%).The prepared porous carbon composite exhibits improved graphitization and enhanced electrical conductivity, opening new ways to design and synthesize 3D graphene/phenolic resin porous carbon composites for various applications such as supercapacitors, catalysts, and fuel cells. Mingzhu S. et al.53 prepared carbon fiber paper-based composite (GCPC) by impregnating carbon fiber in graphene and carbophenol-modified phenolic resin solution (GCP). GCP was characterized by thermal gravimetric analysis (TGA), and the electrical conductivity, mechanical properties, pore distribution, and porosity of GCPC were investigated by a four-probe tester, universal testing machine, microtopography, and porous material analyzer, respectively. The results show that the electrical properties and mechanical strength of GCPC were improved with the increase of graphene and cardanol content. The porosity decreased and the proportion of small holes increased with the increase of graphene, while the porosity increased and the proportion of small holes decreased with the increase of cardanol. When the content of cardanol was 20% (mass fraction), the tensile strength of the composite reached 38.17 MPa, the resistivity reached 18.46 mΩ cm, and the porosity reached 67.46%. Vu C. M. et al.54 successfully synthesized epoxy soybean oil (ESO) with soybean oil as raw material, and then prepared epoxy soybean oil modified phenolic resin (ESO-PR) by reaction of ESO with phenol and formaldehyde. Then, the obtained ESO modified phenolic resin (ESO-PR) was used as resin matrices to fabricate glass-fiber-based composites by using prepreg technique. The chemical structures of both epoxidized soybean oil and phenolic resin modified with epoxidized soybean oil were confirmed with the help of Fourier transform infrared spectrometry (FTIR). The mechanical characteristics of fabricated composite materials examined include the tensile property, flexural property, impact property as well as the mode I interlaminar fracture toughness. The test results showed that at 20 wt% of ESO-PR, the mode I interlaminar fracture toughness for both propagation and initiation, the tensile strength, flexural strength and impact strength were increased by 78.3 and 84.5%, 7.0%; 20.5 and 39.7%, respectively. Menapace C. et al.55 studied the thermal stability of phenolic resin adhesives in commercial brake materials, using thermogravimetric analyzer and Raman spectrometer, to analyze the transitions in phenolic resins when heated to 1200 °C in air. The degradation of the resin alone is compared to a mixture of the masterbatch (i.e., the mixture of the resin and all the brake pad components) and the friction material (obtained by the hot pressing and curing process of the masterbatch). The results show that the initial degradation temperature is reduced from 354 °C for pure resin to 303 °C for cured friction material.
4 Conclusions
China's plywood production areas are mainly concentrated in Hebei, Jiangsu, Shandong and other provinces. In 2017, China's plywood production reached 20.9801 million cubic meters. In 2014, China's plywood export output was the highest: 13.21 million cubic meters. The United States is the top market for Chinese plywood exports, with a market share of 18% in 2016. The market share of Japan and Britain has declined, but in recent years it has maintained between 6% and 7% on average. The usage amount of urea-formaldehyde resin in plywood was much higher than that of phenolic resin. In 2017, the usage amount of urea-formaldehyde resin and phenolic resin in plywood was 10.3851 million tons and 1.501 million tons respectively. The production areas of phenolic resin are mainly concentrated in Shanghai, Zhejiang and Jiangsu. There are only a handful of large-scale manufacturers, and the companies with small capacity are mostly. For example, the annual production capacity of Jinan Sheng Quan Group is 350
000 tons and that of Finland Taier Chemical Company (Nanjing) is 150
000 tons. Henan Bangde Chemical Co., Ltd. and Guangdong Jiangmen Kunyi Resin Materials Technology Co., Ltd. have an annual production capacity of about 10
000 tons. Phenolic resins are widely used in the field of wood processing and plywood. In fireproof, adiabatic heat insulation material use scale is taller. For example, in 2014, the usage of phenolic molding compounds, wood processing and laminates, and refractory and thermal insulation materials were 135
000 tons, 123
000 tons, and 113
000 tons, respectively. Therefore, high shear stress and heat resistance are the main research directions of phenolic resins, which are widely used in composite materials and electronics industries. The phenolic resin adhesives with low free aldehyde and phenol content or no free aldehyde and phenol is still a higher requirement for environmental protection in the process of follow-up research and industrial application.
Conflicts of interest
There are no conflicts to declare.
Acknowledgements
This research has been supported by the Practical Innovation Training Program For College Students (201710298039Z), Outstanding Youth Fund Of Nanjing Forestry University (NLJQ2015-005) and Jiangsu University Superior Discipline Construction Project (PAPD).
References
- J. N. Liu, Q. F. Hou, R. F. Hu and W. Q. Wang, Modification and application of phenolic resin, Pioneering with Sciencce and Technology Monthly, 2016, vol. 29, pp. 134–136 Search PubMed.
- Y. M. Sui, Research progress of phenolic resin adhesive, Heilongjiang Science, 2011, vol. 2, pp. 42–44 Search PubMed.
- B. Xue and X. L. Zhang, Application and development trend of phenolic resin, Thermosetting Resin, 2007, 4, 47–50 Search PubMed.
- Z. J. Wang, Study on the synthesis of phenolic resin, Enterprise Technology Development: Under, 2011, vol. 30, pp. 16–17 Search PubMed.
- H. Q. Li, Principle, technology and application of adhesive, South China University of Technology Press, 2017 Search PubMed.
- C. Mougel, T. Garnier, P. Cassagnau and N. Sintes-Zydowicz, Phenolic foams: A review of mechanical properties, fire resistance and new trends in phenol substitution, Polymer, 2019, 164, 86–117 CrossRef CAS.
- S. Q. Liu, P. C. Ning and Z. M. Ding, Research progress of modified phenolic resin, Thermosetting Resin, 2016, 31, 64–70 Search PubMed.
- P. P. Xu, Y. C. Zhou, Y. X. Yu, K. Y. Yang and J. M. Chang, Synthetic Technology of Heat-Resistant and Toughening Phenol Formaldehyde Resin Co-Modified by Bio-Oil and Boric Acid, Sci. Silvae Sin., 2018, 54, 87–95 Search PubMed.
- Z. D. Xu, Toughening modification of phenolic resin, Ph.D. thesis, Qingdao University of Science and Technology, Qingdao, 2014.
- J. W. Feng, C. B. Yu, W. T. Qing and C. Y. Li, Preparation and properties of phenolic resin-based friction material modified with cardanol-graft-LNBR, Polym. Mater. Sci. Eng., 2016, 32, 164–169 CAS.
- L. P. Rong, X. H. Liu, X. Li, D. Y. Zhang, Y. Zhao, G. Wang and J. H. Zhu, Study on properties of epoxy modified phenolic resin emulsion, Chemistry and Bonding, 2016, vol. 38, pp. 27–30 Search PubMed.
- X. M. Nie, Z. P. Gao and F. Q. Yi, Development of Phenolic Resin/Fiberglass Cloth Composite Prepreg Modified by Nitrile-Butadiene Rubber, Engineering Plastics Applications, 2019, vol. 47, pp. 42–45 Search PubMed.
- K. Hirano and M. Asami, Phenolic resins—100 years of progress and their future, React. Funct. Polym., 2016, 73, 256–269 CrossRef.
- Z. Yi, Modification of phenolic resin adhesive and performance characterization, Ph.D. thesis, Beijing Forestry University, Beijing, 2016.
- Y. Badhe and K. Balasubramanian, Reticulated three-dimensional network ablative composites for heat shields in thermal protection systems, RSC Adv., 2014, 4, 43708–43719 RSC.
- Y. J. Dai, Application of phenolic resin in casting, China Petroleum and Chemical Standards and Quality, 2017, vol. 37, pp. 154–155 Search PubMed.
- S. Rapheepraew and E. Vuthichai, Synthesis of poly (siloxane/double-decker silsesquioxane) via dehydrocarbonative condensation reaction and its functionalization, Polymer, 2016, 86, 113–119 CrossRef.
- C. L. Liu, C. J. Zhou, X. X. Zhao, C. Zhang and G. W. Chen, Research Progress on Silicone-Modified Phenolic Resin, Silicone Mater., 2016, 28, 395–402 Search PubMed.
- D. X. Wang, Z. M. Chen, G. X. Zhang, X. C. Liang, B. H. Li, X. Q. Cao and C. Wang, Study on Fast-Curing Phenol-Formaldehyde Resin Adhesive, Chem. Adhes., 2016, 38, 447–449 Search PubMed.
- R. F. Hu, Q. C. Hu and Q. F. Hou, Synthesis and properties of a molybdenum-modified phenolic resin, Pioneering with Science and Technology Monthly, 2017, vol. 30, pp. 137–138 Search PubMed.
- Y. Zhang, S. H. Shen and Y. J. Liu, The effect of titanium incorporation on the thermal stability of phenol-formaldehyde resin and its carbonization microstructure, Polym. Degrad. Stab., 2013, 98, 514–518 CrossRef CAS.
- L. Zhao, Preparation of high heat resistant phenolic epoxy resin and properties of its composites, Ph.D. thesis, North University of China, Taiyuan, 2017.
- S. Li, Y. Han, F. C. Chen, Z. H. Luo, H. Li and T. Zhao, The effect of structure on thermal stability and anti-oxidation mechanism of silicone modified phenolic resin, Polym. Degrad. Stab., 2016, 124, 68–76 CrossRef CAS.
- J. Chen, W. B. Zhang, J. Liu, H. Y. Ge, M. F. Tian, J. Y. Liu and M. Jing, Improved thermal stability of phenolic resin by graphene-encapsulated nano-SiO2 hybrids, J. Therm. Anal. Calorim., 2019, 135, 2377–2387 CrossRef CAS.
- W. J. Yang, R. Marco, N. Maurizio, K. Jose, M. Piming, W. F. Dong, T. Luigi and P. Debora, Preparation and properties of adhesives based on phenolic resin containing lignin micro and nanoparticles: A comparative study, Mater. Des., 2019, 161, 55–63 CrossRef CAS.
- H. Ren and L. L. Zhu, Comparison of the structural characterization of lignophenols and alkaline lignins before and after methylolation, Wood Sci. Technol., 2018, 52, 1133–1151 CrossRef CAS.
- L. Stéphanie and A. Luc, Chemical modification of lignins: Towards bio-based polymers, Prog. Polym. Sci., 2014, 39, 1266–1290 CrossRef.
- T. Marion, M. Uwe, K. Andreas, Z. R. Edith and L. Herfried, Review on impregnation issues in laminates manufacture: opportunities and risks of phenol substitution by lignins or other natural phenols in resins, Eur. J. Wood Wood Prod., 2017, 75, 853–876 CrossRef.
- G. Foyer, B. H. Chanfi, B. Boutevin, S. Caillol and G. David, New method for the synthesis of formaldehyde-free phenolic resins from lignin-based aldehyde precursors, Eur. Polym. J., 2016, 74, 296–309 CrossRef CAS.
- Y. S. Zhang, Z. S. Yuan and C. Xu, Engineering Biomass into Formaldehyde-free Phenolic Resin for Composite Materials, AIChE J., 2015, 4, 1275–1283 CrossRef.
- B. Benoit, C. Sylvain, R. Jean-Jacques and L. Vincent, Cardanol-Based and Formaldehyde-Free Flexible Phenolic Networks, Eur. J. Lipid Sci. Technol., 2018, 7, 1800175–1800196 Search PubMed.
- G. Foyer, B. H. Chanfi, D. Virieux, G. David and S. Caillol, Aromatic dialdehyde precursors from lignin derivatives for the synthesis of formaldehyde-free and high char yield phenolic resins, Eur. Polym. J., 2016, 77, 65–74 CrossRef CAS.
- G. Masoumeh, M. Arunjunai Raj, V. H. Hendrikus, L. Falk and K. Johannes, Paper-based laminates produced with kraft lignin-rich phenol–formaldehyde resoles meet requirements for outdoor usage, Eur. J. Wood Wood Prod., 2018, 76, 481–487 CrossRef.
- K. Somayyeh, N. S. Chen, B. Hamid and N. Mojgan, Comparative analysis of different lignins as phenol replacement in phenolic adhesive formulations, Ind. Crops Prod., 2018, 125, 520–528 CrossRef.
- S. Alexander, S. Fokko, S. Bodo and L. Ralph, Lignins from enzymatic hydrolysis and alkaline extraction of steam refined poplar wood: Utilization in lignin-phenol-formaldehyde resins, Ind. Crops Prod., 2016, 85, 300–308 CrossRef.
- W. Zhang, Y. F. Ma, Y. Z. Xu, C. P. Wang and F. X. Chu, Lignocellulosic ethanol residue-based lignin–phenol–formaldehyde resin adhesive, Int. J. Adhes. Adhes., 2013, 40, 11–18 CrossRef CAS.
- M. Z. Zhao, J. L. Jing, Y. C. Zhu, X. M. Yang, X. F. Wang and Z. C. Wang, Preparation and performance of lignin–phenol–formaldehyde adhesives, Int. J. Adhes. Adhes., 2016, 163–167 CrossRef CAS.
- G. L. Zhang, Application of phenolic resin in adhesive industry, Chemical Industry Management, 2018, vol. 36, pp. 18–19 Search PubMed.
- Y. J. Qi, Y. Xu and X. J. Ma, Application prospect and development present situation of industry of plywood, Wood Processing Machinery, 2016, vol. 27, pp. 48–50 Search PubMed.
- R. Tian, The Research and optimization
of Chinese Piywood Export Market Structure, Ph.D. thesis, Inner Mongolia University of Technology, Neimenggu, 2017.
- J. Li, The Study on China Export Trade of Plywood, Ph.D. thesis, Beijing Forestry University, Beijing, 2018.
- Z. Z. Lv, Problems and countermeasures in export trade of Chinese plywood manufacturers, Foreign Trade Practice, 2018, vol. 4, pp. 52–55 Search PubMed.
- Y. Liu and D. L. Miao, The Reason for High Worldwide Market Shares of China's Plywood and Its Analysis, Forestry Economics, 2013, vol. 33, pp. 447–452 Search PubMed.
- Y. S. Wang, The Empirical Study On Plywood Export of China, Ph.D. thesis, Beijing Forestry University, Beijing, 2011.
- G. H. Zhang, Analysis of Production and Market Phenolic Resin in China, Chemical Industry, 2018, 35, 44–47 Search PubMed.
- Y. M. Zhu, R. Z. Yin, X. T. Pan, Y. Liu and W. Yang, Progress of domestic and foreign phenolic resin and plastics industry in 2010-2011, Thermosetting Resins, 2012, vol. 2, pp. 58–63 Search PubMed.
- J. Ning, Progress of the World's Plastics Industry in 2016-2017 (II), Plast. Ind., 2018, 46, 10–28 Search PubMed.
- B. Li, Y. Y. Wang, N. B. Mahmood, Z. S. Yuan, J. Schmidt and C. B. Xu, Preparation of bio-based phenol formaldehyde foams using depolymerized hydrolysis lignin, Ind. Crops Prod., 2017, 97, 409–416 CrossRef CAS.
- J. J. Li, A. B. Zhang, S. F. Zhang, Q. Gao, W. Zhang and J. Z. Li, Larch tannin-based rigid phenolic foam with high compressive strength, low friability, and low thermal conductivity reinforced by cork powder, Composites, Part B, 2019, 156, 368–377 CrossRef CAS.
- G. Zhao, Q. You, J. P. Tao, C. L. Gu, A. Hafiz, L. P. Ma and C. L. Dai, Preparation and application of a novel phenolic resin dispersed particle gel for in-depth profile control in low permeability reservoirs, J. Pet. Sci. Eng., 2018, 161, 703–714 CrossRef CAS.
- M. Alireza, R. M. Hossein, S. K. Mehdi, S. Sina and A. Amin, Grafting of silica nanoparticles at the surface of graphene for application in novolac-type phenolic resin hybrid composites, Mater. Chem. Phys., 2018, 216, 468–475 CrossRef.
- G. P. Yang, Y. H. Wang, S. Y. Zhou, S. P. Jia, H. Q. Xu and J. B. Zang, Graphene/phenolic resin-based porous carbon composites with improved conductivity prepared via in situ polymerization in graphene hydrogels, J. Mater. Sci., 2018, 54, 2222–2230 CrossRef.
- M. Z. Sang, Y. H. Meng, S. H. Wang and Z. Long, Graphene/cardanol modified phenolic resin for the development of carbon fiber paper-based composites, RSC Adv., 2018, 8, 24464–24469 RSC.
- C. Manh Vu, D. Duc Nguyen, L. Hoang Sinh, H. Jin Choi and T. Duc Pham, Improvement the mode I interlaminar fracture toughness of glass fiber reinforced phenolic resin by using epoxidized soybean oil, Polym. Bull., 2018, 75, 4769–4782 CrossRef.
- C. Menapace, M. Leonardi, M. Secchi, A. Bonfanti, S. Gialanella and G. Straffelini, Thermal behavior of a phenolic resin for brake pad manufacturing, J. Therm. Anal. Calorim., 2019, 137, 759–766 CrossRef CAS.
|
This journal is © The Royal Society of Chemistry 2019 |
Click here to see how this site uses Cookies. View our privacy policy here.