DOI:
10.1039/C9RA05188K
(Review Article)
RSC Adv., 2019,
9, 26142-26164
Recent advances in hexavalent chromium removal from aqueous solutions by adsorptive methods
Received
8th July 2019
, Accepted 13th August 2019
First published on 21st August 2019
Abstract
Chromium exists mainly in two forms in environmental matrices, namely, the hexavalent (Cr(VI)) and trivalent (Cr(III)) chromium. While Cr(III) is a micronutrient, Cr(VI) is a known carcinogen, and that warrants removal from environmental samples. Amongst the removal techniques reported in the literature, adsorption methods are viewed as superior to other methods because they use less chemicals; consequently, they are less toxic and easy to handle. Mitigation of chromium using adsorption methods has been achieved by exploiting the physical, chemical, and biological properties of Cr(VI) due to its dissolution tendencies in aqueous solutions. Many adsorbents, including synthetic polymers, activated carbons, biomass, graphene oxide, and nanoparticles as well as bioremediation, have been successfully applied in Cr(VI) remediation. Initially, adsorbents were used singly in their natural form, but recent literature shows that more composite materials are generated and applied. This review focused on the recent advances, insights, and project future directions for these adsorbents as well as compare and contrast the performances achieved by the mentioned adsorbents and their variants.
1. Introduction
Over the past decades, the preparation and application of various adsorbent materials for the sequestration of toxic hexavalent chromium (Cr(VI)) from environmental samples have received vast attention from researchers across the world.1–5 Chromium(VI) compounds, like hydrogen chromate (HCrO4−), chromate (CrO42−) and dichromate (Cr2O72−), owe their toxicity to the high solubility and diffusivity which permit them to cross the biological membrane tissues easily.6,7 Cr(VI) is toxic even at parts per billion levels.8 The anthropogenic sources of Cr(VI) compounds in environmental samples include seepage or careless disposal from the electroplating, dying, nuclear power, metal finishing, leather tanning, photography and textile industries.9–14 Another environmentally significant chromium species is the trivalent chromium (Cr(III)) which is considered non-toxic at minute concentrations; however, at high concentration, it can be toxic.
Conventional methods such as chemical precipitation, ion exchange, reverse osmosis, coagulation, and adsorption have been used to remove metal ions from different matrices.1,2,4,15–19 Amongst these methods, adsorption is viewed as superior to other methods because of its simplicity, ability for regeneration, cost-effectiveness, and enabling large-scale applications.20,21 Confiscation of Cr(VI) from aqueous solutions by adsorption methods has been accomplished by exploiting its physical (physisorption), chemical (chemisorption) and biological (bioremediation) properties due to its dissolution tendencies in aqueous solutions.1,2 A plethora of adsorbents of different origins including synthetic polymers, activated carbons, biomass, graphene oxide, nanoparticles, and biosorbents have been investigated for Cr(VI) removal. Adsorbents are used in their native form, but the general trend lately has been that of modified adsorbents either by crosslinking, grafting, changing the chemical form or through engineered composite materials. Some adsorbents are used as scaffolds to generate new materials with improved functional groups (adsorption sites) in an adsorbent1@adsorbent2@adsorbent3 fashion. It has been reported that adsorption is limited by dominant functional groups on the surface and within the pores of an adsorbent.22 Hence, the new general trend of re-functionalization of existing adsorbents. It is not a forgone conclusion that composite materials produce superior performance. For example, re-functionalization of activated carbons can result in the loss of carbon structure with the resultant carbon exhibiting pores that extend from one side to the other side of the particle surface. Such types of materials are not ideal for adsorption as they will mostly act as sieves than adsorbents. To date, researchers are striving to find suitable adsorbents for Cr with excellent adsorption capacity, selectivity, and faster binding kinetics while using the minimum dosage concentration. Recent literature shows a wide variability of adsorption performance for several adsorbents. Typical examples include Fe3O4@titanium residue, carbon-coated montmorillonite nanocomposite, electrospun carbon nanofiber mat which yielded the adsorption capacities (mg g−1) of 14,23 100,24 and 119,25 respectively. The Fe3O4@titanium residue adsorbent exhibited superior selectivity for Cr(VI) in the presence of chloride, phosphate, sulphate, arsenite, and arsenate ions but its selectivity collapsed in a binary solution containing fluoride.23 This is an indication that there is a room for new research in terms of adsorbent combinations to generate super adsorbents.
The demand for new or improved adsorbents has been sparked by the complex interactions of Cr(VI) with adsorbent functional groups leading to transformation of Cr(VI) to Cr(III), thus, incomplete removal of Cr. Consequently, four types of mechanistic interactions of Cr(VI) with biomaterials were identified.26 The first scenario involves adsorption through electrostatic forces between positively charged adsorbent functional groups (e.g., NH4+ or COOH2+) and anionic Cr(VI) species. The second mechanism pertains to adsorption of Cr(VI) onto the adsorbent surface, followed by a complete reduction of Cr(VI) to Cr(III). In the third situation, some fraction of anionic Cr(VI) is adsorbed while another fraction of Cr(VI) is reduced to Cr(III) followed by adsorption of Cr(III) on the surface of the biomaterial. A fraction of Cr(VI) is adsorbed while the other fraction is transformed to Cr(III) and released back into solution in the fourth scenario. The transformation (reduction) of Cr(VI) to Cr(III) can either be direct or indirect.27 Direct conversion takes place when Cr(VI) ions are attached to adsorbent sites that can donate electrons (O, S, and N) to facilitate reduction. Besides, the presence of cations like Fe2+ on the adsorbent surface also aids in the reduction of Cr(VI) to Cr(III). Indirect reduction happens when Cr(VI) attached to an adsorption site is reduced by the electrons from adjacent functional groups. While Cr(VI) undergoes reduction to Cr(III), the adsorption sites get transformed (oxidized) and this hinders the reusability of adsorbents for Cr(VI) uptake, for instance, the conversion of hydroxyl to carboxyl groups. Even though some studies still disregard the effect of reduction,21,28 there has been many studies that have accounted for the reduction possibility using several techniques like Fourier transform infrared spectroscopy, energy dispersive X-ray spectroscopy and X-ray photon electron spectroscopy (XPS) to show the oxidation of surface groups and/or presence of Cr3+ species.15,16,29,30 In addition to the four mechanisms listed above, esterification and coupling reactions involving Cr(VI) ions and catechol have been reported.31
Conversion of Cr(VI) into Cr(III) on the surface of nanoscale zerovalent iron has been reported as the mechanism of removal.3,32 The handling of the as-reduced Cr(III) by various studies has been different. While some attempted to explain the re-adsorption of Cr(III),23,33,34 others were content with the fact that Cr(VI) was transformed to its less toxic form.3,15,16,35,36 The neglect that high concentrations of Cr(III) is toxic could be detrimental. Besides the chemisorption processes mentioned, physisorption processes are also well-known particularly with materials possessing high surface area and large pore volumes like activated carbon, graphene oxide, and zeolites.
Recent review articles focusing on the use of different adsorbents in the removal of heavy metals, including Cr(VI) from aqueous solutions have emerged.37–42 Most of these review articles focused on the application of specific adsorbents such as silica-based materials,37 polypyrrole-based adsorbents,38 advanced carbon nanotubes,39 clay minerals,40 functionalized carbon nanotubes, graphene41 and nanomaterials in general.42 Despite the extensive existence of adsorbents for Cr(VI) removal from aqueous solutions, new adsorbents, and modification of existing adsorbing materials have been recently reported.3,43–50 Unlike in previous review articles,37–41 this work focused on reviewing the major adsorbents reported in the literature (up to the year 2019) mainly for the removal of Cr(VI) from aqueous solutions. The objectives of this review included outlining some of the recent developments in the field of adsorption, where a trend of utilizing composites, particularly incorporating nanomaterials, has dominated the recent literature. Also, to provide one-stop resource material for Cr(VI) adsorption.
2. Adsorbent classification, characteristics and development history
Several classes of adsorbents including synthetic polymers, biopolymers, graphene-based, activated carbon (AC), silica and biosorbents, are used in Cr(VI) confiscation. Chitin and cellulose are the two most common biopolymers used in adsorption. Cellulose is a polysaccharide whose molecular weight hinges on the plant material it was extracted from as well as the purification procedure used.51 Cellulose exhibit intrinsic characteristics, such as robustness, abundance, biodegradability, nontoxicity, mechanical, and thermal stability.51,52 Also, cellulose is odourless water soluble linear polymer whose monomeric units of β-D-anhydroglucopyranose are covalently bonded via C1–C4 β-glycosidic linkages.53 Its water solubility property is detrimental for its use as an adsorbent in raw form; hence, numerous functionalization procedures have been adopted. Graft and cross-linking modifications have dominated the research, but sophisticated functionalization such as those reported elsewhere54,55 have shown promising results in Cr(VI) removal. Cellulose modified with β-cyclodextrin and quaternary amines demonstrated high adsorption rates and rebinding properties governed by the pseudo-second-order kinetic model.56 Chitin is derived from shells of crustaceans like crab, crayfish, prawn, and shrimp. Due to the low adsorption performance of chitin, earlier research was concentrated on fabricating chitosan from chitin through deacetylation usually employing a strong base like NaOH to provide reactive sites enriched with NH2 and OH functional groups.57 Chemical modification of cellulose and chitosan with organic ligands and cross-linking21,36,51,54,58,59 dominated earlier research but recently emphasis has been on the incorporation of nanomaterials into their backbone.16,60,61 The latter has been reported to improve the adsorption performance for both adsorbents.
Another interesting class of adsorbents is the graphene oxide (GO) based adsorbents, mainly because of the abundant –OH, –COOH and –C
O functional groups on their surface imparted during oxidation of graphite.62 The presence of such groups makes GO an ideal adsorbent for metal ion chelation and excellent hydrophilic properties owing to hydrogen bonding. However, its recovery from the water after adsorption is troublesome given its high dispersion in water. To avert that, GO have been used as building blocks of other adsorbents in composite manufacturing. As high as 539.53 mg g−1 adsorption capacity was reported for Cr(VI) removal using a composite material prepared from polyethyleneimine (PEI) and GO.60 However, the adsorption equilibrium was reached after 14 h, demonstrating a need for further improvement in such types of adsorbents. Evaluation of the selectivity using solutions containing Ni(II), Cu(II), and Cd(II) cations was unjust because the mode of Cr(VI) removal by the GO–PEI adsorbent was described to proceed via electrostatic interaction between anionic Cr(VI) and protonated amine groups. Therefore, a repulsion of the cationic species was always going to be expected under conditions favouring electrostatic attraction of Cr(VI) to positively charged adsorbent sites. Anions, like sulphates, phosphates, nitrates, fluorides, and chlorides could have been explored.
The abundance, low cost, and presence of diverse functional groups (hydroxyl, carboxyl, carbonyl etc.) in agro-based by-products such as shells and kernels elicit interest from scientists to investigate their potential application in the elimination of pollutants from water. However, low surface area and low adsorption performance limit their utilization in pristine form. For example, a solution containing 80 mg L−1 of Cr(VI) at pH 2 was treated with 2 g L−1 of pristine almond green hull and 99.94% removal efficiency was obtained63 while 3 g L−1 adsorbent dosage concentration of Hibiscus cannabinus kenaf yielded a Langmuir monolayer adsorption capacity of 0.582 mg g−1 in 90 min of equilibration time at pH 7.35 Given the high dosage concentrations used (2 and 3 g L−1) in relatively low concentration of Cr(VI) (80 and 50 mg L−1) clearly illustrates the limitation of utilizing raw biosorbents. Hence, research on the improvement of adsorption performance through various modifications of biosorbents is still ongoing.
The agro-waste materials also play a crucial role as precursors for AC manufacturing. ACs are highly carbonaceous materials possessing large surface area, high porosity, and high adsorption capacity.64 Commercial ACs are costly and non-selective. Researchers continue to investigate alternative cheaper and more available sources of carbon, including plant materials, tyres, coal, bones, municipal waste etc. The heating method and the precursor used influence the final characteristics of ACs. Major research was concerned with improving the properties of the AC through investigating different heating and carbonization temperature as well as studying the influence of various activating agents and activation methods. ACs are highly hydrophobic and in turn, have achieved limited success in metal ion removal, including Cr(VI) as opposed to organic pollutants. Ecofriendly and low-cost ZnO-tetrapods/activated carbon (ZnO-T/AC) nanocomposite synthesized by the hydrothermal method were investigated for Cr(VI) adsorption and were found to provide high adsorption efficiency.65 The ZnO-T/AC nanocomposite was designed to integrate the intrinsic properties of AC and nanoparticles. On the same token, there seems to be limited research on the study of activated carbon/nanoparticles/silica composite for the removal of Cr(VI). Investigation of magnetic nanoparticles supported on AC (AC@Fe3O4@SiO2–NH2–COOH)66 as potential adsorbents for the decontamination of Cr(VI) is one of the few examples in this area. Promising Cr(VI) removal efficiency of 70% from water solution having a salinity of 20
000 ppm adjusted to pH 7 was reported.66 Systems of this nature (AC@Fe3O4@SiO2–NH2–COOH) require skilled chemists for synthesis as they involve lots of steps and reagents. The inclusion of nanomaterials in adsorbents should be conducted in such a way to prevent leaching of nanoparticles during their use. Nanomaterials exhibiting high surface area, non-toxic, easy removal after sorption, and pronounced selectivity of pollutant at trace concentrations are desirable.42
Excitement over the application of mesoporous silica-based adsorbents was triggered by their excellent properties such as uniform pore structure consisting of well-defined pore size, high specific surface area, and larger pore size.67–70 In Sharma et al.,65 it was pointed out that complicated synthesis, longer reaction time needed for synthesis, separation difficulties coupled with the high cost of reagents has limited the practical applications of silica adsorbents. Cr(VI) recoveries between 91.9 and 103% from lake, rain, and river water were reported for carboxylic acid-functionalized mesoporous silica.67 More details on the merits and demerits of various adsorbents can be found in the review by Mohan and Pittman.71
3. Toxicity of chromium
Even though Cr is known to exhibit several oxidation states, but only two forms, Cr(III) and Cr(VI), are of environmental importance. In the aqueous environment, Cr(III) may hydrolyze into several species including Cr(OH)2+, Cr(OH)2+, Cr(OH)4−, neutral species Cr(OH)3− and polynuclear species Cr2(OH)2 and Cr3(OH)45+.63 These hydroxides are less mobile, soluble, and toxic to living organisms due to their tendency to form complexes with organic ligands (natural organic matter) in the environment.72 Also, at minute concentrations, Cr(III) is needed for the metabolism of glucose, lipid and amino acids2 with a recommended average amount per human body per day of 0.4–6 mg.32 In humans, hair has been reported to be the highest accumulator for Cr compounds with concentrations ranging from 0.23 to 3.8 mg kg−1.2 The toxicity of Cr(III) compounds is not as well-documented as that of Cr(VI) compounds but suppression of the immune system activity73 and red blood cells damage74 have been reported. There has been a growing body of evidence narrating the possible conversion of Cr(III) to Cr(V) and subsequently to Cr(VI) compounds in the extracellular75 and intracellular structures.75,76 Lindsay and Farley77 reported the reoxidation of Cr(III) to Cr(VI) during wastewater treatment by chlorination. This new evidence shows that the possible toxicity of Cr(III) compounds at high concentrations cannot be ignored. As such, few studies have focused on the adsorptive removal of Cr3+ from various matrices.78,79
On the other hand, Cr(VI) hydrolyzes to Cr2O72−, CrO42− and HCrO4− which are strong oxidants.71 The Cr(VI) compounds are more mobile and soluble, making them bioavailable. The hexavalent Cr species are toxic as they are associated with a variety of detrimental health effects such as skin rash, weakened immune system, nose irritations and nosebleed, ulcers, allergic reactions, kidney and liver damage, genetic material alteration, gastric damage, and even deaths.80 The toxicity of Cr(VI) on humans has been primarily accredited to the chemical structural resemblance between Cr oxyanions [CrO42−] and sulphate ions [SO42−] making the former able to cross the biological membranes using sulphate routes.81 A detailed study reported by Wise et al.82 showed that Cr(VI) is genotoxic to human urothelial cells, thereby inducing aneuploidy. The DNA damage was inflicted with low Cr(VI) concentrations varying from 0.5–3 μM.83 Due to the aforementioned health effects related to Cr(VI) toxicity, numerous studies have focused on developing methods for the removal of Cr(VI) compounds from various matrices.
4. Removal of Cr(VI) from aqueous solutions
Water treatment technologies for Cr(VI) mitigation emphasize on removal, containment, and/or reduction.2,84–86 Hence, some studies have capitalized on the solubility of Cr(VI) at all pH ranges by first reducing the Cr(VI) to its less toxic form of Cr(III) followed by precipitation.2 Viti et al.87 reported the reduction of Cr(VI) by H2S to proceed via three steps: (i) reduction of sulphates, (ii) reduction of chromate by sulphides and (iii) precipitation of Cr(VI) by sulphide. Chemical precipitation is the most viable commercial method for the removal of metal ions, but it has not enjoyed an overwhelming application in the removal of Cr(VI) because chromates do not precipitate easily using the conventional methods.88 Other challenges associated with chemical precipitation are the generation of sludge rich in toxic metal ions as secondary pollutants, water hardness, and slow reaction speed.89 In this case, it is clear that Cr(VI) abatement strategies require more resources in terms of chemicals, equipment, and intensive labor. Another point in case is the filtration systems which require membranes that can be costly at times and additional chemicals needed to convert Cr(VI) into other forms. Elsewhere, prior to filtration, Cr(VI) was initially reduced with ferrous sulphate to Cr(III) followed by coagulation.18 In a different study, three polymers (chitosan, polyethyleneimine, and pectin) were investigated for a polymer-enhanced ultrafiltration method required for the removal of Cr(VI) from aqueous solutions.9 In their investigation, Aroua et al.9 required an ultrafiltration system that was equipped with polysulfone hollow fiber membrane. In a different study, the application of plant species for abatement of Cr(VI) in irrigation water resulted in the conversion of hexavalent chromium to trivalent chromium in plant species (Phragmites australis and Helianthus annuus).85 In any case, the total chromium removal reported using the two plant species ranged from 54% (Phragmites australis) to 70% (Helianthus annuus).85 A simple conversion of Cr(VI) to its less toxic form of Cr(III) might not be a complete ideal solution for water that enters the environment due to the possibility of unknown environmental conditions that could reverse the situation. Thus, ion exchange and reverse osmosis are limited by their high cost, membrane fouling, and poor stability.90,91 The current review focuses only on adsorption methods as applied in Cr(VI) removal.
4.1. Adsorption process
Adsorption refers to an accumulation of adsorbates at a surface of an adsorbent or the interface between two phases. Adsorption is mostly an exothermic process due to the decrease in surface energy of the adsorbent impelled by the inhibition of the adsorption site atoms' movement induced by the attachment of adsorbate molecules. Adsorbates may attach on the adsorbent through electrostatic attraction, ion exchange, ion-pair interactions, van der Waals forces, cation–π and hydrophobic hydration.58,92 Adsorption could be described by physisorption and chemisorption processes. In physisorption, the bonding of adsorbates to the surface of adsorbent proceeds through weak reversible van der Waals forces while chemisorption takes place via chemical bonds that are mainly irreversible.42 Some studies have postulated that adsorption of Cr(VI) by adsorbents is through electrostatic attractions,15,93 adsorption, and reduction16,23 or just plain adsorption.63 The type and nature of adsorbent and its functional groups determine the mechanism of metal abstraction. The sections below detail how various adsorbents were fabricated to maximize their Cr(VI) uptake from aqueous media with more emphasis on recent trends adopted for the synthesis of adsorbents.
4.2. Application of various adsorbents for Cr(VI) removal
Adsorbents come in various formats including synthetic polymers, waste materials, nanomaterials, and biomaterials. The general rule is that the adsorbents must be insoluble in the solution that contains the analyte to be adsorbed and should contain high surface area with some porosity for physisorption and complementary functional groups for the chemisorption process. In addition, adsorbents must remain intact during the recovery of adsorbates, i.e., should show mechanical stability in the presence of the desorbing medium. To meet such requirements, cross-linking reagents are utilized. Not only does cross-linking improves rigidity, stability, and ruggedness of adsorbents, but it also helps with the incorporation of functional groups necessary for metal abatement as well as serving as scaffolds for further functionalization, e.g., grafting. The inclusion of new functional groups either through cross-linking or grafting enhances the selectivity during metal sequestration. In the case of polymers, size and length of monomers influence the performance in adsorption. Too bulky and longer alkane chains might cause steric hindrance and increased hydrophobicity of which both would lead to poor adsorption performance for metal ions. Therefore, careful consideration and matching of analyte chemistry to that of adsorbent surface functional groups is paramount for optimal performance.
4.2.1. Synthetic polymer-based adsorbents. Recently, the application of synthetic polymers as metal ion abstractors has been a subject of intense research.35,54,92,94–96 Cross-linked poly(4-vinyl pyridine):divinylbenzene copolymers quaternized with amino groups were used as anion exchangers for the removal of Cr(VI).35,97 The quaternized materials performed well in the removal of Cr(VI) from aqueous solutions, but a slight reduction in adsorption performance was observed in the presence of sulphate ions. Kalidhasan et al.92 developed a highly efficient adsorbent for Cr(VI) sequestration by impregnating Amberlite XAD, a synthetic polymer, with tetraoctylammonium bromide (ionic liquid). A Langmuir adsorption capacity of 196.1 mg g−1 was reported, and the enhanced removal of Cr(VI) was attributed to the pivotal role played by ion-pair, electrostatic, cation–π, van der Waals and hydrophobic hydration interactions between sorbate and adsorbent.92Elsewhere, ion-imprinted polymers (IIPs) were utilized to improve the removal of Cr(VI) in the presence of co-ions.98,99 IIPs are defined as materials synthesized by incorporating the analyte as a template during synthesis resulting in a polymer with cavities mimicking the shape, charge, size, and functionality of the template ion. In this context, Pakade et al.98 synthesized a Cr(VI)-IIP from a copolymer of 2-vinyl pyridine and 4-vinyl pyridine containing styrene monomers as spacers to accommodate the bulkiness of Cr(VI) induced by the oxygen atoms around Cr. The prepared IIP exhibited superior selectivity of Cr(VI) against ten-fold high concentration of sulphates from acid mine drainage water sample compared to its control (non-imprinted polymer (NIP)). In a different study, Bayramoglu and Arica99 prepared a Cr(VI)-imprinted polymer from poly(4-vinyl pyridine-co-hydroxyethyl methacrylate) co-monomers. The synthesized IIP exhibited a maximum adsorption capacity of 172 mg g−1. Adsorption equilibrium was reached within 40 min, illustrating that the process was faster and was explained by pseudo-second-order kinetic mechanism. IIP for Cr(VI) was selective in the presence of Cr(III) and Ni(II). Even though IIPs have exhibited superior selectivity towards Cr(VI) with excellent adsorption capacities, the unavailability of commercial sorbents is still an issue of concern. Hence, new sorbents with improved performance are continually sought after.
In a detailed review on the use of different types of adsorbents for Cr(VI) sequestration, it was projected that the application of adsorbents modified with nanomaterials could be an area of intense research.100 Indeed, this has been the case, judging by the publications discussed in this work. Taghizadeh and Hassanpour94 prepared a Cr(VI)-IIP anchored on magnetic multi-walled carbon nanotubes via precipitation polymerization employing 4-vinyl pyridine (4-VP) and 2-hydroxyethyl methacrylate (HEMA) co-monomers. The reported maximum adsorption capacity reached after 30 min of equilibration was 56.1 mg g−1, and the polymer demonstrated a high single selectivity sorption for the Cr(VI) ions in the presence of competing ions (Cu2+, Ni2+, F−, NO3− and SO42−) but nitrate was the prevalent competitor.94 It has to be noted that, the anchoring of 4-VP and HEMA on magnetic multi-walled carbon nanotubes yielded a polymer with lesser performance (56.1 mg g−1 (ref. 94) vs. 172 mg g−1 (ref. 99)) compared to the simple IIP prepared from the same functional monomers. The added cost of synthesizing the magnetic multiwalled carbon nanotubes plus the inferior adsorptive performance make the new method not ideal. Probably, the magnetic multiwalled carbon nanotubes owing to hydrophobicity were not involved in Cr(VI) abatement or the adsorption sites were not easily accessible, hence lower adsorption capacity.
Liang et al. also employed 4-VP and HEMA co-monomers for the synthesis of magnetic Cr(VI) IIP, but the polymer was supported on silica nanoparticles.101 The use of silica nanoparticles tremendously improved the adsorption performance of the polymer by almost 6-folds (up to 311.95 mg g−1). This could be attributed to the high surface area to volume ratio displayed by nanoparticles in relation to multi-walled carbon nanotubes. A combination of vinylimidazole and 3-aminopropyltriethoxysilane co-monomers was utilized to produce a Cr(VI) IIP imprinted on the surface of magnetic nanoparticles.102 The reliability and feasibility of the prepared IIPs were evaluated with real samples, tap water, lake water and river water with a limit of detection (CLOD = 3Sb/m) of 0.29 ng mL−1. Velempini et al.54 prepared Cr(VI)-imprinted polymer by cross-linking carboxymethylcellulose-ethylenediamine with epichlorohydrin. The maximum adsorption capacity described by a Langmuir isotherm was found to be 177.62 mg g−1 at 25 °C and sulphate was reported to be the highest competitor. In the latest work, the researchers have been able to achieve high adsorption capacities and selectivity with their materials. This indicates that a careful selection of a set of monomers and their assimilation to magnetic nanoparticles immobilized to some form of support (e.g., carbon, nanoparticles, graphene oxide, and cellulose) could be the future direction for producing high efficient Cr(VI) IIPs, but silica nanoparticles seem to give best results.
4.2.2. Natural polymer-based adsorbents. Adsorption employing biopolymers is among the recommended methods for the treatment of metal ion pollutants due to the low cost of biomaterials, high effectiveness, minimum chemicals needed, and regeneration opportunities.59–61,103,104 Natural polymers like cellulose, chitin, and chitosan have been used for trace metal mitigation for decades. Chitosan contains chemical functional groups, like NH2 and OH, that can aid in trace metal sequestration and complexation, while cellulose is a polysaccharide mostly dominated by OH− and CHO− groups. Mainly, the modifications of chitosan can be by physical methods (changing from flakes to beads), or chemical methods where cross-linking or grafting functional groups is carried out.21,59,104–106 Recently, more composite materials incorporating chitosan backbone have been reported.60,61 Preethi et al.15 synthesized biopolymers assisted oxyhydroxide materials, aluminum–lanthanum mixed oxyhydroxide (ALMOH) and chitosan/aluminum–lanthanum mixed oxyhydroxide (CSALMOH), and evaluated them for Cr(VI) removal. The maximum adsorption capacities reported were 49.80 and 78.90 mg g−1 for ALMOH and CSALMOH, respectively. The sulphate and the carbonate were found to be the most significant competitors for Cr(VI) adsorption, and the overall selectivity order was SO42− > HCO3− > NO3− > PO43− > Cl−.15 Bhatt et al. experimented the trimesic acid-cross-linked chitosan for the adsorption of Cr(VI).58 Ionic, π–π and hydrogen bonding interactions were described as the forces of recognition that participated in the abstraction of the metal ion resulting in adsorption capacity of 129.53 mg g−1 from an equilibrium concentration of 336.63 mg L−1 but after a very long contact time of 882.5 min. Such long equilibration time could be detrimental for the employment of the adsorbent in industrial applications. Gopal Reddi et al.21 investigated the removal of copper and chromium from aqueous solutions using chitosan-g-maleic anhydride-g-ethylene dimethacrylate adsorbent. The double grafted copolymer of chitosan demonstrated excellent removal capabilities. An adsorption loading of 266.67 mg g−1 was reported for the removal of Cr(VI) by ionic liquid impregnated phosphate chitosan adsorbents.16 It has been reported that superior adsorption performances can be achieved by the modification of both the structure and the functional groups on the adsorbent.16 The structural modification was achieved by cross-linking the chitosan with phosphate while ionic solid ethylhexadecyldimethylammonium bromide was used to functionalize the material. The high adsorption capacities achieved with the modified chitosan adsorbents pointed to the synergistic effects of composite materials and their advantages over individual materials. Biocompatible magnetic nanoparticles imprinted cellulose composites were synthesized for the remediation of Cr(VI) from aqueous solutions.55 In this study, three different adsorbents, nanoparticles, cellulose and hydrotalcite or hydroxyapatite (a double-layered hydroxide), were used to fabricate a biocompatible material of the three-layered structure, Fe3O4@cellulose@double layered hydroxide. It was found that the three-layered adsorbents performed better than the corresponding two-layered adsorbents, Fe3O4@cellulose or cellulose@double layered hydroxide, with adsorption capacities ranging from 25.259–28.739 mg g−1. The selectivity of the prepared three-layered adsorbents was negatively affected by the presence of carbonates (HCO3−) particularly because of the tendency of HCO3− to increase the pH of solution leading to diminished active sites.55 However, this engineered combination did not produce an adsorbent with higher capacity in comparison to, say the ionic liquid impregnated sorbents reported elsewhere.16 It also goes to show that complexity does not always yield better performance. Therefore, in as much as researchers modify or create these composite adsorbents, performance versus cost need to be considered all the time for the adsorption process to remain highly efficient, simple, and cost-effective.
4.2.3. Application of bio-adsorbents for Cr(VI) adsorption. The continued challenge of getting alternative biosorbent materials that are cost-effective and efficient have opened avenues for researchers to try almost any material of plant origin in adsorption of metal ions.1 Lignin, hemicellulose, and cellulose are the major components of biomaterials responsible for metal chelation/complexation and ion exchange during application of such adsorbents.107 Besides the bulky structures of lignin, cellulose, tannins, and hemicellulose as well as trace metal ions, biomaterials possess a cornucopia of functional groups like aldehydes, ethers, alcohols, ketones, and esters.108 Owing to their abundant availability, biodegradability and cheap cost, numerous biosorbents from different sources, Macadamia nutshells,108 pine cone biomass,109 Cannabinus kenaf,35 Masau stones,34 sawdust,6 almond green hull,63 grape peelings,110 lemon peel powder,111 coir pith112 and fungal biomass113 have been successfully used for the adsorption of Cr(VI). Despite the breakthroughs made in using native biosorbent materials, low surface area, low adsorption capacities, high chemical, and biological oxygen demand, as well as total organic carbon caused by leaching of organic components, remain the main drawbacks of applying biosorbents in their raw form.114,115Several treatment methods have been proposed to address these limitations. These include biological treatment with bacteria or fungi, chemical treatment (or bleaching) with an alkaline solution, and oxidizing agents, physical treatment methods like mechanical agitation or sonication, and physicochemical methods such as steam explosion.108 In addition to the above, cross-linking, grafting, converting to activated carbon or fabricating biocomposites are usually the secondary treatments undertaken.62,65,67,68,70,72 Recent literature has revealed that new or alternative adsorbents for the removal of Cr(VI) are experimented.35,63,116 The limitation of biomaterials is the poor surface area, and as such, they are rarely used as scaffolds for preparing composite adsorbents of the type adsorbent1@adsorbent2@adsorbent3 format. New literature on this field mainly focuses on cross-linking coupled grafting. In addition, biomaterials are mainly used as precursors in the production of activated carbons. The typical study, Masau stones cross-linked with epichlorohydrin (ECH) and grafted with diethylenetriamine (DETA) were experimented for the removal of Cr(VI) from aqueous solutions.34 The adsorption capacity of 87.33 mg g−1 was achieved within 250 min. A complex mechanism of removal involving adsorption of Cr(VI) through electrostatic attraction, hydrogen bonding, and π–π interactions was reported.34 Application of lignocellulose-based materials in metal ion confiscation has been covered in several recent reviews.1,2,114,115,117 Hence, the current review will not attempt to repeat those reviews but will instead focus on the overall trend in adsorbent synthesis and application.
4.2.4. Carbon-based adsorbents. Review articles have been presented on the application of AC in the remediation of pollutants such as chromium from water since over a decade ago.71,118 This implies that there is a strong potential for the usage of AC in the removal of chromium from water. The preparation of the AC is usually performed by the carbonization of the raw material at temperatures below 800 °C in the absence of oxygen followed by the activation of the carbonized product (char), which is done either physically or chemically.117,118 Commercial ACs are expensive, and this has led to the generation of low-cost adsorbent by many researchers using waste materials.118–122 Some ACs used for the removal of Cr(VI) from aqueous solutions recently were derived from fox nutshell,119 wood apple shell,120 mango kernel121 and termite feces.122 The AC adsorbents showed good recognition ability for Cr(VI) removal from aqueous solutions with an adsorption capacity of up to 315 mg g−1.123 For example, the adsorption capacities of Cr(VI) at pH 3.0 and temperature of 40 °C were 315 and 186 mg g−1 for the KOH-activated and acid-activated (Acticarbone) carbons, respectively.123 In the same context, the maximum adsorption capacity achieved by Acharya et al.124 for mitigation of Cr(VI) from wastewater by AC developed from Tamarind wood activated with zinc chloride was 28.019 mg g−1. Several investigations have shown that the maximum removal of Cr(VI) from aqueous solutions occurs at acidic conditions probably due to the neutralization of negative charges on the surface of the adsorbents by excess hydrogen ions, thereby enabling the diffusion of hydrogen chromate ions (HCrO4−) and their subsequent adsorption.121,123In addition to using the as-prepared AC, new literature has emerged where re-activation, modification, and functionalization process of the native AC were undertaken.125–131 The post-treatment of AC allows for the addition of new functional groups, increase the amount of existing functional groups, changing from one form to another (e.g., from thiol to amino) or improve on the physical attributes such as specific surface area, pore-volume, and pore size. Functional groups bearing oxygen atoms (phenolic hydroxyl, carbonyl or carboxyl groups) were produced by chemical oxidation of AC.126,132 Oxidizing agents including H3PO4,133 HNO3,125 and KMnO4 (ref. 127) and activating agents such as HCl,134 cationic surfactant135 as well as peracetic acid126 were employed for oxidative post-treatment of AC. Amination of epichlorohydrin cross-linked AC yielded a Langmuir monolayer adsorption capacity of 145 mg g−1.136 In addition to cross-linking and grafting, AC are used as supports for producing much complex systems of the adsorbent1@adsorbent2@adsorbent3. A typical study of the three-level adsorbent composite prepared from carbon@ferrous sulfide@iron showed potential in the removal of Cr(VI) from aqueous solution.72 It should be emphasized that AC does not only act as support, but it can also partake in the adsorption either through physisorption (owing to their large surface areas) or chemisorption (due to the presence of heteroatoms on the surface). Particularly, for Cr(VI) removal, the heteroatoms participate in the reduction process.
Besides AC, biochar and carbon nanotubes are other carbon variants that have been experimented for Cr(VI) adsorption. Ab initio studies demonstrated that carbon nanotubes functionalized with titanium complexes could be potential adsorbents for Cr(VI) removal due to the large specific surface area, structural diversity, good chemical stability, small size, possible regeneration low density and reusability.137 Recently, commercial carbon nanotubes modified with hydrogen peroxide were experimented for Cr(VI) adsorption, and the energies of adsorption obtained from the Dubinin–Radushkevich isotherm ranging between 0.371 kJ mol−1 and 0.870 kJ mol−1 indicated that the process was through physisorption.138 A composite material prepared from AC/Fe3O4/SiO2 capped with amine and carboxylic groups exhibited good recognition of Cr(VI) in the presence of Pb(II).66 The inclusion of nanoparticles in composite fabrication seems to bring beneficial results and adsorbent composite performance enhancement. Magnetite nanoparticles have been widely explored by many researchers chiefly because not only do they offer quick and easy separation with the aid of an external magnet but also their adsorption capacity and inherent large surface area.66 Exploration of other nanomaterials for Cr(VI) decontamination has also emerged.
4.2.5. Nanomaterial based adsorbents. Nanomaterials are defined as materials and structures having a particle size diameter of 1 to 100 nm, at least in one dimension possessing unique optical, mechanical, and magnetic properties.42 Due to the large surface area to volume ratio, nanomaterials have attracted an overwhelming interest as adsorbents. Use of bare nanoparticles in adsorption is problematic owing to difficulties in separating them from aqueous solution after adsorption. To address such challenges, recent literature has concentrated on coupling nanoparticles with other adsorbents to prepare composite materials.43 Dinari and Haghighi139 synthesized nanocomposites on aromatic polyamide and modified ZnO nanoparticles for removal of Cr(VI) from water. The improved removal of Cr(VI) was credited to the s-triazine heterocyclic rings and silane coupling agent attached on the surface of ZnO nanoparticles. The schematic representation showing the preparation of modified ZnO nanoparticles modified by 1,3,5-triazine core silane coupling agent is presented in Fig. 1.
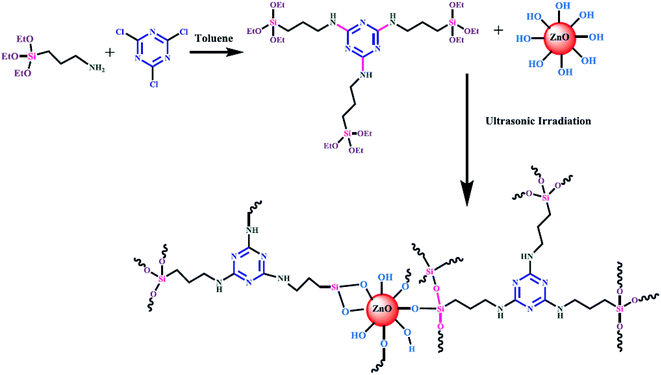 |
| Fig. 1 Surface modification of ZnO nanoparticles by 1,3,5-triazine core silane coupling agent.139 | |
Ren et al.23 successfully synthesized porous magnetite nanoparticles by reducing titanium residue with pyrite under N2 atmosphere. The porous magnetite particles had a surface area of about 11.2 m2 g−1 and the Langmuir adsorption capacity of 14.49 mg g−1. The Cr(VI) was adsorbed and reduced to Cr(III) by the electrons in the system, and in turn, Fe(II) was oxidized to Fe(III) in the process. Dima et al.60 experimented the removal of Cr(VI) by reticulated chitosan micro/nanoparticles (MCH) synthesized by inducing the gelation of a chitosan solution with tripolyphosphate (TPP), a non-toxic polyanion. The particle size had an average diameter of 100 nm. Cross-linking of MCH particles with TPP improved the removal performance due to the prevention of leaching of chitosan particles. Li et al.22 synthesized chitosan nanofibers with an average diameter of 75 nm by electrospinning. An improvement in adsorption capacity from 26.7 to 68.3 mg g−1 compared to non-electrospun chitosan particles was achieved. The nanofibers showed greater recognition of Cr(VI) in the presence of NO3−, Cl−, Na+, Ca2+, and Mg2+, but were negatively affected by the presence of SO42−. The lower adsorption in the presence of SO42− was attributed to the tendency of SO42− ions to cross-link with protonated amino groups, thereby decreasing the number of the binding sites of chitosan.140 A novel material consisting of composite nanofibers (PAN–CNT/TiO2–NH2) was prepared by electrospinning polyacrylonitrile (PAN) and carbon nanotube (CNTs)/titanium dioxide (TiO2) nanoparticles functionalized with amine groups (TiO2–NH2).30 Excellent adsorption capacity of 714.27 mg g−1 was achieved. This is promising considering the equilibrium time was only 30 min and the initial Cr(VI) concentration was 180 mg L−1 adjusted to pH 2. This is a typical system of adsorbent1@adsorbent1@adsorbent2@adsorbent3@adsorbent4 where the ends (adsorbent 1 and 4) carry amino groups that have a high affinity for Cr(VI). It seems four and higher layered adsorbents could be the future judging by the astonishing adsorption capacity achieved.
Nanoscale zerovalent iron (nZVI) has been used for decades in metal ion treatment owing to its low standard redox potential of E° = −0.44 V making it an efficient reductant upon contact with oxidizing agents like Cr(VI), thus reduced to Cr(III).141 This interest in the use of ZVI could only spell its success in remediating pollutants. Numerous modifications have been reported141 including immobilization of nZVI onto supports (activated carbon,3 waste rock4), doping of ZVI with other metals bimetallic systems,142 and combination of ZVI with Fenton-based oxidation. Bimetallic systems have mainly been applied for the dehalogenation of chlorinated organic compounds141 as opposed to Cr(VI) mitigation. The latest work reported in the literature for removal of Cr(VI) from aqueous solutions includes the application of nanomaterials as adsorbents which include core–shell bimagnetic nanoparticles (CoFe2O4@γ-Fe2O3)143 and magnetic arginine-functionalized polypyrrole nanocomposite.144 A full review on the removal of chromium by nanomaterials can be accessed from ref. 42.
4.2.6. Silica-based adsorbents. Various reports on adsorption of Cr(VI) using silica-based adsorbents have been critically reviewed by Dinker and Kulkarni.37 Hence, the present review discusses the most important findings and recent contributions. Due to their high specific surface area, well-defined pore size and larger pore volume, mesoporous silica materials have been utilized as supports for numerous applications.68–70 In earlier modifications, silica particles were coated with synthetic polymers70 or natural polymers like chitosan145,146 in an adsorbent1@adsorbent2 composition. Recent literature has revealed that the modifications have advanced a step further, where preparation of adsorbent1@adsorbent2@adsorbent3 usually involves silica, polymer and a ligand or nanoparticles. A typical example is a thermo-responsive polymer (MS@APTES@PNIPAm) synthesized by grafting mesoporous silica materials with dual functional reactive agents, 3-aminopropyltriethoxysilane (APTES) and N-isopropyl acrylamide (NIPAm).147 The MS@APTES@PNIPAm adsorbent showed good recognition ability for Cr(VI) removal with an adsorption capacity of 123.8 mg g−1. The adsorption process was described by pseudo-second-order and intraparticle diffusion models. Although the equilibration time was relatively long (360 min) but impressive was the low adsorbent dosage concentration (0.2 g L−1) used for a 30 mg L−1 initial Cr(VI) concentration. Elsewhere, treatment of mesoporous silica embedded with magnetite nanoparticles yielded an adsorbent with 50.51 mg g−1 adsorption capacity.148 In this case, it was clear that the grafting of a thermos-responsive polymer (PNIPAm) vastly improved the capability of Cr(VI) removal by mesoporous silica.The adsorption of Cr(VI) from aqueous solutions using silica-based adsorbent has been reported to be greatly influenced by the pH of the solution.149 In this case, Qiu et al.149 achieved the maximum adsorption capacity of 68 mg g−1 when the solution pH ranged from 2.5–5 due to electrostatic attraction between the HCrO4− ions and the adsorbent, whereas, the maximum adsorption capacities of polymer functionalized silica materials were observed at pH 4 in a different study.150 In this instance, the nature of the adsorbent plays a crucial role for adsorption as Cr(VI) exist in various forms depending on the pH; such that H2CrO4 exists at pH less than 1, while HCrO4− prevails in the pH range of 1.0–6.0, and when the pH exceeds 7, CrO42− is formed in solution.71,149,150
4.2.7. Graphene-based adsorbents. Graphene is a porous material made of carbon atoms with reported theoretical surface area values of up to 2630 m2 g−1.89,151 Lack of heteroatoms in graphene makes it poorly soluble in water due to the absence of hydrogen bonding. Thus, despite the high surface area, graphene is a poor adsorbent due to inferior boundary layer needed for metal diffusion into pores. To improve the adsorption performance, numerous research has focused on the oxidation of graphite to graphene oxide (GO) and reduced graphene oxide (RGO) using the Hummers' method or its modifications. On that note, GO and RGO have enjoyed more applications in adsorption due to the presence of oxygenated functional groups such as hydroxyl, carboxyl, and epoxy groups on their surface.89 These groups are pivotal for metal ion binding. Although RGO and GO possess characteristically high surface areas, when used in their pristine form, they tend to restack and agglomerate owing to π–π interactions between sheets leading inferior adsorption performance.62 These limitations have been addressed by integrating other materials such as nanoparticles and organic ligands into the structural backbone of RGO and GO as demonstrated elsewhere.151–154 Numerous modifications of GO and RGO have been reported for the elimination of hexavalent chromium.45,55,151–153 RGO–Fe3O4 nanocomposites prepared by a solvothermal method were evaluated for the removal of hexavalent chromium.153 Ma et al.151 prepared and evaluated ethylenediamine–RGO (ED–RGO) for the removal of Cr(VI). The adsorption capacity achieved was 80 mg g−1. An improvement of adsorption capacity to 92.15 mg g−1 was achieved when Zhang et al.105 slightly modified Ma et al.151 method by incorporating dimethylformamide (DMF) to produce ED–DMF–RGO. Another type of adsorbent1@adsorbent2@adsorbent3 experimented by Li et al.154 for the removal of Cr(VI). The adsorbent was prepared from magnetic mesoporous titanium dioxide–graphene oxide core–shell microspheres and 117.94 mg g−1 sorption capacity was achieved at pH 6.5. Zhang et al.89 demonstrated the applicability of RGO/NiO nanocomposites synthesized via a facile method for their adsorption of Cr(VI) from aqueous solution. The materials showed maximum performance at pH 4, reaching adsorption capacity of 198 mg g−1. The adsorption performance achieved in Zhang et al.89 with adsorbent1@adsorbent2 composition was better than that reported by a 3-level format in ref. 146 and 94. It still goes to show that chemical compatibility is vital.
4.2.8. Hierarchical porous carbon adsorbents. Some researchers have focused on producing ordered 3D structures with improved access to the adsorption sites and better mass transfer.155,156 Hierarchical porous carbon adsorbent with 3D interconnected structure was prepared from a three-system precursor of resorcinol–melamine–formaldehyde anchored on nanocrystalline cellulose through a sol–gel process.157 The material exhibited a higher adsorption capacity of 463 mg g−1 at pH 1 when evaluated for Cr(VI) removal with a batch method. The higher efficiency was attributed to the well-developed porous structure and large surface area of 1808 m2 g−1. The hierarchical porous carbon doped with nitrogen prepared from silkworm cocoon showed high performance for the removal of Cr(VI) from aqueous solution.158 Porous flower-like hierarchical nanostructure SnS2 (nanosheets) synthesized by heating a mixture of SnCl2·2H2O and thiourea at 170 °C in the presence of air for 2 h demonstrated superior adsorption and photocatalytic reduction properties for the hexavalent chromium in aqueous solution compared to SnS2 nanoparticles and hydrothermally treated g-C3N4.159
4.2.9. Synergistic materials. Due to the complex nature of Cr(VI)-adsorbent interactions mentioned earlier, researchers have found ways to capitalize on the different interactions by producing adsorbents that address two or more mechanisms. Adsorption–reduction mechanism has been exploited by many researchers,16,29,30,147,148,158 and examples from the literature are detailed below. Another synergistic Cr(VI) removal methods are the adsorption/catalysis/reduction.160,161
4.2.9.1. Adsorption/reduction. The synergy in adsorption and reduction during the removal of Cr(VI) by adsorbents has been mentioned by several researchers.16,29,30,44,162,163 Anionic Cr(VI) could be adsorbed through electrostatic bonding on protonated sites or through anion exchange. The strong oxidant nature of Cr(VI) causes the surface where it was bonded to be oxidized while itself is reduced to Cr(III). Acidic conditions and existence of electrons catalyze this reduction. As mentioned previously, heteroatoms O, N and S carry those electrons. The transformation of Cr(VI) to Cr(III) under redox conditions is shown in eqn (1) and (2) with possible formation of an insoluble precipitate Cr(OH)3 at basic pH. |
Cr2O72− + 14H+ + 6e− → 2Cr3+ + 7H2O, E0 = 1.33 V
| (1) |
|
Cr2O72− + 4H2O + 3e− → Cr(OH)3 + 5OH−, E0 = −0.13 V
| (2) |
Two possible mechanisms of reduction have been identified, mechanism I and II shown in Fig. 2.113 Mechanism I is a direct reduction of Cr(VI) to Cr(III) where electrons are provided by the adsorption site. Mechanism II is an indirect reduction where an adsorbed Cr(VI) species receives electrons from an adjacent site to facilitate the reduction. Besides, reductants like sulfides, ferrous iron, thiosulphate, zerovalent iron, and C/FeS/Fe composites have also been used.18,72,164,165 Several studies have accounted for the reduction of Cr(VI) during adsorption3,4,16,30,32,166,167 while others have ignored.21,54,92,147,148,158,168,169 Energy-dispersive X-ray spectroscopy and X-ray photoelectron spectroscopy (XPS) has been used to account for the presence or absence of Cr(III) in the surface of adsorbents after adsorption.15,16,29,30 Atomic absorption spectroscopy can be used to account for Cr(III) in solution even though it does not measure it directly but rather calculated from the total chromium determination. The tendency of Cr(VI) to get reduced to Cr(III) imply that only quantifying Cr(VI) left in solution after adsorption could lead to inaccurate results. Therefore, researchers need to ascertain whether there was any Cr(III) in solution after adsorption.
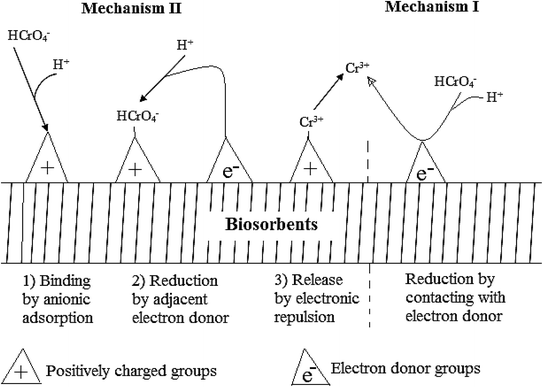 |
| Fig. 2 Adsorption reduction mechanism of Cr(VI) by direct and indirect methods.113 | |
4.2.9.2. Adsorption/catalysis. The application of photocatalytic procedures for the removal of Cr(VI) from aqueous solutions has been reported in the literature.160 In this case, a biocatalyst synthesized from TiO2-impregnated chitosan/xylan hybrid film was applied for photocatalytic reduction of Cr(VI) at a rate of 0.56 × 10−3 ppm min−1 in aqueous solution under ultraviolet irradiation.160 Adsorption ability of hybrid film was due to ionic interactions based on the protonation of the amino group (–NH3+) on its surface, which resulted in highest adsorption (53.22%) occurring at pH 3. In a different study, chromium from water was removed using Mn3O4@ZnO/Mn3O4 composite under simulated sunlight irradiation.22 The authors performed photocatalysis and adsorption concurrently and achieved 92% Cr removal efficiency within 70 min that was better compared to 88.8% achieved within 120 min in the separate two processes. Elsewhere, g-C3N4 nanosheets and a graphene three-dimensional (3D) gel system composed of two metal-free nano-sheets of g-C3N4 and graphene were capable of removing Cr(VI) via the synergistic effect of adsorption and photocatalysis.161 The composite adsorbed 80% Cr(VI) (30 mg L−1) in 30 min.161 Studies in photocatalytic reduction of Cr(VI) and adsorption process of Cr(III) have indicated that these processes are pH-dependent with maximum Cr removal occurring in acidic conditions depending on the surface charge of the adsorbent.160 This could be explained by the variations in the species of Cr(VI) over the pH range (1–14), where at pH = 3.0 for example, Cr exist as HCrO4−, which changes to CrO42− as the pH increases.
4.3. Mechanisms of chromium removal (advancement in interpretation)
Due to the nature and intricacy of the adsorbents used in Cr(VI) removal over the past, several mechanisms for its elimination in water have been reported. Ng et al.170 revisited the adsorption–reduction mechanisms mentioned in the four scenarios in the introduction. The first scenario which concerns only about the adsorption of anionic Cr(VI) through electrostatic attraction appears to be inconclusive and lack the in-depth understanding for the sorption of Cr(VI) compounds according to Ng et al.170 Researchers going for this type of mechanism need to disprove the existence of Cr(III) in solution and on the adsorbent surface. In the second scenario where there is adsorption of Cr(VI) followed by complete reduction of Cr(VI) to Cr(III), the challenge is the sludge formed as a result of recovering the Cr(III) through precipitation. In the third scenario where Cr(VI) is adsorbed by the protonated sites at low pH followed by adsorption of the chelated Cr(III) species, the challenge is the proof of the chelation claim versus the repulsion of Cr3+ by the protonated sites. The greenish colour in the Cr-loaded adsorbent has been used to vouch for the anionic and cationic simultaneous adsorption by Hasan et al.171 but the qualitative evaluation is not enough. Besides the dominant adsorption–reduction mechanism, recent studies have reported three mechanisms all taking place simultaneously, ion-exchange, electrostatic attraction, and reduction.34,36 The ion exchange mechanism was explained on the basis of the presence of CO32− in the interlayer structures of magnetic particles imprinted cellulose-based biocomposites made from hydrotalcite (HT) and hydroxyapatite (Hap).36 The electrostatic attraction of chromate anions to the adsorbent's surface could be the result of the presence of higher valence cations like Ca2+, Mg2+ and Fe3+ in the adsorbent36 as indicated in Fig. 3 and/or protonated sites (OH2+, CN+, NH3+) and quaternary amines (NR3+).34 With such a complex mechanism all taking place simultaneously, the challenge could be getting a suitable solvent for the recovery of adsorbed Cr(VI). Both groups of authors did not perform desorption studies. Instant adsorption and reduction of Cr(VI) to Cr(III) while Fe0 was oxidized to Fe3+ followed by precipitation of Cr3+ and Fe3+ as hydroxides were reported to be the dominant mechanism of removal with nZVI.141 The overall mechanism was represented by eqn (3) and (4). |
Cr2O72− + 2Fe + 14H+ → 2Cr3+ + 2Fe3+ + 7H2O
| (3) |
|
Cr3+ + Fe3+ + 6OH− → Cr(OH)3↓ + Fe(OH)3↓
| (4) |
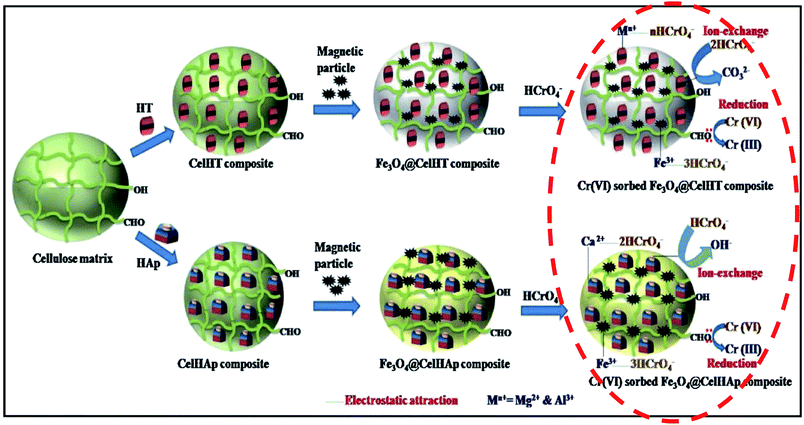 |
| Fig. 3 A possible Cr(VI) sorption mechanism pathway onto magnetic particles imprinted cellulose-based biocomposites. Chromium removal and reduction are occurring at the last step indicated in red, while the other steps show the preparation of biocomposites. Copied from ref. 36, with permission from Elsevier and modified. | |
Two other notable mechanisms involving catechol structures have been reported, esterification between catechol and HCrO4− as well as the coupling between catechol anion and CrO22+.31 However, the esterification mechanism is not popular among researchers, probably because of the difficulties in desorbing the chemically bound Cr(VI). Adsorbents with poor reusability are not ideal as they defeat the purpose of cost-effectiveness in adsorption.
4.4. Re-usability of hexavalent chromium adsorbents
Re-usability of various adsorbents for the removal of water pollutants is generally viewed as a good advancement as this can reduce the costs associated with synthesis and purchase of chemicals required for fabrication or modification of some adsorbents. In addition, re-usability prohibits the disposal of adsorbents after a single-use. This supports the green chemistry principles where it is suggested that the production of a large volume of waste should be avoided and proper waste management system needs to be provided.172 Based on the information presented in Table 1, it is clearly shown that some chromium adsorbents can be re-used after a simple treatment with dilute sodium hydroxide or hydrochloric acid solutions. NaHCO3, NaCl, HCl, HNO3, distilled water and Na2CO3 are other desorbing agents that have been used3,15,23,89 but NaOH is more popular.15,23,30,65,158,167 The choice of the desorbing agent used in desorption is based on the nature of the adsorbent surface functional groups, types of bonding interaction and the adsorption mechanism.148 Araghi et al.148 used sodium hydroxide for chromium desorption as it led to deprotonation of –NH3+ groups on the adsorbent surface which subsequently resulted in the decrease of electrostatic interactions between these groups and HCrO4−.148 In Table 1, the number of repeated use shown indicate the minimum re-usability experiments performed in each case. For example, in the case of polypyrrole magnetic nanocomposite, four adsorption/desorption cycles were conducted.50 In a different study, the ability to re-use the sandwiched nanocomposite adsorbent synthesized using GO, manganese dioxide nanowires, iron oxide nanoparticles and polypyrrole was reported.162 As shown in Table 1, the sandwiched nanocomposite possessed the adsorption capacity of more than 282 mg g−1 within the first four adsorption/desorption cycles; however, this decreased to 264 mg g−1 at the fifth cycle. Other adsorbents which recently showed the capacity to be re-used include AC of Cornulaca monacantha stem,173 ZnO tetrapods as well as AC based hybrid composite,65 AC modified with micro-sized goethite using a facile impregnation method,174 crosslinked chitosan64 and ZnO–TiO2 doped polyacrylonitrile nano fiber-mat.175
Table 1 Re-usability of adsorbents for removal of Cr(VI) from aqueous solutions
Adsorbent |
Number of repeated use |
Adsorption capacity (mg g−1)/performance |
Regeneration process |
Reference |
Polypyrrole magnetic nanocomposite |
4 |
49.7 |
Desorption with 0.1 mol L−1 NaOH and subsequently treated with 2 mol L−1 of HCl |
43 |
Sandwiched nanocomposites |
4 |
>282 |
Desorption is carried out at high pH conditions |
162 |
AC modified with micro-sized goethite |
4 |
Cr removal efficiency of up to 75.1% |
Washed with 0.1 M HCl solution |
174 |
AC of Cornulaca monacantha stem |
5 |
89% adsorption efficiency |
Desorption was carried out with 0.01 mol L−1 NaOH |
173 |
ZnO tetrapods and AC based hybrid composite |
3 |
Cr removal efficiency > 90% |
Washed with 0.1 mol L−1 NaOH, kept at 120 rotations per minute (rpm) for 8 h and dried in vacuum at 60 °C |
65 |
AC modified with micro-sized goethite using a facile impregnation method |
4 |
Cr removal efficiency > 75% |
Washed with HCl solutions (0.1 and 1 mol L−1) |
174 |
Nanocomposite adsorbent |
5 |
>80% adsorption efficiency |
Washed with deionized water and then dried at 100 °C in an oven for 3 h |
64 |
Crosslinked chitosan |
5 |
269 |
Washed with 1 mol L−1 NaOH |
176 |
Tetraethylenepentamine functionalized alginate beads |
5 |
30 (% removal exceeded 80%) |
100 mL of NaCl (1 M)/ethanol (98%) solution mixture with continuous shaking at 250 rpm for 1 h at 25 °C |
177 |
Chitosan grafted GO nanocomposite |
10 |
The percentage removal decreased marginally from 96% to 82% |
Washed with 1 mol L−1 NaOH |
178 |
3.8. Comparison and contrast of adsorbents based on adsorption capacities for chromium(VI)
Usually, the performance of adsorbents is judged by their adsorption capacity values (Table 2). However, in our opinion, this comparison is not a true reflection or determinant of adsorption performance. This is particularly so because of the complexities involved such as the type of adsorbents, functional groups type and density, and adsorption conditions employed including dosage, pH, and initial concentration. In addition to this, there are other variables such as batch versus column adsorption as well as shaking versus stirring; all these give different adsorption capacities. Table 2 depicts the different adsorption capacities for Cr(VI) reported by various researchers (selected randomly) and the diverse conditions adopted. Normally the loading (mg g−1) increases with an increase in the initial concentration of the adsorbate, and the generally accepted explanation is that as the adsorbate initial concentration increases the number of moles of adsorbates in solution surpasses the active sites resulting in saturation and maximum adsorption. Using Fig. 4 to illustrate this point, it can be observed that at a higher initial concentration (300 mg L−1) the percentage removal of the pollutant is minimal (20%) while the loading capacity is at maximum. Of the initial 300 mg L−1, only 60 mg L−1 was removed (20%) and 240 mg L−1 concentration of Cr(VI) was left in solution. Reporting adsorption performance in adsorption capacity somehow hides the truth about the ability of adsorbents. Nonetheless, the adsorption capacity values reported in Table 2 show that researchers are now able to develop adsorbents with very high adsorption capacities, and it is good progress indeed. However, biomass adsorption capacities are still low compared to the rest of the adsorbents, and that need improvement if the low-cost effectiveness of adsorbents is to be advanced. More adsorbents of the type adsorbent1@adsorbent2@adsorbent3 need to be developed using biomass and possibly the type of adsorbent1@adsorbent2@adsorbent3@adsorbent4. However, with such complex and expensive chemical usage in adsorbent preparation, would the adsorption method be still advocated as cheap and simple? The authors do not think so.
Table 2 Comparison of adsorption capacities from various adsorbents for Cr(VI)
Adsorbents |
Adsorption capacity (mg g−1) |
Initial concentration (mg L−1) |
Dosage (g L−1) |
Optimum pH |
Reference |
Aluminium–lanthanum mixed oxyhydroxide (ALMOH) |
49.8 |
200 |
2 |
4.5 |
15 |
Chitosan/aluminum–lanthanum mixed oxyhydroxide (CSALMOH) |
78.9 |
200 |
2 |
4.5 |
15 |
Ionic solid impregnated phosphate chitosan |
266.67 |
1200 |
4 |
3 |
16 |
PAN–CNT/TiO2–NH2 |
714.27 |
300 |
— |
2 |
30 |
Amberlite XAD (synthetic polymer) |
196 |
50 |
5 |
1.5 |
92 |
Cr(VI)-IIP anchored on magnetic multi-walled carbon nanotubes |
56.1 |
400 |
1 |
3 |
94 |
IIP 4-vinyl pyridine and 2-hydroxyethyl methacrylate co-monomers |
311.95 |
1000 |
0.4 |
2 |
101 |
H3PO4 acid-activated (Acticarbone) carbons |
186 |
200 |
0.6 |
3 |
123 |
KOH-activated activated (Acticarbone) carbons |
315 |
200 |
0.6 |
3 |
123 |
MS@APTES@PNIPAm |
123.8 |
31 |
0.2 |
2.5 |
147 |
Mesoporous silica embedded with magnetite nanoparticles |
50.51 |
50 |
1.4 |
2 |
148 |
Magnetic mesoporous titanium dioxide–graphene oxide core–shell microspheres |
117.94 |
— |
— |
6.5 |
156 |
Resorcinol–melamine–formaldehyde (RMF) anchored on a nanocrystalline cellulose |
463 |
400 |
0.2 |
3 |
157 |
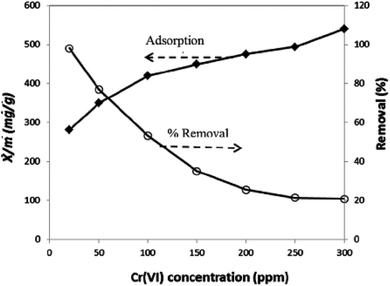 |
| Fig. 4 Demonstration of the effect of initial concentration on percent removal and adsorption capacity.160 | |
4.6. Other parameters influencing adsorption capacity
As alluded to in the preceding paragraph, adsorption capacities should never be read alone to infer on the performance of an adsorbent. Several other parameters influence the adsorption capacities. These include the solution pH, equilibration time, temperature of the solution, co-existing ions, and adsorbent dosage concentration. Solution pH affects both the adsorbate and adsorbent chemistry, i.e., the degree of adsorbent functional groups ionization and speciation of chromium.33 Numerous studies reported on the electrostatic attraction forces between positively charged adsorbent surface groups and anionic Cr(VI) species.21,23,35,54,65 This is particularly more common because Cr(VI) exists as oxyanions (HCrO4−, Cr2O72− and CrO42−) in aqueous solution. Therefore, conditions conducive for negative–positive attraction will favour the electrostatic attraction. Positively charged adsorbent surface groups are produced by protonation or quaternization. Adsorbents relying on the electrostatic attraction usually give high removal efficiencies at low pH values with adsorption capacities decreasing as the pH is increased due to deprotonation and presence of OH− ions competing for adsorption sites with Cr(VI) ions. Initial concentration also affects the type of species present and the sorption capabilities. Thus, speciation is affected by both the concentration and pH. The percentage removal decreases with an increase in solute concentration while the adsorption capacity decreases. Adsorption rate also plays a critical role in sorption processes. Hence, the influence of contact time on the adsorption capacity is always studied for all adsorbents to infer on reaction mechanism governing the process. Most adsorbents share a similar trend, where initially, there is a rapid uptake of Cr(VI) due to the abundance of adsorption sites versus the fixed moles of Cr(VI) in solution, boundary layer adsorption. The second stage is usually characterized by slow adsorption, termed as the diffusion into internal pores. The third and last stage is the saturation of adsorption sites (equilibrium).21,23,33,35,54
Investigation of the effect of co-ions gives an indication of how the prepared adsorbent will perform in real-world samples containing other pollutants that may interfere with Cr(VI) binding to the adsorbent. Cr(VI) behaves as an anion in aqueous media, therefore, investigating its preference by adsorbents using anionic species like F−, SO42−, PO43−, Cl−, and HCO3− (ref. 15 and 23) is justifiable. Nonetheless, the influence of mixtures of anions and cations22,36,56 as well as cations only62 have been studied. The effect of temperature on the adsorption capacities is studied to infer on the spontaneity of the reaction. Both endothermic23,36,63,89,158,167,169 and exothermic15,30 processes for the uptake of Cr(VI) have been reported. The influence of temperature on adsorption is one of the gray areas of the process that are not well researched and explicitly explained. By definition, adsorption is an exothermic process but numerous researchers have justified their endothermic nature findings using scientifically acceptable explanation. With regards to adsorbent dosage concentration, adsorption capacity decreases with increase in sorbent dose. The plausible explanation is that the increase in adsorption performance as the concentration dosage is increased is credited to the increased active adsorption sites as the mass of sorbent is increased. In addition to the aforementioned factors, the presence of surfactants and ionic salts affect the adsorption capacities but there has been very little evidence on their investigation.179
4.7. Most recent applications, challenges and future perspectives
Removal of Cr(VI) from aqueous solutions based on adsorption methods is currently the area of intense research. Recent work include removal of Cr(VI) from water samples using m-phenylenediamine-modified polypyrrole,180 sweet lime peel powder,111 chitosan-based hydrogel,181 nanocarbon bridged nano magnetite network,182 magnetic biochar composite,183 carbon spheres (magnetic ceramsite coated by functionalized nano carbon spheres184 and hollow carbon spheres185), modified sponge iron particles186 and metal–organic framework.187 Recent work has provided evidence that the modified and functionalized adsorbents perform better than their natural forms. For illustration, the equilibrium adsorption capacity achieved for Cr(VI) in water using magnetic biochar was 8.35 mg g−1,183 whereas, the amino-functionalized magnetic biochar yielded the maximum adsorption capacity of 142.86 mg g−1.188
Some adsorbents recently reported in the literature are waste materials which are regarded as low-cost sorbents. Typical examples include eggshell powder,189 orange peels,190 coffee ground and mixed waste tea,191–193 human hair waste194 as well as rock wool.4 The application of these adsorbents in water purification is advantageous in green chemistry perspectives as these are prevented from invading the environment. The current concern with their usage in Cr(VI) removal, which can be investigated in the future arise from their application in deionized water. Therefore, the applicability of the number of reported adsorbents in real samples such as industrial wastewater, seawater, where high concentrations of chromium are expected is not well understood. Another challenge is the inability to re-use some adsorbents. For example, Kera et al.180 demonstrated that the removal of Cr(VI) using m-phenylenediamine-modified polypyrrole adsorbent decreased by approximately 30% after the third adsorption/desorption cycle. This implies that there is a chance that the highly contaminated adsorbent used for Cr(VI) removal from water could be disposed into the environment. Regarding the re-usability of adsorbents, Samuel et al.64 observed a significant decrease in the adsorption/desorption of Cr(VI) after each cycle using a nanocomposite adsorbent material synthesized from chitosan, GO and metal–organic framework. Even though these adsorbents could be useful in water decontamination, their single-use might result in their excessive disposal into the environment. The stability of many adsorbents reported in the literature for Cr(VI) adsorption is not known. The stability of adsorbents stored for an extended period of time has only been investigated in a few studies. For example, Sharma et al.65 investigated the stability of ZnO-tetrapods and AC based hybrid composite by performing adsorption after six months of adsorbent synthesis. The removal efficiency was used as a measure of stability.
4.8. Concluding remarks
The aim of this article was to review some of the major adsorbents used for Cr(VI) confiscation from aqueous solution to provide a one-stop resource material for researchers working on the adsorptive removal of Cr(VI). The classification of adsorbents, characteristics, and their development history together with the toxicity of chromium, its sources, mechanism of removal and recent trends in adsorbent synthesis and preparation were discussed at length. The advancement in the interpretation of the Cr(VI) removal mechanism was outlined, and the main challenge identified was accounting for the fate of the as-reduced Cr(III). Irrespective of the type of adsorbent used, reporting only on adsorption of Cr(VI) through electrostatic attractions is not enough when it is known that Cr(VI) is a strong oxidant susceptible to undergo transformation to Cr(III) upon accepting electrons. Heteroatoms like O, N, and S responsible for the donation of electrons are present in almost every adsorbent. Hence, direct or indirect reduction of Cr(VI) to Cr(III) during adsorption cannot be ruled out. Disproving the presence or absence of Cr(III) in solution or adsorbent surface following Cr(VI) uptake is paramount. The qualitative determination of Cr(III) presence by the greenish-colour of adsorbents after adsorption is not enough proof of reduction on its own. It needs to be supplemented by analytical instruments. Characterization of the adsorbent surface by XPS after adsorption and analysis of solution with atomic absorption spectroscopy does indicate the presence or lack of Cr(III) after adsorption. The removal mechanism is also affected by surface chemistry and physical properties of adsorbents. The characteristics of high surface area, availability, porosity, and cost-effectiveness are considered when developing adsorbents for pollutant removal. AC, GO, nanoparticle, and silica are known for their high surface area values, while biomass exhibit low surface area but abundant functional groups. Functionalization and merging of adsorbents into composite materials produce superior properties. The recent literature shows that most of these adsorbents are no longer used in their pristine form, but several modifications are carried out. Functionalization could be achieved by cross-linking and/or grafting. Better performances have been reported where there were three levels of modifications in an adsorbent1@adsorbent2@adsorbent3 system of layers have been used. Some of these layers not only consisted of ligands but also composite materials from different adsorbents, e.g., silica@nanoparticle@polymer. With such modifications, good (78.90, 123, 196 and 198 mg g−1) and excellent (315 and 714.27 mg g−1) adsorption capacities have been achieved. In the case of PAN@CNT@TiO2@NH2 composite (714.27 mg g−1) the high surface area property of TiO2 was complemented by the inclusion of NH2 groups for Cr(VI) adsorption. It should be taken into cognizance that adsorption capacity values need to be viewed with other parameters influencing adsorption. For instance, some of these adsorbents reached equilibrium after 14 h, while others only needed 30 min. Longer equilibration times could not be ideal for the industrial application of adsorbents. Also, most experiments giving the high values of adsorption capacities were conducted with deionized water. But, it was evident that for much more improved adsorption performance, modification of the structure and functional groups of adsorbents should be performed concurrently. This was demonstrated in the synthesis of hierarchical structure that exhibited high adsorption capacities (463 mg g−1). Adsorbents representing triple or double grafting of the type adsorbent1@adsorbent2@adsorbent3@adsorbent4 composite could also be the future, but adsorption is advocated as a simple and low-cost method. The cost could be offset by the performance (714.27 mg g−1) as demonstrated in the PAN@CNT@TiO2@NH2 composite. Therefore, complex adsorbent preparation should be balanced by their superior adsorption performances; otherwise, it will be fruitless to have a complex adsorbent with poor adsorption capacity.
Conflicts of interest
There are no conflicts to declare.
Acknowledgements
Funding from the National Research Foundation of South Africa under Thuthuka (Grant number: TTK160510164648) and Competitive Program for Unrated Researchers (Grant number: 114415) grants awarded to Dr, VE Pakade and LM Madikizela, respectively, as well as Vaal University of Technology is gratefully appreciated.
References
- P. Miretzky and A. F. Cirelli, Cr(VI) and Cr(III) removal from aqueous solution by raw and modified lignocellulosic materials: a review, J. Hazard. Mater., 2010, 180, 1–19, DOI:10.1016/j.jhazmat.2010.04.060.
- D. Pradhan, L. B. Sukla, M. Sawyer and P. K. S. M. Rahman, Recent bioreduction of hexavalent chromium in wastewater treatment: a review, J. Ind. Eng. Chem., 2017, 55, 1–20, DOI:10.1016/j.jiec.2017.06.040.
- S. Mortazavian, H. An, D. Chun and J. Moon, Activated carbon impregnated by zero-valent iron nanoparticles (AC/nZVI) optimized for simultaneous adsorption and reduction of aqueous hexavalent chromium: Material characterizations and kinetic studies, Chem. Eng. J., 2018, 353, 781–795, DOI:10.1016/j.cej.2018.07.170.
- L. Zhou, R. Li, G. Zhang, D. Wang, D. Cai and Z. Wu, Zero-valent iron nanoparticles supported by functionalized waste rock wool for efficient removal of hexavalent chromium, Chem. Eng. J., 2018, 339, 85–96, DOI:10.1016/j.cej.2018.01.132.
- M. N. Sahmoune, K. Louhab and A. Boukhiar, Advanced biosorbents materials for removal of chromium from water and wastewaters, Environ. Prog. Sustainable Energy, 2010, 3, 284–293, DOI:10.1002/ep.
- C. Raji and T. S. Anirudhan, Batch Cr(VI) removal by polyacrylamide-grafted sawdust: kinetics and thermodynamics, Water Res., 1998, 32, 3772–3780, DOI:10.1016/s0043-1354(98)00150-x.
- C. H. Weng, J. H. Wang and C. P. Huang, Adsorption of Cr(VI) onto TiO2 from dilute aqueous solutions, Water Sci. Technol., 1997, 35, 55–62, DOI:10.1016/s0273-1223(97)00114-5.
- D. E. Kimbrough, Y. Cohen, A. M. Winer, L. Creelman and C. Mabuni, A critical assessment of chromium in the environment, Crit. Rev. Environ. Sci. Technol., 1999, 29, 1–46, DOI:10.1080/10643389991259164.
- M. K. Aroua, F. M. Zuki and N. M. Sulaiman, Removal of chromium ions from aqueous solutions by polymer-enhanced ultrafiltration, J. Hazard. Mater., 2007, 147, 752–758, DOI:10.1016/j.jhazmat.2007.01.120.
- T. N. De Castro Dantas, A. A. D. Neto, M. C. P. D. A. Moura, E. L. B. Neto and E. De Paiva Telemaco, Chromium adsorption by chitosan impregnated with microemulsion, Langmuir, 2001, 17, 4256–4260, DOI:10.1021/la001124s.
- M. Costa, Potential hazards of hexavalent chromate in our drinking water, Toxicol. Appl. Pharmacol., 2003, 188, 1–5, DOI:10.1016/0041-008x(03)00011-5.
- A. Zhitkovich, Importance of chromium-DNA adducts in mutagenicity and toxicity of chromium(VI), Chem. Res. Toxicol., 2005, 18, 3–11, DOI:10.1021/tx049774+.
- Z. Feng, W. Hu, W. N. Rom, M. Costa and M. S. Tang, Chromium(VI) exposure enhances polycyclic aromatic hydrocarbon-DNA binding at the p53 gene in human lung cells, Carcinogenesis, 2003, 24, 771–778, DOI:10.1093/carcin/bgg012.
- J. Wang, K. Zhang and L. Zhao, Sono-assisted synthesis of nanostructured polyaniline for adsorption of aqueous Cr(VI): effect of protonic acids, Chem. Eng. J., 2014, 239, 123–131, DOI:10.1016/j.cej.2013.11.006.
- J. Preethi, S. M. Prabhu and S. Meenakshi, Effective adsorption of hexavalent chromium using biopolymer assisted oxyhydroxide materials from aqueous solution, React. Funct. Polym., 2017, 117, 16–24, DOI:10.1016/j.reactfunctpolym.2017.05.006.
- S. S. Kahu, A. Shekhawat, D. Saravanan and R. M. Jugade, Two fold modified chitosan for enhanced adsorption of hexavalent chromium from simulated wastewater and industrial effluents, Carbohydr. Polym., 2016, 146, 264–273, DOI:10.1016/j.carbpol.2016.03.041.
- L. Lin, X. Xu, C. Papelis, T. Y. Cath and P. Xu, Sorption of metals and metalloids from reverse osmosis concentrate on drinking water treatment solids, Sep. Purif. Technol., 2014, 134, 37–45, DOI:10.1016/j.seppur.2014.07.008.
- G. Qin, M. J. Mcguire, N. K. Blute, C. Seidel and L. Fong, Hexavalent chromium removal by reduction with ferrous sulfate, coagulation, and filtration: a pilot-scale study, Environ. Sci. Technol., 2005, 39, 6321–6327, DOI:10.1021/es050486p.
- E. Rodrigues, O. Almeida, H. Brasil, D. Moraes and M. A. L. Reis, Adsorption of chromium(VI) on hydrotalcite-hydroxyapatite material doped with carbon nanotubes: equilibrium, kinetic and thermodynamic study, Appl. Clay Sci., 2019, 172, 57–64, DOI:10.1016/j.clay.2019.02.018.
- A. Mittal, L. Krishnan and V. K. Gupta, Removal and recovery of malachite green from wastewater using an agricultural waste material, de-oiled soya, Sep. Purif. Technol., 2005, 43, 125–133, DOI:10.1016/j.seppur.2004.10.010.
- M. R. Gopal Reddi, T. Gomathi, M. Saranya and P. N. Sudha, Adsorption and kinetic studies on the removal of chromium and copper onto chitosan-g-maleic anhydride-g-ethylene dimethacrylate, Int. J. Biol. Macromol., 2017, 104, 1578–1585, DOI:10.1016/j.ijbiomac.2017.01.142.
- N. Li, Y. Tian, J. Zhao, J. Zhang, J. Zhang, W. Zuo and Y. Ding, Efficient removal of chromium from water by Mn3O4@ZnO/Mn3O4 composite under simulated sunlight irradiation: synergy of photocatalytic reduction and adsorption, Appl. Catal., B, 2017, 214, 126–136, DOI:10.1016/j.apcatb.2017.05.041.
- G. Ren, X. Wang, P. Huang, B. Zhong, Z. Zhang, L. Yang and X. Yang, Chromium(VI) adsorption from wastewater using porous magnetite nanoparticles prepared from titanium residue by a novel solid-phase reduction method, Sci. Total Environ., 2017, 607–608, 900–910, DOI:10.1016/j.scitotenv.2017.06.103.
- J. Wei, C. Tu, G. Yuan, D. Bi, L. Xiao, B. K. G. Theng, H. Wang and Y. Sik, Carbon-coated montmorillonite nanocomposite for the removal of chromium(VI) from aqueous solutions, J. Hazard. Mater., 2019, 368, 541–549, DOI:10.1016/j.jhazmat.2019.01.080.
- Z. Yuan, X. Cheng, L. Zhong, R. Wu and Y. Zheng, Preparation, characterization and performance of an electrospun carbon nanofiber mat applied in hexavalent chromium removal from aqueous solution, J. Environ. Sci., 2018, 77, 75–84, DOI:10.1016/j.jes.2018.06.016.
- B. Saha and C. Orvig, Biosorbents for hexavalent chromium elimination from industrial and municipal effluents, Coord. Chem. Rev., 2010, 254, 2959–2972, DOI:10.1016/j.ccr.2010.06.005.
- D. Park, C. K. Ahn, Y. M. Kim, Y. S. Yun and J. M. Park, Enhanced abiotic reduction of Cr(VI) in a soil slurry system by natural biomaterial addition, J. Hazard. Mater., 2008, 160, 422–427, DOI:10.1016/j.jhazmat.2008.03.044.
- H. Panda, N. Tiadi, M. Mohanty and C. R. Mohanty, Studies on adsorption behavior of an industrial waste for removal of chromium from aqueous solution, S. Afr. J. Chem. Eng., 2017, 23, 132–138, DOI:10.1016/j.sajce.2017.05.002.
- L. Hlungwane, E. L. Viljoen and V. E. Pakade, Macadamia nutshells-derived activated carbon and attapulgite clay combination for synergistic removal of Cr(VI) and Cr(III), Adsorpt. Sci. Technol., 2018, 36, 713–731, DOI:10.1177/0263617417719552.
- A. Mohamed, W. S. Nasser, T. A. Osman, M. S. Toprak, M. Muhammed and A. Uheida, Removal of chromium(VI) from aqueous solutions using surface modified composite nanofibers, J. Colloid Interface Sci., 2017, 505, 682–691, DOI:10.1016/j.jcis.2017.06.066.
- A. Nakajima and Y. Baba, Mechanism of hexavalent chromium adsorption by persimmon tannin gel, Water Res., 2004, 38, 2859–2864, DOI:10.1080/00344893.2014.911772.
- M. Gheju, Hexavalent chromium reduction with zero-valent iron (ZVI) in aquatic systems, 2011, DOI:10.1007/s11270-011-0812-y.
- I. A. Bhatti, N. Ahmad, N. Iqbal, M. Zahid and M. Iqbal, Chromium adsorption using waste tire and conditions optimization by response surface methodology, J. Environ. Chem. Eng., 2017, 5, 2740–2751, DOI:10.1016/j.jece.2017.04.051.
- A. B. Albadarin, S. Solomon, T. A. Kurniawan, C. Mangwandi and G. Walker, Single, simultaneous and consecutive biosorption of Cr(VI) and Orange II onto chemically modified masau stones, J. Environ. Manage., 2017, 204, 365–374, DOI:10.1016/j.jenvman.2017.08.042.
- M. Omidvar Borna, M. Pirsaheb, M. Vosoughi Niri, R. Khosravi Mashizie, B. Kakavandi, M. R. Zare and A. Asadi, Batch and column studies for the adsorption of chromium(VI) on low-cost Hibiscus Cannabinus kenaf, a green adsorbent, J. Taiwan Inst. Chem. Eng., 2016, 68, 80–89, DOI:10.1016/j.jtice.2016.09.022.
- S. Periyasamy, V. Gopalakannan and N. Viswanathan, Fabrication of magnetic particles imprinted cellulose based biocomposites for chromium(VI) removal, Carbohydr. Polym., 2017, 174, 352–359, DOI:10.1016/j.carbpol.2017.06.029.
- M. K. Dinker and P. S. Kulkarni, Recent advances in silica-based materials for the removal of hexavalent chromium: a review, J. Chem. Eng. Data, 2015, 60, 2521–2540, DOI:10.1021/acs.jced.5b00292.
- H. N. M. Ekramul Mahmud, A. K. Obidul Huq and R. B. Yahya, The removal of heavy metal ions from wastewater/aqueous solution using polypyrrole-based adsorbents: a review, RSC Adv., 2016, 6, 14778–14791, 10.1039/c5ra24358k.
- A. A. Ihsanullah, A. M. Al-Amer, T. Laoui, M. J. Al-Marri, M. S. Nasser, M. Khraisheh and M. A. Atieh, Heavy metal removal from aqueous solution by advanced carbon nanotubes: critical review of adsorption applications, Sep. Purif. Technol., 2016, 157, 141–161, DOI:10.1016/j.seppur.2015.11.039.
- M. K. Uddin, A review on the adsorption of heavy metals by clay minerals, with special focus on the past decade, Chem. Eng. J., 2017, 308, 438–462, DOI:10.1016/j.cej.2016.09.029.
- J. Xu, Z. Cao, Y. Zhang, Z. Yuan, Z. Lou, X. Xu and X. Wang, A review of functionalized carbon nanotubes and graphene for heavy metal adsorption from water: Preparation, application, and mechanism, Chemosphere, 2018, 195, 351–364, DOI:10.1016/j.chemosphere.2017.12.061.
- J. C. Almeida, C. E. D. Cardoso, D. S. Tavares, R. Freitas, T. Trindade, C. Vale and E. Pereira, Chromium removal from contaminated waters using nanomaterials – a review, Trends Anal. Chem., 2019, 118, 277–291, DOI:10.1016/j.trac.2019.05.005.
- U. O. Aigbe, R. Das, W. H. Ho, V. Srinivasu and A. Maity, A novel method for removal of Cr(VI) using polypyrrole magnetic nanocomposite in the presence of unsteady magnetic fields, Sep. Purif. Technol., 2018, 194, 377–387, DOI:10.1016/j.seppur.2017.11.057.
- S. Barnie, J. Zhang, H. Wang, H. Yin and H. Chen, The influence of pH, co-existing ions, ionic strength, and temperature on the adsorption and reduction of hexavalent chromium by undissolved humic acid, Chemosphere, 2018, 212, 209–218, DOI:10.1016/j.chemosphere.2018.08.067.
- P. Janik, B. Zawisza, E. Talik and R. Sitko, Selective adsorption and determination of hexavalent chromium ions using graphene oxide modified with amino silanes, Microchim. Acta, 2018, 185, 1–8, DOI:10.1007/s00604-017-2640-2.
- N. H. Mthombeni, S. Mbakop, S. C. Ray, T. Leswifi, A. Ochieng and M. S. Onyango, Highly efficient removal of chromium(VI) through adsorption and reduction: a column dynamic study using magnetized natural zeolite-polypyrrole composite, J. Environ. Chem. Eng., 2018, 6, 4008–4017, DOI:10.1016/j.jece.2018.05.038.
- N. K. Mondal, B. Sambrita and B. Das, Decontamination and optimization study of hexavalent chromium on modified chicken feather using response surface methodology, Appl. Water Sci., 2019, 9, 1–15, DOI:10.1007/s13201-019-0930-z.
- S. Mousavi, F. Shahraki, M. Aliabadi, A. Haji, F. Deuber and C. Adlhart, Surface enriched nano fiber mats for efficient adsorption of Cr(VI) inspired by nature, J. Environ. Chem. Eng., 2019, 7, 102817, DOI:10.1016/j.jece.2018.102817.
- L. Qian, S. Liu, W. Zhang, Y. Chen, D. Ouyang, L. Han, J. Yan and M. Chen, Enhanced reduction and adsorption of hexavalent chromium by palladium and silicon rich biochar supported nanoscale zero-valent iron, J. Colloid Interface Sci., 2019, 533, 428–436, DOI:10.1016/j.jcis.2018.08.075.
- A. Sarkar, A. Ranjan and B. Paul, Synthesis, characterization and application of surface – modified biochar synthesized from rice husk, an agro-industrial waste for the removal of hexavalent chromium from drinking water at near-neutral pH, Clean Technol. Environ. Policy, 2019, 21, 447–462, DOI:10.1007/s10098-018-1649-5.
- H. Kang, R. Liu and Y. Huang, Graft modification of cellulose: Methods, properties and applications, Polymer, 2015, 70, A1–A16, DOI:10.1016/j.polymer.2015.05.041.
- R. Kumar, R. K. Sharma and A. P. Singh, Cellulose based grafted biosorbents – journey from lignocellulose biomass to toxic metal ions sorption applications – a review, J. Mol. Liq., 2017, 232, 62–93, DOI:10.1016/j.molliq.2017.02.050.
- T. Heinze, Cellulose: Structure and Properties, Adv. Polym. Sci., 2015, 271, 1–52, DOI:10.1007/12.
- T. Velempini, K. Pillay, X. Y. Mbianda and O. A. Arotiba, Epichlorohydrin crosslinked carboxymethyl cellulose-ethylenediamine imprinted polymer for the selective uptake of Cr(VI), Int. J. Biol. Macromol., 2017, 101, 837–844, DOI:10.1016/j.ijbiomac.2017.03.048.
- S. Periyasamy, P. Manivasakan and C. Jeyaprabha, Fabrication of nano-graphene oxide assisted hydrotalcite/chitosan biocomposite: an efficient adsorbent for chromium removal from water, Int. J. Biol. Macromol., 2019, 132, 1068–1078, DOI:10.1016/j.ijbiomac.2019.03.232.
- Y. Zhou, Q. Jin, T. Zhu and Y. Akama, Adsorption of chromium(VI) from aqueous solutions by cellulose modified with β-CD and quaternary ammonium groups, J. Hazard. Mater., 2011, 187, 303–310 CrossRef CAS PubMed.
- M. Kaya, O. Seyyar, T. Baran and T. Turkes, Bat guano as new and attractive chitin and chitosan source, Front. Zool., 2014, 11, 1–10 CrossRef PubMed.
- R. Bhatt, B. Sreedhar and P. Padmaja, Chitosan supramolecularly cross linked with trimesic acid – facile synthesis, characterization and evaluation of adsorption potential for chromium(VI), Int. J. Biol. Macromol., 2017, 104, 1254–1266, DOI:10.1016/j.ijbiomac.2017.06.067.
- S. Hena, Removal of chromium hexavalent ion from aqueous solutions using biopolymer chitosan coated with poly-3-methyl thiophene polymer, J. Hazard. Mater., 2010, 181, 474–479, DOI:10.1016/j.jhazmat.2010.05.037.
- J. B. Dima, C. Sequeiros and N. E. Zaritzky, Hexavalent chromium removal in contaminated water using reticulated chitosan micro/nanoparticles from seafood processing wastes, Chemosphere, 2015, 141, 100–111, DOI:10.1016/j.chemosphere.2015.06.030.
- S. Gokila, T. Gomathi, P. N. Sudha and S. Anil, Removal of the heavy metal ion chromium(VI) using chitosan and alginate nanocomposites, Int. J. Biol. Macromol., 2017, 104, 1459–1468, DOI:10.1016/j.ijbiomac.2017.05.117.
- J. H. Chen, H. T. Xing, H. X. Guo, W. Weng, S. R. Hu, S. Li, Y. H. Huang, S. Xue and S. zhen Bo, Investigation on the adsorption properties of Cr(VI) ions on a novel graphene oxide (GO) based composite adsorbent, J. Mater. Chem. A, 2014, 2, 12561–12570, 10.1039/c4ta02004a.
- N. Nasseh, L. Taghavi, B. Barikbin and A. R. Harifi-Mood, The removal of Cr(VI) from aqueous solution by almond green hull waste material: kinetic and equilibrium studies, J. Water Reuse Desalin., 2017, 7, 449–460, DOI:10.2166/wrd.2016.047.
- M. S. Samuel, V. Subramaniyan, J. Bhattacharya, C. Parthiban, S. Chand and N. D. P. Singh, A GO–CS@MOF [Zn(BDC)(DMF)] material for the adsorption of chromium(VI) ions from aqueous solution, Composites, Part B, 2018, 152, 116–125, DOI:10.1016/j.compositesb.2018.06.034.
- M. Sharma, M. Joshi, S. Nigam, S. Shree, D. K. Avasthi, R. Adelung, S. K. Srivastava and Y. Kumar Mishra, ZnO tetrapods and activated carbon based hybrid composite: adsorbents for enhanced decontamination of hexavalent chromium from aqueous solution, Chem. Eng. J., 2019, 358, 540–551, DOI:10.1016/j.cej.2018.10.031.
- M. H. Fatehi, J. Shayegan, M. Zabihi and I. Goodarznia, Functionalized magnetic nanoparticles supported on activated carbon for adsorption of Pb(II) and Cr(VI) ions from saline solutions, J. Environ. Chem. Eng., 2017, 5, 1754–1762 CrossRef CAS.
- Q. Zhu, L. Zhao, D. Sheng, Y. Chen, X. Hu and H. Lian, Speciation analysis of chromium by carboxylic group functionalized mesoporous silica with inductively coupled plasma mass spectrometry, Talanta, 2019, 195, 173–180, DOI:10.1016/j.talanta.2018.11.043.
- Q. Yuan, N. Li, Y. Chi, W. Geng, W. Yan, Y. Zhao, X. Li and B. Dong, Effect of large pore size of multifunctional mesoporous microsphere on removal of heavy metal ions, J. Hazard. Mater., 2013, 254–255, 157–165, DOI:10.1016/j.jhazmat.2013.03.035.
- J. L. Blin and M. Impéror-Clerc, Mechanism of self-assembly in the synthesis of silica mesoporous materials: in situ studies by X-ray and neutron scattering, Chem. Soc. Rev., 2013, 42, 4071–4082, 10.1039/c2cs35362h.
- M. Karimi, A. Shojaei, A. Nematollahzadeh and M. J. Abdekhodaie, Column study of Cr(VI) adsorption onto modified silica-polyacrylamide microspheres composite, Chem. Eng. J., 2012, 210, 280–288, DOI:10.1016/j.cej.2012.08.046.
- D. Mohan and C. U. Pittman, Activated carbons and low cost adsorbents for remediation of tri- and hexavalent chromium from water, J. Hazard. Mater., 2006, 137, 762–811, DOI:10.1016/j.jhazmat.2006.06.060.
- R. Yang, Y. Wang, M. Li and Y. Hong, A new carbon/ferrous sulfide/iron composite prepared by an in situ carbonization reduction method from hemp (cannabis sativa L.) stems and its Cr(VI) removal ability, ACS Sustainable Chem. Eng., 2014, 2, 1270–1279, DOI:10.1021/sc500092z.
- G. R. R. Bernardo, R. M. J. Rene and A. D. l. T. Alfaro-De la, Chromium(III) uptake by agro-waste biosorbents: chemical characterization, sorption–desorption studies, and mechanism, J. Hazard. Mater., 2009, 170, 845–854, DOI:10.1016/j.jhazmat.2009.05.046.
- M. Suwalsky, R. Castro, F. Villena and C. P. Sotomayor, Cr(III) exerts stronger structural effects than Cr(VI) on the human erythrocyte membrane and molecular models, J. Inorg. Biochem., 2008, 102, 842–849, DOI:10.1016/j.jinorgbio.2007.11.020.
- L. E. Wu, A. Levina, H. H. Harris, Z. Cai, B. Lai, S. Vogt, D. E. James and P. A. Lay, Carcinogenic chromium(VI) compounds formed by intracellular oxidation of chromium(III) dietary supplements by adipocytes, Angew. Chem., Int. Ed., 2016, 55, 1742–1745, DOI:10.1002/anie.201509065.
- H. A. Headlam and P. A. Lay, Spectroscopic characterization of genotoxic chromium(V) peptide complexes: oxidation of chromium(III) triglycine, tetraglycine and pentaglycine complexes, J. Inorg. Biochem., 2016, 162, 227–237, DOI:10.1016/j.jinorgbio.2016.06.015.
- D. R. Lindsay, K. J. Farley and R. F. Carbonaro, Oxidation of CrIII to CrVI during chlorination of drinking water, J. Environ. Monit., 2012, 14, 1789–1797, 10.1039/c2em00012a.
- S. E. Gomez-Gonzalez, G. G. Carbajal-Arizaga, R. Manriquez-Gonzalez, W. De La Cruz-Hernandez and S. Gomez-Salazar, Trivalent chromium removal from aqueous solutions by a sol–gel synthesized silica adsorbent functionalized with sulphonic acid groups, Mater. Res. Bull., 2014, 59, 394–404, DOI:10.1016/j.materresbull.2014.07.035.
- E. Agrafioti, G. Bouras, D. Kalderis and E. Diamadopoulos, Biochar production by sewage sludge pyrolysis, J. Anal. Appl. Pyrolysis, 2013, 101, 72–78, DOI:10.1016/j.jaap.2013.02.010.
- S. C. McBain, H. H. Yiu and J. Dobson, Magnetic nanoparticles for gene and drug delivery. TL - 3, Int. J. Nanomed., 2007, 3, 169–180, DOI:10.1002/ddr.
- C. Cervantes and J. Campos-García, Reduction and Efflux of Chromate by Bacteria, Microbiol Monogr, 2007. vol. 6, pp. 234–241, DOI:10.1007/7171.
- S. S. Wise, A. L. Holmes, L. Liou, R. M. Adam and J. P. Wise, Hexavalent chromium induces chromosome instability in human urothelial cells, Toxicol. Appl. Pharmacol., 2016, 296, 54–60, DOI:10.1016/j.taap.2016.02.015.
- W. Liu, F. Chaspoul, C. Botta, M. De Méo and P. Gallice, Bioenergetics and DNA alteration of normal human fibroblasts by hexavalent chromium, Environ. Toxicol. Pharmacol., 2010, 29, 58–63, DOI:10.1016/j.etap.2009.10.001.
- R. Suthanthararajan, E. Ravindranath, K. Chitra, B. Umamaheswari, T. Ramesh and S. Rajamani, Membrane application for recovery and reuse of water from treated tannery wastewater, Desalination, 2004, 164, 151–156, DOI:10.1016/s0011-9164(04)00174-2.
- E. Ranieri, U. Fratino, D. Petruzzelli and A. C. Borges, A comparison between Phragmites australis and Helianthus annuus in chromium phytoextraction, Water, Air, Soil Pollut., 2013, 224 DOI:10.1007/s11270-013-1465-9.
- T. Basegio, A. P. Beck Leão, A. M. Bernardes and C. P. Bergmann, Vitrification: an alternative to minimize environmental impact caused by leather industry wastes, J. Hazard. Mater., 2009, 165, 604–611, DOI:10.1016/j.jhazmat.2008.10.045.
- C. Viti and L. Giovannetti, in Environmental Bioremediation Technologies, ed. S. N. Singh and R. D. Tripathi, Springer, Berlin, 2007, p. 57 Search PubMed.
- A. Del Campo, T. Sen, J. P. Lellouche and I. J. Bruce, Multifunctional magnetite and silica-magnetite nanoparticles: Synthesis, surface activation and applications in life sciences, J. Magn. Magn. Mater., 2005, 293, 33–40, DOI:10.1016/j.jmmm.2005.01.040.
- K. Zhang, H. Li, X. Xu and H. Yu, Synthesis of reduced graphene oxide/NiO nanocomposites for the removal of Cr(VI) from aqueous water by adsorption, Microporous Mesoporous Mater., 2018, 255, 7–14, DOI:10.1016/j.micromeso.2017.07.037.
- H. Liu, F. Yang, Y. Zheng, J. Kang, J. Qu and J. P. Chen, Improvement of metal adsorption onto chitosan/Sargassum sp. composite sorbent by an innovative ion-imprint technology, Water Res., 2011, 45, 145–154, DOI:10.1016/j.watres.2010.08.017.
- J. Hu, I. M. C. Lo and G. Chen, Fast removal and recovery of Cr(VI) using surface-modified jacobsite (MnFe2O4) nanoparticles, Langmuir, 2005, 21, 11173–11179, DOI:10.1021/la051076h.
- S. Kalidhasan, A. Santhana Krishna Kumar, V. Rajesh and N. Rajesh, Enhanced adsorption of hexavalent chromium arising out of an admirable interaction between a synthetic polymer and an ionic liquid, Chem. Eng. J., 2013, 222, 454–463, DOI:10.1016/j.cej.2013.02.083.
- V. Neagu, Removal of Cr(VI) onto functionalized pyridine copolymer with amide groups, J. Hazard. Mater., 2009, 171, 410–416, DOI:10.1016/j.jhazmat.2009.06.016.
- M. Taghizadeh and S. Hassanpour, Selective adsorption of Cr(VI) ions from aqueous solutions using a Cr(VI)-imprinted polymer supported by magnetic multiwall carbon nanotubes, Polymer, 2017, 132, 1–11, DOI:10.1016/j.polymer.2017.10.045.
- S. Kim and T. G. Lee, Removal of Cr(VI) from aqueous solution using functionalized poly (GMA-co-EGDMA)-graft-poly(allylamine), React. Funct. Polym., 2019, 134, 133–140, DOI:10.1016/j.reactfunctpolym.2018.11.016.
- E. Makhado, S. Pandey and J. Ramontja, Microwave-assisted green synthesis of xanthan gum grafted diethylamino ethyl methacrylate: an efficient adsorption of hexavalent chromium, Carbohydr. Polym., 2019, 222, 114989, DOI:10.1016/j.carbpol.2019.114989.
- V. Neagu and S. Mikhalovsky, Removal of hexavalent chromium by new quaternized crosslinked poly(4-vinylpyridines), J. Hazard. Mater., 2010, 183, 533–540, DOI:10.1016/j.jhazmat.2010.07.057.
- V. Pakade, E. Cukrowska, J. Darkwa, N. Torto and L. Chimuka, Selective removal of chromium(VI) from sulphates and other metal anions using an ion-imprinted polymer, Water SA, 2011, 37, 529–538, DOI:10.4314/wsa.v37i4.11.
- G. Bayramoglu and M. Y. Arica, Synthesis of Cr(VI)-imprinted poly(4-vinyl pyridine-co-hydroxyethyl methacrylate) particles: its adsorption propensity to Cr(VI), J. Hazard. Mater., 2011, 187, 213–221, DOI:10.1016/j.jhazmat.2011.01.022.
- V. Pakade and L. Chimuka, Polymeric sorbents for removal of Cr(VI) from environmental samples, Pure Appl. Chem., 2013, 85, 2145–2160, DOI:10.1351/pac-con-12-11-17.
- Q. Liang, J. Geng, H. Luo, W. Fang and Y. Yin, Fast and selective removal of Cr(VI) from aqueous solutions by a novel magnetic Cr(VI) ion-imprinted polymer, J. Mol. Liq., 2017, 248, 767–774, DOI:10.1016/j.molliq.2017.10.114.
- X. Qi, S. Gao, G. Ding and A. N. Tang, Synthesis of surface Cr(VI)-imprinted magnetic nanoparticles for selective dispersive solid-phase extraction and determination of Cr(VI) in water samples, Talanta, 2017, 162, 345–353, DOI:10.1016/j.talanta.2016.10.040.
- A. Bhatnagar and M. Sillanpää, Applications of chitin- and chitosan-derivatives for the detoxification of water and wastewater – a short review, Adv. Colloid Interface Sci., 2009, 152, 26–38, DOI:10.1016/j.cis.2009.09.003.
- R. Huang, B. Yang and Q. Liu, Removal of chromium(VI) ions from aqueous solutions with protonated crosslinked chitosan, J. Appl. Polym. Sci., 2013, 129, 908–915, DOI:10.1002/app.38685.
- Y. Zhang, H. L. Ma, J. Peng, M. Zhai and Z. Z. Yu, Cr(VI) removal from aqueous solution using chemically reduced and functionalized graphene oxide, J. Mater. Sci., 2013, 48, 1883–1889, DOI:10.1007/s10853-012-6951-8.
- S. Sessarego, S. C. G. Rodrigues, Y. Xiao, Q. Lu and J. M. Hill, Phosphonium-enhanced chitosan for Cr(VI) adsorption in wastewater treatment, Carbohydr. Polym., 2019, 211, 249–256, DOI:10.1016/j.carbpol.2019.02.003.
- A. E. Ofomaja and Y. S. Ho, Effect of pH on cadmium biosorption by coconut copra meal, J. Hazard. Mater., 2007, 139, 356–362, DOI:10.1016/j.jhazmat.2006.06.039.
- L. C. Maremeni, S. J. Modise, F. M. Mtunzi, M. J. Klink and V. E. Pakade, Adsorptive removal of hexavalent chromium by diphenylcarbazide-grafted Macadamia nutshell powder, Bioinorg. Chem. Appl., 2018, 2018 DOI:10.1155/2018/6171906.
- S. Dawood and T. K. Sen, Removal of anionic dye Congo red from aqueous solution by raw pine and acid-treated pine cone powder as adsorbent: equilibrium, thermodynamic, kinetics, mechanism and process design, Water Res., 2012, 46, 1933–1946, DOI:10.1016/j.watres.2012.01.009.
- E. Rosales, J. Meijide, T. Tavares, M. Pazos and M. A. Sanromán, Grapefruit peelings as a promising biosorbent for the removal of leather dyes and hexavalent chromium, Process Saf. Environ. Prot., 2016, 101, 61–71, DOI:10.1016/j.psep.2016.03.006.
- N. M. Rane, S. V. Admane and R. S. Sapkal, Adsorption of hexavalent chromium from wastewater by using sweetlime and lemon peel powder by batch studies, Waste Manag. Resour. Effic., 2019, pp. 1207–1220, DOI:10.1007/978-981-10-7290-1.
- P. Suksabye and P. Thiravetyan, Cr(VI) adsorption from electroplating plating wastewater by chemically modified coir pith, J. Environ. Manage., 2012, 102, 1–8, DOI:10.1016/j.jenvman.2011.10.020.
- D. Park, Y. S. Yun, J. H. Jo and J. M. Park, Mechanism of hexavalent chromium removal by dead fungal biomass of Aspergillus niger, Water Res., 2005, 39, 533–540, DOI:10.1016/j.watres.2004.11.002.
- W. S. Wan Ngah and M. A. K. M. Hanafiah, Removal of heavy metal ions from wastewater by chemically modified plant wastes as adsorbents: a review, Bioresour. Technol., 2008, 99, 3935–3948, DOI:10.1016/j.biortech.2007.06.011.
- A. Abdolali, W. S. Guo, H. H. Ngo, S. S. Chen, N. C. Nguyen and K. L. Tung, Typical lignocellulosic wastes and by-products for biosorption process in water and wastewater treatment:
A critical review, Bioresour. Technol., 2014, 160, 57–66, DOI:10.1016/j.biortech.2013.12.037.
- V. E. Pakade, T. D. Ntuli and A. E. Ofomaja, Biosorption of hexavalent chromium from aqueous solutions by Macadamia nutshell powder, Appl. Water Sci., 2017, 7, 3015–3030, DOI:10.1007/s13201-016-0412-5.
- J. M. Dias, M. C. M. Alvim-Ferraz, M. F. Almeida, J. Rivera-Utrilla and M. Sanchez-Polo, Waste materials for activated carbon preparation and its use in aqueous-phase treatment: a review, J. Environ. Manage., 2007, 85, 833–846, DOI:10.1016/j.jenvman.2007.07.031.
- O. Ioannidou and A. Zabaniotou, Agricultural residues as precursors for activated carbon production – a review, Renewable Sustainable Energy Rev., 2007, 11, 1966–2005, DOI:10.1016/j.rser.2006.03.013.
- A. Kumar and H. M. Jena, Adsorption of Cr(VI) from aqueous solution by prepared high surface area activated carbon from Fox nutshell by chemical activation with H3PO4, J. Environ. Chem. Eng., 2017, 5, 2032–2041, DOI:10.1016/j.jece.2017.03.035.
- K. M. Doke and E. M. Khan, Equilibrium, kinetic and diffusion mechanism of Cr(VI) adsorption onto activated carbon derived from wood apple shell, Arabian J. Chem., 2017, 10, S252–S260, DOI:10.1016/j.arabjc.2012.07.031.
- M. K. Rai, G. Shahi, V. Meena, R. Meena, S. Chakraborty, R. S. Singh and B. N. Rai, Removal of hexavalent chromium Cr(VI) using activated carbon prepared from mango kernel activated with H3PO4, Resour.-Effic. Technol., 2016, 2, S63–S70, DOI:10.1016/j.reffit.2016.11.011.
- C. A. Demarchi, B. S. Michel, N. Nedelko, A. Ślawska-Waniewska, P. Dłużewski, A. Kaleta, R. Minikayev, T. Strachowski, L. Lipi, J. D. Magro and C. A. Rodrigues, Preparation, characterization, and application of magnetic activated carbon from termite feces for the adsorption of Cr(VI) from aqueous solutions, Powder Technol., 2019, 354, 432–441, DOI:10.1016/j.powtec.2019.06.020.
- L. Khezami and R. Capart, Removal of chromium(VI) from aqueous solution by activated carbons: Kinetic and equilibrium studies, J. Hazard. Mater., 2005, 123, 223–231, DOI:10.1016/j.jhazmat.2005.04.012.
- J. Acharya, J. N. Sahu, B. K. Sahoo, C. R. Mohanty and B. C. Meikap, Removal of chromium(VI) from wastewater by activated carbon developed from tamarind wood activated with zinc chloride, Chem. Eng. J., 2009, 150, 25–39, DOI:10.1016/j.cej.2008.11.035.
- S. Yao, J. Zhang, D. Shen, R. Xiao, S. Gu, M. Zhao and J. Liang, Removal of Pb(II) from water by the activated carbon modified by nitric acid under microwave heating, J. Colloid Interface Sci., 2016, 463, 118–127, DOI:10.1016/j.jcis.2015.10.047.
- R. Fang, H. Huang, W. Huang, J. Ji, Q. Feng, Y. Shu, Y. Zhan, G. Liu and R. Xie, Influence of peracetic acid modification on the physicochemical properties of activated carbon and its performance in the ozone-catalytic oxidation of gaseous benzene, Appl. Surf. Sci., 2017, 420, 905–910, DOI:10.1016/j.apsusc.2017.05.228.
- G. Zhang, Y. Sun, P. Zhao, Y. Xu, A. Su and J. Qu, Characteristics of activated carbon modified with alkaline KMnO4 and its performance in catalytic reforming of greenhouse gases CO2/CH4, J. CO2 Util., 2017, 20, 129–140, DOI:10.1016/j.jcou.2017.05.013.
- S. Omobayo, M. Asif, A. I. Mohammed, N. Baig, A. A. Al-arfaj and T. A. Saleh, Poly(amidoxime) modified magnetic activated carbon for chromium and thallium adsorption: Statistical analysis and regeneration, Process Saf. Environ. Prot., 2019, 121, 254–262, DOI:10.1016/j.psep.2018.10.008.
- B. Li, W. Yin, M. Xu, X. Tan, P. Li, J. Gu, P. Chiang and J. Wu, Facile modification of activated carbon with highly dispersed nano-sized a-Fe2O3 for enhanced removal of hexavalent chromium from aqueous solutions, Chemosphere, 2019, 224, 220–227, DOI:10.1016/j.chemosphere.2019.02.121.
- S. Mortazavian, A. Saber, J. Hong, J. Bae, D. Chun, N. Wong, D. Gerrity, J. Batista, K. J. Kim and J. Moon, Synthesis, characterization, and kinetic study of activated carbon modified by polysulfide rubber coating for aqueous hexavalent chromium removal, J. Ind. Eng. Chem., 2019, 69, 196–210, DOI:10.1016/j.jiec.2018.09.028.
- J. Valentín-Reyes, R. García-Reyes, A. García-González, E. Soto-Regalado and F. Cerino-Córdova, Adsorption mechanisms of hexavalent chromium from aqueous solutions on modified activated carbons, J. Environ. Manage., 2019, 236, 815–822, DOI:10.1016/j.jenvman.2019.02.014.
- V. K. Gupta and T. A. Saleh, Sorption of pollutants by porous carbon, carbon nanotubes and fullerene – an overview, Environ. Sci. Pollut. Res., 2013, 20, 2828–2843, DOI:10.1007/s11356-013-1524-1.
- V. O. Njoku and B. H. Hameed, Preparation and characterization of activated carbon from corncob by chemical activation with H3PO4 for 2,4-dichlorophenoxyacetic acid adsorption, Chem. Eng. J., 2011, 173, 391–399, DOI:10.1016/j.cej.2011.07.075.
- H. Gong, Z. Chen, Y. Fan, M. Zhang, W. Wu and W. Wang, Surface modification of activated carbon for siloxane adsorption, Renewable Energy, 2015, 83, 144–150, DOI:10.1016/j.renene.2015.04.004.
- H. D. Choi, W. S. Jung, J. M. Cho, B. G. Ryu, J. S. Yang and K. Baek, Adsorption of Cr(VI) onto cationic surfactant-modified activated carbon, J. Hazard. Mater., 2009, 166, 642–646, DOI:10.1016/j.jhazmat.2008.11.076.
- V. E. Pakade, L. C. Maremeni, T. D. Ntuli and N. T. Tavengwa, Application of quaternized activated carbon derived from Macadamia nutshells for the removal of hexavalent chromium from aqueous solutions, S. Afr. J. Chem., 2016, 69, 180–188, DOI:10.17159/0379-4350/2016/v69a22.
- A. Paula, C. Pérez and S. Binotto, Carbon nanotubes functionalized with titanium complexes for hexavalent chromium adsorption: an ab initio approach, Comput. Theor. Chem., 2017, 1113, 110–119, DOI:10.1016/j.comptc.2017.05.017.
- M. De Luz-asunción, E. E. Pérez-ramírez, A. L. Martínez-hernández, V. M. Castano, V. Sánchez-mendieta and C. Velasco-santos, Non-linear modeling of kinetic and equilibrium data for the adsorption of hexavalent chromium by carbon nanomaterials: dimension and functionalization, Chin. J. Chem. Eng., 2019, 27, 912–919, DOI:10.1016/j.cjche.2018.08.024.
- M. Dinari and A. Haghighi, Ultrasound-assisted synthesis of nanocomposites based on aromatic polyamide and modified ZnO nanoparticle for removal of toxic Cr(VI) from water, Ultrason. Sonochem., 2018, 41, 75–84, DOI:10.1016/j.ultsonch.2017.09.023.
- D. Anjali Devi, B. Smitha, S. Sridhar and T. M. Aminabhavi, Novel crosslinked chitosan/poly(vinylpyrrolidone) blend membranes for dehydrating tetrahydrofuran by the pervaporation technique, J. Membr. Sci., 2006, 280, 45–53, DOI:10.1016/j.memsci.2006.01.003.
- F. Fu, D. D. Dionysiou and H. Liu, The use of zero-valent iron for groundwater remediation and wastewater treatment: a review, J. Hazard. Mater., 2014, 267, 194–205, DOI:10.1016/j.jhazmat.2013.12.062.
- E. M. Zahran, D. Bhattacharyya and L. G. Bachas, Reactivity of Pd/Fe bimetallic nanotubes in dechlorination of coplanar polychlorinated biphenyls, Chemosphere, 2013, 91, 165–171, DOI:10.1016/j.chemosphere.2012.12.037.
- A. F. C. Campos, H. A. L. de Oliveira, F. N. da Silva, F. G. da Silva, P. Coppola, R. Aquino, A. Mezzi and J. Depeyrot, Core–shell bimagnetic nanoadsorbents for hexavalent chromium removal from aqueous solutions, J. Hazard. Mater., 2019, 362, 82–91, DOI:10.1016/j.jhazmat.2018.09.008.
- M. Chigondo, H. Kamdem, M. Bhaumik, K. Pillay and A. Maity, Magnetic arginine-functionalized polypyrrole
with improved and selective chromium(VI) ions removal from water, J. Mol. Liq., 2019, 275, 778–791, DOI:10.1016/j.molliq.2018.11.032.
- T. Shi, D. Yang, H. Yang, J. Ye and Q. Cheng, Preparation of chitosan crosslinked modified silicon material and its adsorption capability for chromium(VI), Appl. Clay Sci., 2017, 142, 100–108, DOI:10.1016/j.clay.2016.11.023.
- T. R. Sethy and P. K. Sahoo, Highly toxic Cr(VI) adsorption by (chitosan-g-PMMA)/silica bionanocomposite prepared via emulsifier-free emulsion polymerisation, Int. J. Biol. Macromol., 2019, 122, 1184–1190, DOI:10.1016/j.ijbiomac.2018.09.069.
- J. H. Chang, J. Kim and H. Lee, PNIPAm grafted amino-functionalized mesoporous silica for thermo-responsive chromium elimination, Appl. Surf. Sci., 2017, 424, 115–121, DOI:10.1016/j.apsusc.2017.01.168.
- S. Hozhabr Araghi, M. H. Entezari and M. Chamsaz, Modification of mesoporous silica magnetite nanoparticles by 3-aminopropyltriethoxysilane for the removal of Cr(VI) from aqueous solution, Microporous Mesoporous Mater., 2015, 218, 101–111, DOI:10.1016/j.micromeso.2015.07.008.
- J. Qiu, Z. Wang, H. Li, L. Xu, J. Peng, M. Zhai, C. Yang, J. Li and G. Wei, Adsorption of Cr(VI) using silica-based adsorbent prepared by radiation-induced grafting, J. Hazard. Mater., 2009, 166, 270–276, DOI:10.1016/j.jhazmat.2008.11.053.
- S. Nayab, H. Baig, Z. Oluz, H. Duran and B. Yameen, Silica based inorganic–organic hybrid materials for the adsorptive removal of chromium, RSC Adv., 2018, 8, 23963–23972, 10.1039/c8ra04209h.
- H. L. Ma, Y. Zhang, Q. H. Hu, D. Yan, Z. Z. Yu and M. Zhai, Chemical reduction and removal of Cr(VI) from acidic aqueous solution by ethylenediamine-reduced graphene oxide, J. Mater. Chem., 2012, 22, 5914–5916, 10.1039/c2jm00145d.
- J. Geng, Y. Yin, Q. Liang, Z. Zhu and H. Luo, Polyethyleneimine cross-linked graphene oxide for removing hazardous hexavalent chromium: adsorption performance and mechanism, Chem. Eng. J., 2019, 361, 1497–1510, DOI:10.1016/j.cej.2018.10.141.
- L. Zhou, H. Deng, J. Wan, J. Shi and T. Su, A solvothermal method to produce RGO–Fe3O4 hybrid composite for fast chromium removal from aqueous solution, Appl. Surf. Sci., 2013, 283, 1024–1031, DOI:10.1016/j.apsusc.2013.07.063.
- L. Li, H. Duan, X. Wang and C. Luo, Adsorption property of Cr(VI) on magnetic mesoporous titanium dioxide–graphene oxide core–shell microspheres, New J. Chem., 2014, 38, 6008–6016, 10.1039/c4nj00782d.
- L. Han, Y. Zhong, Y. Su, L. Wang, L. Zhu and X. Fei, Nanocomposites based on 3D macroporous biomass carbon with SnS2 nanosheets hierarchical structure for efficient removal of hexavalent chromium, Chem. Eng. J., 2019, 369, 1138–1149, DOI:10.1016/j.cej.2019.03.096.
- W. Lyu, J. Wu, W. Zhang, Y. Liu, M. Yu and Y. Zhao, Easy separated 3D hierarchical coral-like magnetic polyaniline adsorbent with enhanced performance in adsorption and reduction of Cr(VI) and immobilization of Cr(III), Chem. Eng. J., 2019, 363, 107–119, DOI:10.1016/j.cej.2019.01.109.
- H. Su, Y. Chong, J. Wang, D. Long, W. Qiao and L. Ling, Nanocrystalline celluloses-assisted preparation of hierarchical carbon monoliths for hexavalent chromium removal, J. Colloid Interface Sci., 2018, 510, 77–85, DOI:10.1016/j.jcis.2017.08.019.
- J. Sun, Z. Zhang, J. Ji, M. Dou and F. Wang, Removal of Cr 6+ from wastewater via adsorption with high-specific-surface-area nitrogen-doped hierarchical porous carbon derived from silkworm cocoon, Appl. Surf. Sci., 2017, 405, 372–379, DOI:10.1016/j.apsusc.2017.02.044.
- H. Wei, C. Hou, Y. Zhang and Z. Nan, Scalable low temperature in air solid phase synthesis of porous flower-like hierarchical nanostructure SnS2 with superior performance in the adsorption and photocatalytic reduction of aqueous Cr(VI), Sep. Purif. Technol., 2017, 189, 153–161, DOI:10.1016/j.seppur.2017.08.014.
- J. Ananpattarachai and P. Kajitvichyanukul, Enhancement of chromium removal efficiency on adsorption and photocatalytic reduction using a bio-catalyst, titania-impregnated chitosan/xylan hybrid film, J. Cleaner Prod., 2016, 130, 126–136, DOI:10.1016/j.jclepro.2015.10.098.
- X. Wang, Y. Liang, W. An, J. Hu, Y. Zhu and W. Cui, Removal of chromium(VI) by a self-regenerating and metal free g-C3N4/graphene hydrogel system via the synergy of adsorption and photo-catalysis under visible light, Appl. Catal., B, 2017, 219, 53–62, DOI:10.1016/j.apcatb.2017.07.008.
- W. Liu, L. Yang, S. Xu, Y. Chen, B. Liu, Z. Li and C. Jiang, Efficient removal of hexavalent chromium from water by an adsorption-reduction mechanism with sandwiched nanocomposites, RSC Adv., 2018, 8, 15087–15093, 10.1039/c8ra01805g.
- T. D. Ntuli and V. E. Pakade, Hexavalent chromium removal by polyacrylic acid-grafted Macadamia nutshell powder through adsorption–reduction mechanism: adsorption isotherms, kinetics and thermodynamics, Chem. Eng. Commun., 2019, 0, 1–16, DOI:10.1080/00986445.2019.1581619.
- T. A. Kurniawan, G. Y. S. Chan, W. H. Lo and S. Babel, Physico-chemical treatment techniques for wastewater laden with heavy metals, Chem. Eng. J., 2006, 118, 83–98, DOI:10.1016/j.cej.2006.01.015.
- J. jian Pan, J. Jiang and R. kou Xu, Removal of Cr(VI) from aqueous solutions by Na2SO3/FeSO4 combined with peanut straw biochar, Chemosphere, 2014, 101, 71–76, DOI:10.1016/j.chemosphere.2013.12.026.
- X. Lv, Y. Zhang, W. Fu, J. Cao, J. Zhang, H. Ma and G. Jiang, Zero-valent iron nanoparticles embedded into reduced graphene oxide-alginate beads for efficient chromium(VI) removal, J. Colloid Interface Sci., 2017, 506, 633–643, DOI:10.1016/j.jcis.2017.07.024.
- S. Wei, D. Li, Z. Huang, Y. Huang and F. Wang, High-capacity adsorption of Cr(VI) from aqueous solution using a hierarchical porous carbon obtained from pig bone, Bioresour. Technol., 2013, 134, 407–411, DOI:10.1016/j.biortech.2013.02.040.
- C. Hua, R. Zhang, F. Bai, P. Lu and X. Liang, Removal of chromium(VI) from aqueous solutions using quaternized chitosan microspheres, Chin. J. Chem. Eng., 2017, 25, 153–158, DOI:10.1016/j.cjche.2016.08.024.
- J. Zhou, Y. Wang, J. Wang, W. Qiao, D. Long and L. Ling, Effective removal of hexavalent chromium from aqueous solutions by adsorption on mesoporous carbon microspheres, J. Colloid Interface Sci., 2016, 462, 200–207, DOI:10.1016/j.jcis.2015.10.001.
- Z. Ng, J. Lim, H. Daud, S. Ng and M. J. K. Bashir, Reassessment of adsorption–reduction mechanism of hexavalent chromium in attaining practicable, Process Saf. Environ. Prot., 2016, 102, 98–105, DOI:10.1016/j.psep.2016.02.014.
- S. H. Hasan, K. K. Singh, O. Prakash, M. Talat and Y. S. Ho, Removal of Cr(VI) from aqueous solutions using agricultural waste “maize bran”, J. Hazard. Mater., 2008, 152, 356–365, DOI:10.1016/j.jhazmat.2007.07.006.
- A. Gałuszka, Z. Migaszewski and J. Namieśnik, The 12 principles of green analytical chemistry and the SIGNIFICANCE mnemonic of green analytical practices, TrAC, Trends Anal. Chem., 2013, 50, 78–84, DOI:10.1016/j.trac.2013.04.010.
- A. Sharma, K. K. Thakur, P. Mehta and D. Pathania, Efficient adsorption of chlorpheniramine and hexavalent chromium (Cr(VI)) from water system using agronomic waste material, Sustainable Chem. Pharm., 2018, 9, 1–11, DOI:10.1016/j.scp.2018.04.002.
- M. Su, Y. Fang, B. Li, W. Yin, J. Gu, H. Liang, P. Li and J. Wu, Enhanced hexavalent chromium removal by activated carbon modified with micro-sized goethite using a facile impregnation method, Sci. Total Environ., 2019, 647, 47–56, DOI:10.1016/j.scitotenv.2018.07.372.
- D. Mohan and C. U. Pittman Jr., Activated carbons and low cost adsorbents for remediation of tri- and hexavalent chromium from water, J. Hazard. Mater., 2006, 137, 762–811 CrossRef CAS PubMed.
- M. Vakili, S. Deng, T. Li, W. Wang, W. Wang and G. Yu, Novel crosslinked chitosan for enhanced adsorption of hexavalent chromium in acidic solution, Chem. Eng. J., 2018, 347, 782–790, DOI:10.1016/j.cej.2018.04.181.
- A. M. Omer, R. E. Khalifa, Z. Hu, H. Zhang, C. Liu and X. Ouyang, Fabrication of tetraethylenepentamine functionalized alginate beads for adsorptive removal of Cr(VI) from aqueous solutions, Int. J. Biol. Macromol., 2019, 125, 1221–1231, DOI:10.1016/j.ijbiomac.2018.09.097.
- M. S. Samuel, J. Bhattacharya, S. Raj, N. Santhanam, H. Singh and N. D. P. Singh, Efficient removal of chromium(VI) from aqueous solution using chitosan grafted graphene oxide (CS-GO) nanocomposite, Int. J. Biol. Macromol., 2019, 121, 285–292, DOI:10.1016/j.ijbiomac.2018.09.170.
- S. Hokkanen, A. Bhatnagar and M. Sillanpää, A review on modification methods to cellulose-based adsorbents to improve adsorption capacity, Water Res., 2016, 91, 156–173 CrossRef CAS PubMed.
- N. H. Kera, M. Bhaumik, K. Pillay, S. S. Ray and A. Maity, m-Phenylenediamine-modified polypyrrole as an efficient adsorbent for removal of highly toxic hexavalent chromium in water, Mater. Today Commun., 2018, 15, 153–164, DOI:10.1016/j.mtcomm.2018.02.033.
- P. Becalli Vilela, A. Dalalibera, E. C. Duminelli, V. A. Becegato and A. T. Paulino, Adsorption and removal of chromium(VI) contained in aqueous solutions using a chitosan-based hydrogel, 2018, DOI:10.1007/s11356-018-3208-3.
- X. Xu, H. Zhang, C. Ma, H. Gu, H. Lou, S. Lyu, C. Liang, J. Kong and J. Gu, A superfast hexavalent chromium scavenger: Magnetic nanocarbon bridged nanomagnetite network with excellent recyclability, J. Hazard. Mater., 2018, 353, 166–172, DOI:10.1016/j.jhazmat.2018.03.059.
- D. Zhong, Y. Zhang, L. Wang, J. Chen, Y. Jiang, D. C. W. Tsang, Z. Zhao, S. Ren, Z. Liu and J. C. Crittenden, Mechanistic insights into adsorption and reduction of hexavalent chromium from water using magnetic biochar composites: key roles of Fe3O4 and persistent free, Environ. Pollut., 2018, 243, 1302–1309, DOI:10.1016/j.envpol.2018.08.093.
- L. Zhou, G. Zhang, M. Wang, D. Wang, D. Cai and Z. Wu, Efficient removal of hexavalent chromium from water and soil using magnetic ceramsite coated by functionalized nano carbon spheres, Chem. Eng. J., 2018, 334, 400–409, DOI:10.1016/j.cej.2017.10.065.
- H. Liang, B. Song, P. Peng, G. Jiao, X. Yan and D. She, Preparation of three-dimensional honeycomb carbon materials and their adsorption of Cr(VI), Chem. Eng. J., 2019, 367, 9–16, DOI:10.1016/j.cej.2019.02.121.
- G. Zhu, J. Song, W. Dong, J. Lu, Y. Wang, W. Jiang and P. Guo, Removal of hexavalent chromium from water by modified sponge iron particles and insights into mechanism, 2018, pp. 26173–26181 Search PubMed.
- J. Guo, J. Li and C. Wang, Adsorptive removal of Cr(VI) from simulated wastewater in MOF BUC-17 ultra fine powder, J. Environ. Chem. Eng., 2019, 7, 102909, DOI:10.1016/j.jece.2019.102909.
- W. Cai, J. Wei, Z. Li, Y. Liu, J. Zhou and B. Han, Preparation of amino-functionalized magnetic biochar with excellent adsorption
performance for Cr(VI) by a mild one-step hydrothermal method from peanut hull, Colloids Surf., A, 2019, 563, 102–111, DOI:10.1016/j.colsurfa.2018.11.062.
- H. Daraei, A. Mittal, M. Noorisepehr and J. Mittal, Separation of chromium from water samples using eggshell powder as a low-cost sorbent: kinetic and thermodynamic studies, Desalin. Water Treat., 2015, 53, 214–220, DOI:10.1080/19443994.2013.837011.
- E. Ben Khalifa, B. Rzig, R. Chakroun, H. Nouagui and B. Hamrouni, Chemometrics and intelligent laboratory systems application of response surface methodology for chromium removal by adsorption on low-cost biosorbent, Chemom. Intell. Lab. Syst., 2019, 189, 18–26, DOI:10.1016/j.chemolab.2019.03.014.
- W. Cherdchoo, S. Nithettham and J. Charoenpanich, Removal of Cr(VI) from synthetic wastewater by adsorption onto coffee ground and mixed waste tea, Chemosphere, 2019, 221, 758–767, DOI:10.1016/j.chemosphere.2019.01.100.
- G. V. K. Mohan, A. N. Babu and K. K. K. Ravindhranath, Removal of chromium(VI) from water using adsorbent derived from spent coffee grounds, Int. J. Environ. Sci. Technol., 2019, 16, 101–112, DOI:10.1007/s13762-017-1593-7.
- M. Nigam, S. Rajoriya, S. Rani and P. Kumar, Adsorption of Cr(VI) ion from tannery wastewater on tea waste: kinetics, equilibrium and thermodynamics studies, J. Environ. Chem. Eng., 2019, 7, 103188, DOI:10.1016/j.jece.2019.103188.
- N. K. Mondal and B. Sambrita, Potentiality of waste human hair towards removal of chromium(VI) from solution: kinetic and equilibrium studies, Appl. Water Sci., 2019, 9, 1–8, DOI:10.1007/s13201-019-0929-5.
|
This journal is © The Royal Society of Chemistry 2019 |