DOI:
10.1039/C9RA04451E
(Paper)
RSC Adv., 2019,
9, 25583-25591
Screening of microalgae for biosynthesis and optimization of Ag/AgCl nano hybrids having antibacterial effect
Received
13th June 2019
, Accepted 8th August 2019
First published on 15th August 2019
Abstract
Here we report a facile and novel bio-synthesis technique, using algal extract to reduce silver metal ions into Ag/AgCl nanoparticles. Different concentrations of metallic precursors of silver nitrate (0.1 mM, 0.2 mM, 0.5 mM and 1 mM) were tested with alcoholic extract prepared from biomass of Chlorella sp. for nanoparticle biosynthesis which was screened out of four species namely Chlorella sp., Lyngbya putealis, Oocystis sp. and Scenedesmus vacuolatus. The biomolecules present in the alcoholic extract assisted in the synthesis of nanoparticles by reducing the metallic salt to metal ions and acting as capping agents in order to stabilize the particles. The synthesized particles were characterized for physico-chemical properties. DLS analysis of particles prepared from Chlorella sp. shows the particles with size of 90.6 nm. These biosynthesized nanoparticles show great potential applications in antibacterial activity.
Introduction
Biological synthesis of nanoparticles is an economical and environmentally friendly method, but its optimization is a challenge. Biosynthesis involves either the extract from some green sources like plants, algae, fungus, and bacteria or the micro-organism itself which includes the intracellular synthesis of these particles. Here, in the present study, we are focusing on Ag/AgCl nanoparticles synthesis using freshwater green algae. The toxic chemical species used as capping agents in the chemical synthesis of nanoparticles causes a significant problem of environmental toxicity when it interacts with the biological systems causing detrimental effects on the organisms sustaining that system.1 In recent years there are many researchers around the world working in the field of biogenic nanoparticles synthesis. Freshwater algal species like Scenedesmus has been used for synthesis of 5–10 nm size silver nanoparticles.2 Green chemistry approaches for nanoparticles synthesis includes the interactive studies of microbial extracts or microbes itself with the metallic precursor of the nanoparticles to be synthesized; which generates a link between the nanoscience and microbes leading to microbial nanotechnology.3 Silver nanoparticles have been paid significant attention by many scientists because of its properties like antimicrobial activity and catalytic performance.2 Other than silver, many other nanoparticles like gold, platinum etc. have also been synthesized using this green chemistry approach. Some people have used plants like Azadirachta indica and others have used micro-organisms like green algae for synthesis.4,5 Cotton ball alga which is a freshwater alga has been used for silver nanoparticle synthesis to explore its antibacterial properties.6 Most of the studies for synthesis of silver nanoparticles using microalgae have been done using silver nitrate as a metallic precursor with a concentration of 1 mM.6,7 Ferriera et al. have used 3.5 mM AgNO3 for Ag/AgCl nanoparticles synthesis using Chlorella vulgaris.8 Similar studies have been done using corn husk extract with 2 mM AgNO3 as metallic precursor.9–11 Thus there is always a chance of high concentration of metallic salt going to environment which led us to optimize for lower concentration of metallic precursor in manufacturing nanoparticles. Earlier researchers have carried out screening of different species to biosynthesize silver nanoparticles for its antimicrobial activity. In the study reported by Patel et al. eight species were screened to find the best one, for nanoparticle synthesis.12 Various chemical and physical methods are being used for nanoparticle synthesis having their own advantages and disadvantages. Chemical methods utilize loads of chemicals and physical methods involve costly processes like attrition, pyrolysis, etc.13 Chemically modified compounds can cause environmental hazards as well as increase the reaction cost. However, the efficiency of chemicals methods cannot be questioned but green synthesis would be a better approach if one can optimize the process of biosynthesis.14 We do not require so many reactants which complexes the synthesis process and increase the production cost. It involves very simple process of reduction which results in nucleation and growth of nanoparticles. Algal ability to handle higher concentrations of metallic precursors and produce valuable biomass for purposes like biofuel generation, pigments, omega-3, and other high value-added compounds make it a suitable candidate for nano factories of nature.15–17 The exact mechanism of reduction differs from one species to another and the type of nanoparticles to be synthesized. Gold shaping protein has been identified in microalgae which can shape the gold nanoparticles being synthesized.13 High and low protein molecules are involved in formation of silver nanoparticles. Studies shows the comparison of two different procedures for synthesis; first one is using the live cells and incubating it with precursor salt for silver nanoparticle synthesis, and the second method includes the use of cell-free media and incubation of silver nitrate within this media to produce nanoparticles.12,18,19 However, identifying the exact biomolecules responsible for this bio-reduction process is a challenge as each species may have some different molecule acting as bio reductant in dominant form.13 The functional groups from different biomolecules like proteins, carbohydrates, lipids and water stretching bonds play a vital role in bio reduction.20,21 Proteins and amino acids have also been used for production of protein gold hybrid acting as stabilizing and capping agents enhancing efficiency and applicability in biomedical sciences.22,23 In present study, we propose an optimization and characterization process for the synthesis of Ag/AgCl nanoparticles after screening different microalgae species for biosynthesis. This study emphasizes on one of the essential factors i.e. optimization process of nanoparticles biosynthesis at different concentrations of silver nitrates. We tried the synthesis of silver nanoparticles with each of the mentioned concentration of silver nitrate by reducing them with extract prepared from 5 g biomass of the Chlorella sp. which was chosen after screening from different algal species. Toxicity of the biosynthesized particles is comparatively low as compared to those synthesized with chemical or physical methods leading to many applications related to pharmaceuticals and antibacterial studies. The biosynthesis of Ag/AgCl nanoparticles is a challenge as it may happen that only AgCl nanoparticles might be synthesized during the process because the reducing agents present in the extract may not be able to convert the AgCl into AgNPs where chlorine is an integral part of algal micronutrient and helps in various metabolic pathways. Some researchers have used NaOH24 for controlling the conversion of AgCl to AgNPs during reduction process while we propose that this conversion can be controlled by utilizing optimum concentration of metallic precursor and one can also play with the amount of extract to be added to optimize the process. Therefore, this study is important to show if Ag/AgCl nanoparticles hybrid can be produced which can act as an antibacterial agent or not. Objective of this study is production of Ag/AgCl nanoparticles while optimizing the best concentration of metallic precursor to be used for synthesis and the screening of algal species to be used for extract preparation in order to synthesize the nano hybrids along with possible use as an antibacterial agent.
Materials and methods
Growth and culture conditions of microalgae
Four different axenic strains of freshwater green algae were tested for their efficiency as producers of nanoparticles using extracellular synthesis method. The species used during this experiment were Chlorella sp., Lyngbya putealis, Oocystis sp. and Scenedesmus vacuolatus. The mentioned axenic species of algae were grown aseptically and maintained in unmodified BG-11 medium (pH-7.2).25 The cultures were maintained in the culture room at a temperature of 28 ± 5 °C with 12
:
12 hours of light and dark photoperiod and harvested on 10th day. During the light period, light intensity of 3000 lux was maintained using white fluorescent lamps at an appropriate distance from the cultures to sustain proper growth of culture.
Design of study
Alcoholic extract of Chlorella sp., Scenedesmus vacuolatus, Lyngbya putealis, and Oocystis sp. were prepared using 5 g dried biomass of each species in 50 ml of 95% ethanol and keeping it on stirrer for shaking (24 h). After that, the extracts were taken and centrifuged at 4000 rpm for 20 minutes, and then the supernatant was filtered using Whatman filter paper, 125 mm.26 This extract was then used for biosynthesis of nanoparticles.
Biosynthesis of Ag/AgCl nanoparticles
The extracts obtained were used as a reducing molecule for silver nitrate, metallic precursor in current study. The extract prepared from each species were used against 1 mM AgNO3 in the ratio of 90
:
10 (90 ml AgNO3 and 10 ml of extract) for initial screening of the cells based on their reducing ability for silver nitrate to corresponding nanoparticles.
Process optimization set up
After screening of the species, different concentrations of silver nitrate (0.1 mM, 0.2 mM, 0.5 mM and 1 mM) were explored for synthesis of nanoparticles using extract prepared from biomass of Chlorella sp. 10 ml of extract prepared from biomass was incubated with 90 ml of 0.1 mM, 0.2 mM, 0.5 mM and 1 mM AgNO3. pH of the reaction mixture was 7.37 ± 0.2 and the reaction was carried out at room temperature 28 °C ± 2 °C. Cells of Chlorella sp. have an average cell size of 5.7 μm ± 0.2 μm measured using Olympus cell counter model-R1 and chlorophyll pigment concentration of 13.5 ± 0.1 mg L−1. A time-based UV-visible spectroscopic study was carried out, and the synthesized product was scanned in the wavelength range of 300 nm to 700 nm with time, i.e., at 0, 20, 40 minutes, 3 h, 5 h and continued till 192 h to check the stability of the product in each combination of metallic precursor. Then the particles were centrifuged and lyophilized for detailed characterization as discussed in next section.
Characterization of Ag/AgCl nanoparticles
The particles synthesized were characterized using particulate systems nanoplus version 5.22 (Micromeritics Instruments Corporation) to get an approximate estimation of particle size synthesized from extracts of different microalgae. UV-visible spectrophotometry was done with a wavelength scan ranging from 300 nm to 700 nm at varying time interval from 0 minutes to 192 h using DR-6000 HACH spectrophotometer. Samples were then centrifuged at 20
000g for 40 minutes through Beckman Coulter Optima XPN-80 Ultra centrifuge to separate the nanoparticles from aqueous medium and then lyophilized at 0.05 psi and −50 °C through Christ, Alpha 1-2 LD plus lyophilizer. Powder XRD characterization was done using Bruker D2 phaser powder XRD instrument. The 2θ range used for the study was 20° to 80° with a step size of 0.3 seconds, to know the crystalline phase and its purity. FE-SEM imaging was done using Supra 55 Zeiss FE-SEM instrument, for confirmation of shape and approximate size of the particles. FTIR characterization of the samples were done using PerkinElmer, Spectral version 50.5.1 instrument to obtain the functional groups involved in the reduction process. TEM analysis was done using TEM-VM 200 kV for size estimation.
Antimicrobial assay
The synthesized nanoparticles of Ag/AgCl were observed for antimicrobial activity with varying concentrations along with a negative control in Bacillus subtilis, Bacillus sphaericus, Bacillus pasteurii (from NCIM, Pune) and E. coli-DH5α (in-house strain) at 37 °C in incubator. Bacterial species were diffused through nutrient broth media and agar of bacteriological grade, and biosynthesized nanoparticles were loaded in the solidified wells to check the antibacterial activity. 20 μl of Ag/AgCl nanoparticles synthesized from different concentrations of metallic precursors were taken and used for drug diffusion assay to observe zone of inhibition which pertains to the effect of the particles on growth of bacterial strains mentioned above.
Results and discussion
Size estimation using dynamic light scattering
The synthesized particles from extracts of different species were analysed for size estimation and shown in Fig. 1. This depicts real-time size monitoring of the nanoparticles synthesized from species which shows an average particle size of 90.6 nm and polydispersity index of 0.331 for Chlorella sp. whereas the size of nanoparticles synthesized by Lyngbya putealis and Scenedesmus vacuolatus shows an average size of 241.8 nm and 136.2 nm with a polydispersity index of 0.215 and 0.253, respectively. So, based on the size of the particles obtained, Chlorella sp. is selected for further optimization. DLS data shows smallest particle size in Chlorella sp. in comparison to other two. DLS data was used as an indicator of size for nanoparticles. Polydispersity of the particles was well in range.
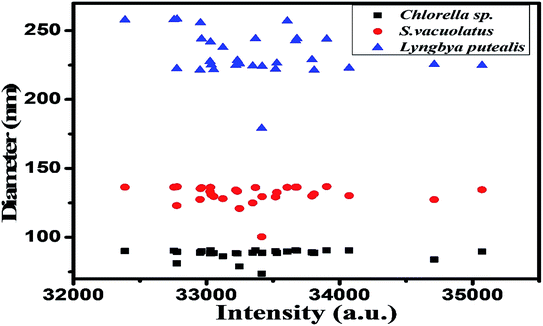 |
| Fig. 1 DLS analysis of particles prepared from different algal species. | |
UV-vis spectroscopy analysis of the varying concentration of metallic salts incubated with extract prepared from Chlorella sp. biomass
UV-visible data showed that extract prepared from Oocystis sp. was not able to reduce metallic precursor to metallic nanoparticles and gave an intense peak at approximately 660 nm. This indicates presence of pigments which is shown in Fig. 2. UV-visible and DLS data indicates Chlorella sp. best fit for our study. Hence further optimization of biosynthesis process was carried out with the extract prepared from 5 g biomass of Chlorella sp. with varying concentrations of AgNO3 (1 mM, 0.5 mM, 0.1 mM and 0.2 mM) as presented in Fig. 3(A)–(D). Fig. 3 shows a peak at approximately 420 nm indicating the formation of AgNPs. Particles synthesized from 1 mM concentration showed an additional peak at around 660 nm even after 192 h which may be due to impurity. Interestingly 0.5 mM concentration of metallic precursor showed negligible peak supporting usage of extract towards biosynthesis of particles. There are several reports of silver nanoparticles synthesis where surface plasmon resonance (SPR) was found to be at different wavelengths ranging from 410 nm to 460 nm.5,27 So, it was confirmed from the data that each concentration of metallic precursor can lead to production of nanoparticles but the stability and purity of the product depends on the concentration of precursor and extract.
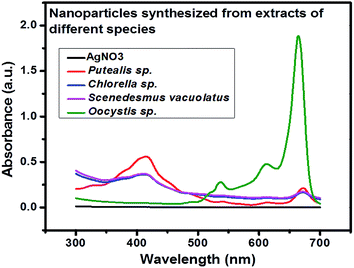 |
| Fig. 2 UV-visible spectroscopic study of Ag/AgCl particles synthesized from different algal species. | |
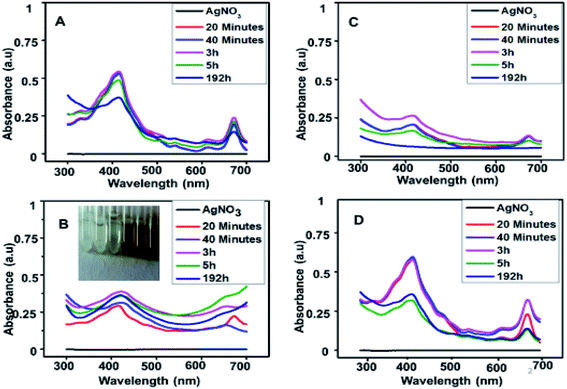 |
| Fig. 3 UV-visible spectra of nanoparticles synthesized with (A) 1 mM, (B) 0.5 mM, (C) 0.1 mM and (D) 0.2 mM AgNO3 with time. | |
It is observed from Fig. 3(A)–(D) that 1 mM and 0.2 mM concentrations show a peak at 420 nm as well as 660 nm indicates that the pigments and other reducing groups have not been utilized well in these samples. In 0.1 mM concentration, the peak at 420 nm indicates formation of AgNPs which found to be deteriorated with time, indicating that the product is not stable. In 0.5 mM concentration, the product remains stable even at 192 h. Therefore, it can be safely concluded that 0.5 mM is better than other concentrations to utilize the extract and form a stable product.
X-ray diffraction (XRD) analysis of biosynthesized nanoparticles
XRD was carried out at different concentrations of metallic precursors and the obtained data confirms formation of Ag/AgCl nanoparticles. XRD pattern was indexed using the JCPDS, file no. 31-1238 for AgCl and JCPDS file no. 65-2871 for Ag (Table 1).
Table 1 Crystalline planes of synthesized Ag/AgCl nanoparticles
2 Theta (°) |
Plane |
2 Theta (°) |
Plane |
27.89 |
(111) |
57.6 |
(222) |
32.4 |
(200) |
67.7 |
(400) |
46.4 |
(220) |
74.4 |
(331) |
55.1 |
(311) |
77.3 |
(420) |
The XRD pattern corresponds to the crystalline cubic phase of AgCl nanoparticles. The peak obtained at 38.3° corresponds to (111) plane of cubic Ag28 at 0.5 mM and 0.2 mM concentration of AgNO3 and extract of Chlorella sp. So, it was confirmed from the XRD data that the Ag/AgCl nanoparticles were successfully synthesized and the peaks for different concentration ranges can be seen in Fig. 4. The optimum result is given by 0.5 mM concentration of AgNO3 as along with diffraction lines of AgCl nanoparticles it also shows an intense diffraction peak of AgNPs at 38.3° which was not present in particles synthesized from any other concentrations except 0.2 mM. The peak at 38.3° was not as significant in 0.2 mM as in 0.5 mM concentration used for biosynthesis of Ag/AgCl nanoparticles. Therefore further analysis of this sample was done and considered to be better among other data sets as XRD gives information about the purity of the particles produced and 0.5 mM metallic precursor concentrations showed a significant and characteristic peak for Ag/AgCl nanoparticles, so it can be concluded that 0.5 mM concentration of AgNO3 is the best concentration to be used for nanoparticle synthesis. Nanoparticles synthesized from 0.1 mM was not taken into consideration for XRD as it was not stable which is evident from the UV-visible spectrophotometry data shown in Fig. 3(C). Presence of peaks for AgCl nanoparticles raised a curiosity that from where this chloride ion is coming as we have not added any external source for chlorine. We have washed the biomass well before utilizing it for extract preparation. The chloride ions are present inside the algal cells play a vital role in asparagine synthesis and thus providing an insight to metabolomics of nitrogen and its relationship with ion transport,29 however its exact estimation in the algal or plant cells remains a challenge.30 The powder XRD data which indicated synthesis of Ag/AgCl nano hybrids.
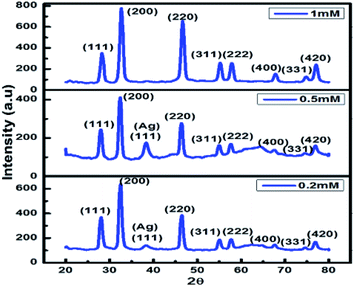 |
| Fig. 4 Shows the characteristic peaks of Ag/AgCl nanoparticles with varying AgNO3 concentration (0.2 to 1 mM) prepared using 5 g biomass extract of Chlorella sp. | |
Fig. 4 represents that 0.5 mM is the optimum concentration of metallic precursor to be used for Ag/AgCl nanoparticle biosynthesis.
Scanning electron microscopy (SEM)
The presence of spherical nanoparticles can be inferred from Fig. 5(A) and (B) as indicated by red arrows. These samples are further characterized by Transmission Electron Microscopy (TEM) for the size determination.
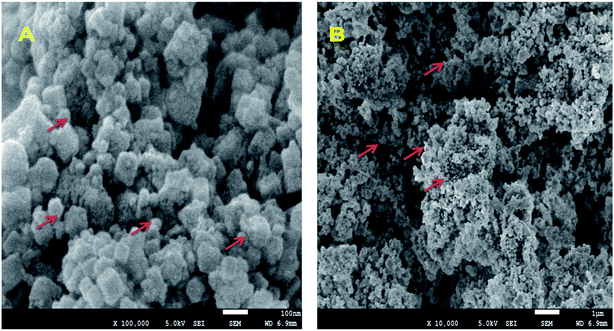 |
| Fig. 5 SEM image of nanoparticles synthesized using 0.5 mM AgNO3 (A) 100k× (B) 10k×. | |
FTIR (Fourier-transform infrared spectroscopy)
The lyophilized powder of Ag/AgCl nanoparticles synthesized with extract from Chlorella sp. biomass and 0.5 mM concentration of AgNO3. The biomass of Chlorella sp. was analysed with FTIR and the result obtained is shown in Fig. 6.
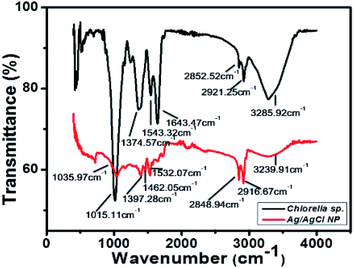 |
| Fig. 6 Ag/AgCl nanoparticles FTIR data analysis of biomass obtained from Chlorella sp. | |
The functional groups identified in the biomass of Chlorella sp. is described in Table 2. Functional groups like amides (N–H), lipid carbohydrates having mainly CH2 and CH3 stretching and (O–H) group can act as reducing groups and reduce the metallic ions in to metallic nanoparticles, i.e., Ag+ to Ag.31−37 All the groups present in the sample analyzed have been described in Table 2. The peak in Fig. 6 at 3239.91 cm−1, 2916.67 cm−1, 2848.94 cm−1, 1532.07 cm−1, 1462.05 cm−1 show the formation of nanoparticles. It shows the reduced value at these peaks corresponding to the algal biomass used for nanoparticle synthesis and showed following values at same peak positions 3285.92 cm−1, 2921.25 cm−1, 2852.52 cm−1, 1643.47 cm−1, 1543.32 cm−1 which indicates the utilization of this reducing groups in formation of nanoparticles and these peaks are also responsible for the antibacterial properties shown by the particles formed and represented in next section. There are several other peaks which may be due to the capping agents from the algal biomass present around the nanoparticles and stabilize the particles.38 Different functional groups help in reduction of metallic precursors to metallic nanoparticles and acts as capping agents to provide stability to the synthesized particles, in the present study it was found that lipids and proteins along with the stretching movements of (O–H) was more involved in the reduction process which lead to the formation of Ag/AgCl nanoparticles.
Table 2 Functional group analysis of Chlorella sp. and Ag/AgCl nanoparticles synthesized biomass through FTIR
Biomass wavenumber (cm−1) |
Ag/AgCl wavenumber (cm−1) |
Assigned functional groups |
1035.97 |
1015.11 |
Carbohydrate |
νs(C–O–C) of polysaccharides |
1374.57 |
1397.28 |
Protein |
δs(CH2) and δs (CH3) bending of methyl |
Carboxylic acid |
νs(C–O) of COO− group of carboxylates |
1543.32 |
1462.05 |
Protein |
δas(CH2) and δas (CH3) bending of methyl |
Lipid |
δas(CH2) bending of methyl |
Protein |
Amide II band |
Mainly δ(N–H) bending and ν(C–N) stretching |
1643.47 |
1532.07 |
Protein |
Amide II band |
Mainly δ(N–H) bending and ν(C–N) stretching |
Protein |
Amide I band mainly ν(C O) stretching |
2852.52 |
2848.94 |
Lipid and carbohydrate |
Mainly νas(CH2) and νs(CH2) stretching |
2921.25 |
2916.67 |
Lipid and carbohydrate mainly νas(CH2) and νs(CH2) stretching |
3285.92 |
3239.91 |
Water |
ν(O–H) stretching |
Transmission electron microscopy (TEM)
TEM analysis of particles synthesized using 0.5 mM silver nitrate concentration was done and spherical shape nanoparticles were observed. The histogram was prepared from the same image which shows the size of the particles obtained during TEM imaging to be 10–20 nm and shown in Fig. 7(A) and (B), respectively. The SAED pattern gave results corresponding to the XRD pattern observed and polycrystalline nature of the synthesized nanoparticles which can be inferred from the Fig. 7(C), same type of pattern was obtained by AgCl nanoparticles synthesized from Klebsiella planticola (MTCC 2277).39 Interplanar distance was calculated using the SAED pattern based on which we have given orientation planes to the rings present in SAED pattern.
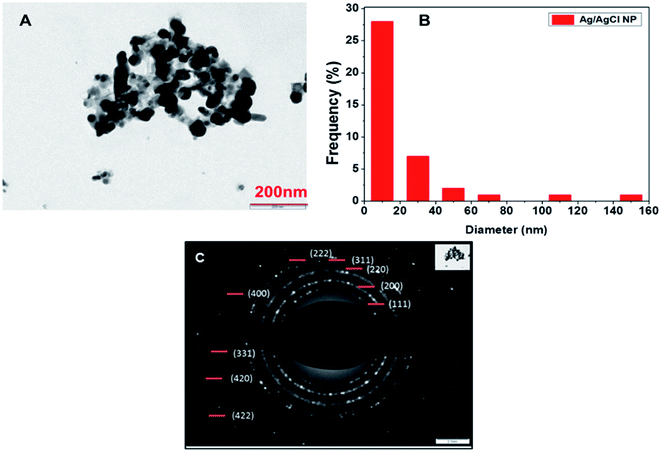 |
| Fig. 7 (A) TEM image of synthesized nanoparticles at 200 nm (B) histogram drawn from the particles image (C) SAED pattern of the particles. | |
Rings present in SAED pattern was used to assign planes to each ring based on the interplanar distances d = 0.314 (111) (AgCl), 0.286 (200) (AgCl), 0.236 (111) (Ag) 0.206 (400), 0.145 (220), 0.127 (331), 0.117 (222), and 0.165 (311).24,39,40 It also shows the polycrystalline nature of the Ag/AgCl nanoparticles. A bell-shaped histogram could not be obtained in our study as there were few particles having size in range of 140 to 160 nm and most of the particles fall in the range of 10–20 nm, this suggests that the particles formed were polydisperse in nature. TEM images and SAED pattern provides proof of nanoparticles synthesis and formation of a polycrystalline compound whose planes belongs to mixed phase of Ag/AgCl nanoparticles as confirmed by XRD and the calculations of interplanar distances.
Antimicrobial activity of the synthesized nanoparticles
The antimicrobial activity was tested through drug diffusion assay with nanoparticles synthesized from different concentrations of AgNO3 on bacterial strains, Bacillus subtilis, Bacillus sphaericus, Bacillus pasteurii and E. coli (DH5-α) as shown in Fig. 8(A)–(D). Balaz et al. have studied at higher concentrations of metallic precursors from 1 mM to 10 mM to synthesize AgNPs and zone of inhibition was not observed even at 1 mM for S. aureus and S. typhimurium.40 In present case, zone of inhibition was found with nanoparticles synthesized from 0.5 mM and 1 mM for B. subtilis and E. coli. This suggests that 0.5 mM can be used to synthesize Ag/AgCl nanoparticles and possess antibacterial effect. Similar studies have been done for green synthesis of Ag/AgCl nanoparticles through extract prepared from seeds of Conium maculate and tested antibacterial activity on L. monocytogenes 1298. Successful results have been obtained at 300 ppm
10 which shows our study fits in line to the previous results obtained by other researchers. This study provides an insight on how concentration of metallic precursor can modulate the antibacterial properties of the synthesized nanoparticles. Cellulose, Ag and AgCl hybrids have also been studied in order to check its antibacterial candidature and obtained a zone of inhibition up to 2 mm in case of E. coli whereas present study found 7.6 ± 0.1 mm zone of inhibition for E. coli which is approximately 4 folds higher than reported by Dong et al.41 Thus Ag/AgCl nano hybrids can be used as antibacterial agents widely. The zone of inhibition was visible in all concentrations as shown in Table 3. However, the nanoparticles synthesized with 0.5 and 1 mM metallic precursor concentration showed better zone of inhibition in comparison to others and restricted growth of bacteria to almost equal extent. This further signifies that the 0.5 mM concentration of silver nitrate is optimum for synthesis of Ag/AgCl.
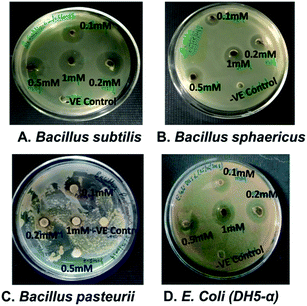 |
| Fig. 8 Zone of inhibition after treatment by Ag/AgCl nanoparticles synthesized using different concentrations of AgNO3 (0.1 mM, 0.2 mM, 0.5 mM and 1 mM) for (A) Bacillus subtilis (B) Bacillus sphaericus (C) Bacillus pasteurii and (D) E. coli (DH5-α). | |
Table 3 Antibacterial activity of Ag/AgCl particles showing zone of inhibition obtained in different bacterial strains
Concentration of AgNO3 (mM) used for nanoparticle synthesis |
Zone of inhibition (mm) |
B. sphaericus |
B. subtilis |
B. pasteurii |
E. coli (DH5-α) |
0.1 |
1.1 ± 0.2 |
1.8 ± 0.2 |
2.08 ± 0.1 |
1.6 ± 0.1 |
0.2 |
2.6 ± 0.2 |
2.9 ± 0.1 |
0 |
2.6 ± 0.1 |
0.5 |
4.91 ± 0.1 |
8.6 ± 0.2 |
11.25 ± 0.1 |
7.6 ± 0.1 |
1 |
4.84 ± 0.1 |
7.5 ± 0.2 |
0 |
7.5 ± 0.2 |
To test the significance of the data, two-way ANOVA was done. P-value (0.007) was less than 0.05, indicates the data is indeed significant.
Conclusions
Novel Ag/AgCl nano hybrids of 10–20 nm were successfully synthesized through extract of Chlorella sp. The process for nanoparticle biosynthesis was optimized and found that 0.5 mM AgNO3 was best concentration of metallic precursor. This study signifies that process of biosynthesis should be optimized for each algal species, since they have different reducing groups controlling the process and stability of particles. Synthesized particles have been further tested as an antimicrobial agent against E. coli (DH5-α), Bacillus subtilis, Bacillus sphaericus and Bacillus pasteurii strains. Significant P value of 0.007 was found for zone of inhibition obtained with different sets of bacterial strains treated with nanoparticles, further proving the antimicrobial effect of synthesized particles.
Conflicts of interest
There are no conflicts to declare.
Acknowledgements
The authors acknowledge funding support provided by DST-ECR project (ECR/2017/001567) and UGC, New Delhi. Funding organization has not played any role in study design, decision to publish or preparation of the manuscript. Authors are thankful to Prof. Pradeep Mathur, Director, IIT Indore, for encouraging research and providing necessary facilities. Authors are also thankful to sophisticated Instruments Facility (SIC), IIT Indore for facilities provided.
References
- A. Ahmad, P. Mukherjee, S. Senapati, D. Mandal, M. I. Khan and R. Kumar, Colloids Surf., B, 2003, 28(4), 313 CrossRef CAS.
- J. Jena, N. Pradhan, R. R. Nayak, B. P. Dash, L. B. Sukla and P. K. Panda, J. Microbiol. Biotechnol., 2014, 24(4), 522 CrossRef CAS PubMed.
- S. Otari, R. Patil, N. Nadaf, S. Ghosh and S. Pawar, Mater. Lett., 2012, 72, 92 CrossRef CAS.
- M. Hosea, B. Greene, R. Mcpherson, M. Henzl, M. D. Alexander and D. W. Darnall, Inorg. Chim. Acta, 1986, 123(3), 161 CrossRef CAS.
- S. S. Shankar, A. Rai, A. Ahmad and M. Sastry, J. Colloid Interface Sci., 2004, 275(2), 496 CrossRef CAS PubMed.
- S. N. Sinha, D. Paul, N. Halder, D. Sengupta and S. K. Patra, Appl. Nanosci., 2015, 5(6), 703 CrossRef CAS.
- M. Yousefzadi, Z. Rahimi and V. Ghafori, Mater. Lett., 2014, 137, 1 CrossRef CAS.
- V. da Silva Ferreira, M. E. ConzFerreira, L. M. Lima, S. Frasés, W. de Souza and C. Sant'Anna, Enzyme Microb. Technol., 2017, 97, 114 CrossRef CAS PubMed.
- M. Villanueva-Ibáñez, M. Yañez-Cruz, R. Álvarez-García and M. Hernández-Pérez, Mater. Lett., 2015, 152, 166 CrossRef.
- H. Alishah, S. Pourseyedi, S. E. Mahani and S. Y. Ebrahimipour, Extract-mediated synthesis of Ag@AgCl nanoparticles using Conium maculatum seeds: characterization, antibacterial activity and cytotoxicity effect against MCF-7 cell line, RSC Adv., 2016, 6(77), 73197–73202 RSC.
- C. An, S. Peng and Y. Sun, Facile synthesis of sunlight-driven AgCl: Ag plasmonic nanophotocatalyst, Adv. Mater., 2010, 22(23), 2570–2574 CrossRef CAS PubMed.
- V. Patel, D. Berthold, P. Puranik and M. Gantar, Biotechnology Reports, 2015, 5, 112 CrossRef PubMed.
- P. Agarwal, R. Gupta and N. Agarwal, J. Nanotechnol., 2019, 1, 7392713 Search PubMed.
- G. Gahlawat and A. R. Choudhury, RSC Adv., 2019, 9(23), 2944 RSC.
- M. A. Borowitzka, J. Appl. Phycol., 2013, 25(3), 743–756 CrossRef CAS.
- Y. Chisti, Biotechnol. Adv., 2007, 25(3), 294 CrossRef CAS PubMed.
- L. Christenson and R. Sims, Biotechnol. Adv., 2011, 29(6), 686 CrossRef CAS PubMed.
- G. Singaravelu, J. S. Arockiamary, V. G. Kumar and K. A. Govindaraju, Colloids Surf., B, 2007, 57, 97 CrossRef CAS PubMed.
- S. Leclerc and K. J. Wilkinson, Environ. Sci. Technol., 2013, 48(1), 358 CrossRef PubMed.
- S. A. Dahoumane, C. Yéprémian, C. Djédiat, A. Couté, F. Fiévet, T. Coradin and R. A. Brayner, J. Nanopart. Res., 2014 Oct 1, 16(10), 2607 Search PubMed.
- Y. N. Mata, E. Torres, M. L. Blazquez, A. Ballester, F. M. González and J. A. Munoz, J. Hazard. Mater., 2009, 166(2–3), 612–618 CrossRef CAS PubMed.
- H. Ding, H. Li, X. Wang, Y. Zhou, Z. Li, J. K. Hiltunen, J. Shen and Z. Chen, Chem. Mater., 2017, 29(19), 8440–8448 CrossRef CAS.
- D. Han, H. Li., X. Wang, Y. Zhou, Z. Li, J. Kalervo Hiltunen, J. Shen and Z. Chen, Chem. Mater., 2017, 29(19), 8440–8448 CrossRef.
- J. Li, X. Tian, M. Habasi, K. Chen, N. Pang and P. Hu, CrystEngComm, 2012, 14(22), 7621 RSC.
- R. Y. Stanier, R. Kunisawa, M. Mandel and G. Cohen-Bazire, Bacteriol. Rev., 1971, 35(2), 171 CAS.
- I. Ibraheem, B. Abd-Elaziz, W. Saad and W. Fathy, J. Nanomed. Nanotechnol., 2016, 7(409), 2 Search PubMed.
- V. S. Ramkumar, A. Pugazhendhi, P. Sivagurunathan and G. D. Saratale, Biotechnology Reports, 2017, 14, 1–7 CrossRef PubMed.
- S. E. Rognes, Phytochemistry, 1980, 19(11), 2287 CrossRef CAS.
- J. A. Raven, J. Exp. Bot., 2016, 68(3), 359 Search PubMed.
- D. Y. Duygu, A. U. Udoh, T. B. Ozer, A. Akbulut, I. A. Erkaya and K. Yildiz, Afr. J. Biotechnol., 2012, 11(16), 3817 CAS.
- L. G. Benning, V. Phoenix, N. Yee and M. Tobin, Geochim. Cosmochim. Acta, 2004, 68(4), 729 CrossRef CAS.
- M. Giordano, M. Kansiz, P. Heraud, J. Beardall, B. Wood and D. McNaughton, J. Appl. Phycol., 2001, 37(2), 271 CrossRef CAS.
- A. Dean, M. C. Martin and D. Sigee, Phycologia, 2007, 46(2), 151 CrossRef.
- M. N. Afsar and J. B. Hasted, Infrared Phys., 1978, 18, 835 CrossRef CAS.
- R. Keller, The sigma library of FTIR spectra, Sigma Chemical Company, St. Louis, Mo, 1986, vol. 1, p. 2894 Search PubMed.
- A. P. Dean and D. C. Sigee, Eur. J. Phycol., 2006, 41(2), 201 CrossRef CAS.
- S. Al Rashed, S. Al Shehri and N. M. Moubayed, Biomed. Res., 2018, 29(13), 2859 CrossRef CAS.
- K. Paulkumar, S. Rajeshkumar, G. Gnanajobitha, M. Vanaja, C. Malarkodi and G. Annadurai, International Journal of Green Chemistry and Bioprocess, 2013, 3(1), 12 Search PubMed.
- C. Zhang, H. Hua, J. Liu, X. Han, Q. Liu and Z. Wei, Nano-Micro Lett., 2017, 9(4), 49 CrossRef PubMed.
- M. Balaz, L. Balazova, M. Kovacova, N. Daneu, A. Salayova and Z. Bedlovicova, Adv. Nano Res., 2019, 7(2), 125 Search PubMed.
- Y. Y. Dong, F. Deng, J. J. Zhao, J. He, M.-G. Ma and F. Xu, Carbohydr. Polym., 2014, 99, 166 CrossRef CAS PubMed.
|
This journal is © The Royal Society of Chemistry 2019 |
Click here to see how this site uses Cookies. View our privacy policy here.