DOI:
10.1039/C8RA10288K
(Paper)
RSC Adv., 2019,
9, 10049-10057
Synthesis and properties of castor oil based plasticizers
Received
15th December 2018
, Accepted 18th March 2019
First published on 29th March 2019
Abstract
A series of environment-friendly plasticizers has been synthesized from castor oil through a mild esterification/epoxidation reaction. The modified epoxy acetylated castor oil (EACO) can plastify poly(vinyl chloride) (PVC) efficiently, even better than the commercial plasticizers dioctyl terephthalate (DOTP) and epoxidized soybean oil (ESO), in terms of in tensile strength, migration stability, solvent extraction stability and thermal stability. Specifically, the tensile strength and elongation at break of a PVC sample plastified by epoxy acetylated castor oil (EACO) were 18.5 and 10.0% higher than that of DOTP, and 13.9 and 23.8% higher than that of ESO, respectively. Volatility, migration, solvent extraction and thermal stability tests indicated that the presence of carbon–carbon double bonds and hydroxy groups reduce the compatibility of a plasticizer with PVC while the presence of epoxy groups and ester bonds can improve the plasticizing effect of the plasticizer on PVC. In addition, alkyl groups can improve the plasticizing effect on PVC while benzene rings increase the rigidity of the PVC. The design strategy based on castor oil highlights a sustainable avenue for preparing cost-effective and high-efficiency plasticizers.
Introduction
Poly(vinyl chloride) (PVC) is currently one of the largest produced synthetic resin products in the world. Adding an appropriate amount of plasticizer to PVC resin can improve the processibility, plasticity, flexibility, stretchability etc. Dioctyl phthalate (DOP), as the most applied typical plasticizer, has been widely used to plastify PVC for decades. Considering the direct and indirect threat of phthalate plasticizers for human health as well as the environment,1–3 dioctyl terephthalate (DOTP) is gradually replacing DOP as the main plasticizer in PVC. However, DOTP is still produced from petrochemical resources, hence finding cheap, environmentally friendly, and renewable alternative raw materials has become an urgent issue to relieve the crisis arising from fossil fuel depletion and environmental hazards. Vegetable oils have been increasingly used in the field of plasticizers because of their wide distribution, non-toxicity, low price, environmentally friendly properties and renewable nature.4
Plasticizers prepared from vegetable oils mainly include epoxy vegetable oils,5–8 and epoxidized fatty acid esters.9 In these types of plasticizers, the presence of the epoxy group makes the plasticizers have a better plasticizing effect on PVC and plays a role as stabilizer.10 When heated, PVC undergoes autocatalytic dehydrochlorination, whereas the epoxide group can scavenge the produced HCl which would have been constantly generated by a free radical reaction from PVC through induced catalytic degradation.11 Currently, an epoxy fatty acid multi-ester,12 dimer acid ester13 and fatty acid glycidyl ester14 are also used as plasticizers in PVC. However, most of these plasticizers, which are based on vegetable oils still have a problem in terms of their also being edible food ingredients.
Castor oil, a land-grown oil, is an inedible oil and a highly renewable resource for many chemical industries, and is mainly produced in Asia and Africa. Castor oil molecules contain hydroxy groups, carbon–carbon double bonds and ester bonds, which can be undergo epoxidation, sulfonation, halogenation, esterification, hydrolysis and other reactions to produce different varieties of plasticizers. For example, epoxidized methyl acetoricinoleate is a common castor oil based plasticizer with high epoxy value and good thermal stability,15 but a large amount of glycerol is produced during its preparation, which represents excess capacity. Two types of epoxy acetyl castor oil polyol ester plasticizers were synthesized by Jia et al. and had better plasticizing effect than DOP and ESO,16 but the tensile strength of the PVC blends was much lower than that from ESO, which makes its replacing of soybean oil impractical. A castor oil-based diglycidyl ester plasticizer (C26-DGE) was prepared by Chen et al.17 with ricinoleic acid as raw material and results showed that the plasticizer endowed the PVC matrix with enhanced compatibility and flexibility. Besides, a series of phosphorus-containing flame retardant plasticizers based on castor oil as a secondary plasticizer were synthesized and the results showed that the material added plasticizers had improved tensile strength, with good heat insulation and flame retardant properties, but the elongation at break was decreased.18–21
Our team has conducted a substantial amount of work on the synthesis of plasticizers, especially in clean production processes.22–24 In this study, we synthesized five environment-friendly plasticizers derived from castor oil through mild esterification/epoxidation reactions. The synthetic plasticizers were added to PVC (along with a heat stabilizer), and the properties were investigated and compared to the commercial plasticizers DOTP and ESO. We compared the effects of epoxy groups and carbon–carbon double bonds, ester groups and hydroxyl groups, and alkyl and benzene rings on plasticizing PVC to attempt to find an environment-friendly plasticizer with good plasticity ability using a simple synthesis method. We expect more competitive results compared with traditional plasticizers to lead the way towards green and sustainable routes for PVC application.
Experimental section
Materials
Hydrogen peroxide, acetic anhydride, benzoyl chloride, acetic acid, calcium oxide, toluene, sodium hydroxide, sodium carbonate, hydrochloric acid and acetone were provided by Nanjing Chemical Reagent Co., Ltd. (China); castor oil and the cation exchange resin were provided by Anhui Xinyuan Chemical Co., Ltd. (China).
Synthesis of epoxy castor oil (ECO)
Castor oil (93.4 g), acetic acid (18.0 g), toluene (100 mL) and cation exchange resin catalyst (4.7 g) were added to a 500 mL four-necked flask equipped with a stirrer, a thermometer, a constant pressure funnel and a ball condenser, 30% hydrogen peroxide (39.7 g) was dropwise added in the reaction mixture over 2 h and stirred at 60 °C for 5 h. Then the reaction mixture was filtered to recycle the cation exchange resin, and the remaining fluid was washed to pH = 7 with sodium carbonate solution and distilled water. Then the water and solvent were removed by vacuum distillation. Scheme 1 shows the synthesis routes of all products.
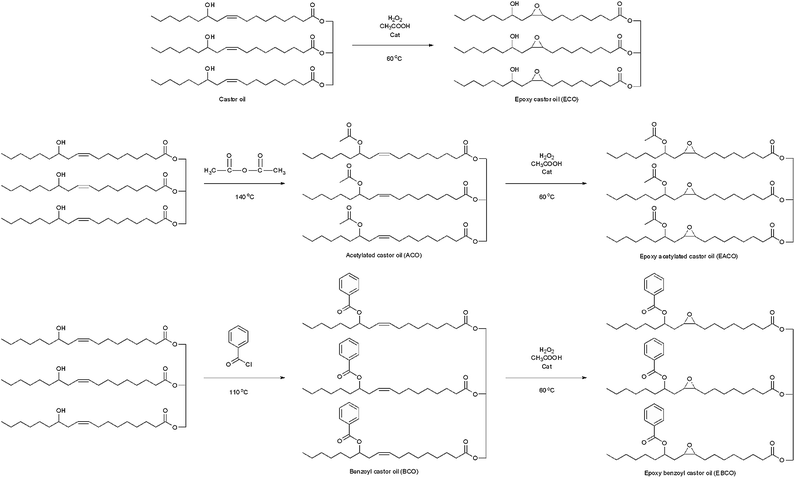 |
| Scheme 1 Synthesis of ECO, ACO, EACO, BCO and EBCO. | |
Synthesis of acetylated castor oil (ACO)
Castor oil (93.4 g) and acetic anhydride (27.5 g) were added to a 500 mL four-necked flask, and were stirred at 140 °C for 2 h. Then the reaction mixture was washed to pH = 7 with sodium carbonate solution and distilled water. Then the water was removed by vacuum distillation.
Synthesis of epoxy acetylated castor oil (EACO)
Castor oil (93.4 g) and acetic anhydride (27.5 g) were added to a 500 mL four-necked flask and were stirred at 140 °C for 2 h. Then the reaction mixture was cooled to 60 °C, and toluene (100 mL) and cation exchange resin catalyst (4.7 g) were added, after that 30% hydrogen peroxide (39.7 g) was added dropwise to the reaction mixture over 2 h and then stirred for 5 h. Then the reaction mixture was filtered to recycle the cation exchange resin, and the remaining fluid was washed to pH = 7 with sodium carbonate solution and distilled water. Then the water and solvent were removed by vacuum distillation.
Synthesis of benzoyl castor oil (BCO)
Castor oil (93.4 g), benzoyl chloride (38.0 g), calcium oxide (10.6 g) and toluene (100 mL) were added to a 500 mL four-necked flask and were stirred at 110 °C for 3 h. Then the reaction mixture was washed to pH = 7 with sodium hydroxide solution and distilled water. Then the water and solvent were removed by vacuum distillation.
Synthesis of epoxy benzoyl castor oil (EBCO)
BCO (121.5 g), acetic acid (18.0 g), toluene (100 mL) and cation exchange resin catalyst (4.7 g) were added to a 500 mL four-necked flask and were heated and 30% hydrogen peroxide (39.7 g) was added dropwise to the reaction mixture over 2 h and stirred at 60 °C for 5 h. Then the reaction mixture was filtered to recycle the cation exchange resin, and the remaining fluid was washed to pH = 7 with sodium carbonate solution and distilled water. Then the water and toluene were removed by vacuum distillation.
Preparation of plasticized PVC samples
The PVC, plasticizer and heat stabilizer were mixed in the ratio of 50
:
25
:
1. The mixture was blended for 7 min at 170 °C using a HAAKE PolyLab OS torque rheometer, and then placed in a flat-panel curing machine and pressed for 4 min at 170 °C and 2 min at room temperature. The PVC blends were labeled as PVC-DOTP (PVC/DOTP), PVC-ESO (PVC/ESO), PVC-ECO (PVC/ECO), PVC-ACO (PVC/ACO), PVC-EACO (PVC/EACO), PVC-BCO (PVC/BCO) and PVC-EBCO (PVC/EBCO).
Characterizations and measurements
FT-IR spectra were recorded on a Nicolet FTIR-360 (Nicolet Instrument Corp., USA) Fourier transform infrared spectrophotometer. The spectra was acquired in the range of 400 to 4000 cm−1.
1H NMR spectra were recorded by using an AVANE400 (Bruker Company, Switzerland) with deuterated chloroform as a solvent.
Tensile strength and elongation at break of all PVC samples were determined according to the GB/T 1040.1-2006 (China) under ambient conditions by using an E43.104 Universal Testing Machine (MTS Instrument Corp., China).
The volatility stability tests were determined by GB/T 3830-2008, PVC samples were cut into 50 mm × 50 mm squares, placed in an oven for 24 h at 80 °C, and then cooled to room temperature in a desiccator. The mass changes were measured by weighing before and after heating. The volatility rate was calculated as the ratio of the evaporated and the initial plasticizer.
Migration stability tests were determined according to the ISO 176-2005, PVC samples were cut into 50 mm × 50 mm squares, and covered with activated carbon on both sides. Samples were placed in an oven for 24 h at 20 and 70 °C, and weight loss was measured before and after heating.
The solvent extraction stability tests were determined according to the GB/T3830-2008, PVC samples with 50 mm × 50 mm shape were immersed in five different solvents (distilled water, 30% (w/w) acetic acid, 30% (w/w) sodium hydroxide, 50% (w/w) ethanol and cyclohexane) for 24 h at room temperature. Subsequently, samples were placed in an oven for 8 h at 50 °C and cooled to room temperature in a desiccator. The mass rate changes were measured before and after these processes.
The dynamic mechanical analyses were performed via a DMTA Q800 (TA Instruments, US) using rectangular samples of geometry 35 mm (L) × 12.5 mm (W) × 3.2 mm (T). The oscillatory frequency of the dynamic tests were 1 Hz. The temperature was raised at a rate of 3 °C min−1 from −60 to +120 °C.
The thermal ability of PVC blends was characterized in a DTG-60AH TGA thermal analysis instruments set-up (Netzsch Instrument Crop., Germany) in an N2 atmosphere (50 mL min−1) at a heating rate of 10 °C min−1. 5 mg of samples were placed into platinum pans and scanned from 30 to 600 °C.
The fracture surface morphology of the PVC blends after tensile fracture were analysed by a Hitachi S-4800 (Hitachi, Japan) scanning electron microscope (SEM) operated at 1 kV.
Results and discussion
FTIR and NMR characterization of the synthesized plasticizers
The FT-IR spectra of CO, ECO, ACO, EACO, BCO and EBCO are shown in Fig. 1. Compared to CO and ECO, the hydroxy group signal at 3400 cm−1 almost disappeared in the spectra of ACO, EACO, BCO and EBCO, which indicated that acylation reaction occurred. The epoxy group at 1068 cm−1 appeared in the FT-IR of ECO, EACO and EBCO, while the carbon–carbon double bonds of CO, ACO and BCO at around 3010 cm−1 disappeared, which demonstrated that the carbon–carbon double bonds have been converted into epoxy groups.
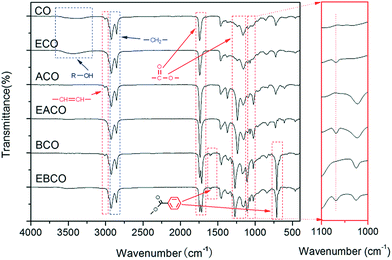 |
| Fig. 1 FT-IR spectra of CO and the synthesized plasticizers. | |
Fig. 2 shows the 1H NMR spectra of CO, ECO, ACO, EACO, BCO and EBCO. Compared to the CO 1H NMR spectra, the proton signals in the 5.45–5.55 ppm region of ECO, EACO and EBCO spectra associated with carbon–carbon double bond bands were weakened, and signals in the 2.95–3.15 ppm region of ECO, EACO and EBCO spectra associated with epoxy groups bands indicated that during the epoxidation reaction, most of the carbon–carbon double bonds have been converted. The signals in the 2.01 ppm region of ACO and EACO spectra are associated with shifted methyl hydrogens of the acetyl group, so verifying the acetylation reaction. Also, the proton signals in the 7.45–7.55 and 8.1 ppm region of BCO and EBCO spectra associated with benzene revealed that the hydroxy group of castor oil was acylated with benzoyl chloride.
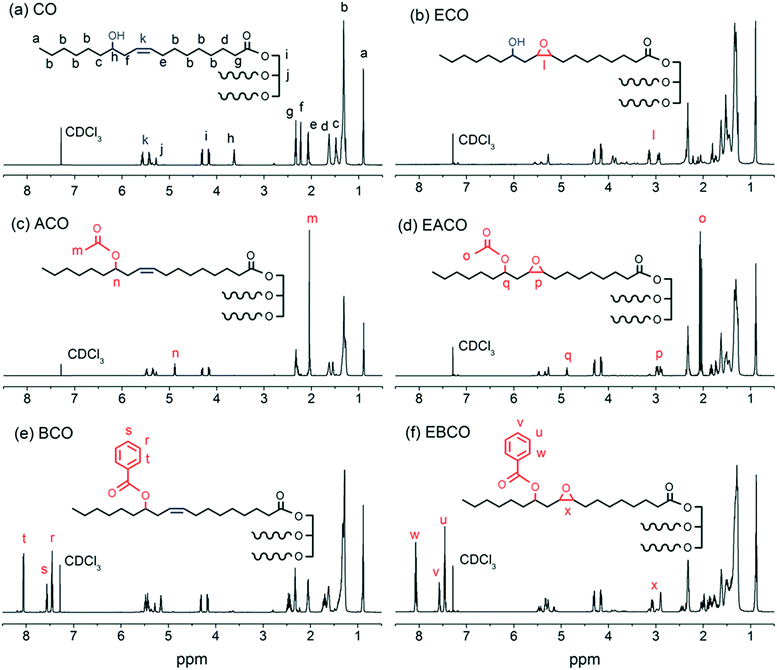 |
| Fig. 2 1H NMR spectrum of (a) CO, (b) ECO, (c) ACO, (d) EACO, (e) BCO and (f) EBCO. Note: ‘ ’ represents the same structure and functional group as the first molecular chain in their respective chemical structures. | |
Tensile test
The results of tensile strength and elongation at break are presented in Fig. 3. PVC-ECO shows a lower tensile strength and lower elongation compared with the other six samples. After acetylation or benzoylation of hydroxy groups and subsequent epoxidation, there is an obvious increase in tensile strength and elongation of PVC-EACO and PVC-EBCO, which indicated that the hydroxy group can reduce the compatibility of plasticizers with PVC.15 Compared to PVC-ACO and PVC-BCO, PVC-EACO and PVC-EBCO displayed superior tensile strength and break elongation, which can be attributed to that the epoxidation of carbon–carbon double bonds enhanced the plasticizer so as to be compatible with PVC.25,26 The tensile strength and elongation at break of PVC-EACO were 18.5 and 10.0% higher than PVC-DOTP, and 13.9 and 23.8% higher than PVC-ESO, respectively, demonstrating that the plasticizing effect of EACO on PVC is more efficient than that of DOTP and ESO. This may be because EACO has more epoxy groups than DOTP and more ester bonds than ESO, which can obviously increase the compatibility of plasticizers and PVC.14 PVC-EBCO showed the highest tensile strength compared with the other six samples and lower elongation than PVC-DOTP, PVC-ESO, PVC-ACO and PVC-EACO, which meant that the rigid benzene ring on the plasticizer structure can improve the tensile strength of the blend and reduce the fluidity of the plasticizer in PVC.27Plasticizer EBCO can thus be used in materials requiring high rigidity.
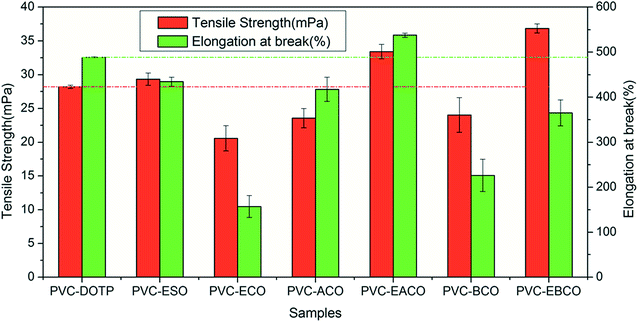 |
| Fig. 3 Tensile properties of the PVC blends. | |
Volatility stability test
The volatility stability of all plasticizers was investigated, and the results are shown in Fig. 4. PVC-ECO shows the highest volatility, which proved that the plasticizer ECO exhibited worst compatibility towards PVC owing to the presence of the hydroxyl group. The lower mass change of PVC-EACO and PVC-EBCO when compared to PVC-ACO and PVC-BCO indicated that the epoxide functional groups of EACO and EBCO play a more significant role than the double bonds of ACO and BCO. Additionally, the mass change of PVC films plastified by acetylation-modified plasticizers is less than that upon benzoylation modification, which illustrated that alkyl groups can increase the compatibility of plasticizers and PVC more than benzene rings.
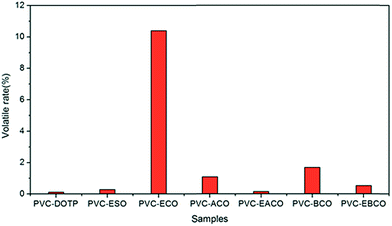 |
| Fig. 4 Volatility properties of the PVC blends. | |
Migration stability test
The migration stability of the different plasticizers in the PVC blends is shown in Fig. 5. At 20 °C, the weight loss of all samples is small, on account of the low molecular mobility at low temperature. At 70 °C, the trend is similar to that of volatility stability. The results further illustrate that the hydroxy group reduced the compatibility of plasticizers with PVC while the epoxy group could improve the volatility and migration stability of the plasticizer.
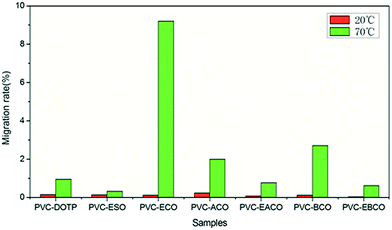 |
| Fig. 5 Migration stabilities of the PVC blends. | |
Migration stability test
The solvent extraction stability of the different plasticizers in PVC blends is shown in Fig. 6. The weight loss of PVC blends is strongly affected by the strength of the interactions between the plasticizers and the polymer chain.28 PVC-ECO, PVC-ACO and PVC-BCO show higher dissolution rate than PVC-DOTP, PVC-ESO and PVC-EACO in distilled water, 30% (w/w) acetic acid, 30% (w/w) sodium hydroxide and 50% (w/w) ethanol, which indicate that the hydroxy group and the double bonds have a negative effect on the interactions between the plasticizers and PVC, which made more plasticizer transfer to the solvent from PVC. However, in cyclohexane, PVC-ESO, PVC-ECO, PVC-EACO and PVC-EBCO have a lower extraction rate than the other three samples, which may be because the epoxy group is a polar group, and has a stronger interaction with the polar parts of the PVC molecules, which reduces the plasticizer dissolution rate.27 Moreover, PVC-EBCO presents a higher dissolution rate compared with PVC-EACO, even though EBCO has no hydroxy groups or carbon–carbon double bonds. However, the non-polar benzene rings of EBCO serve to weaken the attractive forces between the plasticizer and PVC chains, thus increasing the free volume and imparting flexibility to the polymer.29 On the whole, PVC-EACO had better solvent extraction stability than PVC-DOTP and PVC-ESO, which signifies that EACO has higher potential application value in food packing and medical devices.
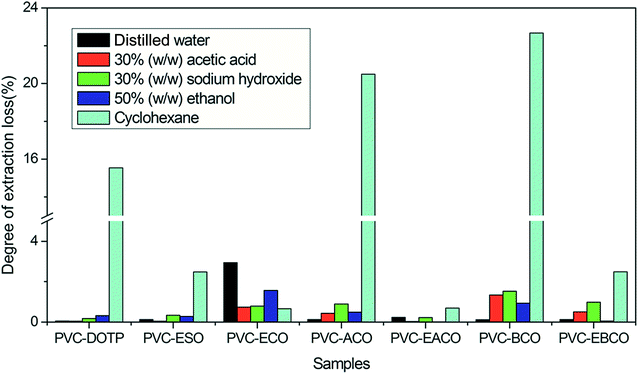 |
| Fig. 6 Solvent extraction stabilities of the PVC blends. | |
Dynamic mechanical property test
According to Fig. 7, the storage modulus of PVC-EACO is higher than that of PVC-DOTP and PVC-ESO, which indicated that PVC-EACO has a higher elasticity. At the same time, the acetylation and epoxidation modified PVC test pieces had higher storage modulus than for benzoylation and non-epoxidation samples, demonstrating that the alkyl and epoxy groups enhance the PVC elasticity compared to the benzene ring and carbon–carbon double bonds.
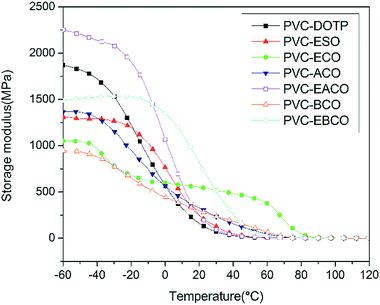 |
| Fig. 7 Storage modulus of the PVC blends. | |
The loss modulus (Fig. 8), which represents the amount of energy lost by viscous deformation of the material, is characterized by a peak value as well as the curve of the damping factor (tan
δ, Fig. 9), and generally the peak value of T is taken as the glass transition temperature. In most cases, a narrow tan
δ peak and single Tg of the polymer composite indicate excellent homogeneity.26 PVC-DOTP, PVC-ESO, PVC-ACO, PVC-EACO, PVC-BCO and PVC-EBCO showed only one tan
δ peak, and the Tg values were 35.2, 39.8, 70.2, 35.7, 76.9 and 60.1 °C respectively, indicating that the six plasticizers were compatible with PVC, while ECO was not, with two tan
δ peaks at −34.8 and 86.4 °C in PVC-ECO. PVC-EACO and PVC-EBCO show a lower Tg value than PVC-ACO and PVC-EBCO respectively, which demonstrates that the epoxy groups can make the molecule more polar, and hydrogen bonds formed between the molecular chains can ultimately increase the viscous activity of the mixture.30
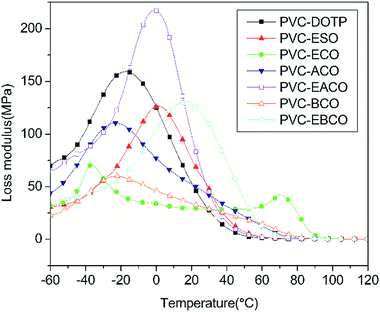 |
| Fig. 8 Loss modulus of the PVC blends. | |
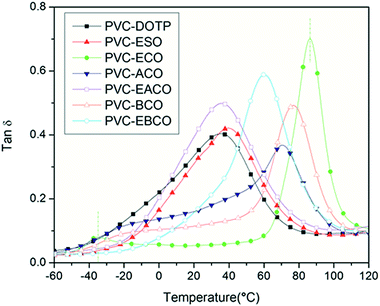 |
| Fig. 9 Loss tangent curves as a function of temperature of the PVC blends. | |
Thermogravimetric analysis
The TGA of PVC plasticized with different plasticizers is presented in Fig. 10. From the TGA curves of PVC blends, it can be seen that all of the PVC blends were thermally stable in a nitrogen atmosphere below 220 °C and exhibited a two-stage thermal degradation process above that temperature. The first degradation at about 260–350 °C corresponds to the elimination of a large amount of HCl. The second stage at about 440–480 °C is attributed to the decomposition of the PVC main chain and plasticizers. The thermal performance data including the weight loss of 10% (T10%), the weight loss of 50% (T50%) and the maximum weight-loss temperature rate (TP1 and TP2) are summarized in Table 1. T10%, T50% and TP1 values of PVC plasticized with ESO, ECO, ACO, EACO, BCO and EBCO were higher than for DOTP, which may be because compared to DOTP, the other six plasticizers have more ester bond structures and epoxy groups, which are associated with higher thermal stability than the lower-thermally stable chain segments of DOTP, thereby increasing the thermal stability of the PVC blends.16 The TP1 values of PVC plasticized with ESO, ECO, EACO and EBCO were higher than those with DOTP, ACO and BCO, which proves that epoxy groups can absorb hydrogen chloride degraded by light and heat, preventing the continuous decomposition of PVC and prolonging the lifetime of PVC.31,32 In addition, the TP2 values of PVC-ACO and PVC-BCO are lower than those of PVC-EACO and PVC-EBCO, which may be because the double bonds in ACO and BCO reduced the thermal stability. The char residue of PVC plasticized with DOTP is higher than that of the other plasticizers, indicating that ESO, ECO, ACO, EACO, BCO and EBCO could decrease the char residue of PVC blends more than DOTP. From these data, we could conclude that the castor oil based plasticizers could improve the thermal stability and reduce the amount of char residue of PVC blends.
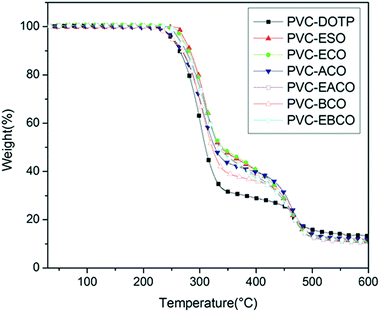 |
| Fig. 10 TGA curves of the PVC blends. | |
Table 1 TGA data of the PVC blends
Samples |
T10%/°C |
T50%/°C |
TP1/°C |
TP2/°C |
Residue (%) |
PVC-DOTP |
264.4 |
310.1 |
300.9 |
465.0 |
13.16 |
PVC-ESO |
283.1 |
337.4 |
310.6 |
460.8 |
10.89 |
PVC-ECO |
280.3 |
340.1 |
307.9 |
465.3 |
11.08 |
PVC-ACO |
268.3 |
326.9 |
302.4 |
459.0 |
11.83 |
PVC-EACO |
275.7 |
334.8 |
309.6 |
465.5 |
10.22 |
PVC-BCO |
268.8 |
322.2 |
301.7 |
457.8 |
11.47 |
PVC-EBCO |
274.5 |
335.2 |
307.2 |
466.6 |
10.74 |
Fracture surface morphology analysis
The fracture surface morphology of pulled and cut plasticized PVC blends is shown in Fig. 11. As can be seen, the section topography of the different PVC blends is quite different. PVC-DOTP, PVC-ESO, PVC-ACO, PVC-EACO, PVC-BCO and PVC-EBCO clearly showed numerous small protrusions and non-retracted filaments, which are typical features of ductile fracture, indicating that the plasticizers have great compatibility with PVC and a good plasticizing effect. However, PVC-ECO did not have obvious protrusions and filaments, and the edge of the fracture was very smooth, which indicated that ECO did not show a good plasticizing effect with PVC. The section topography of PVC-EACO was much more complex than the others, and protrusions and filaments were more numerous and longer, which indicates that the plasticizer EACO had the best plasticizing effect, even better than that of DOTP and ESO.
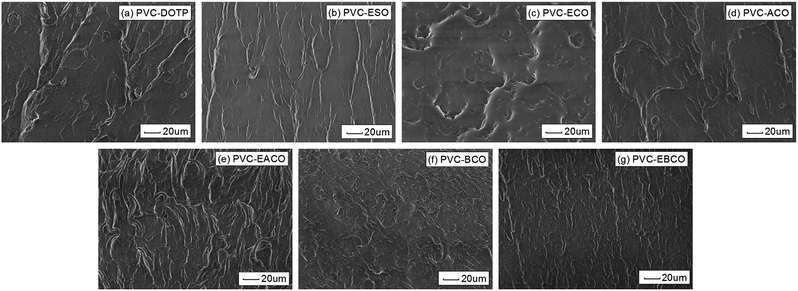 |
| Fig. 11 SEM images of (a) PVC-DOTP, (b) PVC-ESO, (c) PVC-ECO, (d) PVC-ACO, (e) PVC-EACO, (f) PVC-BCO and (g) PVC-EBCO. | |
Conclusions
In this study, five types of environment-friendly material plasticizers based on castor oil were synthesized. The synthetic plasticizers were added to PVC as the main plasticizer, and the properties of these PVC blends were investigated and compared to the commercial plasticizers DOTP and ESO. Tensile properties showed that the tensile strength and elongation at break of the PVC sample plastified by EACO were 18.5 and 10.0% higher than that of DOTP, and 13.9 and 23.8% higher than that of ESO, respectively. EACO also had generally better volatility, solvent extraction and migration stability properties than those of DOTP and ESO, and better than the other four plasticizers synthesized. All the tests illustrated that compared to hydroxy groups, carbon–carbon double bonds and phenyl groups, ester bonds, epoxy groups and alkyl groups can make plasticizers more compatible with PVC, showing better plasticizing effect and better thermal stability. Based on the above studies, the plasticizer EACO based on castor oil shows an excellent performance, in addition to this, during its synthesis, acetic acid produced by acetylation could be used in the subsequent epoxidation reaction to avoid excessive use of acid, and catalyst in the epoxidation reaction can be recycled. As an environmental-friendly plasticizer, it may have potential applications in medical equipment, children’s toys, food packaging materials and other fields.
Conflicts of interest
There are no conflicts to declare.
Acknowledgements
The authors would like to thank the State Forestry Administration 948 project foundation for financial support under the Grant No 2015-4-55, China; and the Priority Academic Program Development of Jiangsu Higher Education Institutions (PAPD), China. The article is also supported by the Doctorate Fellowship Foundation of Nanjing Forestry University.
Notes and references
- L. Giuseppe, D. F. Claudio and V. Alberto, Reprod. Toxicol., 2004, 19, 27–33 CrossRef PubMed.
- G. Latini, Neonatology, 2000, 78, 269–276 CrossRef CAS PubMed.
- L. M. Kamendulis, J. S. Isenberg, J. H. Smith, G. Pugh Jr, A. W. Lington and J. E. Klaunig, J. Toxicol. Environ. Health, Part A, 2002, 65, 569–588 CrossRef CAS PubMed.
- L. M. De Espinosa and M. A. R. Meier, Eur. Polym. J., 2011, 47, 837–852 CrossRef.
- P. G. Demertzis, K. A. Riganakos and K. Akrida, Eur. Polym. J., 1991, 27, 231–235 CrossRef.
- P. G. Nihul, S. T. Mhaske and V. V. Shertukde, Iran. Polym. J., 2014, 23, 599–608 CrossRef CAS.
- M. A. Semsarzadeh, M. Mehrabzadeh and S. S. Arabshahi, Eur. Polym. J., 2002, 38, 351–358 CrossRef CAS.
- B. Badra and T. B. Mohamed, J. Appl. Polym. Sci., 2008, 107, 3442–3450 CrossRef.
- O. Fenollar, N. L. Sanchez, S. D. Garcia, J. López and R. J. Balart, J. Mater. Sci., 2009, 44, 3702–3711 CrossRef CAS.
- B. M. Abdullah and J. Salimon, J. Appl. Sci., 2010, 1, 1543–1553 Search PubMed.
- P. Karmalm, T. Hjertberg, A. Jansson and R. Dahl, Polym. Degrad. Stab., 2009, 94, 2275–2281 CrossRef CAS.
- M. Li, S. Li, J. Xia, C. Ding, M. Wang, L. Xu, X. Yang and K. Huang, Materials & Design, 2017, 122, 366–375 CrossRef CAS.
- J. Chen, X. Li, Y. Wang, J. Huang, K. Li, X. Nie and J. Jiang, J. Appl. Polym. Sci., 2016, 133, 43668–43675 Search PubMed.
- J. Chen, X. Li, Y. Wang, J. Huang, K. Li, X. Nie and J. Jiang, Eur. J. Lipid Sci. Technol., 2017, 119, 1600216 CrossRef.
- W. Xu, X. Ge, L. Jin and R. Shao, Chem. Ind. Eng. Prog., 2015, 34, 1983–1988 CAS.
- P. Jia, M. Zhang, L. Hu, G. Feng, C. Bo and Y. Zhou, ACS Sustainable Chem. Eng., 2015, 3, 2187–2193 CrossRef CAS.
- J. Chen, Z. Liu, X. Nie and J. Jiang, J. Mater. Sci., 2018, 53, 8909–8920 CrossRef CAS.
- P. Jia, M. Zhang, L. Hu, C. Bo and Y. Zhou, J. Therm. Anal. Calorim., 2015, 120, 1731–1740 CrossRef CAS.
- P. Jia, M. Zhang, C. Liu, L. Hu, G. Feng, C. Bo and Y. Zhou, RSC Adv., 2015, 5, 41169–41178 RSC.
- P. Jia, M. Zhang, L. Hu, C. Liu, G. Feng, X. Yang, C. Bo and Y. Zhou, RSC Adv., 2015, 5, 76392–76400 RSC.
- F. Wang, S. Pan, P. Zhang, H. Fan, Y. Chen and J. Yan, Fibers Polym., 2018, 19, 1057–1063 CrossRef CAS.
- J. Tan, T. Lu, J. Zhang, B. Xie, F. Zhu, M. Chen and X. Zhu, J. Taiwan Inst. Chem. Eng., 2018, 86, 18–24 CrossRef CAS.
- H. Zhang, F. Zhu, Y. Xu, X. Zhang and X. Zhu, Synth. Commun., 2017, 47, 486–495 CrossRef CAS.
- H. Zhang, D. Liu, T. Kang, Y. Wang, X. Zhang and X. Zhu, Chin. J. Org. Chem., 2016, 36, 1104–1110 CrossRef CAS.
- M. T. Rodríguez, S. J. García, R. Cabello and J. J. Suay, J. Coat. Technol. Res., 2005, 2, 557–564 CrossRef.
- S. Lee, M. S. Park, J. Shin and Y. W. Kim, Polym. Degrad. Stab., 2018, 147, 1–11 CrossRef CAS.
- J. Chen, Z. Liu, J. Jiang, X. Nie, Y. Zhou and R. E. Murray, RSC Adv., 2015, 5, 56171–56180 RSC.
- N. Lardjane and N. Belhaneche-Bensemra, J. Appl. Polym. Sci., 2009, 111, 525–531 CrossRef CAS.
- J. Kastner, D. G. Cooper, M. Marić, P. Dodd and V. Yargeau, Sci. Total Environ., 2012, 432, 357–364 CrossRef CAS PubMed.
- X. Wu, X. Zhang, S. Yang, H. Chen and D. Wang, J. Am. Oil Chem. Soc., 2000, 77, 561–563 CrossRef CAS.
- P. Karmalm, T. Hjertberg, A. Jansson and R. Dahl, Polym. Degrad. Stab., 2009, 94, 2275–2281 CrossRef CAS.
- H. Hosney, B. Nadiem, I. Ashour, I. Mustafa and A. EI-Shibiny, J. Appl. Polym. Sci., 2018, 135, 46270 CrossRef.
|
This journal is © The Royal Society of Chemistry 2019 |
Click here to see how this site uses Cookies. View our privacy policy here.