DOI:
10.1039/C9RA00645A
(Paper)
RSC Adv., 2019,
9, 11602-11613
Impact of asphalt aging temperature on chemo-mechanics
Received
24th January 2019
, Accepted 2nd April 2019
First published on 12th April 2019
Abstract
As the use of warm and cold asphalt mixing technologies provides an opportunity to save energy during production, it is important to determine if this lower mixing temperature also has a long-term effect on the binder chemical and rheological properties and performance. In this study, a link between the chemistry and rheology of bituminous binders with a focus on short-term aging temperature is proposed. This link is made using a rheological aging index (RAI), the crossover temperature and a chemical aging index (CAI). The RAI is calculated using the difference in the integration areas under shear modulus master curves generated from Dynamic Shear Rheometer (DSR) data on unaged and aged bitumen. The cross over temperature is defined as that when the material transitions from elastic to viscous behaviour. The CAI is obtained from Fourier-transform infrared spectroscopy (FTIR) measurements by combining the carbonyl and sulfoxide indices. In addition, the effect of aging on the molecular size distribution of the binders was evaluated using Gel Permeation Chromatography (GPC). Two asphalt binders from two sources at two RTFOT aging temperatures 123 °C and 163 °C corresponding to warm mixing and hot mix mixing temperatures respectively were used. The rheological aging index, the chemical aging index and GPC delivered the same trends, showing that the short-term aging temperature has a significant effect on long-term chemical and rheological properties. The extent of this depends on the source as some binders were identified as being more aging resistant.
1. Introduction and motivation
Bituminous binders age during their service life. This is due to different processes such as oxidation and changes in molecular organisation.1–4 There are primarily two stages to aging of asphalt concrete: short-term aging that occurs during the fabrication process in the asphalt mixing plant and long-term aging that occurs during the pavement's service life. The aging process affects the mechanical performance negatively in terms of cracking and fatigue resistances and ideally reducing or retarding the aging process is desired. Understanding the behaviour of the material after it goes through the aging process is important as long-term performance and durability is a priority in designing this construction material. Bitumen aging can be characterised chemically and physically. The chemical characterisation of aging due to oxidation has been successfully done using Fourier Transform Infrared Spectroscopy (FTIR). Oxidative aging results in an increase in the carbonyl and sulfoxide indexes.4,5 Furthermore, the aging manifests itself into stiffening and more elastic behaviour, thus increased brittleness of the binder. This aspect can be characterised using rheological tests such as with the dynamic shear rheometer (DSR).6 Loeber et al.7 have shown that temperature has a strong influence on the rheological properties of bitumen governed by the individual constituents, which can change with aging. It remains to be seen if manufacturing temperature has a direct effect on the short and long term aging of bitumen. Therefore, the question remains whether or not the warm mix technologies that reduce mixing temperatures, have a positive effect on bitumen properties after long-term aging. However, current research results addressing the lower production temperatures, which lead to less oxidation during short-term ageing and also affect long-term ageing of binders and asphalt mixtures are not conclusive.8 Combining the chemical characteristics as a result of aging with the physical ones can provide a better understanding of the aging process.9,10
As the use of warm and cold mixing technologies provides an opportunity to save energy and reduce emissions during production,11 the goal of this research was to determine if this lower mixing temperature also has an effect on long term performance of the binders. As part of this inter-laboratory study the analysis of conventional properties with penetration value at 25 °C and softening point temperature has shown a difference in properties after short term aging with different short-term ageing temperatures. This difference vanishes for the penetration value and to a lesser degree for the softening point temperature after long-term laboratory ageing.13 As opposed to conventional properties, rheological analyses allow investigations into the properties over a wide range of temperatures and frequencies and thereby allowing a more holistic evaluation of the materials' behaviour. To this end, the development of complex shear modulus and phase angle confirm initial trends from the conventional properties of penetration and softening point. The results showed, a more significant increase in complex modulus at higher testing temperatures, corresponding to low frequencies. After long-term aging a significant reduction in the phase angle was seen. The overall results showed that lower short-term ageing temperatures have a lower impact on binder properties. However, after long term ageing, the differences in properties seen for short-term ageing vanish.12,13
The goal of this study was to understand the effect of warm mix technologies on aging, in order to contribute to better understanding of the overall benefits of warm mix technologies. Specifically, to investigate the long term effect of short term aging temperature by linking the chemistry to the physical properties of bituminous binders. To this end, a rheological aging index (RAI) based on differences in integration areas under master curves of unaged and aged bitumen from DSR was used.15 The cross-over parameters were also used to characterise the aging affect, these parameters on both temperature and shear complex modulus indicates the transition between a more predominantly elastic behaviour and more predominantly viscous behaviour.16,17 In addition, a chemical aging index (CAI) is used by combining the carbonyl and sulfoxide indices.14 This is part of the results of an inter-laboratory study with 12 participating laboratories on four binders from four different crude sources. The current paper focuses on data from one laboratory, two binders and two temperatures; 123 °C and 163 °C. Additional results addressing other aspects of the this research can be found in previous publications.12,13,19–21
2. Materials
In the wider scope of the inter-laboratory study four 70/100 pen bituminous binders, from different sources were used. These were labelled B501, B502, B503, and B504. Through preliminary technological tests such as penetration and softening point, two families of binders were identified. This paper reports on the results of binders B502 and B504 as representative of these two families of binders. In addition, suffixes, B_Temp and C_Temp are used to give information on the state of ageing condition; original, after RTFOT, and after RTFOT + PAV, and the ageing temperature (Temp) as detailed in the following section.
3. Methods
The binders were first short-term aged with the rolling thin film oven test (RTFOT)23 (state of aging B) at two different temperatures (Temp), 163 °C and 123 °C, corresponding to the following suffix labelling, B_163 and B_123, respectively. Next, the same binders were additionally long-term aged with the Pressure Ageing Vessel (PAV)24,33 (state of aging C) and further identified with suffixes C_163 and C_123. The virgin, short-term and long-term aged samples were rheologically and chemically characterised. For the rheological testing, the DSR was used,22 Fourier-transform infrared spectroscopy (FTIR) was selected to evaluate ageing from a chemical viewpoint.4,5,18 In addition, conventional material characterisation techniques, such as penetration value at 25 °C and softening point temperature were used according to European Standards25,26 as well as gel permeation chromatography.27
3.1 Aging
Short-term ageing was simulated using the rolling thin film oven test RTFOT following the European standard.23 In this study, the test was carried out for 75 min and at two different temperatures 163 °C and 123 °C which covers temperatures commonly used for hot and warm applications.28,29
Long-term ageing was simulated using the Pressure Ageing Vessel (PAV) test.24 This procedure is conventionally assumed to reproduce the ageing occurring during the pavement service life. In this study, the test was carried out in accordance with EN 14769 after RTFOT with the above mentioned two temperatures. Samples were tested at a temperature of 100 °C and an air pressure of 2.1 MPa for 20 hours.
3.2 DSR
DSR22 was used to obtain the rheological properties of the asphalt binders after being subjected to the different aging conditions. Amplitude sweep tests were initially performed to determine the strain level to be imposed during the temperature–frequency sweep tests in order to remain within the linear viscoelastic domain. Complex shear modulus |G*| and phase angle δ, were measured over a wide range of temperatures and frequencies. A parallel-plate geometry with an 8 mm diameter and 2 mm gap was used for temperatures between −10 °C and +30 °C (with imposed controlled strain of 0.1%) while a 25 mm diameter and 1 mm gap was selected for temperatures between +30 °C to +80 °C (at controlled strain of 1%). Tests were conducted with temperature steps of 10 °C and a frequency sweep from 0.1 Hz to 10 Hz at each temperature. A minimum of two replicates (up to a maximum of five) per testing condition was used during the experimentation.
Christensen–Anderson–Marasteanu (CAM) model30,31 was used to generate the master curves for complex modulus, |G*|, and phase angle, δ:
|
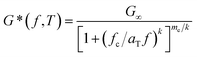 | (1) |
|
 | (2) |
where,
f is the frequency (Hz);
fc is the location parameter;
aT is the horizontal shift factor at temperature
T′,
G∞ is the glassy shear modulus when frequency tends to infinity;
k,
me are dimensionless shape parameter. Shift factors,
aT, were derived from the well-known Williams–Landel–Ferry (WLF) expression
32 using the time temperature superposition principle:
|
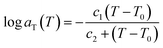 | (3) |
where,
T0 is the reference temperature equal to 20 °C in the present paper;
c1 is a constant;
c2 is a temperature constant.
3. 2. 1 Rheological aging index (RAI). As can be seen from a representative master curve shown in Fig. 1, the behaviour of the binder is temperature and frequency dependent. Following the method developed by Cavalli et al.15 a rheological ageing index (RAI) was defined to incorporate the changes over a large frequency range by calculating the difference between the area under the aged and unaged master curves within a definite range of reduced frequencies chosen to ensure that the same integral limits for all calculations according to equation4 is used. Hence, the following equation holds: |
 | (4) |
where |G*| is the complex modulus and ξ is the logarithm of the reduced frequency between the integral limits. Thus, the result of the integral, which is the area between the two master curves in double logarithmic scale, was subsequently calculated, as shown in the example of Fig. 1.
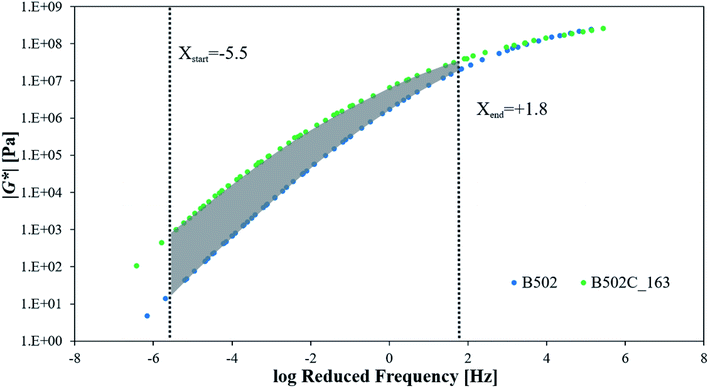 |
| Fig. 1 Principle of the aging index calculated using the area between the aged and unaged master curve at a reference temperature of 20 °C. | |
3.3 GPC
Gel permeation chromatography (GPC) is a technique to separate macro-molecules from each other and thereby it is a powerful tool to analyse the size of the molecules in a substance. In the scope of this work GPC was used to determine the length of the bitumen polymer chains and thereby determine whether any chemical reaction took place during the aging process. The experimental procedure is as follows. The sample is dissolved in a suitable organic solvent, in this case THF. The resulting emulsion is then poured through a filter-like device, called the column, to the detector where the data is recorded. The column consists of a gel containing stacked multi-porous polymers that act as a filter. The porous gel network contains holes of different diameter. The smaller particles can go through the gel pores while larger particles cannot. Thus, the smaller particles must traverse a larger volume and therefore elute later. The large molecules cannot enter the gel and elute earlier. The output is attained through the UV detector in this case measuring a signal which is proportional to the concentration of the elute. The device is calibrated using a calibration substance. As a result, relative molar masses in comparison to the standard substance are obtained. This technique allows quantifying the molar mass of the sample as a function of the quantity, thus it generates a distribution curve of the molecular sizes contained in the substance. The parameters used in this experiment were: sample concentration of 1.0 mg ml−1 (155 mg/30 ml THF and then 1
:
5 diluted in THF); injection volume: 50 μl; equipment: HPLC-Pump Agilent G1310B/1260 Iso Pump; Auto sampler Agilent 1200 Series, Instant Pilot G4208A; Column oven SFD 12590; Variable Wavelength Detector Agilent 1260 (DEABB05519); eluent solvent: THF high purity (ROMIL, GB), 1.0 ml min−1; GPC column: 1× Agilent PL 5M-mixed C Guard, 5 μm, 50 × 7.5 mm; 2× Agilent PL 5M-mixed C, 5 μm, 300 × 7.5 mm; oven temperature: 30 °C; UV: wave range 215 nm; software: Agilent GPC/SEC G7850 AA/GB13470039; sampling rate: 9 s−1.
3.4 FTIR
Infrared spectrometry was used to characterise the change in the chemical structure of bitumen as a result of aging. Fourier Transform Infra-Red (FTIR) Spectroscopy is an analytical tool which characterises the different functional groups of chemical components. The test has been performed in the range of 4000 cm−1 to 400 cm−1 wave numbers. Oxidation of bitumen typically shows up as changes in carbonyl and sulfoxide peaks around 1700 cm−1 and 1030 cm−1 wave numbers respectively. Petersen reported that during oxidation sulfoxide peaks occur first and then the S
O bonds are changed further and a decrease of sulfoxide is observed.1 The carbonyl peaks take longer to develop, i.e. after more aging. As a result, sulfoxide can be used as indicator of short term aging and both carbonyl + sulfoxide index used as overall oxidation index. Carbonyl bonds encompass various species including acid or ester, which are not necessarily indication of oxidation. Changes in carbonyl and sulfoxide peaks are compared relatively to those chemical bonds that are not affected by aging such as aliphatic groups to determine the carbonyl (ICO) and sulfoxide (ISO) indexes. In this study the area under the curve, after base line correction, was used to calculate the ICO and ISO indexes as shown in equations5 and:6 |
 | (5) |
|
 | (6) |
where the integration limits for the areas were: carbonyl 1660 cm−1 to 1720 cm−1, sulfoxide 995 cm−1 to 1047 cm−1, reference (aliphatic) 1350 cm−1 to 1395 cm−1 and to 1525 cm−1.
3.4.1 Chemical aging index. The sum of both indices (ICO + ISO) was used to determine the chemical aging index (CAI) before and after aging as previously proposed.14 Thus, the chemical aging index is defined as:
4. Results
4.1 Physical properties
The technological test results of penetration and softening point for binders B502 and B504 in virgin state and after short term and long term aging are reported in Table 1. It can be seen that the difference in penetration and softening point between the two binders seen in virgin state vanishes after long term aging.
Table 1 Penetration and softening point values
|
Designation |
Pen, [0.1 mm] |
R&B, °C |
Virgin |
B502 |
71 |
48 |
B504 |
81 |
46 |
Short term 123C |
B502B_123 |
57 |
51 |
B504B_123 |
68 |
48 |
Short term 163C |
B502B_163 |
45 |
54 |
B504B_163 |
52 |
52 |
Long term 123C |
B502C_123 |
31 |
62 |
B504C_123 |
33 |
57 |
Long term 163C |
B502C_163 |
31 |
62 |
B504C_163 |
30 |
60 |
The fitting parameters of the CAM model (eqn (1) and (2)) and WLF (eqn (3)) for asphalt binder B502 and B504 are calculated and shown in Table 2.
Table 2 The fitting parameters of the CAM model and WLF equation for asphalt binder B502 and B504
Asphalt binder |
G0 (MPa) |
G∞ (MPa) |
fc |
k |
me |
c1 |
c2 |
R2 |
B502 |
0 |
850 |
24.45 |
0.18 |
1.08 |
16.02 |
141.75 |
1.000 |
B502B_123 |
0 |
850 |
11.26 |
0.17 |
1.09 |
17.63 |
152.15 |
1.000 |
B502B_163 |
0 |
850 |
2.79 |
0.16 |
1.12 |
18.16 |
154.05 |
1.000 |
B502C_123 |
0 |
1000 |
0.03 |
0.12 |
1.26 |
20.90 |
168.54 |
1.000 |
B502C_163 |
0 |
1000 |
0.01 |
0.12 |
1.30 |
21.06 |
168.09 |
1.000 |
B504 |
0 |
850 |
146.23 |
0.21 |
1.03 |
13.36 |
123.13 |
1.000 |
B504B_123 |
0 |
850 |
93.38 |
0.20 |
1.04 |
13.76 |
125.12 |
1.000 |
B504B_163 |
0 |
850 |
31.87 |
0.18 |
1.06 |
14.49 |
130.31 |
1.000 |
B504C_123 |
0 |
1000 |
25.23 |
0.18 |
1.08 |
14.11 |
118.10 |
1.000 |
B504C_163 |
0 |
1000 |
5.51 |
0.17 |
1.08 |
15.46 |
134.23 |
1.000 |
Master curves of |G*| vs. reduced frequency were built using these models. The results at a reference temperature of 20 °C for binder B502 and B504 in virgin state, after RTFOT with 123 °C and 163 °C and after RTFOT and consequent PAV aging are shown in Fig. 2 and 3, respectively. It can be seen that the norm of the complex modulus |G*| increases after RTFOT aging, with aging temperature of 163 °C having a larger influence on the increase of |G*|. This increase is significantly higher in comparison to the virgin binder and depends on the bitumen source. Considering a reference temperature of 20 °C and a conventional frequency f = 1.59 Hz, (10 rad s−1) it can be observed that such a stiffening effect for binder B502 with the RTFOT temperature of 163 °C is 65% and, in the case of RTFOT temperature of 123 °C, 21%. This shows that RTFOT temperature has an impact on the complex modulus. After subsequent PAV aging the difference is less significant 250% vs. 211% respectively. A similar trend is seen with binder B504 with a corresponding increase in |G*| in comparison to virgin state of 56% and 15% at 163 °C and 123 °C, respectively for short-term aging with RTFOT. This corresponds to an increase of shear modulus of 247% and 163% after long-term aging with PAV. The results show that depending on the binder source aging sensitivity can differ significantly.
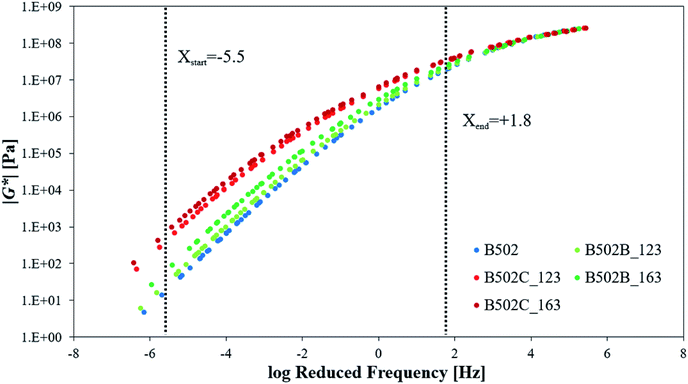 |
| Fig. 2 Master curves of |G*| vs. reduced frequency at a reference temperature of 20 °C for binder B502 in virgin state, after RTFOT at 123 °C, 163 °C (condition B) and consequent PAV aging (condition C). | |
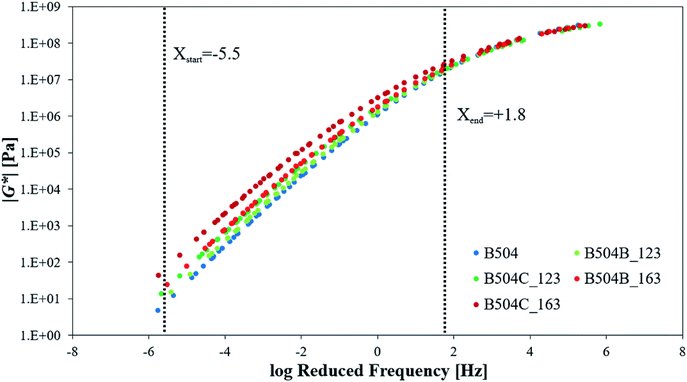 |
| Fig. 3 Master curves of |G*| vs. reduced frequency at a reference temperature of 20 °C for binder B504 in virgin state, after RTFOT at 123 °C, 163 °C (condition B) and consequent PAV aging (condition C). | |
Comparing Fig. 2 and 3 it becomes immediately apparent that the temperature (or aging) sensitivity is very much dependent on the frequency considered therefore if one uses a single frequency as sometimes practiced for comparison of binders, then the total picture can be missed. For example the highest difference is seen in the low frequency/high temperature domain, whereas the difference in the moduli vanishes at the high frequency/low temperature domain. For exactly this reason a wide range of frequencies and temperatures are used in the calculation of the RAI, and therefore the area difference under the master curves. The difference is apparent to the naked eye even without any calculations as the curves are spread apart in the case of B502 Fig. 2 and closer in the case of B504 Fig. 3.
Using the area under the master curves, an aging index (RAI) was defined as outlined in the previous section. This aging index quantifies the change in the rheological properties of the binder over a wide spectrum of frequencies and temperatures. In the present study, as shown in eqn (4), the integration variable, and therefore the reduced frequency range, is between −5.5 and +1.8. Tables 3 and 4 report the RAI calculated for binders B502 and B504, respectively. It can be observed that the RTFOT aging temperature has a significant effect on the rheological aging of the binders. For example, in case of B502 this effect can be seen when comparing RAI of 6.86 × 106 when RTFOT temperature of 163 °C is used vs. 2.28 × 106 when RTFOT of 123 °C is used. This leads to a relatively smaller difference after PAV aging for which a corresponding RAI of 23.82 × 106 and 20.63 × 106 is computed. The aging procedure affected the rheological properties of binder B504 less in comparison to B502 indicating that this binder may be less aging susceptible. This was true in all aging stages but more prominent when comparing the coupling effect of RTFOT (at 163 °C) + PAV which results in an RAI of 23.82 × 106 vs. 12.38 × 106 for binder B502 and B504, respectively.
Table 3 Rheological aging index (RAI) for binder B502
Asphalt binder |
Area under the master curve |
Area between virgin and aged binder (rheological aging index – RAI) |
% of virgin area |
B502 |
1.67 × 107 |
— |
|
B502B_123 |
1.90 × 107 |
2.28 × 106 |
13.7 |
B502B_163 |
2.35 × 107 |
6.86 × 106 |
41.2 |
B502C_123 |
3.73 × 107 |
20.63 × 106 |
124.0 |
B502C_163 |
4.05 × 107 |
23.82 × 106 |
143.0 |
Table 4 Rheological aging index (RAI) for binder B504
Asphalt binder |
Area under the master curve |
Area between virgin and aged binder (rheological aging index – RAI) |
% of virgin area |
B504 |
1.36 × 107 |
— |
|
B504B_123 |
1.48 × 107 |
1.19 × 106 |
8.74 |
B504B_163 |
1.78 × 107 |
4.23 × 106 |
31.1 |
B504C_123 |
1.79 × 107 |
4.28 × 106 |
31.4 |
B504C_163 |
2.60 × 107 |
12.38 × 106 |
91.0 |
In addition to RAI, the crossover temperature was computed for the entire set of binders under the five different aging conditions. Storage modulus (G′) and the loss modulus (G′′) can be plotted vs. temperature at one frequency. At low temperatures the material is more elastic therefore G′ > G′′. As the temperature increases at phase angle of 45° G′ = G′′. After this point as the temperature increases G′ < G′′ and the material behavior more viscous. This cross over parameter, defined as the temperature when G′′ is equal to G′ increases for more severe aging as a result of hardening effects.16 In the present study, a conventional reference frequency of 1.59 Hz (10 rad s−1) was set for calculation purposes. An increase of the crossover temperature indicates that the elastic part of the complex modulus is dominant up to higher temperatures. Fig. 4 shows the evolution of the crossover temperature with aging for binders B502 and B504.
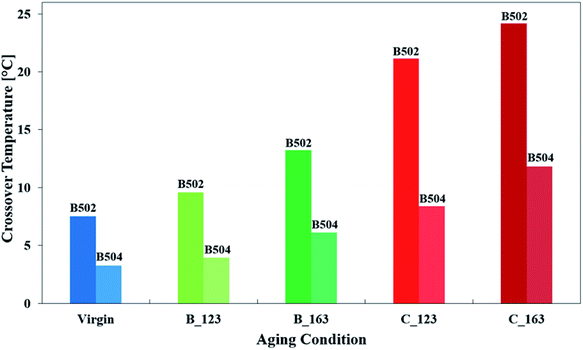 |
| Fig. 4 Cross over temperature of B502 and B504 at various aging states. | |
The bar chart shown in Fig. 4 indicates that there is an increase of the crossover temperature with aging. In addition it corroborates the findings based on the RAI supporting the hypothesis that binder B504 is less age susceptible with lower crossover temperature changes in comparison to binder B502 in all aging states. For example at the maximum aging state of C_163 which is after RTFOT at 163 °C and PAV the crossover temperature of B504 is 11.81C whereas that of B502 is 24.16C. This shows that in comparison to virgin state with the crossover temperature of 3.26 and 7.52, B502 shows a greater increase in the crossover temperature parameter. As shown in Fig. 5, there is a good trend between the crossover temperature and the complex modulus at all aging states showing that materials with a higher crossover temperature display a stiffer behaviour with an exponential trend. The trend line for the virgin binders is shown here only for the purpose of comparison with the other two trend lines, indicating a small change in the exponent in the case of RTFOT aging and a decrease of one order of magnitude in case of PAV aging.
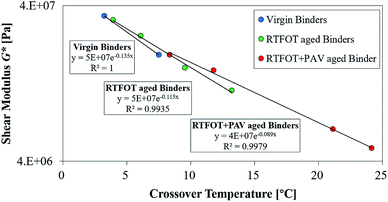 |
| Fig. 5 Relationship between crossover temperature and complex modulus at conventional reference frequency of 1.59 Hz (10 rad s−1) for binders B502 and B504. | |
4.2 Chemical properties
4.2.1 GPC. The results of the GPC analysis for two representative types of bitumen within the larger scope of the study B501 and B504 are shown in Fig. 6 and 7. The figures show the change in the UV signal over time reflecting the size of the molecules at the investigated stages; namely at virgin, stage B and stage C. It is apparent from both figures that there is a change in the molecular size distribution after aging. This shift towards larger molecular sizes is more severe with increase in temperature (123 vs. 163) and aging stage (RTFOT vs. RTFOT + PAV). Generally, the reason for GPC curve shifts can be twofold: (1) the bitumen has aged and therefore oxidized or (2) the bonds were damaged through a chemical or physical event. It was shown by Wang et al. that in the case the binder is unmodified, the molecules of the asphalt gradually transform into larger molecules during aging.27 When the binder is SBS modified, two peaks are observed, one for the modifier which has bigger molecular weight and one for the smaller molecular substances in asphalt. Fig. 6 and 7 display a large peak attributed to bitumen and a small shoulder whereby the large peak is broader for B504. Due to aging the shoulder increases in intensity indicating the gradual transformation into larger molecules as indicated above. The two binders clearly react differently to aging; B501 does not shown a clear difference between virgin and RTFOT at 123 whereas B504 already shows a distinct increase in the shoulder after the first aging state. These results corroborate other findings in this study that the aging susceptibility can vary based on bitumen origin. In the present case the GPC results indicate that B504 was more aging susceptible.
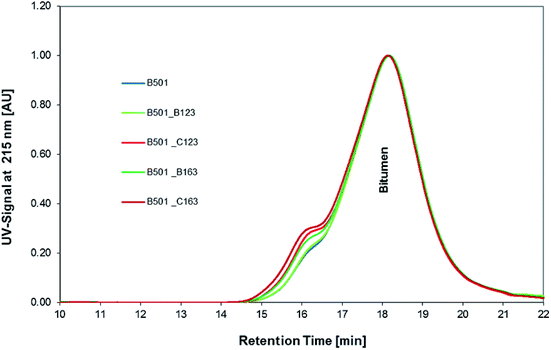 |
| Fig. 6 GPC chromatogram of bitumen B501. | |
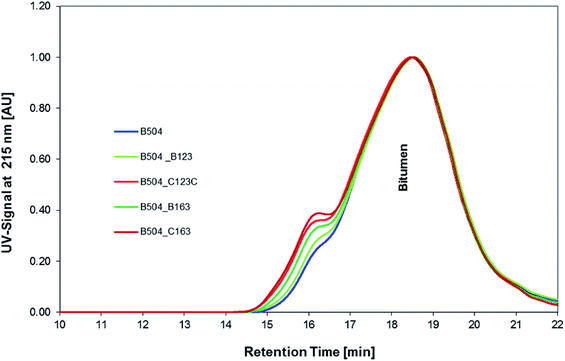 |
| Fig. 7 GPC chromatogram of bitumen B504. | |
4.2.2 FTIR. Fig. 8 and 9 show the FTIR spectra from one participating lab for the two binders B502 and B504 and close up of the sulfoxide and carbonyl bands respectively. From these diagrams the sulfoxide and carbonyl indexes are calculated based on the area method as described in Section 3. The carbonyl and sulfoxide indexes for both binders are shown in Fig. 10 and 11. From the chemical information it is apparent that the two binders react distinctly different to aging. As shown in these figures, unlike B504, after RTFOT ageing at 123 °C binder B502 showed no visible increase in the carbonyl index, only after PAV ageing, a distinct band for carbonyl structures could be detected. As for the sulfoxide index, all binder samples show absorbance in this band already in the unaged state. In some cases, the RTFOT at 123 °C did not change ISO significantly, whereas ISO increased for RTFOT at 163 °C. As for PAV, there was an increase after RTFOT at 123 °C, as well as at 163 °C. The increase seems to be stronger with increasing RTFOT temperature. Thus, the data show that RTFOT temperature and therefore mix production temperature, i.e. short term aging, has a stronger impact on the formation of sulfoxide structures than on carbonyl structures. In addition, the long-term aged state after PAV is affected by the short-term ageing temperature. This corroborated the findings of Peterson1 as stated earlier.
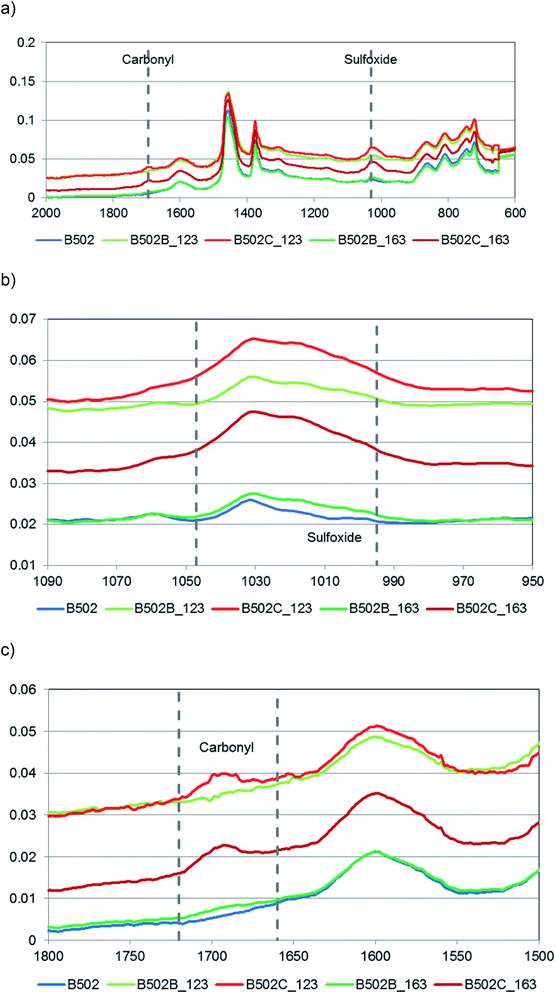 |
| Fig. 8 FTIR spectra of binder B502 in unaged and aged states (a); close-up of the sulfoxide band (b) and calbonyl band (c). | |
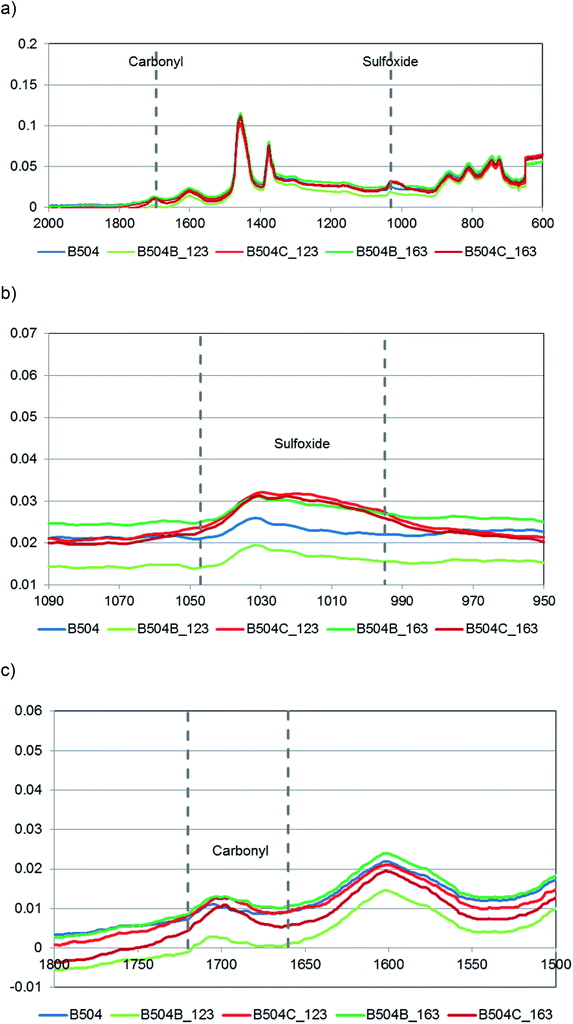 |
| Fig. 9 FTIR spectra of binder B504 in unaged and aged states (a); close-up of the sulfoxide band (b) and calbonyl band (c). | |
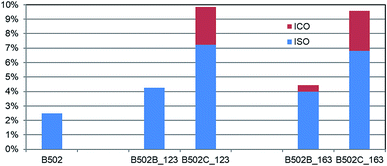 |
| Fig. 10 Chemical aging index (CAI) for binder B502 at different aging states. | |
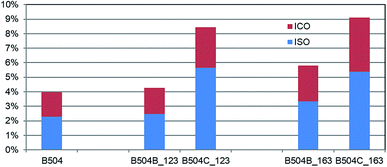 |
| Fig. 11 Chemical aging index (CAI) for binder B504 at different aging states. | |
It is worth noting that B504 displayed already a carbonyl peak at the non-aged stage, meaning that oxidation and aging is not the only contributing factors for the carbonyl peak and thereby the index. A possible cause of the carbonyl peak at virgin state is the common industry fabrication practice to blend different grades of bitumen to obtain a third grade. For example mixing a harder bitumen with softer bitumen. This will cause a high carbonyl index in virgin state. For this particular source the cause is the typical spectrum from naphthenic bitumen, it contains naphthenic acid that appears in ketone.
Combining ICO and ISO as in eqn (7) to deliver a chemical aging index shown in Fig. 10 and 11 allows comparing the two binders in terms of their combined chemical susceptibility to aging. Considering the CAI of B502; after short-term aging it went from 2.4% to 4.2% (123 °C) and 9.8% (163 °C) and that of B504 went from 3.9% to 4.2% (123 °C) and then to 8.4% (163 °C) it can be observed that B502 was more susceptible to short-term aging. On the other hand, CAI of B502 went from 9.8% to 9.6% (163 °C) after long-term aging whereas B504 went from 8.4% to 9.1%, indicating that B504 was more susceptible to long-term aging, corroborating the GPC results. At 123 °C B502 was less aged than B504 with CAI = 4.4% vs. 5.8%, whereas the opposite was observed when aging temperature of 163 °C was used: CAI = 9.6 vs. 9.1. These results show that B504 was less chemically aged that B502 at the higher temperatures. Furthermore, results indicate the importance of considering both types of aging as the short term and long term aging susceptibility can be temperature and binder dependent.
4.3 Chemo mechanical coupling
The Chemical Aging Index (CAI) is plotted vs. the Rheological Aging Index (RAI) in Fig. 12. As can be seen from these plots an exponential relationship between the two can be established. A reasonable correlation coefficient R2 = 0.79 for B504 (Fig. 12b) shows a much better trend between the chemical aging and rheological aging in comparison to a weak correlation for B502 (Fig. 12a) with an R2 = 0.12. The lower R2 of B502 of course affects the overall R2 = 0.24 when all data is plotted (Fig. 12c). These results confirm the fact that the two binders react differently to the same aging procedures as it was seen in the results presented in the previous sections.
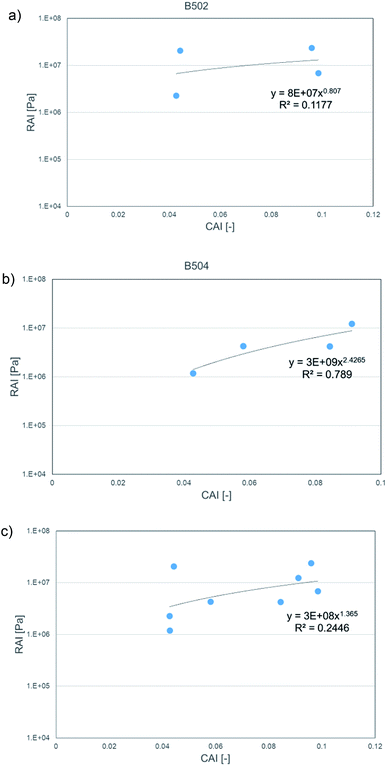 |
| Fig. 12 Chemical aging index (CAI) vs. Rheological Aging Index (RAI) for the two binders B502 (a) and B504 (b) and all data plotted (c). | |
5. Conclusions
Using a chemo-mechanical approach the effect of short-term aging temperature on long-term properties of binders was investigated. For the chemical analysis FTIR and GPC and for the physical/mechanical analysis complex shear modulus from DSR were used. A rheological aging index calculated from the area under the G* master curves and a chemical aging index combining the carbonyl and sulfoxide indexes was used. Two binders with the same penetration grade but from different sources were short term aged using RTFOT and thereafter long term aged using PAV. The results show that depending on the binder source aging sensitivity can differ significantly. The rheological aging index showed that different binders react differently to aging and some are more “aging resistant”. Reduced RTFOT aging temperature has a significant effect on RTFOT and PAV aging as determined by the rheological aging index RAI. There is a good trend between the crossover temperature (phase angle = 45°) and the complex modulus. The chemical investigations using FTIR has shown that mix production temperature has a stronger impact on the formation of sulfoxide structures than for carbonyl structures. The long-term aged state after PAV is affected by the short-term ageing temperature. These results show the importance of considering both types of aging as the short term and long term aging susceptibility do not always follow the same trend. Depending on the binder there can exist a reasonable correlation between the rheological aging and chemical aging. The results of this study confirm that lower short term aging temperatures can be beneficial in reducing the long term aging of bitumen. This aspect should be considered in simulating aging of reduced temperature mixtures in the laboratory and further standardization of such procedures.
Conflicts of interest
The authors declare that there is no conflict of interest in the work presented in this paper.
Acknowledgements
The authors would like to acknowledge the contributions of the active members of the Rilem 252-CMB technical committee in terms of experimental results and supplying bitumen samples. Mr Sivotha Hean is gratefully acknowledged for performing and analysing the GPC experiments at Empa. IMP is gratefully acknowledged for providing the FTIR and DSR data. Q8, Nynas, TU Vienna and Repsol are gratefully thanked for providing the bitumen for the tests.
References
- J. C. Petersen and R. Glaser, Asphalt Oxidation Mechanisms and the Role of Oxidation Products on Age Hardening Revisited, Road Mater. Pavement Des., 2011, 12, 795–819, DOI:10.1080/14680629.2011.9713895.
- D. Lesueur, The colloidal structure of bitumen: Consequences on the rheology and on the mechanisms of bitumen modification, Adv. Colloid Interface Sci., 2009, 145, 42–82 CrossRef CAS PubMed.
- X. Lu and U. Isacsson, Effect of ageing on bitumen chemistry and rheology, Constr. Build. Mater., 2002, 16, 15–22, DOI:10.1016/S0950-0618(01)00033-2.
- D. Mastrofini and M. Scarsella, The Application of Rheology to the Evaluation of Bitumen Ageing, Fuel, 2000, 79(9), 1005–1015, DOI:10.1016/S0016-2361(99)00244-6.
- P. Marsac, N. Piérard, L. Porot, W. Van den bergh, J. Grenfell and V. Mouillet, et al., Potential and limits of FTIR methods for reclaimed asphalt characterisation, Mater. Struct., 2014, 47, 1–14, DOI:10.1617/s11527-014-0248-0.
- F. L. Roberts, P. S. Kandhal, E. R. Brown, D. Y. Lee and T. W. Kennedy, Hot Mix Asphalt Materials, Mixture Design, and Construction, National Asphalt Pavement Association Education Foundation, Lanham, MD, 1996 Search PubMed.
- L. Loeber, G. Muller, J. Morel and O. Sutton, Bitumen in Colloid Science: A Chemical, Structural and Rheological Approach, Fuel, 1998, 77(13), 1443–1450, DOI:10.1016/S0016-2361(98)00054-4.
- A. Banerjee, A. Smit and J. Prozzi, The effect of long-term aging on the rheology of warm mix asphalt binders, Fuels, 2012, 97, 603–611 CrossRef CAS.
- N. Glotova, V. Gorshkov, B. Kats, T. Shapiro and G. Yureva, Changes in Rheological Properties and Chemical-Composition of Asphalts Upon Aging, Chem. Technol. Fuels Oils, 1980, 16(3–4), 278–280 CrossRef.
- L. Poulikakos, S. dos Santos, M. Bueno, S. Kuentzel, M. Hugener and M. Partl, Influence of short and long term aging on chemical, microstructural and macro-mechanical properties of recycled asphalt mixtures, Constr. Build. Mater., 2014, 51, 414–423 CrossRef.
- A. Ventura, A. Jullien and P. Monéron, Polycyclic aromatic hydrocarbons emitted from a hot-mix drum, asphalt plant: study of the influence from use of recycled bitumen, J. Environ. Eng. Sci., 2007, 6(6), 727–734 CrossRef CAS.
- L. D. Poulikakos, B. Hofko, L. Porot, X. Lu, H. Fischer and N. Kringos, Impact of temperature on short-and long-term aging of asphalt binders, RILEM Technical Letters, 2016, 1, 6–9 CrossRef.
- B. Hofko, A. Cannone Falchetto, J. Grenfell, L. Huber, X. Lu, L. Porot, L. D. Poulikakos and Z. You, Effect of short-term ageing temperature on bitumen properties, Road Mater. Pavement Des., 2017, 18(suppl. 2), 108–117, DOI:10.1080/14680629.2017.1304268.
- M. Mousavi, F. Pahlavan, D. Oldham, S. Hosseinnezhad and E. H. Fini, Multiscale Investigation of Oxidative Aging in Biomodified Asphalt Binder, J. Phys. Chem. C, 2016, 120(31), 17224–17233 CrossRef CAS.
- M. C. Cavalli, M. Zaumanis, E. Mazza, M. N. Partl and L. D. Poulikakos, Effect of Aging on the Mechanical and Chemical Properties of RAP Binder Treated with Bio-Based Rejuvenators, Composites, Part B, 2018, 141, 174–181 CrossRef CAS.
- L. Porot and P. Eduard, Addressing asphalt binder ageing through the viscous to elastic transition, Proceedings of the ISAP Symposium 2016, Jackson Hole, 2016 Search PubMed.
- L. Porot, Rheology and Bituminous binder, a review of different analyses, Rilem CMB symposium September, Braunschweig, 2018 Search PubMed.
- B. Hofko, M. Z. Alavi, H. Grothe, D. Jones and J. Harvey, Repeatability and sensitivity of FTIR ATR spectral analysis methods for bituminous binders, Mater. Struct., 2017, 50(3), 187 CrossRef.
- B. Hofko, L. Porot, A. Cannone Falchetto, L. Poulikakos, L. Huber, X. Lu, K. Mollenhauer and H. Grothe, FTIR spectral analysis of bituminous binders: reproducibility and impact of ageing temperature, Mater. Struct., 2018, 51(2), 45 CrossRef.
- P. Mikhailenko, et al., Comparison of ESEM and physical properties of virgin and laboratory aged asphalt binders, Fuel, 2019, 235, 627–638 CrossRef CAS.
- G. Ferrotti, et al., Comparison between bitumen aged in laboratory and recovered from HMA and WMA lab mixtures, Mater. Struct., 2018, 51(6), 150 CrossRef.
- EN 14770, Bitumen and bituminous binders - Determination of complex shear modulus and phase angle - Dynamic Shear Rheometer (DSR), 2012 Search PubMed.
- EN 12607-1, Bitumen and bituminous binders - Determination of the resistance to hardening under influence of heat and air - Part 1: RTFOT method, 2014 Search PubMed.
- EN 14769, Bitumen and bituminous binders - Accelerated long-term ageing conditioning by a Pressure Ageing Vessel (PAV) 2012:16, 2012 Search PubMed.
- EN 1426, Bitumen and bituminous binders - Determination of needle penetration, 2015 Search PubMed.
- EN 1427, Bitumen and bituminous binders - Determination of the softening point - Ring and Ball method, 2015 Search PubMed.
- Y. Wang, L. Sun and Y. Qin, Aging mechanism of SBS modified asphalt based on chemical reaction kinetics, Constr. Build. Mater., 2015, 91, 47–56 CrossRef.
- M. C. Rubio, G. Martinez, L. Baena and F. Moreno, Warm mix asphalt: an overview, J. Cleaner Prod., 2012, 24, 76–84 CrossRef CAS.
- A. R. Abbas, M. Nazzal, S. Kaya, S. Akinbowale, B. Subedi, M. S. Arefin and L. Abu Qtaish, Effect of Aging on Foamed Warm Mix Asphalt Produced by Water Injection, J. Mater. Civ. Eng., 2016, 28(11), 04016128 CrossRef.
- M. O. Marasteanu, and D. A. Anderson, Improved model for bitumen rheological characterization, in Eurobitume workshop on performance related properties for bituminous binders, European Bitumen Association, Brussels, Belgium, 1999 Search PubMed.
- M. Zeng, H. U. Bahia, H. Zhai, M. R. Anderson and P. Turner, Rheological modeling of modified asphalt binders and mixtures (with discussion), J. Assoc. Asphalt Paving Technol., 2001, 70, 403–441 Search PubMed.
- M. L. Williams, R. F. Landel and J. D. Ferry, The temperature dependence of relaxation mechanisms in amorphous polymers and other glass-forming liquids, J. Am. Chem. Soc., 1955, 77(14), 3701–3707 CrossRef CAS.
- H. Bahia and D. Anderson, The Pressure Aging Vessel (PAV): A Test to Simulate Rheological Changes Due to Field Aging, Physical Properties of Asphalt Cement Binders, STP1241-EB, ed. J. Hardin, ASTM International, West Conshohocken, PA, 1995, pp. 67–88, DOI:10.1520/STP18189S.
|
This journal is © The Royal Society of Chemistry 2019 |
Click here to see how this site uses Cookies. View our privacy policy here.