DOI:
10.1039/C9RA04699B
(Paper)
RSC Adv., 2019,
9, 26483-26486
Alkene hydroboration with pinacolborane catalysed by lithium diisobutyl-tert-butoxyaluminum hydride†
Received
23rd June 2019
, Accepted 17th August 2019
First published on 23rd August 2019
Abstract
Here we developed a highly efficient alkene hydroboration protocol, showing that various alkyl boronates can be smoothly obtained in good yields by reacting alkenes with pinacolborane (HBpin) in the presence of 5 mol% lithium diisobutyl-tert-butoxyaluminum hydride. The coordination of aluminate ions with lithium cations allowed for effective hydride transfer during hydroboration, and the obtained boronate ester was further used for C–C coupling, trifluoroboronate salt formation, and oxidation to alcohol.
Introduction
The increasing interest in catalytic hydro-functionalization (hydrosilylation/boration) and manipulation has inspired the development of suitable protocols for the synthesis of organometallics.1,2 In particular, much attention has been directed at the catalytic hydroboration of unsaturated hydrocarbons, e.g., noble metal-catalyzed hydroboration of alkenes and alkynes, as these reactions afford organoboron compounds as versatile building blocks for a variety of transformations and cross-coupling reactions. In this regard, metal catalyzed (precious metal catalyst) hydroboration of alkenes and alkynes have been studied extensively to synthesize these valuable precursors.3,4 Although Earth-abundant transition metal and main group metal catalysts are also used in hydroboration reactions (especially in those of carbonyl compounds) and can exhibit activities similar to those of their noble metal counterparts, most of these catalysts however, correspond to pincer or phosphine ligand-containing complexes.5 The catalytic hydroboration of carbonyl compounds has been widely investigated, whereas that of alkenes and alkynes with readily available main group hydride reagent has received little attention6 (Fig. 1).
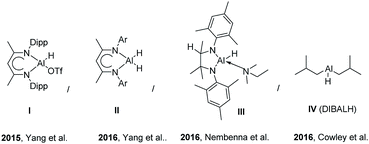 |
| Fig. 1 Reported aluminum hydrides for catalytic hydroboration of C O, C C bonds. | |
Group 13 hydrides have been extensively studied because of their ability to store hydrogen, participate in various organic transformations, and mediate the reduction of unsaturated substrates.7 In particular, mono- and dihydrides of Al, the third most abundant element (8.1%) in the Earth's crust [after O (46.6%) and Si (27.7%)], have been used for the hydroboration of carbonyls and alkynes.
In 2015, Yang et al.8 reported [LAlH(OTf)] (L = HC(CMeNAr)2, Ar = 2,6-iPr2C6H3)-catalyzed hydroboration of carbonyl groups and the addition of trimethylsilyl cyanide to aldehydes and ketones. In another investigation, Yang et al. reported Al dihydride LAlH2 (L = HC(CMeNAr)2, Ar = 2,6-Et2C6H3)-catalyzed hydroboration of terminal alkynes and dehydrocoupling of boranes with amines, phenols, and thiols in deuterated solvents.9 More recently, Bismuto et al. reported DIBAL-H (10 mol%) catalyzed hydroboration of alkynes and the hydroboration of alkenes with pinacolborane (HBpin) catalyzed by commercially available Al hydrides such as the highly reactive LiAlH4 and/or Na bis(2-methoxyethoxy)aluminum hydride (Red-Al), exploring the substrate scope for 10 mol% LiAlH4 (Scheme 1).10,11 Mulvey et al. reported that Al-containing anionic ate complexes with alkali metal (Li–Al cooperativity) featured synergistic reactivity for effective hydroboration. In their study, Mulvey et al. compared the hydroboration of aldehydes, ketones, imines and alkynes in the presence of bimetallic lithium aluminates and neutral aluminum counterparts as catalysts.12
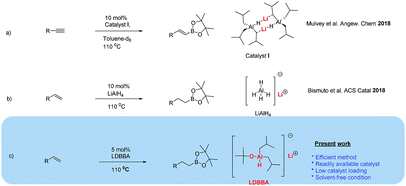 |
| Scheme 1 (a and b) Previously reported Al hydride-catalyzed hydroborations and (c) Al hydride-catalyzed hydroboration developed herein. | |
Based on the works of Yang, Bismuto, Thomas, Mulvey, and our recent reports on selective and partial reductions promoted by Al-containing catalysts, we herein present the results of our research on the catalytic hydroboration of alkenes with lithium diisobutyl-tert-butoxyaluminum hydride (LDBBA) and HBpin.
Lithium diisobutyl-tert-butoxyaluminum hydride (LDBBA) is a new class of reducing agent know for the partial reduction of esters and amides (tertiary and Weinreb amides) to aldehydes.13 LDBBA was also reported for the one pot synthesis of secondary alcohols such as, vinyl and propargyl alcohols from the ester precursor.14 Recently, LDBBA was successfully applied in the flow chemistry to achieve the selective reduction of esters.15
Due to the mild nature, easy to handle and simple work up procedure associated with LDBBA, we applied for catalytic hydroboration of alkenes in an effective manner under solvent-free condition (Scheme 1).
Recently reported aluminium hydrides for catalytic hydroboration reactions are depicted in below figure.
Results and discussion
When alkene (styrene) hydroboration was performed with 3.0 equiv. HBpin and 10 mol% catalyst (LDBBA) at room temperature, the corresponding boronate was obtained only in small yield (entry 1, Table 1). Higher conversions were obtained when the reaction temperature was increased and the loading of HBpin was reduced, e.g., quantitative conversion was achieved with a 10 mol% catalyst loading within 2 h (entry 2). Based on this observation, we tried to further improve the reaction conditions. The reaction was repeated under the conditions of entry 2 with reduced catalyst loading of 5 mol%, which, again, resulted in quantitative hydroboration within 2 h (entry 3). Notably, our method proved to be more effective than the recently reported method using highly reactive LiAlH4.
Table 1 Optimization of reaction conditions for LDBBA-catalyzed hydroboration of alkenes

|
Entry |
Catalyst (mol%) |
HBpin (equiv.) |
Temp. |
Time (h) |
Conversiona (%) |
Conversions were determined from GC peak area ratios based on starting material consumption. Isolated yield. Reaction with mL toluene; LTBA (lithium tri-tert-butoxy aluminum hydride). |
1 |
LDBBA (10) |
3.0 |
rt |
24 |
18% |
2 |
LDBBA (10) |
1.5 |
110 °C |
2 |
99% |
3 |
LDBBA (5) |
1.5 |
110 °C |
2 |
99% |
4 |
LDBBA (5) |
1.2 |
110 °C |
2 |
99% (91)b |
5 |
LDBBA (5) |
1.2 |
110 °C |
1 |
74% |
6c |
LDBBA (5) |
1.2 |
110 °C |
2 |
72% |
7 |
LDBBA (1) |
1.2 |
110 °C |
2 |
57% |
8 |
No catalyst |
2.0 |
110 °C |
12 |
23% |
9 |
DIBALH (5) |
1.2 |
110 °C |
2 |
59% |
10 |
LTBA (5) |
1.2 |
110 °C |
2 |
79% |
11 |
Red-Al (5) |
1.2 |
110 °C |
4 |
85% 11 |
Considering this results, we next decreased HBpin loading from 1.5 to 1.2 equiv., demonstrating that quantitative hydroboration was achieved. The resulting crude boronate was extracted with ethyl acetate, volatiles evaporation followed by column chromatography from silica gel affording the isolated yield of the corresponding alkyl boronate equaled 91% (entry 4). However, conversion decreased to 74% when the reaction time was reduced to 1 h (entry 5). Although alkene hydroboration was also observed for catalyst loading of 1 and 0 mol% at higher HBpin loadings, no significant conversion to product was achieved in both cases (entries 6 and 7). Therefore, it was concluded that optimal conditions for the conversion of alkene to alkyl boronate correspond to 5 mol% LDBBA, 1.2 equiv. HBpin, 110 °C, and a reaction time of 2 h (entry 4).
With the optimized conditions in hand, we explored the substrate scope (Scheme 2), revealing that both electron-rich alkenes (bearing 2-methyl, 3-methyl, 4-methyl, 4-methoxy, 4-tert-butyl substituents) and electron-poor alkenes (bearing 4-chloro, 4-bromo and 4-fluoro substituents) reacted in the same manner to afford the corresponding boronates in good yields. Allylbenzene was converted to the expected product in good yield (97%), while a longer reaction time was required for an alpha methyl-substituted styrene (prop-1-en-2-ylbenzene). A polyaromatic substrate 2-vinylnaphthalene and an aliphatic alkenes (1-heptene, 1-decene) were converted to the corresponding boronate esters in good yields. In addition, a dialkene 1,7-heptene was also afforded corresponding bis-boronate smoothly in good yield.
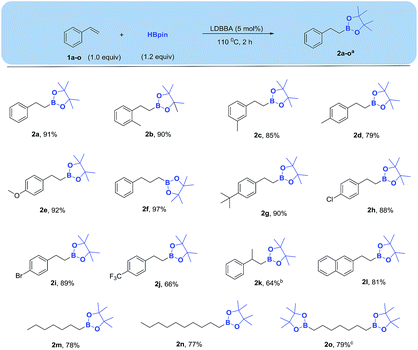 |
| Scheme 2 Substrate scope for LDBBA-catalyzed hydroboration of alkenes. Reaction condition: alkene (1.0 mmol), HBpin (1.2 mmol), LDBBA (5 mol%), 110 °C, 2 h; aisolated yields; bHBpin (2.0 equiv.), 6 h; cHBpin (2.4 equiv.), 4 h. | |
Because of their significant utility, boronates are often transformed into other substances in organic synthesis. Herein, boronate ester 2a was treated with H2O2 in the presence of aqueous NaOH or methanolic KHF2 to afford the corresponding alcohol or trifluoro boronate products, respectively, in good yield.16 Further, the Suzuki reaction of 2a with 4-nitrobromobenzene afforded 1-nitro-4-phenethylbenzene (Scheme 3).17
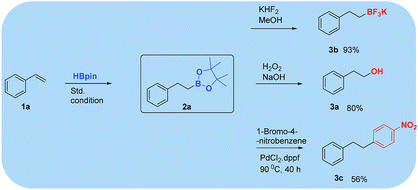 |
| Scheme 3 Transformation of 4,4,5,5-tetramethyl-2-phenethyl-1,3,2-dioxaborolane 2a. | |
Conclusions
In conclusion, we demonstrated that alkenes can be efficiently hydroborated in the presence of commercial lithium diisobutyl-tert-butoxyaluminum hydride (LDBBA), revealing that a catalyst loading of 5 mol% is sufficient to produce alkyl boronates from a variety of substituted alkenes in good to excellent yields. In addition, boronate 2a was used in a cross-coupling reaction and employed to prepare synthetically valuable synthons such as the corresponding alcohol and potassium trifluoroborate salt. The coordination of anionic aluminate with lithium allowed for effective hydride transfer during hydroboration. Thus, the present method of alkene hydroboration is a good alternative to the existing complex transition and precious metal-mediated hydroborations.
Conflicts of interest
There are no conflicts to declare.
Acknowledgements
This study was supported by the National Research Foundation of Korea Grant funded by the Korean Government (2017R1D1A1B03035412).
Notes and references
- Synthesis and application of organoboron compounds, ed. E. Fernandez and A. Whiting, Springer, Heidelberg, 2015 Search PubMed; Boronic Acids, ed. D. G. Hall, Wiley-VHC, Weinheim, Germany, 2011 Search PubMed.
-
(a) H. C. Brown and B. C. S. Rao, J. Am. Chem. Soc., 1956, 78, 5694–5695 CrossRef CAS;
(b) H. C. Brown, N. R. De Lue, G. W. Kabalka and H. C. Hedgecock, J. Am. Chem. Soc., 1976, 98, 1290–1291 CrossRef CAS;
(c) C. M. Crudden and D. Edwards, Eur. J. Org. Chem., 2003, 4695–4712 CrossRef CAS;
(d) J. V. Obligacion and P. J. Chirik, Nat. Rev. Chem., 2018, 2, 15–34 CrossRef CAS PubMed;
(e) J. Schmidt, J. Choi, A. T. Liu, M. Slusarczyk and G. C. Fu, Science, 2016, 354, 1265–1269 CrossRef CAS PubMed; For catalyzed hydrosilylations & transformations see:
(f) T. Tuttle, D. Wang, W. Thiel, J. Köhler, M. Hofmann and J. Weis, Organometallics, 2006, 25, 4504–4513 CrossRef CAS;
(g) K. Itami, K. Mitsudo, A. Nishino and J. Yoshida, J. Org. Chem., 2002, 67, 2645–2652 CrossRef CAS PubMed;
(h) Y. Nakajima and S. Shimada, RSC Adv., 2015, 5, 20603–20616 RSC;
(i) T. Saito, Y. Nishimoto, M. Yasuda and A. Baba, J. Org. Chem., 2006, 71, 8516–8522 CrossRef CAS PubMed;
(j) S. Kim and J. H. Park, J. Org. Chem., 1988, 53, 3111–3113 CrossRef CAS;
(k) Z. Wang and S. Chang, Org. Lett., 2013, 15, 1990–1993 CrossRef CAS PubMed;
(l) X. Jia and Z. Huang, Nat. Chem., 2016, 8, 157–161 CrossRef CAS PubMed.
-
(a) D. Mannig and H. Nöth, Angew. Chem., Int. Ed. Engl., 1985, 24, 878–879 CrossRef;
(b) T. Hayashi, Y. Matsumoto and Y. Ito, J. Am. Chem. Soc., 1989, 111, 3426–3428 CrossRef CAS;
(c) D. A. Evans and G. C. Fu, J. Org. Chem., 1990, 55, 2280–2282 CrossRef CAS;
(d) D. A. Evans, G. C. Fu and B. A. Anderson, J. Am. Chem. Soc., 1992, 114, 6679–6685 CrossRef CAS;
(e) A. Leyva, X. Zhang and A. Corma, Chem. Commun., 2009, 4947–4949 RSC;
(f) C. Gunanathan, M. Hölscher, F. Pan and W. Leitner, J. Am. Chem. Soc., 2012, 134, 14349–14352 CrossRef CAS PubMed;
(g) D. Noh, H. Chea, J. Ju and J. Yun, Angew. Chem., Int. Ed., 2009, 48, 6062–6064 CrossRef CAS PubMed;
(h) S. Kisan, V. Krishnakumar and C. Gunanathan, ACS Catal., 2017, 7, 5950–5954 CrossRef CAS;
(i) Y. Xi and J. F. Hartwig, J. Am. Chem. Soc., 2016, 138, 6703–6706 CrossRef CAS PubMed;
(j) Y. Yamamoto, R. Fujikawa, T. Umemoto and N. Miyaura, Tetrahedron, 2004, 60, 10695–10700 CrossRef CAS;
(k) D. Fiorito and C. Mazet, ACS Catal., 2018, 8, 9382–9387 CrossRef CAS.
-
(a) N. Miyaura, K. Yamada and A. Suzuki, Tetrahedron Lett., 1979, 20, 3437–3440 CrossRef;
(b) N. Miyaura and A. Suzuki, Chem. Rev., 1995, 95, 2457–2483 CrossRef CAS;
(c) C. C. Chong and R. Kinjo, ACS Catal., 2015, 5, 3238–3259 CrossRef CAS;
(d) C. Sandford and V. K. Aggarwal, Chem. Commun., 2017, 53, 5481–5494 RSC;
(e) G. Hu, W. Chen, T. Fu, Z. Peng, H. Qiao, Y. Gao and Y. Zhao, Org. Lett., 2013, 15, 5362–5365 CrossRef CAS PubMed;
(f) B. S. L. Collins, C. M. Wilson, E. L. Myers and V. K. Aggarwal, Angew. Chem., Int. Ed., 2017, 56, 11700–11733 CrossRef CAS PubMed.
-
(a) H. Zhang and Z. Lu, ACS Catal., 2016, 6, 6596–6600 CrossRef CAS;
(b) Z. Zuo, J. Yang and Z. Huang, Angew. Chem., Int. Ed., 2016, 55, 10839–10843 CrossRef CAS PubMed;
(c) G. Zhang, H. Zeng, J. Wu, Z. Yin, S. Zheng and J. C. Fettinger, Angew. Chem., Int. Ed., 2016, 55, 14369–14372 CrossRef CAS PubMed;
(d) H. B. Daat, C. L. Rock, M. Flores, T. L. Groy, A. C. Bowman and R. J. Trovitch, Chem. Commun., 2017, 53, 7333 RSC;
(e) S. R. Tamang, D. Bedi, S. S. Haghighi, C. R. Smith, C. Crawford and M. Findlater, Org. Lett., 2018, 20, 6695–6700 CrossRef CAS PubMed;
(f) Y. Wu, C. Shan, Y. Sun, P. Chen, J. Ying, J. Zhu, L. Liu and Y. Zhao, Chem. Commun., 2016, 52, 13799–13802 RSC;
(g) J. V. Obligacion, J. M. Neely, A. N. Yazdani, I. Pappas and P. J. Chirik, J. Am. Chem. Soc., 2015, 137, 5855–5858 CrossRef CAS PubMed.
-
(a) V. K. Jakhar, M. K. Barman and S. Nembenna, Org. Lett., 2016, 18, 4710–4713 CrossRef CAS PubMed;
(b) G. I. Nikonov, ACS Catal., 2017, 7, 7257–7266 CrossRef CAS;
(c) A. Harinath, I. Banerjee, J. Bhattacharjee and T. K. Panda, New J. Chem., 2019, 43, 10531–10536 RSC.
-
(a) A. J. Downs and C. R. Pulham, Chem. Soc. Rev., 1994, 23, 175–184 RSC;
(b) S. Aldridge and A. J. Downs, Chem. Rev., 2001, 101, 3305–3366 CrossRef CAS PubMed;
(c) X. Liu, H. W. Langmi, S. D. Beattie, F. F. Azenwi, G. S. McGrady and C. M. Jensen, J. Am. Chem. Soc., 2011, 133, 15593–15597 CrossRef CAS PubMed;
(d) L. A. Berben, Chem.–Eur. J., 2015, 21, 2734–2742 CrossRef CAS PubMed;
(e) W. Uhl, C. Appelt, J. Backs, H. Westenberg, A. Wollschlager and J. Tannert, Organometallics, 2014, 33, 1212–1217 CrossRef CAS.
- Z. Yang, M. Zhong, X. Ma, S. De, C. Anusha, P. Parameswaran and H. W. Roesky, Angew. Chem., Int. Ed., 2015, 54, 10225–10229 CrossRef CAS PubMed.
- Z. Yang, M. Zhong, X. Ma, K. Nijesh, S. De, P. Parameswaran and H. W. Roesky, J. Am. Chem. Soc., 2016, 138, 2548–2551 CrossRef CAS PubMed.
- A. Bismuto, S. P. Thomas and M. J. Cowley, Angew. Chem., Int. Ed., 2016, 55, 15356–15359 CrossRef CAS PubMed.
- A. Bismuto, M. J. Cowley and S. P. Thomas, ACS Catal., 2018, 8, 2001–2005 CrossRef CAS.
-
(a) V. A. Pollard, M. A. Fuentes, A. R. Kennedy, R. McLellan and R. E. Mulvey, Angew. Chem., Int. Ed., 2018, 57, 1–6 CrossRef PubMed;
(b) V. A. Pollard, S. A. Orr, R. McLellan, A. R. Kennedy, E. Hevia and R. E. Mulvey, Chem. Commun., 2018, 54, 1233 RSC.
-
(a) M. S. Kim, Y. Mi and D. K. An, Tetrahedron Lett., 2007, 48, 5061–5064 CrossRef CAS;
(b) S. B. Choi, K. J. Lee and D. K. An, Bull. Korean Chem. Soc., 2008, 29, 1407–1408 CrossRef CAS;
(c) J. S. An, W. K. Shin and D. K. An, Bull. Korean Chem. Soc., 2015, 36, 2928–2931 CrossRef CAS.
-
(a) M. J. Chae, A. R. Jeon, T. Livinghouse and D. K. An, Chem. Commun., 2011, 47, 3281–3283 RSC;
(b) M. J. Chae, A. R. Jeon, J. K. Park and D. K. An, Tetrahedron Lett., 2011, 52, 1718–1720 CrossRef CAS.
-
(a) J. M. Muñoz, J. Alcázar, A. Hoz and A. Díaz-Ortiz, Eur. J. Org. Chem., 2012, 2012, 260–263 CrossRef;
(b) N. Alonso, J. M. Muñoz, B. Egle, J. L. Vrijdag, W. M. De Borggraeve, A. de la Hoz, A. Díaz-Ortiz and J. Alcázar, J. Flow Chem., 2014, 4, 105–109 CrossRef.
-
(a) K. Yanga and Q. Song, Green Chem., 2016, 18, 932–936 RSC;
(b) L. Zhang, Z. Zuo, X. Leng and Z. Huang, Angew. Chem., Int. Ed., 2014, 53, 2696–2700 CrossRef CAS PubMed.
-
(a) G. A. Molander, C. S. Yun, M. Ribagorda and B. Biolatto, J. Org. Chem., 2003, 68, 5534–5539 CrossRef CAS PubMed;
(b) G. A. Molander and C. S. Yun, Tetrahedron, 2002, 58, 1465–1470 CrossRef CAS.
Footnote |
† Electronic supplementary information (ESI) available. See DOI: 10.1039/c9ra04699b |
|
This journal is © The Royal Society of Chemistry 2019 |
Click here to see how this site uses Cookies. View our privacy policy here.