DOI:
10.1039/C8RA10059D
(Paper)
RSC Adv., 2019,
9, 4295-4302
Luminescence properties and energy transfer of K3LuF6:Tb3+,Eu3+ multicolor phosphors with a cryolite structure†
Received
7th December 2018
, Accepted 15th January 2019
First published on 1st February 2019
Abstract
In recent years, compounds with a cryolite structure have become excellent hosts for luminescent materials. In this paper, Tb3+ doped and Tb3+/Eu3+ co-doped K3LuF6 phosphors were prepared via a high temperature solid phase sintering method. The XRD, SEM, as well as photoluminescence excitation (PLE) and emission (PL) spectra were measured to investigate the structure and luminescence properties of the as-prepared samples. In the Tb3+/Eu3+ co-doped K3LuF6 samples, both characteristic emission spectra of Tb3+ and Eu3+ could be observed and the emission color of the K3LuF6:0.12Tb3+,xEu3+ phosphors could be adjusted from green to yellowish pink and the corresponding CIE values could be regulated from (0.2781, 0.5407) in the green area to (0.4331, 0.3556) in the yellowish pink area by controlling the concentration ratio of Eu3+/Tb3+. In addition, the energy transfer mechanism in Tb3+/Eu3+ co-doped K3LuF6 was calculated to be a quadrupole–quadrupole interaction from Tb3+ to Eu3+ based on the Dexter's equation.
1. Introduction
Fluoride compounds are potential hosts for luminescent materials due to their suitable chemical stability and excellent luminous properties, and as such, have received a lot of attentions over the past few decades.1–4 Cryolite is an important kind of fluoride compound, the chemical formula of which can be expressed as M3NF6, where M represents monovalent cations such as alkali metal ions (Li+, Na+, K+, Rb+) and NH4+, and the sites of N can be occupied by trivalent cations, that is Al3+, Y3+, Sc3+, Ga3+, etc.5,6 In addition, the N sites can also be occupied by rare earth ions by isomorphic replacement in the cryolite lattice, such as K3GaF6,7 K3InF6,8 and K3LuF6.9 Since the luminescence behavior of rare earth ions depends on the matrix, it is significant to explore novel cryolite luminescent materials.
On the other hand, multicolor single-phase phosphors occupy an important seat in the field of luminescent materials. In particular, rare-earth ion-doped luminescent materials mixed with blue and UV LEDs are fabricated to produce white light. However, a traditional single-color single-phase strategy usually requires a complicated synthetic process, multiple excitation wavelengths with different responses of the various dopants to the excitation wavelength and reabsorption of the dopant.10 Single excitation wavelength excited phosphors with multicolor emissions (cyan-emitting, blue-green-emitting, and blue-red-emitting phosphors) can efficiently avoid the above problems and make it easier to obtain a phosphor with high luminous efficiency, excellent color rendering index (CRI), good thermal and chromatic stability. Accordingly, multicolor single-phase phosphors have received attention over the past few decades.11–13
Energy transfer is an important way to realize single-phase multicolor phosphors, which have been investigated in abundant hosts.14 As we know, energy transfer is achieved by ion pairs from a sensitizer to activator, such as Eu2+/Tb3+, Ce3+/Eu2+, Ce3+/Dy3+, Tb3+/Eu3+, Er3+/Sm3+, Tm3+/Dy3+ and so on.15–20 Due to 5D0 → 7F2 electronic transitions, Eu3+-doped luminescent materials can emit strong red light.21 Tb3+ has been widely used together with Eu3+ to produce multicolor emission by means of energy transfer, such as in CaMoO4:Tb3+/Eu3+,22 Ba2La3(SiO4)3F:Tb3+/Eu3+, and23 Na2MgSiO4:Tb3+/Eu3+.24 However, until now, there have been no reports on the crystal structure, luminescence properties and energy transfer investigations of multicolor-emitting K3LuF6:Tb3+,Eu3+ phosphors with a cryolite structure.
In the present work, Tb3+ and Tb3+/Eu3+ activated cryolite-type K3LuF6 compounds were synthesized in a simple way and the crystal structure and luminescence properties, as well as the energy transfer mechanism between Tb3+ and Eu3+ in K3LuF6 were thoroughly studied. Benefiting from the crystal field environment of the cryolite-type samples, the emission color of K3LuF6:Tb3+,Eu3+ could be continuously regulated from green to yellowish pink by changing the content ratio of Tb3+/Eu3+.
2. Experimental section
2.1. Materials and synthesis
K3LuF6:xTb3+ (x = 0.01, 0.03, 005, 0.07, 0.09, 0.10, 0.12 and 0.13) and K3LuF6:0.12Tb3+,xEu3+ (x = 0.03, 005, 0.07, 0.10 and 0.13) phosphors were prepared via a high temperature solid-state method. The raw materials included K2CO3 (AR), Lu2O3 (99.99%), NH4HF2 (AR), Eu2O3 (99.99%) and Tb4O7 (99.99%). Firstly, the raw materials were weighed according to the stoichiometric ratios of the reactions and were then ground in a mortar for nearly 10 min, resulting in a uniform mixture. Considering the loss of fluorine sources at high temperatures, a 30% excess of NH4HF2 was required during the weighing process. Secondly, the mixture was moved to a furnace and heated at heating rate of 4° min−1 and was maintained at 800 °C for 3 h under an argon atmosphere. Finally, the systems were naturally cooled down to room-temperature and evenly re-ground prior to characterization.
2.2. Characterization
The powder X-ray diffraction (PXRD) measurements of the phosphors were performed using a Bruker Corporation D8 powder X-ray diffractometer (Germany) equipped with a Cu kα radiation source at 0.15406 nm, a tube voltage of 40 kV and a tube current of 40 mA. The step scanning rate used was 8° min−1 over the 2θ range from 10° to 70°. Morphological analysis of the compounds was carried out using a scanning electron microscope (SEM, JSM-6701F, Hitachi, Japan). The photoluminescence (PL) and photoluminescence excitation behavior were measured using a Hitachi F-4600 fluorescence spectrophotometer at room temperature, with a xenon lamp (400 V, 150 W) acting as the light source.
3. Results and discussion
3.1. Crystal structure
The PXRD patterns of the as-prepared K3LuF6:xTb3+ (x = 0.01, 0.03, 005, 0.07, 0.09, 0.1, 0.12 and 0.13) and K3LuF6:0.12Tb3+,xEu3+ (x = 0.03, 005, 0.07, 0.1 and 0.13) phosphors are shown in Fig. 1(a) and (b). The standard PXRD pattern of K3LuF6 (JCPDS no. 27-467) is shown as a reference. As shown in Fig. 1(a) and (b), all of the PXRD patterns of the as-prepared K3LuF6:Tb3+ and K3LuF6:0.12Tb3+,Eu3+ samples can be accurately assigned to the standard card of K3LuF6, without the appearance of any impurity peaks, which indicates that the introduction of Tb3+ and Eu3+ in K3LuF6 does not bring about any significant changes in the crystal structure. Fig. 1(c) shows the crystal structure of K3LuF6, which belongs to a monoclinic phase with a space group of P21/n. As depicted in Fig. 1(c), the Lu atom coordinates to six F atoms forming [LuF6] octahedra, with the Lu atom in the centre. Two nonequivalent sites for the K atoms can be found in the crystal structure of K3LuF6, that is, twelve-coordinated K and six-coordinated K. Based on the charge balance and effective ionic radii, Tb3+ and Eu3+ were thought to occupy the sites of Lu3+. Fig. 1(d) shows the SEM image of K3LuF6:0.12Tb3+,0.07Eu3+, which indicates the prepared sample with an irregular shape and the particle size ranges from a few hundred nanometers to dozens of microns (Fig. 2).
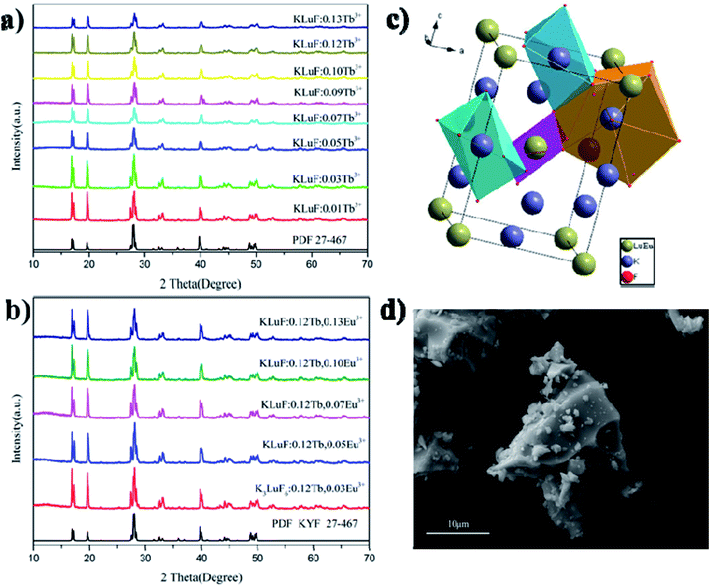 |
| Fig. 1 (a) The PXRD patterns of the as-prepared K3LuF6:xTb3+ (x = 0.01, 0.03, 005, 0.07, 0.09, 0.10, 0.12 and 0.13) and (b) K3LuF6:0.12Tb3+,xEu3+ (x = 0.03, 005, 0.07, 0.10 and 0.13) phosphors, (c) the crystal structure of K3LuF6, and (d) the SEM image of the K3LuF6:0.12Tb3+,0.07Eu3+ sample. | |
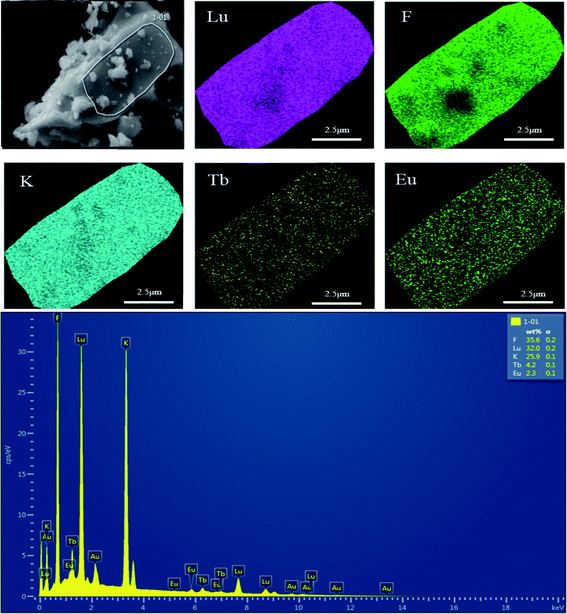 |
| Fig. 2 SEM image, elemental mapping images and EDX spectrum of the K3LuF6:0.12Tb3+,0.07Eu3+ phosphor. | |
The elemental composition and distribution of the prepared K3LuF6:0.12Tb3+,0.07Eu3+ were investigated and the results are shown in Fig. 3. In addition, the elemental composition and distribution of the prepared K3LuF6:0.12Tb3+,xEu3+ (x = 0, 0.03, 0.05, 0.07, 0.10, and 0.13) are shown in Fig. S1–S6,† among which the Au element was introduced to enhance the conductivity of the material during testing. The particles in all the samples seem like blocks with aggregation. The energy-dispersive X-ray (EDX) spectrum illustrates the existence of elemental K, Lu, F, Tb, and Eu in the sample, except for in the Tb3+ single-doped sample. Moreover, the elemental mapping results confirmed that all of the elements in the sample were homogenously distributed over the whole area.
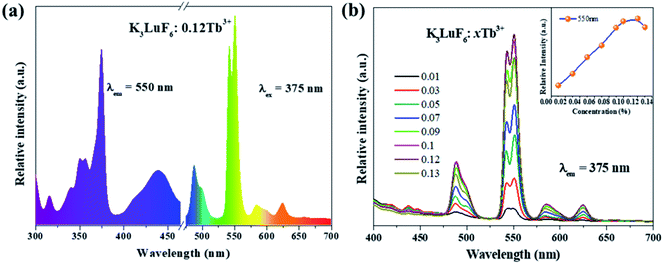 |
| Fig. 3 (a) The photoluminescence excitation (PLE) (λem = 550 nm) and emission (PL) (λex = 375 nm) spectra of the K3LuF6:0.12Tb3+ phosphor, and (b) the emission PL spectra (λex = 375 nm) of the K3LuF6:xTb3+ (x = 0.01, 0.03, 005, 0.07, 0.09, 0.10, 0.12 and 0.13) phosphors. The inset depicts the relative emission-intensity trends for the 550 nm peak (5D4–7F3) in terms of the Tb3+ concentration. | |
3.2. Photoluminescence properties and energy transfer process
Fig. 3(a) shows the PLE (λem = 550 nm) and PL (λex = 375 nm) spectra of the K3LuF6:0.12Tb3+ phosphor. As depicted in Fig. 3(a), the PLE spectrum of the K3LuF6:0.12Tb3+ sample shows typical transitions of Tb3+ ranging from 310 to 460 nm, attributed to 7F6–5H7 (315 nm), 7F6–5D2 (340 and 351 nm), 7F6–5D4 (357 nm), 7F6–5D3 (375 nm), and 7F6–5G6 (440 nm), respectively. The excitation peak at 375 nm (7F6–5D3) is the most intense, matching well with near ultraviolet light LED chips, indicating that this sample has the potential for application in LEDs. When the phosphor was monitored at 375 nm, the PL spectrum of the K3LuF6:0.12Tb3+ sample exhibited several peaks located at 488/499, 540/550, 586/599, and 624 nm, respectively. These peaks can be assigned as the characteristic emission of 5D4–7Fn (n = 6, 5, 5 and 3). Fig. 3(b) shows the emission PL spectra of the K3LuF6:xTb3+ (x = 0.01, 0.03, 005, 0.07, 0.09, 0.1, 0.12 and 0.13) phosphors monitored at 375 nm, and the inset depicts the relative emission-intensity trends for the 550 nm peak (5D4–7F3) regarding the Tb3+ concentration. Upon 375 nm excitation, all of the PL spectra of the K3LuF6:xTb3+ samples showed characteristic Tb3+ emission peaks, attributed to J = 6, 5, 4, and 3. It is well known that the emission intensity of the 5D3–7FJ (J = 6, 5, 4, and 3) transitions of Tb3+ are quenched upon an increase in the Tb3+ concentration, ascribed to the cross-relaxation effect regarding the 5D3–7FJ levels.25–27 Meanwhile, the emission intensity for the 550 nm (5D4–7F3) peak increased upon an increase in the concentration of the Tb3+ concentration up to K3LuF6:0.12Tb3+,and then it decreased due to concentration quenching.28 Thus, the Tb3+ doping concentration was fixed at 0.12 (mol) for the co-doping of Tb3+ and Eu3+ in K3LuF6.
In order to further investigate the possibility of energy transfer behavior between Tb3+ and Eu3+ in K3LuF6, K3LuF6:0.12Tb3+,xEu3+ (x = 0.03, 005, 0.07, 0.1 and 0.13) phosphors were prepared. The PLE and PL spectra of the Tb3+ and Eu3+ single-doped, as well as Tb3+/Eu3+ co-doped K3LuF6 phosphors are shown in Fig. 4. Under 393 nm excitation, the as-prepared K3LuF6:0.1Eu3+ sample shows sharp peaks at 579, 590, 613, 624, and 656 nm in Fig. 4(a), which are due to the 5D0–7F0, 5D0–7F1, 5D0–7F2, 5D0–7F2, and 5D0–7F3 transitions of Eu3+, respectively. In addition, the emission intensity of the peak at 613 nm is the highest among the transitions of Eu3+, as shown in Fig. 4(b). When monitored at 613 nm, the PLE spectrum of K3LuF6:0.1Eu3+ exhibits a series of sharp excitation bands between 300 and 500 nm, centered at 318, 361, 379, 393, and 414 nm, which can be attributed to the 7F0–5H5, 7F0–5D4, 7F0–5L6, 7F0–5L7 and 7F0–5D3 transitions, respectively.29,30 The strongest excitation peak can be obviously observed at 393 nm. The PLE and PL spectra of the K3LuF6:0.12Tb3+,0.1Eu3+ phosphor are illustrated in Fig. 4(b). As shown in Fig. 4(c), when the co-doped sample was excited by near ultraviolet light at 375 nm, both characteristic emission peaks of Tb3+ and Eu3+ could be observed in the PL spectrum, indicating that energy transfer may occur between Tb3+ and Eu3+ in K3LuF6. Moreover, when monitored by 613 nm light, which is the typical emission wavelength of Eu3+, the K3LuF6:0.12Tb3+,0.1Eu3+ phosphor showed a characteristic excitation peak (375 nm) of Tb3+, while the peak at 375 nm could not be found in the excitation spectrum. Therefore, energy transfer from Tb3+ to Eu3+ exists in the K3LuF6 host and the emission color for K3LuF6:Tb3+/Eu3+ can be regulated according to different energy transfer efficiencies by adjusting the ratio of Eu3+/Tb3+.31 However, when the monitoring light was changed to 550 nm, the excitation spectrum of the co-doped sample did not exhibit the typical Eu3+excitation peak. Therefore, it could be inferred that the energy transfer process is irreversible in this system.
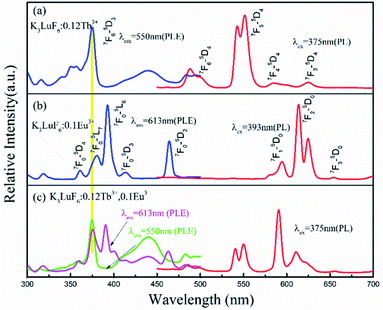 |
| Fig. 4 The PLE and PL spectra of (a) K3LuF6:0.12Tb3+, (b) K3LuF6:0.1Eu3+, and (c) K3LuF6:0.12Tb3+,0.1Eu3+. | |
To further investigate the energy transfer process, the K3LuF6:0.12Tb3+,xEu3+ (x = 0, 0.03, 005, 0.07, 0.1 and 0.13) samples were synthesized using the same method. The PL spectra of K3LuF6:0.12Tb3+,xEu3+ (x = 0, 0.03, 0.05, 0.07, 0.1 and 0.13) under the excitation of 375 nm were recorded and the results are shown in Fig. 5. The inset shows the relative emission intensity at 550, 590, and 613 nm, respectively. Upon 375 nm excitation and with increasing Eu3+ concentration, the emission intensity of Tb at 550 nm (5D4–7F3 transitions) decreases all the time and the emission intensity of Eu3+ at 590 nm (5D0–7F1 transitions) increases and reaches a maximum at x = 0.10, after which the emission intensity decreases, caused by the concentration quenching of Eu3+ itself.32 The results indicate that energy transfer occurs from Tb3+ to Eu3+ in the K3LuF6 host. The emission intensity of Eu3+ at 613 nm (5D0–7F2 transitions) increases and reaches a maximum at x = 0.03, then the emission intensity decreases upon an increase in the Eu3+ concentration, which can be ascribed to the concentration quenching of Eu3+.
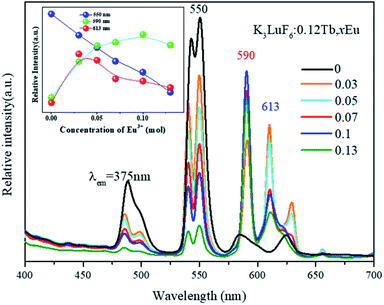 |
| Fig. 5 The PL spectra of K3LuF6:0.12Tb3+,xEu3+ (x = 0, 0.03, 005, 0.07, 0.1 and 0.13) under excitation at 375 nm. The inset shows the relative emission intensities at 550, 590, and 613 nm. | |
Fig. 6 shows energy levels of the possible energy transfer mechanism between Tb3+ and Eu3+. As depicted in Fig. 6, the Tb3+ electrons in the 7F6 ground state energy level can be excited by n-UV light and jump to the 5D3 excited state level. Then, they relax to the 5D4 transition state due to multi-phonon relaxation. As is known, the excited states are not stable, and Tb3+ can exhibit green light when the electrons leap back from 5D4 to 7FJ (J = 6, 5, 4, and 3) with non-radiative processes from 5D3 to 5D4. On the other hand, some Tb3+ electrons transfer their energy from the 5D4 (Tb3+) level to the excited state of Eu3+ (5D1 and 5D2) by cross-relaxation, and then the Eu3+ (5D1 and 5D2) electrons relax to the 5D0 level in a non-radiative process, resulting in 5D0–7FJ (J = 0, 1, 2, 3, and 4) transitions of Eu3+, which produce a red emission color.
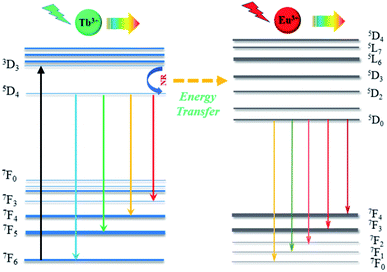 |
| Fig. 6 Energy levels of the possible energy transfer mechanism from Tb3+ to Eu3+. | |
In order to further understand the energy transfer mechanism of multi-polar interactions between the Tb and Eu ions in this system, the Dexter equation can be applied, as follows:33,34
in which
ηs0 is the quantum efficiency of Tb
3+ in the absence of Eu
3+and
ηs in the presence of Eu
3+,
C represents the total concentration of Tb
3+ and Eu
3+,
n is a constant value, 3, 6, 8 or 10, corresponding to the exchange, dipole–dipole, dipole–quadrupole and quadrupole–quadrupole interactions, respectively.
35,36 The value of
η0/
ηs can be approximately calculated from the ratio of the photoluminescence intensity (
Is0/
IS). Furthermore,
Fig. 7 shows the
Is0/
IS vs. Cn/3 (
n = 3, 6, 8, or 10) correlation diagram of these phosphors. When
n = 10, an optical linear relationship was observed, which corresponds to the quadrupole–quadrupole interaction between Tb
3+ and Eu
3+ in K
3LuF
6.
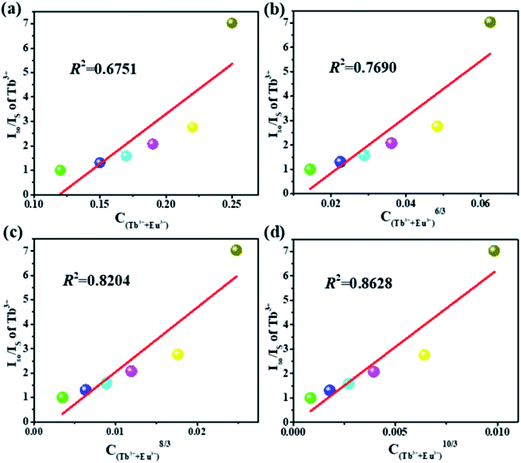 |
| Fig. 7 Dependence of Is0/IS of Tb3+ on (a) C, (b) C6/3, (c) C8/3 and (d) C10/3 for the K3LuF6:0.12Tb3+,xEu3+ (x = 0, 0.03, 005, 0.07, 0.1 and 0.13) samples. | |
The CIE chromaticity coordinate diagram of the K3LuF6:0.12Tb3+,xEu3+ (x = 0, 0.03, 005, 0.07, 0.1 and 0.13) phosphors under 375 nm excitation was calculated on the basis of the corresponding emission spectra, which are depicted in Table 1 and Fig. 8. As shown in Table 1 and Fig. 8, the emission color of K3LuF6:0.12Tb3+,xEu3+ can change from green (0.2781, 0.5407) to yellowish pink (0.4331, 0.3556) upon an increase in the concentration of Eu3+. Therefore, K3LuF6:0.12Tb3+,xEu3+ can be efficiently excited by 375 nm as an adjustable luminescence material for use in n-UV LEDs.
Table 1 The CIE chromaticity coordinates of the K3LuF6:0.12Tb3+,xEu3+ (x = 0, 0.03, 005, 0.07, 0.10 and 0.13) samples under 375 nm excitation
No. |
K3LuF6:0.12Tb3+,xEu3+ |
(x, y) |
1 |
x = 0 |
(0.2781, 0.5047) |
2 |
x = 0.03 |
(0.3710, 0.4289) |
3 |
x = 0.05 |
(0.3812, 0.4069) |
4 |
x = 0.07 |
(0.3857, 0.4019) |
5 |
x = 0.10 |
(0.3958,0.3750) |
6 |
x = 0.13 |
(0.4331, 0.3556) |
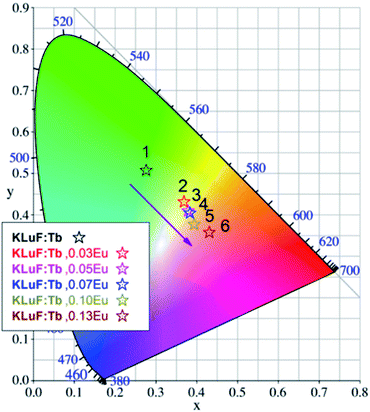 |
| Fig. 8 The CIE chromaticity coordinate diagram of the K3LuF6:0.12Tb3+,xEu3+ (x = 0, 0.03, 005, 0.07, 0.10 and 0.13) samples under 375 nm excitation. | |
4. Conclusions
In summary, single-phase K3LuF6:xTb3+ (x = 0.01, 0.03, 005, 0.07, 0.09, 0.1, 0.12 and 0.13) and K3LuF6:0.12Tb3+,xEu3+ (x = 0.03, 005, 0.07, 0.1 and 0.13) samples were successfully prepared via a high temperature solid-state phase sintering method. In order to determine the energy transfer mechanism between the Tb3+ and Eu3+ ions, the photoluminescence properties and decay lifetimes were measured, and the results showed an energy transfer mechanism from Tb3+ to Eu3+ in K3LuF6, determined as a quadrupole–quadrupole interaction. Characteristic emissions for both Tb3+ and Eu3+ can be observed in the PL spectrum of the co-doped phosphors at an excitation of 375 nm (Tb3+), while the luminescent colors of the K3LuF6:0.12Tb3+,xEu3+ samples can be regulated by changing the concentration of Eu3+. All of the above results show that the phosphor could be an ideal single-phase multicolor phosphor.
Conflicts of interest
There are no conflicts to declare.
Acknowledgements
This present work is supported by the National Natural Science Foundation of China (Grant No. 41672044).
References
- M. Runowski and S. Lis, Synthesis of lanthanide doped CeF3:Gd3+, Sm3+ nanoparticles, exhibiting altered luminescence after hydrothermal post-treatment, J. Alloys Compd., 2016, 661, 182–189 CrossRef CAS.
- M. Runowski, J. Marciniak, T. Grzyb, D. Przybylska, A. Shyichuk, B. Barszcz, A. Katrusiak, S. Lis, A. Katrusiak and S. Lis, Lifetime Nanomanometry – High-Pressure Luminescence of Up-converting Lanthanide Nanocrystals-SrF2:Yb3+, Er3+, Nanoscale, 2017, 9, 16030–16037 RSC.
- L. Qin, P. Q. Cai, C. L. Chen, J. Wang, S. Kim, Y. L. Huang and H. J. Seo, Optical performance of the Ba5Al3F19:Eu2+ blue phosphors with high thermal stability, J. Alloys Compd., 2018, 738, 372–378 CrossRef CAS.
- V. D. Shcherbakov and A. S. Nizamutdinov, Study of Mn2+ luminescence in β-PbF2, J. Lumin., 2019, 194, 321–326 Search PubMed.
- T. T. Deng, E. H. Song and Y. Y. Zhou, Tailoring photoluminescence stability in double perovskite red phosphors A2BAlF6:Mn4+ (A = Rb, Cs; B = K, Rb) via neighboring-cation modulation, J. Mater. Chem. C, 2017, 5, 12422–12429 RSC.
- D. Yang, L. B. Liao, Q. F. Guo, L. J. Wang, L. F. Mei, H. K. Liu and T. S. Zhou, A novel phosphor of Eu3+-activated Na3GaF6: Synthesis, structure, and luminescence properties, J. Lumin., 2019, 194, 321–326 Search PubMed.
- T. T. Deng, E. H. Song and Y. Y. Zhou, Stable narrowband red phosphor K3GaF6:Mn4+ derived from hydrous K2GaF5 (H2O) and K2MnF6, J. Mater. Chem. C, 2017, 5, 9588–9596 RSC.
- S. K. Saroj, P. Rawat, M. Gupta, G. V. Prakash and R. Nagarajan, Double perovskite K3InF6 as upconversion phosphor and its structural transformation by rubidium substitution, Eur. J. Inorg. Chem., 2018, 44, 4826–4833 CrossRef.
- J. K. Cao, F. F. Hu, L. P. Chen, H. Guo, C. K. Duan and M. Yin, Wide-range thermometry based on green up-conversion luminescence of K3LuF6:Yb3+/Er3+ bulk oxyfluoride glass ceramics, J. Am. Ceram. Soc., 2017, 100, 2108–2114 CrossRef CAS.
- P. Du and J. S. Yu, Self-activated multicolor emissions in Ca2NaZn2(VO4)3:Eu3+ phosphors for simultaneous warm white light-emitting diodes and safety sign, Dyes Pigm., 2017, 147, 16–23 CrossRef CAS.
- C. L. Liao, R. P. Cao, W. D. Wang, W. Hu, G. T. Zheng, Z. Y. Luo and P. Liu, Photoluminescence properties and energy transfer of NaY(MoO4)2:R (R =Sm3+/Bi3+, Tb3+/Bi3+, Sm3+/Tb3+) phosphors, Mater. Res. Bull., 2018, 97, 490–496 CrossRef CAS.
- B. Han, Y. Z. Dai, J. Zhang, B. B Liu and H. Z. Shi, Development of near-ultraviolet-excitable single-phase white-light-emitting phosphor KBaY(BO3)2:Ce3+,Dy3+ for phosphor-converted white light emitting-diodes, Ceram. Interfaces, 2018, 44, 14803–14810 CrossRef CAS.
- Q. F. Guo, C. L. Zhao, Z. Q. Jiang, L. B. Liao, H. K. Liu, D. Yang and L. F. Mei, Novel emission-tunable oxyapatites-type phosphors: Synthesis, luminescent properties and the applications in white light emitting diodes with higher color rendering index, Dyes Pigm., 2017, 139, 361–371 CrossRef CAS.
- B. Li, X. Y. Huang and J. Lin, Single-phased white-emitting Ca3Y(GaO)3(BO3)4: Ce3+,Tb3+,Sm3+ phosphors with high-efficiency: Photoluminescence, energy transfer and application in near-UV-pumped white LEDs, J. Lumin., 2019, 205, 115–121 CrossRef.
- P. L. Li, Z. J. Wang, Q. L. Guo and Z. P. Yang, Luminescence and energy transfer of 432 nm blue LED radiation-converting phosphor Ca4Y6O(SiO4)6: Eu2+, Mn2+ for warm white LEDs, RSC Adv., 2015, 5, 4448–4453 RSC.
- L. Li, X. H. Tang, Z. J. Wu, Y. F. Zheng, S. Jiang, X. Tang, G. T. Xiang and X. J. Zhou, Simultaneously tuning emission color and realizing optical thermometry via efficient Tb3+/Eu3+ energy transfer in whitlockite-type phosphate multifunctional phosphors, J. Alloys Compd., 2018, 780, 266–275 CrossRef.
- Y. L. Zhu, Y. J. Liang, M. F. Zhang, M. H. Tong, G. G. Li and S. Wang, Structure, luminescence properties and energy transfer behavior of color-adjustable Sr3Gd2(Si3O9)2: Ce3+, Tb3+/Mn2+ phosphors, RSC Adv., 2015, 5, 98350–98360 RSC.
- C. Y. Xu, Y. H. Song, H. X. Guan, Y. Sheng, P. C. Ma, X. Q. Zhou, Z. Shi and H. F. Zou, The photoluminescence, thermal properties and tunable color of Na1−xAl1+2xSi1−2xO4: xCe3+/Tb3+/Dy3+ via energy transfer: a single-component multicolor-emitting phosphor, Phys. Chem. Chem. Phys., 2017, 19, 22197–22209 RSC.
- K. Li and R. V. Deun, Photoluminescence and energy transfer properties of a novel molybdate KBaY(MoO4)3:Ln3+ (Ln3+ = Tb3+, Eu3+, Sm3+, Tb3+/Eu3+, Tb3+/Sm3+) as a multi-color emitting phosphor for UV w-LEDs, Dalton Trans., 2018, 47, 6995–7004 RSC.
- M. M. Jiao, Q. F. Xu, M. L. Liu, C. L. Yang and Y. J. Yu, Efficient green phosphor realized by Ce3+→Tb3+ energy transfer in Li3Sc2(PO4)3 for ultraviolet white light-emitting diodes, Phys. Chem. Chem. Phys., 2018, 20, 26995–27002 RSC.
- L. Li, W. X. Chang, W. Y. Chen, Z. S. Feng, C. L. Zhao, P. F. Jiang, Y. J. Wang, X. J. Zhou and A. Suchocki, Double perovskite LiLaMgWO6:Eu3+ novel red-emitting phosphors for solid state lighting: Synthesis, structure and photoluminescent properties, Ceram. Int., 2017, 43, 2720–2729 CrossRef CAS.
- S. D. Li, Q. Y. Meng, S. C. Lü and W. J. Sun, Study on optical temperature sensing properties of Tb3+, Eu3+ co-doped CaMoO4 phosphor, J. Lumin., 2018, 200, 103–110 CrossRef CAS.
- X. X. Ma, L. B. Liao, Q. F. Guo, H. K. Liu, T. S. Zhou and L. F. Mei, Luminescence properties and energy transfer investigations of Ba2La2.85−xTb0.15Eux(SiO4)3F multicolor phosphor, RSC Adv., 2018, 8, 27332–27341 RSC.
- X. M. Zhu and Z. F. Zhou, Photoluminescence and energy transfer mechanism of a novel tunable color phosphor Na2MgSiO4:Tb3+, Eu3+, J. Lumin., 2017, 188, 589–594 CrossRef CAS.
- S. Lee and S. Park, Preparation and luminescent properties of Tb3+ and Tb3+–Ce3+ doped Ba9Y2Si6O24 phosphors, J. Lumin., 2013, 143, 215–218 CrossRef CAS.
- Z. X. Shi, J. Wang and X. Guan, Upconversion multicolor tuning of NaY(WO4)2:Tb3+ with Eu3+ doping, J. Rare Earths, 2018, 36, 911–916 CrossRef CAS.
- M. M. Shang, S. Huang and J. Lin, Multicolor emissions and photoluminescence properties for Ca3Al4ZnO10:Ce3+/Eu3+/Tb3+/Mn2+ phosphors, J. Lumin., 2018, 204, 493–498 CrossRef CAS.
- T. A. Safeera and E. I. Anila, An investigation on the luminescence quenching mechanism of ZnGa2O4:Tb3+ phosphor, J. Lumin., 2019, 205, 277–281 CrossRef CAS.
- X. Y. Li, X. T. Wei, Y. G. Qin, Y. H. Chen, C. K. Duan and M. Yin, The emission rise time of BaY2ZnO5:Eu3+ for non-contact luminescence thermometry, J. Alloys Compd., 2016, 657, 353–357 CrossRef CAS.
- X. J. Zhou, L. N. Chen, S. Jiang, G. T. Xiang, L. Li, X. Tang, X. B. Luo and Y. Pang, Eu3+ activated LiSrVO4 phosphors: Emission color tuning and potential application in temperature sensing, Dyes Pigm., 2018, 151, 219–226 CrossRef CAS.
- F. F. Hu, Z. M. Zhao, F. F. Chi, X. T. Wei and M. Yin, Structural characterization and temperature-dependent luminescence of CaF2:Tb3+/Eu3+ glass ceramics, J. Rare Earths, 2017, 35, 536–541 CrossRef CAS.
- Z. H. Li, H. Y. Ma, N. Li, Y. Du and Q. Y. Shao, New phosphors of β-BaB2O4:RE3+ (RE3+ = Eu3+, Tb3+), J. Alloys Compd., 2018, 747, 340–347 CrossRef CAS.
- L. G. Van Uitert, Characterization of energy transfer interactions between rare earth ions, J. Electrochem. Soc., 1967, 114, 1048–1053 CrossRef CAS.
- D. L. Dexter and J. H. Schulman, Theory of Concentration Quenching in Inorganic Phosphors, J. Chem. Phys., 1954, 22, 1063–1070 CrossRef CAS.
- X. Zhang, P. He, L. Zhou, J. Shi and M. Gong, Energy transfer and luminescent properties of a green-to-red colour tunable Tb3+, Eu3+ co-doped K2Y(WO4)(PO4) phosphor, Mater. Res. Bull., 2014, 60, 300–307 CrossRef CAS.
- B. Li, B. Devakumar, L. W. Jin, L. L. Sun and X. Y. Huang, Synthesis, energy transfer and photoluminescence properties of thermal stable multicolour-emitting Ca3Gd(AlO)3(BO3)4: Tb3+, Eu3+ phosphors, J. Lumin., 2018, 204, 386–393 CrossRef CAS.
Footnote |
† Electronic supplementary information (ESI) available. See DOI: 10.1039/c8ra10059d |
|
This journal is © The Royal Society of Chemistry 2019 |
Click here to see how this site uses Cookies. View our privacy policy here.