DOI:
10.1039/C9RA05338G
(Paper)
RSC Adv., 2019,
9, 28987-28995
Antibacterial activity of Litsea cubeba essential oil and its mechanism against Botrytis cinerea
Received
12th July 2019
, Accepted 31st August 2019
First published on 16th September 2019
Abstract
Litsea cubeba essential oil (LCEO) extracted from the fruit of the Litsea tree is a broad-spectrum bacteriostatic agent that has been used to treat ailments for thousands of years in China. The objective of our study was to assess the inhibitory effect of LCEO on Botrytis cinerea, a fungus that causes the putrification of fruits and vegetables. After being treated with 1.0% LCEO, the electrical conductivity of the fungal cells increased, and the contents of soluble reducing sugars and proteins slowly increased over treatment time. After being treated for 48 h with 1.0% LCEO, scanning electron microscopy (SEM) and transmission electron microscopy (TEM) images clearly showed damage to hyphae cells when compared with the normal growth of the control groups. Additional studies showed that the ergosterol content in the cell membrane significantly decreased with an increase in the LCEO concentration, and the electrophoretic bands of the proteins assayed using the sodium dodecyl sulfate-polyacrylamide gel electrophoresis method significantly changed at different LCEO concentrations. LCEO damaged the cell membrane and changed the cell membrane permeability, leading to the changes in some components in the cytoplasm, such as soluble reducing sugars, proteins, and ergosterol. In general, the antimicrobial activity of LCEO is attributable to a unique pathway and involves a series of events both on the surface and within the cytoplasm of the fungal cell.
Introduction
Botrytis cinerea1 is a widely hosted fungus that infects a variety of fruits and vegetables. In a humid environment, the surface layers of the fruits and vegetables are prone to hosting a large number of mold layers (conidiophores and conidia) called gray mold, which is widely distributed in the air. It can infect field crops and cause huge economic losses during their postharvest stages.2,3 Most of the postharvest diseases of fruits and vegetables are controlled by chemical fungicides.4,5. However, these fungicides remain on the fruits and vegetables and can affect human health and the environment. In addition, the large-scale use of chemically fungicides causes resistance to pathogenic microorganisms. Therefore, it is prudent that the use of these fungicides be kept to a minimum or halted. At the same time, it is critical that a natural, safe, and nontoxic fungicide be developed. Studies have reported that essential oils (EOs) can inhibit potential fungal infections in fruits and vegetables, thus effectively extending the shelf life of these fruits and vegetables6–8 and their safety and nontoxic effects meet the requirements for green antibacterial agents.9,10. LCEO is extracted from the fruit of the Litsea tree, an aromatic oil plant from Southern China. The main components of the oil are terpenoids and their oxygen-containing derivatives. Studies have shown that LCEO is widely used in antibacterial and food preservation due to its broad-spectrum bactericidal, biodegradable, safe and non-toxic side effects.11–13.
The antibacterial mechanism of EOs are usually not through a single action but encompass multiple actions.14. The first of these actions is to destroy the pathological structure of pathogenic cells or mycelia, such as the cell membrane, cytoplasm, and other basic structures, and to dissolve mycelium, which results in irreversible damage to the cells.15,16. The second is to affect the structure and function of the cells, energy production, and synthesis of cellular metabolites and their function and to inhibit respiration.17 The third is to inhibit the production and germination of bacteria and conidia, thereby blocking the generation of pathogens and causing aging and death of the cells.18 The hydrophobic character of EOs enables them to distribute into the membrane of the cell and the lipids of the mitochondria, which makes the cell structure unstable, destroys its integrity, and increases the permeability of the cell membrane.19,20 For example, thyme oil can inhibit the synthesis of proteins and lead to significant changes the proteins in outer membrane of bacteria, thereby affect the ability of the bacteria to attack and exert their antibacterial effects.21 Zhang et al.22 have shown that cinnamon EOs can destroy the integrity of the cell wall of Escherichia coli and inhibit the synthesis of its components, thereby playing a bactericidal role. Tian et al.23 have shown that clove and pineapple EOs can inhibit the synthesis of microbial ergosterol, thus undermining the integrity of the cell. Cox et al.24 have shown that the mechanism by which tea tree oil causes the ultimate death of microorganisms is to destroy the permeability of the cell membrane and accompany by the loss in the chemical permeability. Vasconcelos et al.25 have shown that the bacteriostatic mechanism of cinnamon EOs and its compounds inhibit the bacteria's membranes and membrane porins, movement, and biofilm formation by destroying cells. Ulanowska et al.26 have used radioisotope labeling to show that genistein inhibits, to some extent, the synthesis of certain pathogenic proteins. However, there are few studies on how LCEO inhibits the growth of B. cinerea and that have elucidated its antibacterial mechanisms.
Thus, the aim of this study was to investigate the mechanisms by which LCEO inhibits the growth of B. cinerea at the cellular level. A better understanding of LCEO's antibacterial actions and the possible mechanisms by which to protect fruits and vegetables will be helpful for its use in developing new bacteriostatic agents.
Experimental
Microorganisms and chemicals
Botrytis cinerea were obtained from China Microbial Culture Collection and maintained in slants of nutrient agar at 4 °C. Ethyl acetate, glutaric acid, osmium acid, and monometallic sodium orthophosphate were domestic analytical pure. Litsea cubeba fruits produced in Yongshun County, Hunan Province, China, and LCEO were extracted by using steam distillation. Gas chromatography-mass spectrometry (Thermo DSQII GC-MS, USA) was used to determine the LCEO components and content. These ingredients mainly include geranial (35.24%), neral (33.63%), limonene (9.05%), citronellal (3.14%), cineole (1.84%), linalool (1.80%), β-myrcene (1.69%), 1-caryophyllene (1.64%), α-pinene (1.22%), geraniol (1.10%), β-pinene (0.83%), nerol (0.81%), α-terpineol (0.61%), sabinene (0.48%), camphene (0.41%), caryophyllene oxide (0.29%), methyl-2-isopropenyl-4-hexenal (0.27%), β-elemene (0.27%), borneol (0.07%).
Collection of hyphae
Botrytis cinerea was cultured for 1 d on potato dextrose agar medium (PDA) and then a cake of hyphae was extracted using a puncher. The extracted cake was inoculated into PDA medium at five cakes per sterile conical flask. The bottles were shaken at 150 rpm at 25 °C for 2 d. Under aseptic conditions, the hyphae were stripped from the sterile conical flask, reinoculated into the newly configured PDA medium, and incubated at 25 °C for 2 d while shaking at 150 rpm.
Effect of LCEO on mycelium growth
The effect of LCEO on mycelium growth was evaluated using the agar dilution method.27 Different concentrations of LCEO and Tween 80 were added to the sterile PDA medium and evenly mixed so that the final concentration of LCEO in each PDA medium was 0% (the control group), 0.5%, and 1.0%, respectively. After cooling for 15 min, the medium solidified. Then, 0.1 mL fungal suspension was injected into the PDA medium, which was uniformly coated using an applicator. The medium was sealed with a sealing membrane and placed in a mold incubator at 28 °C for cultivation. The diameter of the fungal colony was measured every 24 h and recorded until it no longer increased. The procedures described were repeated three times for the different concentrations.
Effect of LCEO on leakage in Botrytis cinerea hyphae
Change in electrical conductivity after LCEO treatment. The electrical conductivity of the mycelium was determined according to the method of Kong et al.28 Briefly, the fungus strain was inoculated on PDA medium and incubated 4 d, after which the fungus cake was removed from along the edge of the colony using a punch of a diameter of 6 mm, transferred into PDA medium, and cultured for 2 d with continuous shaking at 150 rpm. After filtering the culture solution by suction, the hyphae were washed with physiological saline. Two grams of hyphae were placed into a 50 mL beaker and washed three times with sterile deionized water. The mycelium treated with 0.5% and 1.0% LCEO was measured for electrical conductivity at 0, 2, 4, 6, 8 and 10 h, and each experiment was repeated three times.
Changes in soluble protein content after LCEO treatment. The integrity of the cells was examined by determining the amount of proteins released into the supernatant. The concentrations of proteins in the supernatants were determined using the Bradford method.29 After the mycelia were treated with 1.0% LCEO, the protein content was measured every 2 h. The cells were then separated by centrifugation at 10
000 rpm for 5 min at 4 °C. To determine the concentration of the proteins released from the cytoplasm, the absorbance of the supernatant was measured at 595 nm using the TU-19 UV/VIS spectrophotometer (Beijing Purkinje General Instrument Co, Ltd., Beijing, China).
Changes in soluble reducing sugars after LCEO treatment. The amount of soluble reducing sugars was determined using the method of Stephen Dygert with modifications.30 Briefly, seven different tubes were used to prepare a series of different concentrations of glucose solution. The glucose standard concentrations were 0, 10, 20, 30, 40, and 50 μg mL−1. Then, 1.0, 0.9, 0.8, 0.7, 0.6, and 0.5 mL distilled water were added to the tubes, respectively, after which 4 mL anthrone reagent was added to each tube and rapidly cooled in a cold water bath. The tubes were then immersed into a boiling water bath, boiled for another 10 min, and brought to room temperature using a cold water bath. The wavelength was measured at 620 nm using the TU-19 UV/VIS spectrophotometer (Beijing Purkinje General Instrument Co, Ltd.). The first tube was the blank to quickly measure the absorbance of the remaining tubes. The glucose content was used as the abscissa and the absorbance value as the ordinate for creating the standard curve.Two grams of hyphae were placed into a 50 mL beaker, and 20 mL distilled water was added to create the control group. LCEO and Tween 80 were added to the experimental group and diluted to 20 mL with distilled water. These samples were conducted for 2 d with continuous shaking at 150 rpm.
Effect of LCEO on the morphology of B. Cinerea
Morphological changes in B. cinerea were observed using scanning electron microscopy (SEM) with the JSM-IT 100 according to the method of Bajpai, et al.31 with modifications. The mycelia were treated with 1.0% LCEO for 48 h, washed three times with 50 mM phosphate buffer solution (PBS, pH 7.3), and centrifuged at 4000 rpm. The suspension was centrifuged at 1500 rpm for 10 min and washed twice with 0.1 M PBS (pH 7.4). The cells were then fixed in 2.5% glutaraldehyde solution for 4 h. The sample was dehydrated in a graded ethanol series (30%, 50%, 80%, 90%, and 100%) and then the ethanol was replaced with 100% t-butanol. Finally, all samples were sputter coated with gold for 2 min in an ion coater and then microscopically examined using SEM.
Pretreatments of the indicators for transmission electron microscopy (TEM) using the Tecnai G2 Spirit Bio-Twin transmission electron microscope were the same as that for SEM. After postfixing using osmic acid, the cells were dehydrated using alcohol and permeated with white resin. The cells were then embedded after roasting at 55 °C for 48 h and 70 nm sections were prepared on copper grids and stained with lead citrate and uranyl acetate. TEM was used to observe the ultrastructure of the cells.
Determination of ergosterol content in cell membranes
The ergosterol content of B. cinerea was measured as previously described with slight modifications.32 An accurately weighed 0.0050 g ergosterol standard was dissolved in 95% ethanol to 50 mL, and 0.1 mg mL−1 ergosterol standard mother solution was obtained. We transferred 1, 2, 3, 4, and 5 mL above solution and diluted each to 10 mL with ethanol. Concentrations of 0.01, 0.02, 0.03, 0.04, and 0.05 mg mL−1 were obtained, respectively, using the above measured solutions and dilutions. Absorbance A was measured at a wavelength of 282 nm using the TU-19 UV/VIS spectrophotometer (Beijing Purkinje General Instrument Co, Ltd.). A standard curve of mass concentration C to absorbance A was obtained.
We weighed out 0.5 g hyphae from the treated and control and placed each sample into a sterilized 50 mL Erlenmeyer flask. To these, we added 10 mL 20% sodium hydroxide solution and 5 mL 95% ethanol, and saponified the solution for 1.5 h in a water bath at 90 °C, after which we mixed 5 mL 95% ethanol for 1 h for a secondary saponification. After cooling to room temperature, 10 mL petroleum ether was added and the solution shaken for 20 min, let stand for 30 min, then extracted twice, and the upper liquid was removed and washed with distilled water. The supernatant (1 mL) was removed and diluted to 10 mL with 95% ethanol. The absorbance was then determined using the TU-19 UV/VIS spectrophotometer (Beijing Purkinje General Instrument Co, Ltd.) at 282 nm.
SDS-PAGE electrophoresis of the B. cinerea proteins
SDS-PAGE is the most important and widely used technology to analyze proteins. The experimental method was conducted according to Huang et al.33 with a slight modification. Samples of five bacterial cells were centrifuged at 10
000 rpm for 5 min, and the supernatants were discarded. The cell pellets were rinsed and resuspended in 0.01 mol L−1 PBS. Ultrasonic wave (200 W) was applied for 10 min to disrupt the fungal cell suspension, which was then centrifuged for 3 min at 10
000 rpm. The supernatant was then collected and the protein concentration determined. A buffer was added into 80 mL samples of the supernatant at a protein concentration of approximately 3 mg mL−1. The mixture was boiled for 5 min and cooled on ice, after which 25 mL supernatant from each sample was collected for SDS-PAGE analysis. After electrophoresis, the gel was stained with Coomassie brilliant blue R-250 and then decolorized to obtain separated protein bands.
Statistical analyses
Each experiment was conducted in triplicate, and all values were reported as the mean ± standard deviation (SD) using Microsoft excel. The data were statistically analyzed using one-way analysis of variance. P < 0.05 was considered statistically significant.
Results and discussion
Effect of LCEO on mycelium growth
The effects of different LCEO concentrations on the growth of B. cinerea mycelia are shown in Fig. 1 LCEO inhibited the growth of B. cinerea in a dose-dependent manner in concentrations ranging from 0 to 1.0% (v/v). Mycelia without essential oil treatment (CK) had a diameter of 7.3 cm after 4 d, which increased slowly with an increase in treatment time. However, after treating with 0.5% LCEO for 2 and 4 d, the diameter of the hyphae was only 3.2 cm and 5 cm, respectively. After 6 d, the mycelia rapidly increased. This indicated that 0.5% LCEO had a strongly inhibiting effect on the mycelia in the early stages of treatment. We observed that mycelia growth was completely controlled with 1.0% LCEO. On the whole, LCEO showed a pronounced antifungal activity against the tested B. cinerea in a dose-dependent manner, which are basically identical with Li et al. reports.34
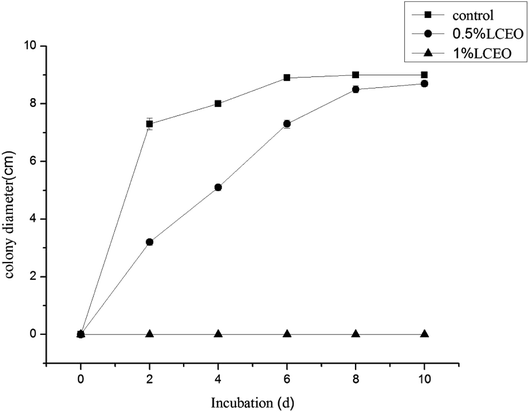 |
| Fig. 1 Inhibition effect of mycelium by different LCEO concentrations. | |
Effect of LCEO on leakage of B. cinerea components
Changes in conductivity in B. cinerea treated with LCEO. A change in conductivity indirectly reflects a change in cell membrane permeability. The greater the value change, the greater the leakage of intracellular electrolytes and indicates the greater the degree of cell damage.31,35 As shown in Fig. 2, during the first 2 h of the test, the conductivity of the control group increased, which might have been a result of normal lysis and death of the bacteria. However, there were few changes in conductivity in the control over longer periods of time (P < 0.05). In contrast, the conductivity of the suspension increased immediately after being treated with 1.0% LCEO and continued to rapidly increase as treatment time increased (P < 0.05). The conductivity after treatment was up to 70.2 ms cm−1 after 2 h and was 2.08 times higher than that of the control, which indicated that LCEO destroyed the bacteria cells and caused electrolyte exosmosis, such as potassium, calcium, and sodium.36,37
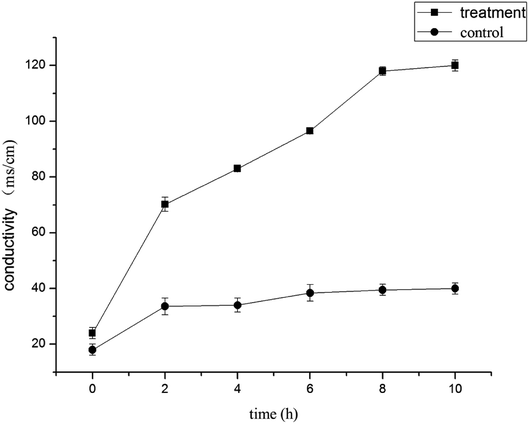 |
| Fig. 2 Electrical conductivity effects of LCEO on mycelium. | |
Changes in the soluble protein content of mycelium treated with LCEO. The results of protein leakage are shown in Fig. 3. Protein leakage in the control was relatively low and had changed little over time (P > 0.05). But in the treatment group with 1.0% LCEO, it changed from 0.225 to 3.663 mg g−1 with incubation for 2 h, and further increased with a longer incubation time. After being treated with 1.0% LCEO for 6 h, leakage of soluble proteins was as high as 5.237 mg g−1 and tended to be stable, which indicated that LCEO caused protein leakage from the cells by damaging the cell membrane.38 Similar findings have also been reported in the study of Meng et al.39
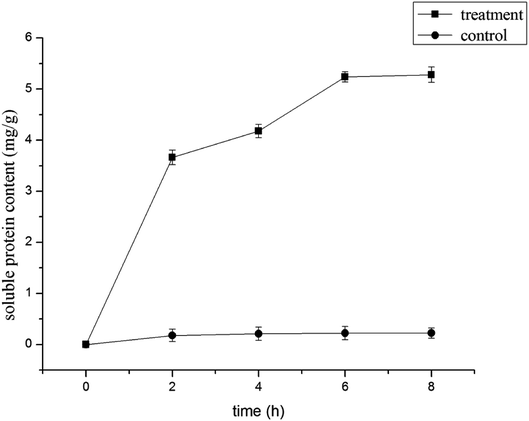 |
| Fig. 3 Effect of LCEO on soluble protein content of Botrytis cinerea. | |
Changes in the content of reducing sugars in mycelium treated with LCEO. The changes in the content of reducing sugars after treatment with 1.0% LCEO are shown in Fig. 4. At first, we could not detect any changes in reducing sugar content in the control cells. After treatment with 1.0% LCEO for 1 h, the amount of reducing sugars increased to 1.42 mg g−1. As the treatment time of LCEO increases, the reducing sugar content has not changed much. In the control, the reducing sugar content increased to 0.125 mg g−1. This might be the result of intracellular osmotic pressure, which leads to the exchange of intracellular and extracellular substances. As the treatment time increased, the amount of reducing sugars leaked from the cell slightly higher than the control group (P < 0.05). The results showed that LCEO caused rapid losses of proteins and reducing sugars from the cells, which indicated irreversible damage to the cytoplasmic membranes.31
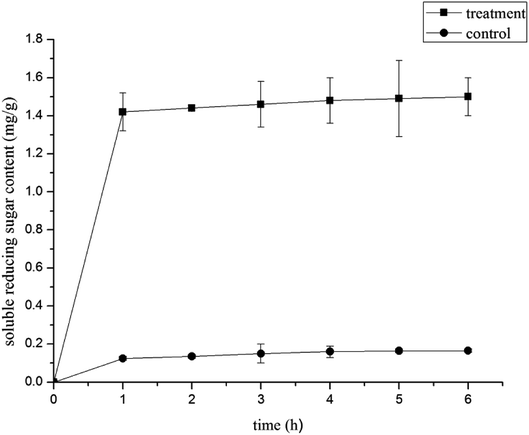 |
| Fig. 4 Effect of LCEO treatment on soluble reducing sugar content of Botrytis cinerea. | |
Effects on the morphology and ultrastructure of hyphae
The suppression of hyphae growth after 1.0% LCEO treatment was generally associated with alterations in their morphology and ultrastructure.40–42 Morphological changes in B. cinerea were observed using SEM. The morphology of the control samples without LCEO (Fig. 5a and b) were normal with uniform and linearly shaped hyphae of a constant diameter and a smooth surface. After treatment with the 1.0% LCEO for 48 h, fungal growth and spore germination were suppressed. The treated hyphal structure had undergone several morphological changes, such as collapsed and flattened and empty hyphae or shriveled hyphae with moniliform malformations (Fig. 5c and d). However, the results in the previous study indicate that 1.0% LCEO has an inhibitory effect on B. cinerea, which is consistent with the observation under scanning electron microscopy.
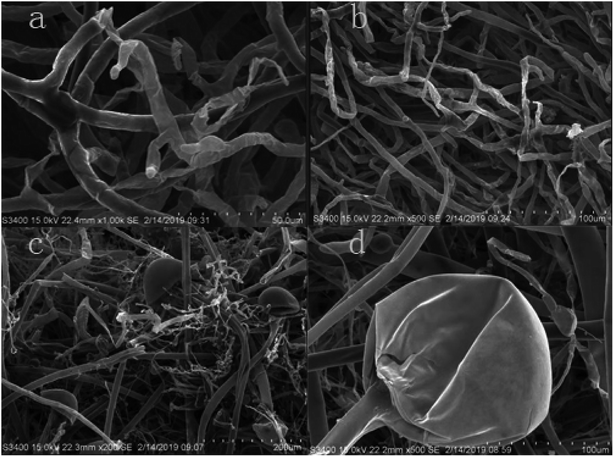 |
| Fig. 5 Effect of LCEO on mycelia under SEM. | |
TEM sections of the control showed the typical fungal ultrastructure with normal cell wall thickness, a regular and intact plasma membrane, cell morphology rules, and intact organelles in the mycelium (Fig. 6a–c). After treatment with 1.0% LCEO for 48 h, the general cell ultrastructure was modified, and the integrity of the cell structure was clearly destroyed. Fig. 6f shows that the conspicuous alterations of the mycelia were ultrastructural, ruptured plasmalemma that was detached from the cell wall, and plasmolysis. Simultaneously, the intracellular constituents were seriously damaged. Fig. 6e shows that the integrity of the cell wall was destroyed, and the cell membrane ruptured, which resulted in an outflow of intracellular contents, leaving only a small amount of vague contents in the cell lumen. Only a small amount of inclusions were observed in some cells, whereas others were completely empty (Fig. 6d). After 1.0% LCEO treatment in general, cell permeability changed, which was the result of the damage to the plasma membrane at various intervals, leading to the loss of the normal shape of the mycelia and the formation of membrane-bound vesicles inside the cells. Our results are in agreement with those of the study by Yu et al.43
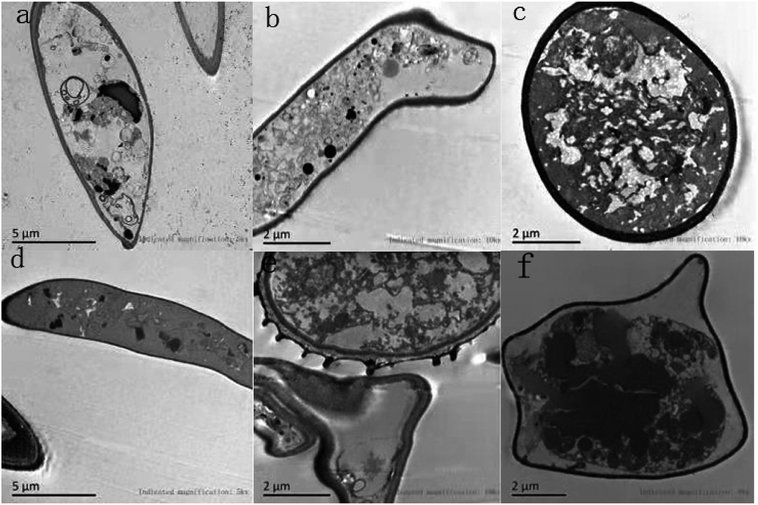 |
| Fig. 6 Effect of LCEO on mycelia under TEM. | |
Effects of LCEO on ergosterol content in the mycelium cell membrane
The plasma membrane plays a vital role in maintaining a homeostatic environment, exchanging materials, and transferring energy and information in the cells to keep them healthy and alive. Previous reports have demonstrated that one of main antibacterial targets of EOs is to destroy the fungus' plasma membrane.44 Ergosterol is the major sterol component in the cell membrane and is responsible for maintaining cell function and integrity.
The efficacies of LCEO on the content of ergosterol in the plasma membrane of B. cinerea are shown in Table 1. The ergosterol content was determined at 0%, 0.5%, 1%, 1.5%, and 2% LCEO with a value of 20.60 ± 1.29, 13.60 ± 0.54, 12.67 ± 0.53, 6.90 ± 1.02, and 3.90 ± 0.53 μg g−1, respectively. These results demonstrated that the ergosterol content in the plasma membrane was significantly inhibited by different concentrations of LCEO. Compared to that in the control group, the ergosterol content in the treated group significantly decreased (P < 0.05). At the same time, with the increase of LCEO concentration, the lower ergosterol content in treatment group. Those are similar to the studies of Khan et al.45 and Li et al.46
Table 1 Effects of different concentrations of LCEO on ergosterol content
LCEO concentration (v/v, %) |
0 |
0.5 |
1.0 |
1.5 |
2.0 |
Ergosterol content (μg g−1) |
20.60 ± 1.29 |
13.60 ± 0.54 |
12.67 ± 0.53 |
6.90 ± 1.02 |
3.90 ± 0.53 |
SDS-PAGE electrophoresis of proteins in B. cinerea
SDS-PAGE profiles of the proteins from B. cinerea treated with LCEO, which were different from that in the control, are shown in Fig. 7. The mold in the control groups had much clearer protein bands. However, after treatment with LCEO the protein bands became lighter, with some actually disappearing altogether. Specifically, there were eight major bands in the maker. By contrast, three bands at 170, 100, and 70 kDa were observed in the groups treated with 0.5%, 1.0%, and 1.5% LCEO, respectively, and the three bands became much fainter after being treated with the 2.0% LCEO. In addition, the band at approximately 170 kDa completely disappeared in all proteins that were subjected to SDS-PAGE. The protein bands at 70 kDa in the Botrytis cells grew much fainter as the concentration of LCEO increased. Moreover, those bands of less 70 kDa disappeared in all those treated with LCEO. This indicated that LCEO had a remarkable effect on fungal proteins, especially those with a molecular weight less 70 kDa. Similar results were also found in bacteria treated by certain antibacterial compounds, such as the EOs from Dendranthema indicum var. aromaticum and lactic acid.47
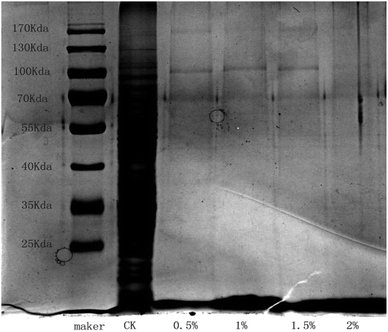 |
| Fig. 7 Effect of different LCEO concentrations on protein content of Botrytis cinerea. | |
In summary, LCEO decreased the content of cellular proteins by permeating and disturbing the cell membranes, as well as had an effect on the cellular proteins either by disrupting them or suppressing their synthesis.43,48
There are several reports on the antibacterial mechanisms of EOs on mycetes. This paper discussed the antibacterial mechanism by which LCEO affects the structure and function of fungal cells, including destroying the cell membrane and inhibiting the synthesis of sterols in the membrane. LCEO can effectively inhibit the growth of B. cinerea by destroying its cell membrane and changing membrane permeability, resulting in leakage of the cell's contents, such as proteins and reducing sugars. Because of the many chemical components of EOs, there is more than one antibacterial mechanism. Therefore, additional research, such as that to identify new chemical compounds in EOs, is needed to fully investigate the antibacterial mechanisms of the LCEO against B. cinerea.
Conclusion
The research of this paper preliminarily revealed the inhibition effect and mechanism of LCEO on B. cinerea. LCEO inhibits the growth of B. cinerea, and this inhibitory effect is increased with increasing LCEO concentrations. At a concentration of 1.0%, LCEO could completely inhibit the growth of B. cinerea. After the 1.0% LCEO treatment, the permeability of the cell membrane changed, which damaged its integrity, changed its conductivity, and altered the content levels of soluble reducing sugars, proteins, and ergosterol, leading to restrained hyphae growth, enlarged hyphae tops, and deformed or even burst hyphae, which finally caused the death of the fungus' somatic cells.
Conflicts of interest
The authors declare no conflict of interest.
Acknowledgements
This work was supported by National Key R&D Program of China (2017YFD0600704).
References
- D. Cantu, A. R. Vicente and L. C. Greve, et al., The intersection between cell wall disassembly, ripening, and fruit susceptibility to Botrytis cinerea, Proc. Natl. Acad. Sci. U. S. A., 2008, 105(3), 859–864 CrossRef CAS PubMed.
- B. Williamson, B. Tudzynski and P. Tudzynski, et al., Botrytis cinerea: the cause of grey mould disease, Mol. Plant Pathol., 2007, 8(5), 561–580 CrossRef CAS PubMed.
- M. Staats, B. P. Van and A. Schouten, et al., Positive selection in phytotoxic protein-encoding genes of Botrytis species, Fungal Genet. Biol., 2007, 44(1), 52–63 CrossRef CAS PubMed.
- X. M. Wang, X. F. Du and Y. Nong, et al., Growth-inhibition of 12 fungicides against Botrytis cinerea in tomato and their preventive effects in field, Pak. J. Bot., 2019, 51(6), 2291–2294 Search PubMed.
- X. J. Li, X. F. Xie and F. G. Xing, et al., Glucose oxidase as a control agent against the fungal pathogen Botrytis cinerea in postharvest strawberry, Food Control, 2019, 105, 277–284 CrossRef CAS.
- E. M. Mekawi, E. Y. Khafagi and F. A. Abdel-Rahman, Effect of pre-harvest application with some organic acids and plant oils on antioxidant properties and resistance to Botrytis cinerea in pepper fruits, Sci. Hortic., 2019, 254, 108736 CrossRef.
- D. Sivakumar, R. S. Wilson Wijeratnam and R. L. C. Wijesundera, et al., Control of post harvest diseases of rambutan using cinnamal, Food Chem., 2002, 21(9), 847–852 CAS.
- R. M. V. Bhaskara, P. L. Angers and A. Gossenlin, et al., Charaterization and use of essential oil from thymus vulgaris aganist Botrytis cinerea and Rhizopus stolonifer in strawberry fruit phytochemistry, Food Chem., 1998, 47(8), 1515–1520 Search PubMed.
- A. Kedia, B. Prakash and P. K. Mishra, et al., Antifungal and antiaflatoxigenic properties of Cuminum cyminum(L.) seed essential oil and its efficacy as a preservative in stored commodities, Int. J. Food Microbiol., 2014, 168–169, 1–7 CrossRef CAS PubMed.
- W. Zhang, J. F. Hu and J. Ju, et al., The inhibitory effect of plant essential oils on foodborne pathogenic bacteria in food, Crit. Rev. Food Sci. Nutr., 2018, 1–55 Search PubMed.
- W. W. Lv, Antibacterial, antifungal and cytotoxic isoquinoline alkaloids from Litsea cubeba, Molecules, 2012, 17(12), 12950–12960 Search PubMed.
- H. W. Wang and Y. Q. Liu, Chemical composition and antibacterial activity of essential oils from different parts of Litsea cubeba, Chem. Biodiversity, 2010, 7(1), 229–235 CrossRef CAS PubMed.
- W. Hu, C. Z. Li and J. M. Dai, et al., Antibacterial activity and mechanism of Litsea cubeba essential oil against methicillin-resistant Staphylococcus aureus (MRSA), Ind. Crops Prod., 2019, 130, 34–41 CrossRef CAS.
- T. T. Liu and T. S. Yang, Antimicrobial impact of the components of essential oil of Litsea cubeba from Taiwan and antimicrobial activity of the oil in food systems, Int. J. Food Microbiol., 2012, 156(1), 68–75 CrossRef CAS PubMed.
- X. F. Shao, H. F. Wang and F. Xu, et al., Effects and possible mechanisms of tea tree oil vapor treatment on the main disease in postharvest strawberry fruit, Postharvest Biol. Technol., 2013, 77(3), 94–101 CrossRef CAS.
- Y. Sun, S. Chen and C. Zhang, et al., Effects of sub-minimum inhibitory concentrations of lemon essential oil on the acid tolerance and biofilm formation of Streptococcus mutans, Arch. Oral Biol., 2018, 87, 235–241 CrossRef CAS PubMed.
- N. Khorshidian, M. Yousefi and E. Khanniri, et al., Potential application of essential oils as antimicrobial preservatives in cheese, Innovative Food Sci. Emerging Technol., 2017, 45, 62–112 CrossRef.
- K. A. Hammer, C. F. Carson and T. V. Riley, Melaleuca altemifolia (tea tree) oil inhibits germ tube formation by Candida albicans, Med. Mycol., 2000, 38(5), 354–361 CrossRef.
- S. A. Burt, Essential oils: their antibacterial properties and potential applications in foods-a review, Int. J. Food Microbiol., 2004, 4(3), 233–253 Search PubMed.
- K. P. Devi, S. A. Nisha and R. Sakthivel, et al., Eugenol (an essential oil of clove) acts as an antibacterial agent against Salmonella typhi by disrupting the cellular membrane, J. Ethnopharmacol., 2010, 130(1), 107–115 CrossRef CAS PubMed.
- A. Marchese, I. E. Orhan and M. Daglia, et al., Antibacterial and antifungal activities of thymol: a brief review of the literature, Food Chem., 2016, 210, 402–414 CrossRef CAS PubMed.
- Y. Zhang, X. Liu and Y. Wang, et al., Antibacterial activity and mechanism of cinnamon essential oil against Escherichia coli and Staphylococcus aure, Food Control, 2016, 59, 282–289 CrossRef CAS.
- J. Tian, X. Ban and Z. Hong, et al., The mechanism of antifungal action of essential oil from Dill (Anethum graveolensL.) on Aspergillus flavus, PLoS One, 2012, 7(1), 30147–30155 CrossRef PubMed.
- S. D. Cox, C. M. Mamm and J. L. Markham, Interactions between components of the essential oil of Melaleuca altermifolia, J. Appl. Microbiol., 2001, 91(3), 429–497 CrossRef PubMed.
- N. G. Vasconcelos, J. Croda and S. Simionatto, Antibacterial mechanisms of cinnamon and its constituents: a review, Microb. Pathog., 2018,(18), 305–667 Search PubMed.
- K. Ulanowska, A. Tkaczyk and G. Konopa, et al., Differential antibacterial activity of genistein arising from global inhibition of DNA, RNA and protein synthesis in some bacterial strains, Arch. Microbiol., 2006, 184(5), 271–278 CrossRef CAS PubMed.
- G. A. Helal, M. M. Sarhan and A. N. K. Abu Shahla, et al., Effects of Cymbopogon citratus L. essential oil on the growth, lipid content and morphogenesis of Aspergillus niger, ML2-strain, J. Basic Microbiol., 2006, 46(6), 456–469 CrossRef CAS PubMed.
- M. Kong, X. G. Chen and C. S. Liu, et al., Antibacterial mechanism of chitosan microspheres in a solid dispersing system against E. coli, Colloids Surf., B, 2008, 65(2), 197–202 CrossRef CAS PubMed.
- M. M. Bradford, A rapid and sensitive method for the quantitation of microgram quantities of protein utilizing the principle of protein-dye binding, Anal. Biochem., 1976, 72(1–2), 248–254 CrossRef CAS.
- S. Dygert, L. H. Li and D. Florida, et al., Determination of reducing sugar with improved precision, Anal. Biochem., 1965, 13(3), 367–374 CrossRef CAS.
- V. K. Bajpai, A. Sharma and K. H. Beak, Antibacterial mode of action of Cudrania tricuspidata fruit essential oil, affecting membrane permeability and surface characteristics of food-borne pathogens, Food Control, 2013, 32(2), 582–590 CrossRef CAS.
- B. A. Arthington-Skaggs, H. Jradi and T. Desai, et al., Quantitation of ergosterol content: novel method for determination of fluconazole susceptibility of Candida albicans, J. Clin. Microbiol., 1999, 37(10), 3332–3337 CAS.
- M. X. Huang, X. Q. Yu and Y. Ye, A study of fibrin zymography method for the assay of plasminogen activators, Adv. Mater. Res., 2012, 569, 789–794 CAS.
- Y. J. Li, W. J. Kong and M. H. Li, et al., Litsea cubeba essential oil as the potential natural fumigant: inhibition of Aspergillus flavus and AFB1 production in licorice, Ind. Crops Prod., 2016, 80, 186–193 CrossRef CAS.
- C. F. Carson, B. J. Mee and T. V. Riley, Mechanism of action of Melaleuca alternifolia (tea Tree) Oil on Staphylococcus aureus determined by time-kill, lysis, leakage, and salt tolerance assays and electron microscopy, Antimicrob. Agents Chemother., 2002, 46(6), 1914–1920 CrossRef CAS PubMed.
- N. Tao, L. Jia and H. Zhou, Anti-fungal activity of Citrus reticulata Blanco essential oil against Penicillium italicum and Penicillium digitatum, Food Chem., 2014, 153(24), 265–271 CrossRef CAS PubMed.
- W. R. Diao, L. L. Zhang and S. S. Feng, et al., Chemical composition, antibacterial activity, and mechanism of action of the essential oil from Amomum kravanh, J. Food Prot., 2014, 77(10), 1740–1746 CrossRef CAS PubMed.
- E. Z. Gomaa, Silver nanoparticles as an antimicrobial agent: a case study on Staphylococcus aureus and Escherichia coli as models for Gram-positive and Gram-negative bacteria, J. Gen. Appl. Microbiol., 2017, 63(1), 36–43 CrossRef CAS PubMed.
- X. X. Meng, D. W. Li and D. Zhou, et al., Chemical composition, antibacterial activity and related mechanism of the essential oil from the leaves of Juniperus rigida Sieb. et Zucc against Klebsiella pneumoniae, J. Ethnopharmacol., 2016, 80(16), 186–193 Search PubMed.
- X. Shao, S. Cheng and H. Wang, et al., The possible mechanism of antifungal action of tea tree oil on Botrytis cinerea, J. Appl. Microbiol., 2013, 114(6), 1642–1649 CrossRef CAS PubMed.
- C. Romagnoli, R. Bruni and E. Andreotti, et al., Chemical characterization and antifungal activity of essential oil of capitula from wild Indian Tagetes patula L., Protoplasma, 2005, 225(1–2), 57–65 CrossRef CAS.
- E. M. Soylu, S. Soylu and S. Kurt, Antimicrobial activities of the essential oils of various plants against tomato late blight disease agent phytophthora infestans, Mycopathologia, 2006, 161(2), 119–128 CrossRef CAS PubMed.
- L. Zhao, H. Y. Zhang and T. Y. Hao, et al., In vitro antibacterial activities and mechanism of sugar fatty acid esters against five food-related bacteria, Food Chem., 2015, 187, 370–377 CrossRef CAS PubMed.
- Y. Hu, J. Zhang and W. Kong, et al., Mechanisms of antifungal and anti-aflatoxigenic properties of essential oil derived from turmeric (Curcuma longa L.) on Aspergillus flavus, Food Chem., 2017, 220, 1–8 CrossRef CAS PubMed.
- A. Khan, A. Ahmad and F. Akhtar, et al., Ocimum sanctum essential oil and its active principles exert their antifungal activity by disrupting ergosterol biosynthesis and membrane integrity, Res. Microbiol., 2010, 16, 816–823 CrossRef PubMed.
- Y. H. Li, X. F. Shao and J. Y. Xu, et al., Effects and possible mechanism of tea tree oil against Botrytis cinerea and Penicillium expansum in vitro and in vivo test, Can. J. Microbiol., 2017, 63(3), 219–227 CrossRef CAS PubMed.
- C. Wang, T. Chang and H. Yang, et al., Antibacterial mechanism of lactic acid on physiological and morphological properties of Salmonella enteritidis, Escherichia coli and Listeria monocytogenes, Food Control, 2015, 47, 231–236 CrossRef CAS.
- X. Zeng, W. Tang and G. Q. Ye, et al., Studies on disinfection mechanism of electrolyzed oxidizing water on E. coli and Staphylococcus aureus, J. Food Sci., 2010, 75(5), 253–260 CrossRef.
Footnote |
† These authors also contributed equally to this work. |
|
This journal is © The Royal Society of Chemistry 2019 |