DOI:
10.1039/C9RA05975J
(Paper)
RSC Adv., 2019,
9, 28886-28893
Impact of an aryl bulky group on a one-pot reaction of aldehyde with malononitrile and N-substituted 2-cyanoacetamide†
Received
1st August 2019
, Accepted 29th August 2019
First published on 13th September 2019
Abstract
In this study, we successfully explored the effect of steric hindrance on the one-pot reaction of different aryl aldehydes with malononitrile and N-substituted 2-cyanoacetamide in the presence of piperidinium acetate as the catalyst. It involved the Knoevenagel condensation of the aldehyde and malononitrile to produce arylidene malononitrile as an intermediate, which was further treated with N-substituted 2-cyanoacetamide to give 6-amino-2-pyridone-3,5-dicarbonitrile derivatives when the less steric bulky group was involved. High steric hindrance changed the earlier reaction route and gave N-substituted 2-cyanoacrylamides via a slower route.
Introduction
The steric effect of bulky groups is a significant part of organic synthetic theory, although the steric bulk of the group may cause either a desirable or undesirable impact on organic synthesis. For example, bulky groups reduce the rate of SN2 reactions because they increase the free energy of activation for the reaction.1 In contrast, tetramethylpiperidine derivatives with sterically hindered amine groups are used as stabilizers in polymers.2 Bulky groups can prevent or reduce reactivity because of their steric hindrance. The steric hindrance of the bulky group also affects the selectivity of the reaction, such as the reaction of an amine with acetylanthranil.3 In this example, acetylanthranil reacted with amines via different pathways according to the size of the amine. The directive effect of steric hindrance is also observed in the nitration and sulfonation in p-cymene.4 Thus, steric hindrance sometimes plays a vital role in the reaction mechanism and it governs the formation of new bonds that decides the structural core of the product(s).
In this study, we tried to explore the effect of the steric bulk from the aryl groups of aldehydes in the one-pot reaction of aryl aldehyde with malononitrile and N-substituted-2-cynoacetamide. The extent of steric hindrance mainly depends on the bulkiness of the aromatic ring as well as another substituent. Therefore, to check the effect of steric hindrance on the synthesis, we chose aldehydes that had different number of rings, which consequently had different steric bulk that might affect the molecular reactivity.
We performed this reaction via a one-pot strategy, which has been reported in many reactions,5–8 because it has certain advantages based on green chemistry concepts such as the reduction of chemical waste, workup procedure, purification process, and time, which make the reaction greener.9 In the literature, the one-pot reaction of aryl aldehyde, malononitrile and N-substituted 2-cyanoacetamide is mostly reported to use piperidine10–12 as a catalyst. Other bases, such as potassium carbonate13 and triethylamine,14 have also been reported. A recyclable and reusable catalyst is more suitable for green synthesis. Many ionic liquids or salts have been reported as reusable catalysts without the loss of their catalytic activities.8,15–19 Herein, we performed the one-pot reaction of aryl aldehyde, malononitrile and a N-benzyl-2-cyanoacetamide derivative using piperidinium acetate as a reusable catalyst. The reactants used in this reaction and other produced impurities are more soluble in diethyl ether or methanol, whereas the product is less soluble in diethyl ether or methanol. This helps to obtain the pure product by filtration and drying to avoid column purification, which makes the synthesis route greener and sustainable.20
Results and discussion
Initially, we selected benzaldehyde 1a, malononitrile 2, and N-benzyl-2-cyanoacetamide 4a as model substrates. The reaction between these compounds was catalyzed by piperidinium acetate, and it was performed using different mol% of a catalyst and different solvents to check their efficiency. The best result was observed when 10 mol% of piperidinium acetate and ethanol (as a solvent) were used (Table 1).
Table 1 Screening of solvent, mol% of catalyst and effect of temperature on the model reactiona
Entry |
Catalyst (mol%) |
Solvent |
Temperature (°C) |
Yield (%) of 5bb |
Reaction was performed for 1 hour by employing 3 mmol benzaldehyde, 3 mmol malononitrile, 3 mmol N-benzyl-2-cyanoacetamide with piperidinium acetate as a catalyst. Isolated yield. |
1 |
10 |
Acetonitrile |
Reflux |
51 |
2 |
10 |
THF |
Reflux |
54 |
3 |
10 |
DCM |
Reflux |
48 |
4 |
00 |
Ethanol |
Reflux |
Trace |
5 |
05 |
Ethanol |
Reflux |
47 |
6 |
10 |
Ethanol |
Room temperature |
Trace |
7 |
10 |
Ethanol |
Reflux |
81 |
8 |
15 |
Ethanol |
Reflux |
81 |
This synthesis was also performed at room temperature up to the reflux temperature. At room temperature, instead of 5a, benzylidenemalononitrile 3a was obtained; however, at a reflux (78 °C) temperature, the desired compound 5a was successfully obtained. From this experience, first, we synthesized benzylidenemalononitrile 3a from benzaldehyde 1a and malononitrile 2 at room temperature within 5 min. N-benzyl-2-cyanoacetamide 4a was then directly added to the reaction mixture without an isolation or purification process, and the reaction was further performed under reflux for 1 h to synthesize compound 5a by a one-pot synthesis (Scheme 1). We successfully synthesized its other derivatives 5b, 5c and 5d in good yields via the same method, employing the chloro and dichloro derivatives of N-benzyl-2-cyanoacetamide (Table 2).
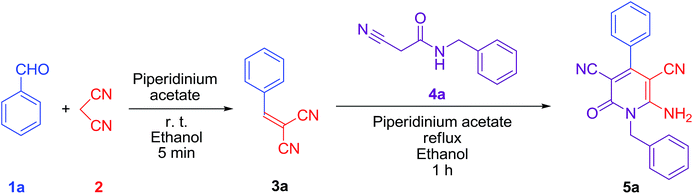 |
| Scheme 1 One-pot synthesis of 6-amino-1-benzyl-2-oxo-4-phenyl-1,2-dihydropyridine-3,5-dicarbonitrile 5a from 3 mmol benzaldehyde 1a, 3 mmol malononitrile 2 and 3 mmol N-benzyl-2-cyanoacetamide 4a catalyzed by 10 mol% piperidinium acetate. | |
Table 2 One-pot synthesis of 6-amino-2-pyridone-3,5-dicarbonitriles a
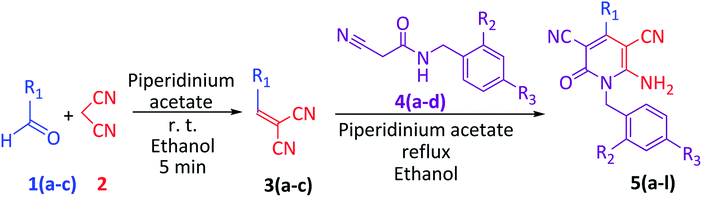
|
Entry |
R1 |
R2 |
R3 |
Product |
Time |
% Yieldb |
Reaction condition: 3 mmol aryl aldehyde 1, 3 mmol malononitrile 2, 3 mmol N-benzyl-2-cyanoacetamide 4 and 10 mol% piperidinium acetate as catalyst. Isolated yield. |
1 |
Phenyl |
H |
H |
5a |
1 h |
81 |
2 |
Phenyl |
Cl |
H |
5b |
1 h |
79 |
3 |
Phenyl |
H |
Cl |
5c |
1 h |
80 |
4 |
Phenyl |
Cl |
Cl |
5d |
1 h |
77 |
5 |
4-Biphenyl |
H |
H |
5e |
1.5 h |
78 |
6 |
4-Biphenyl |
Cl |
H |
5f |
1.5 h |
76 |
7 |
4-Biphenyl |
H |
Cl |
5g |
1.5 h |
77 |
8 |
4-Biphenyl |
Cl |
Cl |
5h |
1.5 h |
76 |
9 |
1-Naphthyl |
H |
H |
5i |
1.5 h |
66 |
10 |
1-Naphthyl |
Cl |
H |
5j |
1.5 h |
64 |
11 |
1-Naphthyl |
H |
Cl |
5k |
1.5 h |
64 |
12 |
1-Naphthyl |
Cl |
Cl |
5l |
1.5 h |
62 |
We produced 6-amino-1-benzyl-4-(biphenyl-4-yl)-2-oxo-1,2-dihydropyridine-3,5-dicarbonitrile 5e and its derivatives 5f, 5g and 5h via a two-step one-pot synthesis in good yield without any notable differences from the synthesis of 5a (Table 2).
The structure of the as-synthesized product was confirmed via mass analysis, 1H-NMR and 13C-APT analysis. The 1H-NMR spectra showed a signal at δ 5.2–5.4 ppm, which confirmed the presence of the CH2 moiety from the benzyl group. In addition, the 1H-NMR spectra showed signals between δ 6.8–7.9 ppm and at δ 8.4–8.6 ppm, which confirmed the presence of aromatic protons and the NH2 group, respectively. In the 13C-APT spectra, signals appeared at δ 44 ppm, δ 75–77 ppm and δ 87–89 ppm, confirming the presence of the CH2 moiety from the benzylic carbon, C-3 and C-5, respectively. In addition, two signals appeared at δ 115 ppm and 116 ppm, which confirmed the CN group. The 13C-APT spectra also displayed signals at δ 124–142 ppm, δ 156 ppm, δ 158 ppm and δ 160 ppm, which confirmed the presence of the aromatic carbon, C-6, C-2 and C-4 of 2-pyridone, respectively. One of the as-synthesized products, 5a, was confirmed by single-crystal X-ray diffraction. The single crystal structural data of 5a indicated that it crystallized with Z = 4 and the centrosymmetric space group P21/c.
In addition, we chose 1-naphthaldehyde 1c to observe the steric effect of two fused aromatic rings on product formation. The desired product 6-amino-1-benzyl-4-(naphthalen-1-yl)-2-oxo-1,2-dihydropyridine-3,5-dicarbonitrile 5i and its derivatives 5j, 5k and 5l were obtained with other impurities, which were easily removed by washing with ice-cold methanol. The product yields for these compounds were comparatively lower than their phenyl and biphenyl derivatives 5a–5h.
Interestingly, the 1H-NMR spectrum of 5i exhibited two signals at δ 5.32 ppm and δ 5.44 ppm, confirming the presence of benzylic protons, which showed geminal coupling with coupling constants of 16.8 Hz and 16.4 Hz, respectively. This is only possible when two benzylic protons
are magnetically nonequivalent, which means that the benzyl group at N-1 in the 2-pyridone ring was sterically hindered by the naphthyl group at C-4 and the free rotation of the benzyl group was restricted. So, the two protons of the methylene group could not interchange and were coupled to each other. The naphthyl and benzyl groups stayed on opposite sides, which was observed in the single-crystal X-ray diffraction study. The single crystal structural data of 5i indicate that it crystallized with Z = 8, and the polar space group Aea2. In contrast, for complexes 5a–5h, the benzyl group at N-1 in the 2-pyridone ring was not sterically hindered with the phenyl or biphenyl group at C-4. Thus, the benzyl group could freely rotate. This was confirmed by the appearance of a singlet peak at δ 5.2–5.4 ppm in their 1H-NMR spectra. Thus, the steric effect of the naphthyl group on the benzyl group was observed and affected the product yields of 5i–5l.
Furthermore, to apply more steric hindrance, we chose 9-anthraldehyde 1d as a reactant and it was first reacted with malononitrile 2. 9-Anthracenylmethylenemalononitrile 3d was produced in the first step, and then it was reacted with N-benzyl-2-cyanoacetamide 4a. Due to a high extent of steric hindrance, 3-(9-anthracenyl)-N-benzyl-2-cyanoacrylamide 6a was produced instead of the desired product. One important observation is that this conversion was completed in 9 h. The same outcome was found in the synthesis of derivatives 6b, 6c, and 6d.
The structures of the produced compounds 6a–6d were confirmed by mass analysis, 1H-NMR and 13C-APT analysis. 1H-NMR spectra showed a doublet signal at δ 4.54–4.89 ppm, which indicated the presence of benzylic protons. In addition, aromatic protons gave signals between δ 7.31 and 8.81 ppm. A singlet signal was observed at δ 9.10–9.14 ppm, which confirmed the presence of vinylic protons. Finally, a triplet signal at δ 9.46–9.47 ppm confirmed the presence of NH next to the benzylic protons. In the 13C-NMR spectra, signals appearing at δ 40–44 ppm confirmed the presence of the benzylic carbon. In addition, signals appearing near δ 115 ppm, δ 117 ppm and δ 124–138 ppm indicated the vinylic carbon next to the nitrile group, the nitrile carbon and the aromatic carbons, respectively. Finally, the signals at δ 150 ppm and δ 160 ppm indicated the presence of the vinylic carbon next to the anthracene ring and the presence of the carbonyl carbon, respectively.
From the LC-MS experiment of 6a, the LC chromatogram showed two distinct peaks with retention times at 6.05 and 6.19 min. So, the isolated product 6a consisted of two components, but the mass spectrum of both components confirmed that both components have the same molecular weight and are geometrical isomers of each other. In general, due to the nonplanarity result from steric hindrance, the Z isomer has a higher chromatographic mobility compared to the E isomer. So, the Z isomer typically elutes first with a lower retention time than the E isomer.21 The retention times with the calculated% area of the isomers of 6a–6d are summarized in Table 3.
Table 3 One-pot synthesis of N-substituted 3-(9-anthracenyl)-2-cyanoacrylamidea
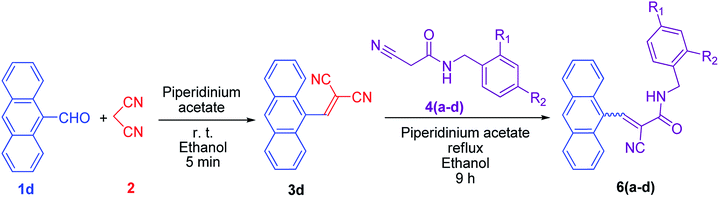
|
Entry |
R1 |
R2 |
Product |
% Yieldb |
Z isomer |
E isomer |
Retention timec (min) |
% Areac |
Retention timec (min) |
% Areac |
Reaction condition: 3 mmol 9-anthraldehyde 1d, 3 mmol malononitrile 2, 3 mmol N-benzyl-2-cyanoacetamide 4 and 10 mol% piperidinium acetate as catalyst. Isolated yield. Calculated from LC chromatogram. |
1 |
H |
H |
6a |
88 |
6.05 |
24 |
6.19 |
76 |
2 |
Cl |
H |
6b |
87 |
6.28 |
32 |
6.42 |
68 |
3 |
H |
Cl |
6c |
86 |
6.32 |
28 |
6.45 |
72 |
4 |
Cl |
Cl |
6d |
87 |
6.64 |
22 |
6.73 |
78 |
Mechanistically, the deprotonation of malononitrile (II) by piperidinium acetate (I) gives the carbanion IV. It attacks the carbonyl group of aryl aldehyde (V) as the aldol reaction gives the arylidene malononitrile (VIII) by the loss of water and reacquired piperidinium acetate with the completion of one cycle. For the conformation, we isolated this intermediate (VIII) from the synthesis of 5i (3c). The spectral analysis of the intermediate (see ESI†) confirms the structure. The deprotonation of N-substituted-2-cyanoacetamide (IX) by piperidinium acetate (I) gives the carbanion X. It attacks arylidene malononitrile (VIII) to give (XI). In the following steps, steric size plays a role. Normally, carbanion X reversibly abstracts a proton from the piperidinium ion to give XII and piperidine, which quickly reacts with acetic acid (already present in the reaction mixture), and gives back piperidinium acetate upon the completion of the second cycle. A lone pair on the nitrogen of XII attacks one of the nitrile groups to generate a cyclic ring. This step is followed by an amine–enamine tautomerization and auto-oxidation to produce the final product XV. However, this is possible only if steric hindrance is not affecting the reaction. If the steric bulk of R1 is large, then it is sterically hindered from the attack of the lone pair from the nitrogen present in XII. Thus, the reaction does not proceed further via cyclization and XII reversibly converts back to XI. Now, the reaction proceeds to another possible pathway ‘b’, which is too slow compared to the former route ‘a’. This alternative route, as shown in Scheme 2, gives a different type of product XVIII and gives back piperidinium acetate with completion of the cycle.
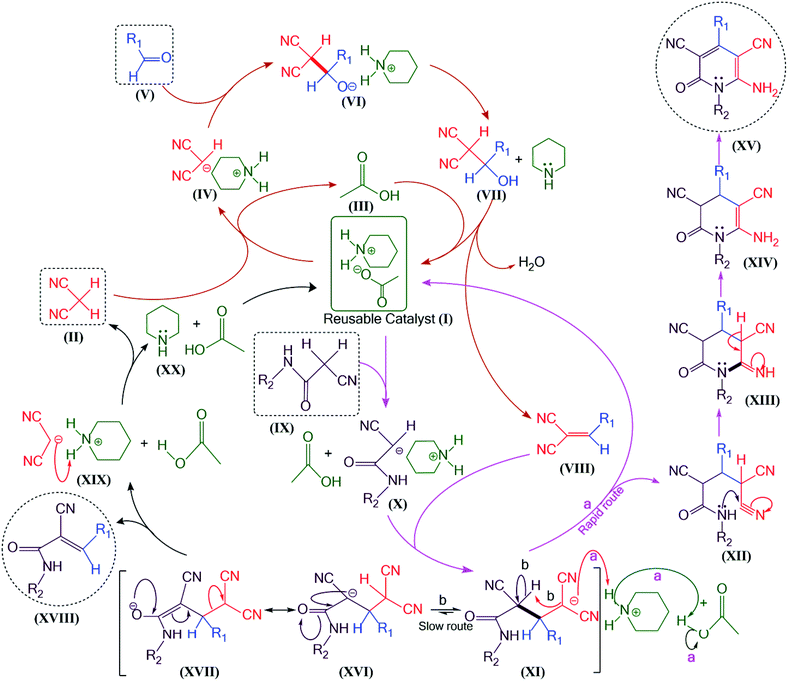 |
| Scheme 2 Plausible mechanism for the one-pot reaction of aryl aldehyde, malononitrile and N-substituted 2-cyanoacetamide catalyzed by piperidinium acetate. The thick line indicates the formation of a new bond. Dotted boxes indicate the reactant and dotted circles indicate the product of the reaction. | |
If the reaction is performed via the multicomponent strategy, in which aldehyde, malononitrile and N-substituted-2-cyanoacetamide reflux together in the presence of piperidinium acetate and ethanol as a solvent for the same time that is required for the two-step one-pot synthesis, the same product will be produced. Mekheimer et al. proposed a mechanism for this,13 in which the aldehyde simultaneously reacts with malononitrile and the 2-cyanoacetamide derivative to give 2-arylidiene malononitrile and the 3-aryl-2-cyano-acrylamide derivative, respectively. The 2-arylidene malononitrile then reacts with the 2-cyanoacetamide derivative and the 3-aryl-2-cyano-acrylamide derivative reacts with malononitrile to give 6-amino-2-pyridone-3,5-dicarbonitrile derivatives. However, we preferred the two-step one-pot synthesis for the steric hindrance study due to the bulky group and the ensured formation of arylidene malononitrile in the first step (it is rapid and done at room temperature). In the next step, arylidene malononitrile is converted to either 2-pyridone or in the case of acrylamide, it depends on the steric size of the aromatic ring.
Recycling of catalyst
After the completion of a reaction, the reaction mixture was poured into water and stirred well. The product was then extracted with ethyl acetate using a separating funnel. For the rapid separation of ethyl acetate and an aqueous layer, brine was added. Then, the aqueous solution was charged and separated in the rotatory evaporator. The mixture of ethanol and water was evaporated at reduced pressure at 60–80 °C, and the residue was collected. Piperidinium acetate was extracted from the residue by ethanol and used as a catalyst for a subsequent synthesis of the same product. The obtained yield of product 5a up to the 4th cycle with reaction time is demonstrated in Fig. 1. The spectral analyses of the fresh catalyst and of the catalyst obtained after the 4th cycle are given in SI.†
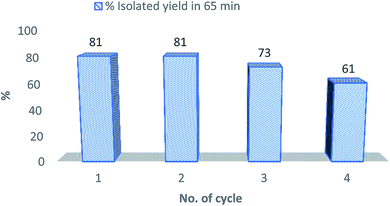 |
| Fig. 1 Recycling of catalyst in the synthesis of 5a. | |
Conclusions
We successfully synthesized 6-amino-2-pyridone-3,5-dicarbonitrile derivatives via a one-pot reaction of aryl aldehyde, malononitrile and N-substituted 2-cyanoacetamide using 10 mol% piperidinium acetate and ethanol as a solvent with a focus on a greener approach (e.g., mild condition, shorter reaction time, good yields, use of reusable catalyst and no column chromatographic purifications). We found a greater steric effect in 1-naphthaldehyde compared to benzaldehyde and biphenyl-4-carboxaldehyde, and it also affected the product yield. Due to the high steric hindrance, the one-pot two-step reaction using 9-anthraldehyde, malononitrile and N-substituted 2-cyanoactamide gave N-substituted 3-(9-anthracenyl)-2-cyanoacrylamide via an alternative slower reaction route instead of the former rapid route. Thus, the selectivity of the one-pot reaction depends on the steric size of the aldehydes. A single crystal study of 5a and 5i showed that 5a crystallized in the monoclinic crystal system with the centrosymmetric space group P21/c, whereas 5i crystallized in the orthorhombic crystal system with the polar space group Aea2.
Experimental section
General information
All chemicals were purchased from commercially available sources and used without further purification. N-Benzyl-2-cyanoacetamide and its chloro derivatives were synthesized following a previously reported procedure.22 Melting points were determined by the open capillary tube method and are uncorrected. The mass analysis was collected on a MS – Agilent 6120 quadrupole. 1H NMR and 13C-APT spectral analyses were recorded using a BRUKER AVANCE II 400 NMR spectrometer using DMSO-d6 as the solvent and TMS as an internal standard. Abbreviations used for the NMR signal are as follows: s = singlet, d = doublet, t = triplet, dd = double doublet, m = multiplet. The chemical shifts are expressed in parts per million and coupling constants (J) are provided in Hertz.
General procedure for the synthesis of piperidinium acetate
In a 100 ml round-bottom flask, an aqueous solution of 0.1 mol piperidine was taken. The solution was then cooled in an ice bath with continuous stirring. An aqueous solution of 0.1 mol acetic acid was then added dropwise through a dropping funnel. After the completion of the addition, water was removed using a rotary evaporator. The resultant product was stored in a vacuum desiccator.
Piperidinium acetate. White solid (14.321 g, 98%) mp 104–106 °C, 1H-NMR (400 MHz, D2O) δ 1.55 (s, 2H), 1.66 (s, 2H), 1.81 (s, 3H), 3.04 (s, 2H); 13C{1H}-APT (100 MHz, D2O) δ 21.5, 22.0, 23.0, 44.5, 180.6.
General scheme and procedure for the synthesis of 5a–5l
A mixture of 3 mmol aldehyde, 3 mmol malononitrile and 0.3 mmol (10 mol%) piperidinium acetate in 10 ml ethanol was stirred at room temperature for 5 minutes to produce arylidene malononitrile. Then, 3 mmol of N-substituted 2-cyanoacetamide was added to the reaction mixture and refluxed for the required time (1–2 h). Upon completion as monitored by TLC, the reaction mixture was cooled and poured into 30 ml water, and then it was extracted in 15 ml ethyl acetate. For purification, the product was stirred in 5 ml ice-cold diethyl ether (more preferable) or 3 ml methanol and filtered. If impurities were still present, they were removed with methanol and the pure product was obtained.
6-Amino-1-benzyl-2-oxo-4-phenyl-1,2-dihydropyridine-3,5-dicarbonitrile (5a). White solid (0.795 g, 81%); mp 236–238 °C; 1H-NMR (400 MHz, DMSO-d6 and CD3OD) δ 5.33 (s, 2H), 7.24 (d, J = 7.2 Hz, 2H), 7.31 (d, J = 7.2 Hz, 1H), 7.38 (t, J = 6.8 Hz, 2H), 7.55–7.57 (m, 5H), 8.45 (s, 2H); 13C{1H}-APT (100 MHz, DMSO-d6 and CD3OD) δ 44.7, 75.6, 87.5, 115.7, 116.4, 126.5, 127.4, 128.0, 128.5, 128.6, 130.3, 134.4, 134.5, 156.5, 159.4, 160.8; MS (ESI-TOF) m/z: [M + H]+ calcd for C20H14N4O 327.12 found: 327.13; elemental analysis calcd (%) for C20H14N4O: C, 73.61; H, 4.32; N, 17.17; O, 4.90 found: C, 73.40; H, 4.32; N, 17.18.
6-Amino-1-(2-chlorobenzyl)-2-oxo-4-phenyl-1,2-dihydropyridine-3,5-dicarbonitrile (5b). White solid (0.852 g, 79%); mp 252–254 °C; 1H-NMR (400 MHz, DMSO-d6) δ 5.26 (s, 2H), 6.92 (d, J = 6.8 Hz, 2H), 7.32–7.37 (m, 2H), 7.53–7.57 (m, 6H), 8.56 (s, 2H); 13C{1H}-APT (100 MHz, DMSO-d6) δ 44.3, 75.8, 87.3, 115.8, 116.4, 125.3, 127.4, 128.0, 128.6, 128.8, 129.5, 130.3, 131.8, 132.1, 134.6, 156.9, 159.2, 161.0; MS (ESI-TOF) m/z: [M + H]+ calcd for C20H13ClN4O 361.08; found: 361.10; elemental analysis calcd (%) for C20H13ClN4O: C, 66.58; H, 3.63; Cl, 9.83; N, 15.53; O, 4.43 found: C, 66.68; H, 3.63; N, 15.54.
6-Amino-1-(4-chlorobenzyl)-2-oxo-4-phenyl-1,2-dihydropyridine-3,5-dicarbonitrile (5c). White solid (0.864 g, 80%); mp 224–226 °C; 1H-NMR (400 MHz, DMSO-d6 and CD3OD) δ 5.30 (s, 2H), 7.27 (d, J = 8.4 Hz, 2H), 7.44 (d, J = 8.4 Hz, 2H), 7.54–7.57 (m, 5H), 8.46 (s, 2H); 13C{1H}-APT (100 MHz, DMSO-d6 and CD3OD) δ 44.3, 115.7, 116.4, 128.0, 128.4, 128.6, 130.3, 132.0, 133.5, 134.5, 156.5, 159.4, 160.8; MS (ESI-TOF) m/z: [M + H]+ calcd for C20H13ClN4O 361.08 found: 361.10; elemental analysis calcd (%) for C20H13ClN4O: C, 66.58; H, 3.63; Cl, 9.83; N, 15.53; O, 4.43 found: C, 66.68; H, 3.62; N, 15.53.
6-Amino-1-(2,4-dichlorobenzyl)-2-oxo-4-phenyl-1,2-dihydropyridine-3,5-dicarbonitrile (5d). White solid (0.910 g, 77%); mp 296–298 °C; 1H-NMR (400 MHz, DMSO-d6) δ 5.20 (s, 2H), 6.97 (d, J = 8.4 Hz, 1H), 7.39 (dd, J = 2 Hz, J = 8.4 Hz, 1H), 7.53–7.59 (m, 5H), 7.73 (d, J = 2 Hz, 1H), 8.56 (s, 2H); 13C{1H}-APT (100 MHz, DMSO-d6) δ 44.1, 75.9, 87.2, 115.8, 116.3, 126.8, 127.4, 128.0, 128.6, 128.9, 130.3, 131.2, 132.4, 133.1, 134.6, 156.9, 159.2, 161.0; MS (ESI-TOF) m/z: [M + H]+ calcd for C20H12C12N4O 395.04 found: 395.06; elemental analysis calcd (%) for C20H12C12N4O: C, 60.78; H, 3.06; Cl, 17.94; N, 14.18; O, 4.05 found: C, 60.79; H, 3.06; N, 14.12.
6-Amino-1-benzyl-4-(biphenyl-4-yl)-2-oxo-1,2-dihydropyridine-3,5-dicarbonitrile (5e). Yellowish white solid (0.940 g, 78%); mp 240–242 °C; 1H-NMR (400 MHz, DMSO-d6) δ 5.36 (s, 2H), 7.27 (d, J = 7.2 Hz, 2H), 7.32 (t, J = 7.2 Hz, 1H), 7.38–7.41 (m, 2H), 7.45 (d, J = 7.2 Hz, 1H), 7.51–7.55 (m, 2H), 7.66 (d, J = 7.6 Hz, 2H), 7.80 (d, J = 7.6 Hz, 2H), 7.89 (d, J = 7.6 Hz, 2H), 8.46 (s, 2H); 13C{1H}-APT (100 MHz, DMSO-d6) δ 45.2, 76.1, 87.9, 116.4, 117.0, 127.0, 127.2, 127.3, 127.9, 128.6, 129.0, 129.3, 129.36, 129.41, 129.6, 134.0, 135.0, 139.5, 142.3, 157.1, 160.0, 160.9; MS (MMES + APCI) m/z: calcd for C26H18N4O 402.15 found: 402.6; elemental analysis calcd (%) for C26H18N4O: C, 77.59; H, 4.51; N, 13.92; O, 3.98 found: C, 77.78; H, 4.50; N, 13.94.
6-Amino-4-(biphenyl-4-yl)-1-(2-chlorobenzyl)-2-oxo-1,2-dihydropyridine-3,5-dicarbonitrile (5f). Light peach solid (0.993 g, 76%); mp 270–272 °C; 1H-NMR (400 MHz, DMSO-d6) δ 5.30 (s, 2H), 6.97 (s, 1H), 7.39 (s, 2H), 7.48 (s, 1H), 7.57 (s, 3H), 7.71 (s, 2H), 7.83 (s, 2H), 7.93 (s, 2H), 8.6 (s, 2H); 13C{1H}-APT (100 MHz, DMSO-d6) δ 44.7, 76.3, 116.5, 117.1, 125.8, 127.2, 127.9, 128.6, 129.3, 129.6, 130.0, 132.6, 134.1, 139.5, 142.4, 157.5, 159.8, 161.1; MS (MMES + APCI) m/z: calcd for C26H17ClN4O 436.11 found: 436.6; elemental analysis calcd (%) for C26H17ClN4: C, 71.48; H, 3.92; Cl, 8.11; N, 12.82; O, 3.66 found: C, 71.47; H, 3.91; N, 12.81.
6-Amino-4-(biphenyl-4-yl)-1-(4-chlorobenzyl)-2-oxo-1,2-dihydropyridine-3,5-dicarbonitrile (5g). Light peach solid (1.008 g, 77%); mp 272–274 °C; 1H-NMR (400 MHz, DMSO-d6) δ 5.32 (s, 2H), 7.29 (d, J = 8.4 Hz, 2H), 7.36–7.46 (m, 3H), 7.52 (t, J = 7.6 Hz, 2H), 7.65 (d, J = 8 Hz, 2H), 7.79 (d, J = 7.6 Hz, 2H), 7.89 (d, J = 8.4 Hz, 2H), 8.49 (s, 2H); 13C{1H}-APT (100 MHz, DMSO-d6) δ 44.2, 75.7, 87.3, 115.9, 116.5, 126.7, 126.8, 128.1, 128.4, 128.6, 128.8, 129.0, 132.0, 133.5, 133.6, 139.0, 141.8, 156.6, 159.5, 160.4; MS (MMES + APCI) m/z: calcd for C26H17ClN4O 436.11 found: 436.6; elemental analysis calcd (%) for C26H17ClN4O: C, 71.48; H, 3.92; Cl, 8.11; N, 12.82; O, 3.66 found: C, 71.47; H, 3.91; N, 12.80.
6-Amino-4-(biphenyl-4-yl)-1-(2,4-dichlorobenzyl)-2-oxo-1,2-dihydropyridine-3,5-dicarbonitrile (5h). Light peach solid (1.071 g, 76%); mp 240–242 °C; 1H-NMR (400 MHz, DMSO-d6) δ 5.22 (s, 2H), 6.99 (d, J = 8.4 Hz, 1H), 7.39–7.45 (m, 2H), 7.59 (t, J = 7.6 Hz, 2H), 7.66 (d, J = 8.4 Hz, 2H), 7.74 (d, J = 2 Hz, 1H), 7.89 (d, J = 7.6 Hz, 2H), 7.90 (d, J = 8 Hz, 2H), 8.60 (s, 2H); 13C{1H}-APT (100 MHz, DMSO-d6) δ 44.2, 75.8, 87.4, 115.9, 116.5, 126.7, 126.8, 127.4, 128.1, 128.8, 128.9, 129.1, 131.2, 132.4, 133.1, 133.5, 138.9, 141.9, 156.9, 159.2, 160.7; MS (MMES + APCI) m/z: calcd for C26H16Cl2N4O 470.07 found: 470.6; elemental analysis calcd (%) for C26H16Cl2N4O: C, 66.25; H, 3.42; Cl, 15.04; N, 11.89; O, 3.39 found: C, 66.41; H, 3.42; N, 11.91.
6-Amino-1-benzyl-4-(naphthalen-1-yl)-2-oxo-1,2-dihydropyridine-3,5-dicarbonitrile (5i). Off-white solid (0.742 g, 66%); mp 262–264 °C; 1H-NMR (400 MHz, DMSO-d6) δ 5.32 (d, J = 16.8 Hz, 1H), 5.44 (d, J = 16.4 Hz, 1H), 7.33 (t, J = 8.0 Hz, 2H), 7.41 (t, J = 7.2 Hz, 2H), 7.60–7.69 (m, 4H), 7.84 (d, J = 9.2 Hz, 1H), 8.05–8.12 (m, 2H), 8.50 (s, 2H); 13C{1H}-APT (100 MHz, DMSO-d6) δ 44.8, 77.1, 89.1, 115.4, 116.1, 124.6, 125.4, 125.9, 126.5, 126.6, 127.3, 127.4, 128.5, 128.5, 129.3, 129.9, 132.5, 133.0, 134.4, 156.5, 159.4, 160.2; MS (MMES + APCI) m/z: calcd for C24H16N4O 376.13 found: 375.80; elemental analysis calcd (%) for C24H16N4O: C, 76.58; H, 4.28; N, 14.88; O, 4.25 found: C, 76.59; H, 4.28; N, 14.88.
6-Amino-1-(2-chlorobenzyl)-4-(naphthalen-1-yl)-2-oxo-1,2-dihydropyridine-3,5-dicarbonitrile (5j). Off-white solid (0.784 g, 64%); mp 230–232 °C; 1H-NMR (400 MHz, DMSO-d6) δ 5.26 (d, J = 17.6 Hz, 1H), 5.33 (d, J = 17.6 Hz, 1H), 7.10 (d, J = 5.2 Hz, 1H), 7.35–7.40 (m, 2H), 7.55–7.58 (m, 1H), 7.61–7.71 (m, 4H), 7.91 (t, J = 5.6 Hz, 1H), 8.06–8.13 (m, 2H), 8.61 (s, 2H); 13C{1H}-APT (100 MHz, DMSO-d6) δ 44.5, 77.3, 88.9, 115.5, 116.1, 124.8, 125.4, 125.5, 125.8, 126.6, 127.2, 127.4, 128.5, 128.8, 129.3, 129.4, 129.9, 131.8, 132.1, 132.6, 133.0, 156.9, 159.2, 160.4; MS (MMES + APCI) m/z: calcd for C24H15ClN4O 410.09 found: 409.80; elemental analysis calcd (%) for C24H15ClN4O: C, 70.16; H, 3.68; Cl, 8.63; N, 13.64; O, 3.89 found: C, 69.99; H, 3.68; N, 13.71.
6-Amino-1-(4-chlorobenzyl)-4-(naphthalen-1-yl)-2-oxo-1,2-dihydropyridine-3,5-dicarbonitrile (5k). White solid (0.788 g, 64%); mp 276–278 °C; 1H-NMR (400 MHz, DMSO-d6) δ 5.29 (d, J = 16.8 Hz, 1H), 5.39 (d, J = 16.4 Hz, 1H), 7.38 (d, J = 8.0 Hz, 2H), 7.47 (d, J = 8.4 Hz, 2H), 7.58–7.64 (m, 3H), 7.67 (t, J = 8.0 Hz, 1H), 7.84 (d, J = 7.2 Hz, 1H), 8.05–8.11 (m, 2H), 8.50 (s, 2H); 13C{1H}-APT (100 MHz, DMSO-d6) δ 44.4, 77.3, 89.0, 115.4, 116.1, 124.7, 125.4, 125.8, 126.6, 127.2, 128.3, 128.4, 128.7, 129.2, 129.3, 129.9, 132.0, 132.5, 133.0, 133.6, 156.5, 159.4, 160.2; MS (MMES + APCI) m/z: calcd for C24H15ClN4O 410.09 found: 409.70; elemental analysis calcd (%) for C24H15ClN4O: C, 70.16; H, 3.68; Cl, 8.63; N, 13.64; O, 3.89 found: C, 69.99; H, 3.68; N, 13.65.
6-Amino-1-(2,4-dichlorobenzyl)-4-(naphthalen-1-yl)-2-oxo-1,2-dihydropyridine-3,5-dicarbonitrile (5l). White solid (0.822 g, 62%); mp 294–296 °C; 1H-NMR (400 MHz, DMSO-d6) δ 5.21 (d, J = 18 Hz, 1H), 5.27 (d, J = 17.6 Hz, 1H), 7.17 (d, J = 8.0 Hz, 1H), 7.44 (dd, J = 2.0 Hz, J = 8.4 Hz 1H), 7.59–7.66 (m, 3H), 7.69 (t, J = 8.0 Hz, 1H), 7.76 (d, J = 2 Hz, 1H), 7.92 (t, J = 5.2 Hz, 1H), 8.06–8.08 (m, 1H), 8.11 (d, J = 8.4 Hz, 1H), 8.62 (s, 2H); 13C{1H}-APT (100 MHz, DMSO-d6) δ 44.4, 77.4, 89.0, 115.4, 116.0, 124.8, 125.4, 125.8, 126.6, 127.1, 127.2, 127.4, 128.5, 128.9, 129.3, 130.0, 131.2, 132.5, 132.5, 133.0, 133.1, 156.9, 159.2, 160.5; MS (MMES + APCI) m/z: calcd for C24H14Cl2N4O 444.05 found: 443.65; elemental analysis calcd (%) for C24H14Cl2N4O: C, 64.73; H, 3.17; Cl, 15.92; N, 12.58; O, 3.59 found: C, 64.98; H, 3.17; N, 12.59.
General scheme and procedure for the synthesis of 6a–6d
A mixture of 3 mmol 9-anthraldehyde, 3 mmol malononitrile and 0.3 mmol (10 mol%) piperidinium acetate in 10 ml ethanol was stirred at room temperature for 5 minutes to produce arylidene malononitrile. Then, 3 mmol of N-substituted 2-cyanoacetamide was added to the reaction mixture and refluxed for 9 hours. Upon completion, as monitored by TLC, the reaction mixture was cooled and poured into water. The resulting solid was filtered off, washed with water, dried and recrystallized from dichloromethane to give the pure products 6a–6d.
3-(Anthracen-9-yl)-N-benzyl-2-cyanoacrylamide (6a). Yellow solid (0.958 g, 88%); mp 220–222 °C; 1H-NMR (400 MHz, DMSO-d6) δ 4.55 (d, J = 5.6 Hz, 2H), 7.31 (t, J = 7.2 Hz, 1H), 7.39–7.47 (m, 4H), 7.60–7.67 (m, 4H), 8.06 (d, J = 8.4 Hz, 2H), 8.20 (d, J = 7.6 Hz, 2H), 8.80 (s, 1H) 9.10 (s, 1H), 9.46 (t, J = 5.6 Hz, 1H); 13C{1H}-APT (100 MHz, DMSO-d6) δ 43.2, 115.0, 117.1, 124.9, 125.8, 126.4, 127.06, 127.12, 127.6, 128.2, 128.4, 128.9, 129.3, 130.6, 138.7, 150.1, 160.4; MS (MMES + APCI) m/z: calcd for C25H18N2O 362.14 found: 362.60; elemental analysis calcd (%) for C25H18N2O: C, 78.86; H, 4.25; N, 13.14; O, 3.75 found: C, 78.88; H, 4.33; N, 13.13.
3-(Anthracen-9-yl)-N-(2-chlorobenzyl)-2-cyanoacrylamide (6b). Orange solid (1.037 g, 87%); mp 196–198 °C; 1H-NMR (400 MHz, DMSO-d6) δ 4.63 (d, J = 5.6 Hz, 2H), 7.35–7.45 (m, 2H), 7.52 (d, J = 7.6 Hz, 1H), 7.91–7.69 (m, 5H), 8.09 (d, J = 8.4 Hz, 2H), 8.21 (d, J = 8.0 Hz, 2H), 8.80 (s, 1H), 9.14 (s, 1H), 9.47 (t, J = 5.2 Hz, 1H); 13C{1H}-APT (100 MHz, DMSO-d6) δ 41.1, 115.0, 116.9, 125.0, 125.9, 126.3, 127.2, 127.3, 128.2, 128.89, 128.94, 129.2, 129.4, 130.6, 132.1, 135.5, 150.3, 160.7; MS (MMES + APCI) m/z: calcd for C25H17ClN2O 396.10 found: 396.60; elemental analysis calcd (%) for C25H17ClN2O: C, 72.96; H, 3.72; Cl, 7.69; N, 12.16; O, 3.47 found: C, 73.02; H, 3.73; N, 12.18.
3-(Anthracen-9-yl)-N-(4-chlorobenzyl)-2-cyanoacrylamide (6c). Yellow solid (1.024 g, 86%); mp 224–226 °C; 1H-NMR (400 MHz, DMSO-d6) δ 4.54 (d, J = 6 Hz, 2H), 7.45–7.50 (m, 4H), 7.60–7.67 (m, 4H), 8.06 (d, J = 8.4 Hz, 2H), 8.20 (d, J = 8.4 Hz, 2H), 8.80 (s, 1H), 9.11 (s, 1H), 9.47 (t, J = 5.6 Hz, 1H); 13C{1H}-APT (100 MHz, DMSO-d6) δ 42.6, 115.0, 116.9, 124.9, 125.8, 126.3, 127.1, 128.2, 128.3, 128.9, 129.4, 129.5, 130.6, 131.6, 137.8, 150.3, 160.5; MS (MMES + APCI) m/z: calcd for C25H17ClN2O 396.10 found: 396.60; elemental analysis calcd (%) for C25H17ClN2O: C, 72.96; H, 3.72; Cl, 7.69; N, 12.16; O, 3.47 found: C, 72.99; H, 3.72; N, 12.15.
3-(Anthracen-9-yl)-2-cyano-N-(2,4-dichlorobenzyl)acrylamide (6d). Yellow solid (1.126 g, 87%); mp 242–244 °C; 1H-NMR (400 MHz, DMSO-d6) δ 4.89 (d, J = 5.6 Hz, 2H), 7.52 (dd, J = 2 Hz, J = 8.4 Hz, 1H), 7.62–7.70 (m, 6H), 8.08 (d, J = 8.4 Hz, 2H), 8.21 (d, J = 8.0 Hz, 2H), 8.81 (s, 1H), 9.13 (s, 1H), 9.46 (t, J = 5.6 Hz, 1H); 13C{1H}-APT (100 MHz, DMSO-d6) δ 40.7, 115.0, 116.8, 125.0, 125.9, 126.3, 127.2, 127.5, 128.2, 128.7, 129.0, 129.2, 130.6, 130.7, 132.5, 134.8, 150.4, 160.7; MS (MMES + APCI) m/z: calcd for C25H16Cl2N2O 430.06 found: 430.50; elemental analysis calcd (%) for C25H16Cl2N2O: C, 67.89; H, 3.26; Cl, 14.31; N, 11.31; O, 3.23 found: C, 67.93; H, 3.27; N, 11.31.
Conflicts of interest
There is no conflict of interest to declare.
Acknowledgements
We are thankful to SERB, New Delhi, India for providing financial assistance from the major research project (file no. EEQ/2016/000376, dated 07/02/2017). We also thank UGC, New Delhi for UGC-CPEPA Phase-II program sponsored under award letter no. F. No.1–14/2002-2016(NS/PE) dated 28th April 2016, the assistance in general and NMR facility in particular. We are also thankful to analytical scientist, Mr Milan Ramavat, from Aether Industries Ltd. Surat for analytical testing.
References
- T. W. G. Solomons, C. B. Fryhle and S. A. Snyder, Organic chemistry, 2016 Search PubMed
. - M. Dagonneau, V. Ivanov, E. Rozantsev, V. Sholle and E. S. Kagan, J. Macromol. Sci., Part C: Polym. Rev., 1982, 22, 169–202 CrossRef
. - L. Errede, J. McBrady and H. Oien, J. Org. Chem., 1977, 42, 656–658 CrossRef CAS
. - H. C. Brown, J. Chem. Soc., 1956, 1248–1268, 10.1039/JR9560001248
. - M. Sharma, D. Rajani and H. Patel, R. Soc. Open Sci., 2017, 4, 170006 CrossRef CAS
. - H. M. Patel, Curr. Bioact. Compd., 2018, 14, 278–288 CrossRef CAS
. - M. G. Sharma, R. M. Vala, D. M. Patel, I. Lagunes, M. X. Fernandes, J. M. Padrón, V. Ramkumar, R. L. Gardas and H. M. Patel, ChemistrySelect, 2018, 3, 12163–12168 CrossRef CAS
. - D. M. Patel, R. M. Vala, M. G. Sharma, D. P. Rajani and H. M. Patel, ChemistrySelect, 2019, 4, 1031–1041 CrossRef CAS
. - Y. Hayashi, Chem. Sci., 2016, 7, 866–880 RSC
. - I. Dyachenko, V. Dyachenko and E. Rusanov, Russ. J. Org. Chem., 2007, 43, 83–89 CrossRef CAS
. - T. T. Khatri and V. H. Shah, J. Korean Chem. Soc., 2014, 58, 366–376 CrossRef CAS
. - A. A. Fadda, R. Rabie, H. A. Etman and A.-A. S. Fouda, Res. Chem. Intermed., 2015, 41, 7883–7897 CrossRef CAS
. - R. Mekheimer, M. Al-Sheikh, H. Medrasi and N. Alsofyani, Molecules, 2018, 23, 619 CrossRef
. - A. Baitha, M. Upadhyay, A. Gopinathan, K. Krishnan and V. V. Dabholkar, Synth. Commun., 2019, 49, 844–851 CrossRef CAS
. - B. Lai, R. Bai and Y. Gu, ACS Sustainable Chem. Eng., 2018, 6, 17076–17086 CrossRef CAS
. - L. P. Hinner, J. L. Wissner, A. Beurer, B. A. Nebel and B. Hauer, Green Chem., 2016, 18, 6099–6107 RSC
. - A. El-Harairy, Yiliqi, M. Yue, W. Fan, F. Popowycz, Y. Queneau, M. Li and Y. Gu, ChemCatChem, 2019 DOI:10.1002/cctc.201900784
. - D. M. Patel, M. G. Sharma, R. M. Vala, I. Lagunes, A. Puerta, J. M. Padrón, D. P. Rajani and H. M. Patel, Bioorg. Chem., 2019, 86, 137–150 CrossRef CAS
. - H. Patel, D. Rajani, M. Sharma and H. Bhatt, Lett. Drug Des. Discovery, 2019, 16, 119–126 CrossRef CAS
. - P. Subramaniam, C. Ramasubbu and S. Athiramu, Green Chem., 2017, 19, 2541–2545 RSC
. - S. G. Levine, K. D. Barhoriak and H. S. Cho, J. Chem. Educ., 1988, 65, 79 CrossRef CAS
. - K. M. Al-Zaydi, Ultrason. Sonochem., 2009, 16, 805–809 CrossRef CAS
.
Footnote |
† Electronic supplementary information (ESI) available: Copies of 1H NMR, 13C NMR and mass spectra of all the synthesized compounds along with crystallographic data of compound 5a and 5i are available. CCDC 1922233 and 1922235. For ESI and crystallographic data in CIF or other electronic format see DOI: 10.1039/c9ra05975j |
|
This journal is © The Royal Society of Chemistry 2019 |
Click here to see how this site uses Cookies. View our privacy policy here.