DOI:
10.1039/C8RA09090D
(Paper)
RSC Adv., 2019,
9, 7777-7785
Preparation of glycoside polymer micelles with antioxidant polyphenolic cores using alkylated poly(arbutin)s†
Received
2nd November 2018
, Accepted 27th February 2019
First published on 8th March 2019
Abstract
This paper describes the synthesis of long-chain-alkylated poly(arbutin)s (poly(Arb)-Rx, where R = alkyl-chain length and x = degree of substitution (DS)) and their aqueous micelle formation. DS was controlled by tailoring the alkyl reagent/main-chain phenol substituent feed ratio. The critical micelle concentrations (CMCs) of poly(Arb)-Rx were determined as 1.3–5.2 mg mL−1 by the surface tension method. Introduction of longer alkyl substituents decreased CMC and also decreased aqueous solubility. In DLS measurement, the average micelle diameters were 225–616 nm, and micelle size decreased with increasing DS because of increased stabilization by hydrophobic alkyl substituents. Transmission electron microscopy indicated that mainly wormlike cylindrical micelles were formed, even with highly hydrophilic polymers. The alkylated polymer exhibited no cytotoxicity, and their antioxidant abilities were evaluated by the β-carotene bleaching method. Only 0.049 mol equivalents of poly(Arb)-C830 to linoleic acid was sufficient to preserve the β-carotene.
Introduction
The glycoside β-arbutin (βArb), shown in Scheme 1, is one of the main polyphenol components of pear fruit, which is cultivated throughout the world.1,2 It has the ability to bind the reactive sites of tyrosinase without being oxidized, making it a potent antagonistic tyrosinase inhibitor and thus preventing the formation of melanin. Furthermore, it is completely nontoxic to humans. Thus, it is often applied in cosmetics as a skin-whitening agent.3,4
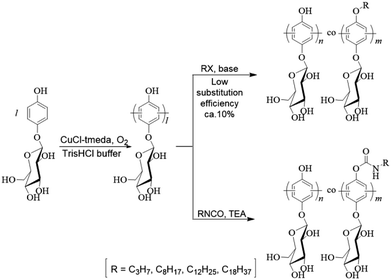 |
| Scheme 1 Synthesis of poly(Arb)-Rx. | |
Phenolic compounds such as tyrosine and catechin are ubiquitous in nature and play important roles in the metabolism of living organisms. Among these phenolic compounds, polyphenols such as catechin have attracted significant research attention owing to their strong antioxidant properties and potential anticancer activities.5,6 They also exhibit anticarcinogenic, antimicrobial, and anti-inflammatory properties,7 making them of enormous benefit for the prevention of disease.
Amphiphilic block copolymers are useful materials in variety of fields. Especially they can form micelles in a specific solvent, which make it possible to function as a drug carrier.8–10 Numerous efforts have been paid to study the micelle formulation ability and the morphology by the use of various kinds of amphiphilic block copolymers.11–13 Inspired by natural compounds, polyphenol derivatives loaded within synthetic polymer micelles were prepared for anti-cancer reagent14 and dermatitis treatment.15 Encapsulation of polyphenols within casein were also reported as a potential usage as a protein-based delivery systems for incorporating hydrophobic nutraceuticals into fat-free clear beverages.16
As development of novel polyphenol compounds, a number of studies on the enzyme-catalyzed oxidative polymerization of phenol derivatives via C–C or C–O coupling have been published,17–19 and a significant number of studies on the polymerization of βArb have been reported.20–25 Unfortunately, the selectivity of C–C coupling over C–O coupling for βArb systems was found to be generally poor, and the number average molecular weight (Mn) of the resulting polymers was limited to 3200, most likely due to the occurrence of enzyme-catalyzed equilibrium polymerization.
We have recently reported the selective C–C oxidative polymerization of βArb using a conventional copper catalyst in a tris(hydroxymethyl)aminomethane hydrogen chloride (Tris–HCl, pH = 9.0) buffer solution to give the corresponding poly(Arb) with Mn values reaching 7200.26 This polymer possesses both a phenolic and a glucose component in each repeating unit; therefore, in aqueous media, it can form a structure in which the polyphenol backbone be wrapped in hydrophilic glucose moieties.
Unlike the enzyme-catalyzed polymerization products mentioned above, the poly(Arb) prepared by our chemical oxidative reaction method exhibits relatively high Mn values and near-perfect C–C coupling selectivity, making it suitable for the preparation of antioxidant micelles coated with glucose moieties. Therefore, we envisioned that this polymer would have great potential for biomedical applications.
Accordingly, in an attempt to tailor the hydrophobicity of the micelle cores and thus their macroscopic properties, we investigated the introduction of n-octyl groups to poly(Arb) via SN2 reactions between n-octyl bromide and the phenolic group. However, a degree of substitution (DS) of only 10% was achieved. Nevertheless, we prepared micelles using the resulting polymer and demonstrated that they could be loaded with pyrene.26
Encouraged by these results and our belief that these novel polymers would be useful in various biomedical applications owing to their unique glucose-wrapped polyphenol structures, we attempted to develop a more effective approach toward the preparation of alkylated poly(Arb)s, hereafter termed poly(Arb)-Rx, where R is the length of the substituted alkyl chain and x denotes DS. We also attempted to develop a reliable method for the determination of their critical micelle concentrations (CMCs).
Herein, we report an effective synthetic approach to the poly(Arb)-Rx species involving the addition of alkyl isocyanates (Scheme 1) and the influence of their structures on micelle formation, as indicated by the surface tension method, dynamic light scattering (DLS) measurements, and transmission electron microscopy (TEM) observations. Furthermore, the cytotoxicity and antioxidant properties of the alkylated polymers were evaluated using the 3-[4,5-dimethylthiazol-2-yl]-2,5-diphenyltetrazolium bromide (MTT) and β-carotene bleaching assays, respectively.
Results and discussion
Polymer synthesis
Poly(Arb) was prepared according to our previously reported procedure (Scheme 1s†). The oxidative reaction was confirmed to proceed by C–C formation using IR, 1H NMR, and 13C NMR analysis. Briefly, in the IR spectra (Fig. 1s†), representative tetrasubstituted benzene C–H bending is observed for poly(Arb), and disubstituted C–H absorption appears for monomeric Arb. The analysis of 1H NMR spectrum (Fig. 2s†) indicates that selective C–C oxidative polymerization proceeded. In the 13C NMR spectrum, the almost equal reduction in the intensities of the two signals corresponding to the tertiary aromatic carbon signals after polymerization indicates that a radical addition reaction accompanying the typical coupling reaction occurred (Fig. 3s†). Therefore, the C–C reaction occurs both ortho and meta to the OH moiety.
Poly(Arb) was then reacted with alkyl isocyanate to give the corresponding poly(Arb)-Rx polymers in NMP in the presence of triethylamine (TEA, 1.2 equiv. to phenolic OH group) as a phenolic proton scavenger at 25 °C for 18 h.
Fig. 1 shows the 1H NMR spectra of (a) Arb, (b) poly(Arb), and (c) poly(Arb)-C810 in DMSO-d6. The feed ratio of octyl isocyanate to the phenolic OH group in poly(Arb) was set to 0.1
:
1, leading to a DS of 7.3% (substitution efficiency 7.3 ÷ 10 × 100 = 73%), as derived from the integration values of the octyl CH3 and aromatic CH protons. Thus, unlike the SN2 reaction using octyl bromide, which had a substitution efficiency of ≈10%, the simple addition used in the current study proceeds in very good efficiency. Therefore, we varied the feed ratio of octyl isocyanate to phenolic group in poly(Arb) from 0.1
:
1 to 0.9
:
1. As shown in 1H NMR spectra (Fig. 6s†), the signal integration of the terminal methyl group at 0.848 ppm increased with increasing the feed ratio, and the calculated DS values showed good agreement with the expected ones calculated from the feed ratio. It should be noticeable that the signal integration assignable to OH moieties in glucose unit observed at around 5 pm is constant to be about 5, indicating the alkylation was selectively occurred at phenolic OH group. Alkylation of poly(Arb) using various alkyl isocyanates for poly(Arb)-Rx samples are summarized in Table 1.
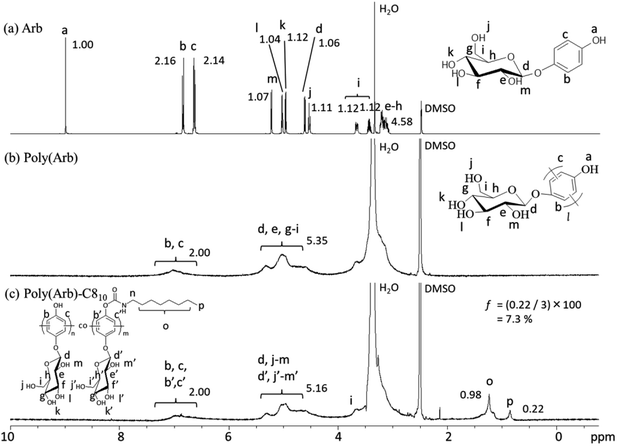 |
| Fig. 1 1H NMR spectra of (a) Arb, (b) poly(Arb), and (c) poly(Arb)-C810 in DMSO-d6. | |
Table 1 Alkylation of poly(Arb) using alkyl isocyanates (RNCO)
Run |
Polym.a |
Yield (%) |
Mnb (kDa) |
Mw/Mnb |
OH in poly(Arb) : RNCO |
Feed |
DSc |
The Mns of the starting poly(Arb)-C8x, poly(Arb)-C12x, and poly(Arb)C3x were 5950, 5950, and 4180, respectively. Determined by GPC (NMP with LiBr, PSt standards). Degree of substitution of the OH group calculated by 1H NMR (400 MHz, DMSO-d6). |
1 |
Poly(Arb)-C810 |
63.4 |
6.47 |
1.55 |
1 : 0.10 |
7.3 |
2 |
Poly(Arb)-C820 |
63.7 |
6.64 |
1.53 |
1 : 0.20 |
20.0 |
3 |
Poly(Arb)-C830 |
85.5 |
6.50 |
1.54 |
1 : 0.30 |
33.1 |
4 |
Poly(Arb)-C840 |
68.3 |
6.57 |
1.52 |
1 : 0.40 |
44.3 |
5 |
Poly(Arb)-C850 |
83.1 |
6.90 |
1.41 |
1 : 0.50 |
54.2 |
6 |
Poly(Arb)-C870 |
78.6 |
7.11 |
1.38 |
1 : 0.70 |
74.0 |
7 |
Poly(Arb)-C890 |
70.3 |
7.18 |
1.39 |
1 : 0.90 |
98.7 |
8 |
Poly(Arb)-C310 |
49.0 |
4.35 |
1.58 |
1 : 0.10 |
11.1 |
9 |
Poly(Arb)-C330 |
30.9 |
4.53 |
1.56 |
1 : 0.30 |
25.0 |
10 |
Poly(Arb)-C350 |
57.9 |
4.81 |
1.53 |
1 : 0.50 |
41.9 |
11 |
Poly(Arb)-C1210 |
61.7 |
6.34 |
1.56 |
1 : 0.10 |
9.8 |
12 |
Poly(Arb)-C1230 |
47.6 |
7.29 |
1.52 |
1 : 0.30 |
23.1 |
13 |
Poly(Arb)-C1250 |
66.9 |
7.47 |
1.54 |
1 : 0.50 |
31.3 |
14 |
Poly(Arb)-C1810 |
65.3 |
7.78 |
1.46 |
1 : 0.10 |
8.4 |
15 |
Poly(Arb)-C1830 |
75.6 |
8.01 |
1.45 |
1 : 0.30 |
16.0 |
16 |
Poly(Arb)-C1850 |
78.6 |
8.20 |
1.67 |
1 : 0.50 |
23.2 |
Thus, the DS value is controllable by changing the feed ratio of the reactant, and the Mn value of the finally obtained polymer increases with the DS value consistently. Higher DS values were occasionally observed (e.g., run 7), probably due to the recovery of a low-molecular-weight fraction of poly(Arb)-C890 during the purification process. When the alkylation was conducted using isocyanate having long alkyl chains such as C12 and C18, the resultant DS values were lower than the expected ones (runs 12, 13, 15, and 16). This can be explained that the alkyl isocyanates having long alkyl group have strong hydrophobic compound, and thus immiscible with NMP solvent. Based on these results, we especially targeted the alkylated polymers having relatively low hydrophobic part for the following studies.
Table 2 summarizes the solubility testing results for the poly(Arb)-Rx samples. Essentially, the solubilities of the alkylated polymers are not significantly changed. However, the solubility in water decreases with DS.
Table 2 Solubilities of Arb, poly(Arb) and poly(Arb)-C8xa
Polymer |
DMSO |
NMP |
THF |
Acetone |
CHCl3 |
MeOH |
H2O |
Polymer 10 mg per solvent 5 mL (++ soluble at room temperature; + soluble after heating; ± partially soluble; − insoluble). DMSO: dimethyl sulfoxide; DMF: N,N-dimethylformamide; NMP: N-methyl-2-pyrrolidone; THF: tetrahydrofuran; CHCl3: chloroform; MeOH: methanol; H2O: water. |
Arb |
++ |
++ |
++ |
++ |
− |
++ |
++ |
Poly(Arb) |
++ |
++ |
− |
− |
− |
− |
++ |
Poly(Arb)-C810 |
++ |
++ |
− |
− |
− |
± |
++ |
Poly(Arb)-C830 |
++ |
++ |
− |
− |
− |
± |
++ |
Poly(Arb)-C850 |
++ |
++ |
− |
− |
− |
± |
++ |
Poly(Arb)-C870 |
++ |
++ |
− |
− |
− |
± |
++ |
Poly(Arb)-C890 |
++ |
++ |
± |
± |
± |
± |
± |
Generally, when an amorphous polymer is mixed with a suitable solvent, it completely dissolves in the media by expanding the polymer coil from its unperturbed dimensions to the extent of the liquid–polymer interactions. However, in the case of poly(Arb)-Rx dissolved in water or organic solvents, the interactions will be different owing to the largely amphiphilic nature of the polymers. Consequently, the chain dimensions in these solvents will be different.
Fig. 2 shows the 1H NMR spectra of poly(Arb)-C830 recorded in D2O, DMSO, and mixtures thereof. DMSO is a good solvent for polyphenol units and the pendant glucose units; thus, all the signals appear clearly, as shown in Fig. 2a. Conversely, water is a good solvent only for the glucose moieties; hence, the polymer must transform into a globular form with the glucose moieties on the outer surface, resulting in micelle formation. Owing to the restricted and crowded structure, the signals for the octyl chains and the glucose groups in water are broadened and weaker. Naturally, poly(Arb) itself can adopt a similar structure on mixing with water. However, the poly(Arb)-Rx structures are more stable in water because of interactions between the hydrophobic alkyl substituents in the core.
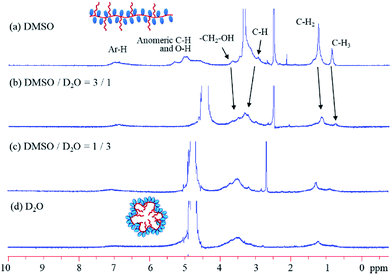 |
| Fig. 2 1H NMR spectra of poly(Arb)-C830 in D2O, DMSO-d6, and mixtures thereof. | |
To further investigate the micelle formation abilities of the polymers, we used surface tension measurements to obtain their CMCs.
Micelle formulation
The fluorescence probe method is a well-known technique for determining the CMCs of amphiphilic block copolymers. Poly(Arb) itself exhibits broad absorption up to 380 nm (Fig. 4s†), making it difficult to accurately obtain its CMCs by observing the fluorescence of the chromophore. Therefore, we used the surface tension method. Fig. 3 illustrates the relationship between surface tension and concentration for the poly(Arb) and poly(Arb)-C8x samples with different DS values in DI water. As expected, the surface tension of the poly(Arb) homopolymer solution is largely independent of concentration. Conversely, the poly(Arb)-C8x samples show behaviour typical of conventional amphiphiles, i.e., the surface tension of the solution decreases with polymer concentration, and the slope becomes more moderate at a specific concentration, the so-called CMC. The CMC and critical surface tension (γc) values are summarized in Table 3. Increasing the DS values of the poly(Arb)-C8x samples leads to a balance between the hydrophobic and hydrophilic segments, and thus the CMC gradually decreases from 4.8 mg mL−1 for C810 to 1.3 mg mL−1 for C830. However, additional alkylation results in an increase in CMC.
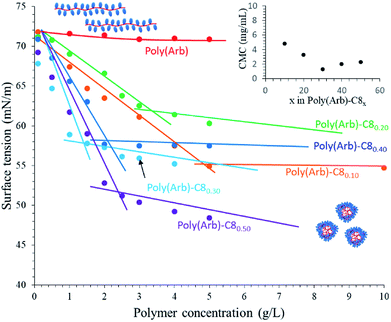 |
| Fig. 3 Effect of DS on the relationship between surface tension and poly(Arb)-C8x concentration. | |
Table 3 CMCs and critical surface tensions (γc) of poly(Arb)-C8xa
Polym. |
OH in poly(Arb) : RNCO |
CMC (mg mL−1) |
γc (mN m−1) |
Feed |
DS |
Calculated by 1H NMR (400 MHz, DMSO-d6). |
Poly(Arb)-C810 |
1 : 0.10 |
7.3 |
4.8 |
55.5 |
Poly(Arb)-C820 |
1 : 0.20 |
20.0 |
3.3 |
62.0 |
Poly(Arb)-C830 |
1 : 0.30 |
33.1 |
1.3 |
57.0 |
Poly(Arb)-C840 |
1 : 0.40 |
44.3 |
2.0 |
58.0 |
Poly(Arb)-C850 |
1 : 0.50 |
54.2 |
2.2 |
52.0 |
As described in the solubility tests (Table 2), poly(Arb)-C870 and poly(Arb)-C890 both exhibit poor solubility in water; therefore, excess alkylation may endow the polymer with an overly hydrophobic amphiphilic balance.
Fig. 4 shows the relationship between surface tension and concentration for polymers with alkyl substituents from C3 to C18 in DI water. The CMC values decrease from 5.2 to 3.4 mg mL−1 with increasing alkyl-chain length (Table 4). Thus, longer alkyl substituents allow the formation of more stable micelles at lower polymer concentrations owing to their optimal amphiphilic balance. Conversely, there seems to be no relationship between γc and alkyl-chain length. Considering the γc values of polyethylene (γc = 34.4),27 the concentration of alkyl groups (i.e., the DS value) as well as the terminal CH3 group should be considered. However, in our poly(Arb)-Rx samples, the substitution position cannot be fully controlled, and thus the trend in the γc values cannot be properly explained.
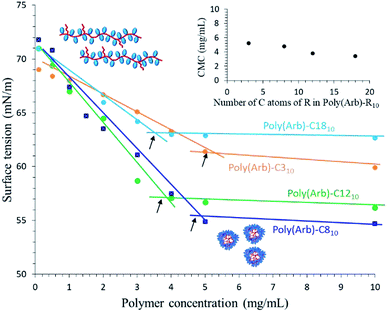 |
| Fig. 4 Effect of alkyl substituents on the relationship between surface tension and poly(Arb)-Rx concentration. | |
Table 4 CMCs and critical surface tensions (γc) of poly(Arb)-Rxa
Polym. |
OH in poly(Arb) : RNCO |
CMC (mg mL−1) |
γc (mN m−1) |
Feed |
DS |
Calculated by 1H NMR (400 MHz, DMSO-d6). |
Poly(Arb)-C310 |
1 : 0.10 |
11.1 |
5.2 |
61.2 |
Poly(Arb)-C810 |
1 : 0.10 |
7.3 |
4.8 |
55.5 |
Poly(Arb)-C1210 |
1 : 0.10 |
9.8 |
3.8 |
57.0 |
Poly(Arb)-C1810 |
1 : 0.10 |
8.4 |
3.4 |
63.0 |
Fig. 5 shows the results of DLS analysis of the prepared micelles in water. Clearly, for both the C3 and C8 samples, the diameters of the micelles decrease on increasing DS, whereas the size distribution for the C3 sample are broad. Poly(Arb) itself is highly water soluble owing to its hydrophilic glucose moieties, and thus short C3 substituent does not endow it with a sufficiently hydrophobic amphiphilic balance to form monodisperse stable micelles. This is largely because the C3 group is smaller than the glucose pendant, and thus the alkyl substituents cannot effectively aggregate in the core. Therefore, the micelle size distributions for poly(Arb)-C3x are much broader than those for poly(Arb)-C8x. This is also indicated by the lower CMCs of the poly(Arb)-C3x samples, as shown in Table 4.
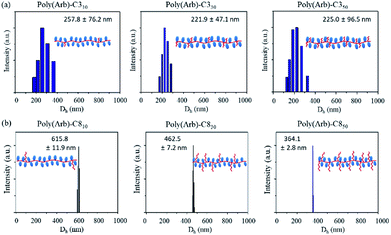 |
| Fig. 5 Effect of R ((a) n-propyl (C3) and (b) n-octyl (C8)) on micelle size in water. | |
We used TEM to investigate the micelle morphologies in a dry condition. Fig. 6 shows images of micelles formed by poly(Arb) samples with different R and DS values. Generally, the morphologies of the micelles from amphiphilic block copolymers are primarily a result of their inherent molecular curvature, i.e., the packing parameter p, where p ≤ 1/3 is a spherical micelle, 1/3 ≤ p ≤ 1/2 is a cylindrical micelle, and 1/2 ≤ p ≤ 1 is a vesicle.28 Thus, the primarily hydrophilic poly(Arb)-C310 should form a spherical micelle. However, the micelles of poly(Arb)-C310 are wormlike structures, and some aggregates are observed. Previously, Satoh et al. prepared amphiphilic rigid-rod polyisocyanates with a highly hydrophilic segment, where the morphology of the formed micelle was needlelike instead of typically spherical.29 For the micelles formed from poly(Arb)-C310 and poly(Arb)-C350, thin (13.6 nm in diameter) to thick (63.3 nm in diameter) curled wormlike micelles were observed. However, the micelle morphology clearly changes from thick and wormlike to fiberlike when poly(Arb)-R10 (R = C8, C12, and C18) samples are used. These results can be explained by the fact that poly(Arb)-Rx consists of a rigid-rod polyphenylene main chain, which inhibits the formation of spherical micelles. The micelle diameters measured by DLS are larger than those observed in TEM; therefore the poly(Arb)-Rx polymer assemblies shrinks due to drying TEM condition.
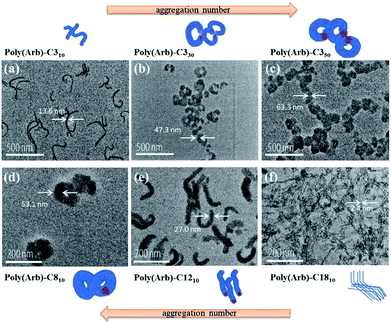 |
| Fig. 6 TEM images of (a) poly(Arb)-C310, (b) poly(Arb)-C330, (c) poly(Arb)-C350, (d) poly(Arb)-C810, (e) poly(Arb)-C1210, and (f) poly(Arb)-C1810, and their plausible aggregated structures. | |
Cytotoxicity assay
We then investigated their cytotoxicities using the poly(Arb)-C8x series in phosphate-buffered saline as a means of establishing their suitability for use as a drug carrier.
As shown in Fig. 7, regardless of DS of the poly(Arb)-C8x, the cell viability seems gradually decreased with increasing the polymer concentration, but the polymers showed almost no cytotoxicity below the concentration of 0.5 mg mL−1, probably because of the existence of the numerous glucose moieties. Although the polymers do not form micelles in this diluted experimental condition, it can be said that the poly(Arb)-Rx polymer itself is not toxic at all toward the L929 and HeLa cells.
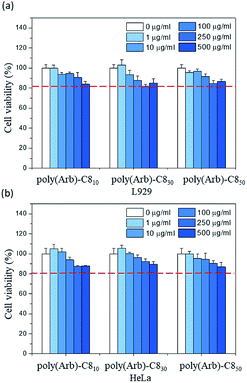 |
| Fig. 7 In vitro cell-viability assay results for poly(Arb)-C8x determined by MTT assays using (a) L929 (normal cells) and (b) HeLa (cancer cells). | |
β-Carotene bleaching assay
We envisioned that a unique property of the alkylated polymers prepared in this study would be their antioxidant properties. Our alkylated poly(Arb)-Rx samples form wormlike micelles, and the polymers show almost no cytotoxicity. Thus their antioxidant properties may provide an additional advantage for their use as a biomaterial. To evaluate their antioxidant abilities, we performed β-carotene bleaching assays using the auto-oxidation of linoleic acid. We first prepared poly(Arb)-C830 micelle solution at the concentration of 2.0 mg mL−1 containing β-carotene and linoleic acid, and tried to evaluate the bleaching assay. However, due to the strong absorption of UV-light by poly(Arb)-C830 itself, the characteristic absorption by β-carotene was completely concealed (Fig. 8s†). Thus, instead of the micelle the antioxidant property of poly(Arb)-C830 itself was studied. To encapsulate β-carotene, linoleic acid, and poly(Arb)-C830, the surfactant Tween 40 was used according to the literature.30 Fig. 8 illustrates the relationship between the normalized OD at 470 nm, which corresponds to the absorbance of β-carotene, and time. Although the control experiment (without poly(Arb)-C830) shows a clear decrease in OD (i.e., bleaching) with time, a very small amount of poly(Arb)-C830 (0.049 mol equivalents as compared to linoleic acid) effectively maintain the orange colour of β-carotene in the solution. This result clearly indicates that poly(Arb)-C830 itself effectively inhibits the radical addition of peroxidized linoleic acid (produced by auto-oxidation) to β-carotene in the hydrophobic environment supplied by Tween 40.
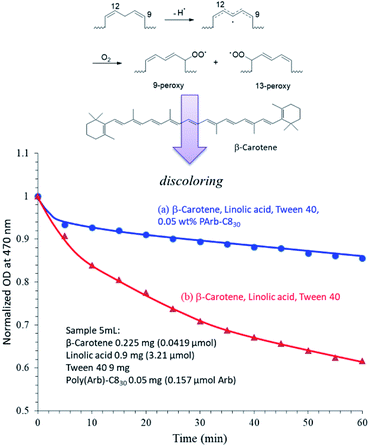 |
| Fig. 8 Monitoring β-carotene (0.0419 μmol; 5 mL) levels in the auto-oxidation of linoleic acid (3.21 μmol; 5 mL) with (a) and without (b) the poly(Arb)-C830 sample (0.157 μmol; 5 mL). | |
Experimental
Materials
Deionized (DI) water was obtained using an Iwaki Glass ASK-2DS water system (0.8 μS cm−1). Tris–HCl buffer solution (pH = 9.0) was prepared by mixing tris(hydroxymethyl)aminomethane solution and diluted hydrochloric acid at 20 °C. βArb and di-μ-hydroxo-bis[(N,N,N′,N′-tetramethylethylenediamine)copper(II)]chloride (CuCl-tmeda) complex were purchased from TCI (Japan). Poly(arb) was prepared and purified according to our previously reported method.17 Alkyl isocyanates and triethyl amine (TEA) were purchased from Wako Pure Chemical Industries (Japan) and used as received. Dialysis was performed using CellSepH1 (Membrane Filtration Products, Inc. MWCO: 1000 Da) against DI water for 2 d. Other reagents and solvents were used as provided.
Measurements
Fourier-transform infrared spectra were measured using a Jasco IR-5500 spectrometer (Jasco Co., Ltd.) from KBr tablets. 1H and 13C nuclear magnetic resonance (NMR) analysis was performed on a Bruker AC-400P spectrometer at 400 and 100 MHz, respectively. Deuterated water (D2O) and dimethyl sulfoxide (DMSO-d6) were used as the solvents. The number- and weight-average molecular weights (Mn and Mw, respectively) were measured using gel permeation chromatography (GPC) on a Tosoh HLC-8120 gel permeation chromatographer equipped with refractive index and UV (ultraviolet) detectors. Samples were separated by a consecutive polystyrene (PSt) gel column (TSK-GEL α-M × 2) at 40 °C. The samples were eluted with N-methylpyrrolidone (NMP) containing 0.01 mol L−1 lithium bromide at a flow rate of 0.7 mL min−1. Surface tension measurements was performed on a KYOWA interface measurement analysis system (DM300) with a plastic syringe (22G stainless needle) using the pendant drop method at 25 °C. The instrument was calibrated using standard PSt samples. DLS measurements were performed with an Otsuka ELSZ-2000 in DI water at 25.0 °C. The angle was fixed at 90°. TEM (JEM-2100, JEOL, Japan) was used to investigate the morphologies of the micelles.
Synthesis of poly(Arb)-Rx
Typical procedure for poly(Arb)-C850: poly(Arb) (Mn = 5950, Mw/Mn = 1.58, 0.200 g) dissolved in 1 mL NMP was placed in a two-necked flask equipped with a three-way stopcock. Into this solution, TEA (0.0899 g, 0.888 mmol) and octyl isocyanate (0.5 equiv., 0.0575 g, 0.370 mmol) were added, and the reaction was maintained for 18 h at 25 °C. The resultant solution was transferred into a dialysis membrane and dialyzed for 2 d against DI water. The crude product was obtained by freeze-drying and further purified by reprecipitation in hexane. The precipitate was collected and dried at 100 °C for 12 h to afford a pale brown powder. Yield: 83.1%. DS: 54.2%. Mn (GPC) = 6900, Mw/Mn (GPC) = 1.49. IR (KBr): ν 1039 (C–O, urethane), 1072 (C–OH, glucose), 1200 (C–O–C, glucose), 1616 (C
C, Ar), 1705 (C
O, urethane), 2853 (C–H, aliphatic), 2925 (C–H, Ar), 3423 (O–H) cm−1. 1H NMR (400 MHz, DMSO-d6): δ 7.06 (b, 2.0H, Ar C–H), 5.76–4.34 (b, 5.16H, glucose OH, anomeric C–H), 3.81–3.05 (b, overlapped with H2O, glucose C–H, CH2), 1.38–1.08 (b, 0.98H, octyl CH2), 0.848 (s, 0.22H, octyl CH3) ppm. 13C NMR (100 MHz, DMSO-d6, ppm) δ 156.15, 119.81, 103.26, 76.88, 76.55, 73.47, 69.47, 60.80, 45.54, 31.26, 28.73, 26.39, 22.12, 13.98, 9.00. Elemental analysis (C16.878H24.130N0.542O7.542) calculated C: 57.06, H: 6.60, N: 2.14 (%); found C: 56.38, H: 7.17, N: 2.54 (%).
Micelle formulation study
Polymer micelles were prepared by dissolving poly(arb)-Rx (5 mg) in DMSO (0.5 mL), followed by the addition of DI water (5 mL, 0.167 mL min−1). The micelle solution was purified by dialysis against DI water for 5 d. Surface tension measurements were performed 10 times, and the average of the data was used. The CMC values of the poly(arb)-Rx samples were calculated from the crossover point in the plots between surface tension and polymer concentration. Size distribution analysis was performed using DLS. The physical structures of the micelles were observed by TEM. The dialyzed aqueous micelle solution was placed on a TEM grid and dried before measurement.
Cytotoxicity assay
HuCC-T1 human cholangiocarcinoma cells were purchased from the Health Science Research Resources Bank (Osaka, Japan). Cells were seeded into 96-well plates at a density of 5 × 104 cells per well and incubated for 24 h in 5% CO2 at 37 °C. After removing the culture medium (with serum), the wells were washed with phosphate buffer. Each well was then recharged with 100 μL of fresh Roswell Park Memorial Institute 1640 media (without fetal bovine serum) containing poly(Arb)-Rx micelles. The cells were incubated for an additional 32 h. Subsequently, 25 μL of MTT (3 mg mL−1) was added to each well. After 4 h, 100 μL of a sodium dodecyl sulphate (SDS)/HCl solution (SDS 10% w/v, 0.01 M HCl) was added to each well, and the absorbance was measured at 570 nm after 12 h (Infinite M200 Pro microplate reader, Tecan, Switzerland). Viable cells were expressed as a percentage compared with the control. The results were obtained from three different experiments and expressed as means ± standard deviation as an error range.
β-Carotene bleaching assay
This bleaching assay was performed according to a modification of Miller's procedure.21 Briefly, linoleic acid (0.20 mL, 100 mg mL−1 solution in chloroform), β-carotene (0.50 mL, 1 mg mL−1 solution in chloroform), and Tween 40 (1.00 mL, 200 mg mL−1 solution in chloroform) were mixed and stirred to homogeneity. The solvent was then removed under a gentle nitrogen stream, and DI water (100 mL) was added. A 45 mL aliquot of the resulting solution was mixed with phosphate buffer (4.0 mL, 0.2 mol L−1, pH 6.8) and stirred for 30 min. Into 4.90 mL of this solution, poly(Arb)-C830 solution (0.10 mL, 0.05 wt% in DI water) was added, and the resulting solution was heated to 50 °C. The OD at 470 nm (log10(T470/100)) was measured using a UV-Vis spectrometer. As a control experiment, 0.10 mL of DI water alone was added in the final step.
Conclusions
In this study, we demonstrated unique micelle formulation by alkylated poly(Arb) samples, which were obtained by the simple addition of alkyl isocyanates to the poly(Arb) backbone in the presence of TEA. The morphology of the micelles can be dictated by tailoring the alkyl-chain length and DS. Thin to thick wormlike micelles as well as fibrous micelles can be provided depending on the chain used. The micelles have structures comprising a surface shell of outwardly oriented glucose moieties and a core comprising the phenolic substituents. The alkylated polymers exhibit antioxidant properties and almost no cytotoxicity. Thus, this unique glucose-shelled polyphenol may be useful as a novel biomedical material.
Conflicts of interest
There are no conflicts to declare.
Acknowledgements
This work was supported by a JSPS KAKENHI Grant-in-Aid for Scientific Research (C) (Grant Number JP18K05211). The authors also thank to Mr Kuniaki Sasaki (technical staff in Iwate University) for TEM measurement. The authors would like to thank Enago (http://www.enago.jp) for the English language review.
References
- M. N. Clifford, Miscellaneous phenols in foods and beverages–nature, occurrence and dietary burden, J. Sci. Food Agric., 2000, 80, 1126–1137 CrossRef CAS.
- M. A. Christopher and F. P. Gregory, Renewable Resources and Enzymatic Processes to Create Functional Polymers: Adapting Materials and Reactions from Food Processing, J. Polym. Environ., 2002, 10, 77–84 CrossRef.
- W. Zhu and J. Gao, The use of botanical extracts as topical skin-lightening agents for the improvement of skin pigmentation disorders, J. Invest. Dermatol. Symp. Proc., 2008, 13, 20–24 CrossRef CAS PubMed.
- J. L. O'Donoghue and V. M. D. Dabt, Hydroquinone and its analogues in dermatology–a risk-benefit viewpoint, J. Cosmet., Dermatol. Sci. Appl., 2006, 5, 196–203 CrossRef PubMed.
- J. Jankun, S. H. Selman, R. Swiercz and E. SkrzypczakJankun, Why drinking green tea could prevent cancer, Nature, 1997, 387, 561 CrossRef CAS PubMed.
- A. Bordoni, S. Hrelia, C. Angeloni, E. Giordano, C. Guarnieri, C. M. Caldarera and P. L. Biagi, Green tea protection of hypoxia/reoxygenation injury in cultured cardiac cells, J. Nutr. Biochem., 2002, 13, 103–111 CrossRef CAS PubMed.
- R. Puupponen-Pimiä, L. Nohynek, C. Meier, M. Kähkönen, M. Heinonen, A. Hopia and K. M. Oksman-Caldentey, Antimicrobial properties of phenolic compounds from berries, J. Appl. Microbiol., 2001, 90, 494–507 CrossRef.
- N. Nishiyama and K. Kataoka, Nanostructured Devices Based on Block Copolymer Assemblies for Drug Delivery: Designing Structures for Enhanced Drug Function, Adv. Polym. Sci., 2006, 193, 67–101 CrossRef CAS.
- K. Osada, R. J. Christie and K. Kataoka, Polymeric micelles from poly(ethylene glycol)-poly(amino acid) block copolymer for drug and gene delivery, J. R. Soc., Interface, 2009, 6, 5325–5339 CrossRef PubMed.
- R. T. Chacko, J. Ventura, J. Zhuang and S. Thayumanavan, Polymer nanogels: a versatile nanoscopic drug delivery platform, Adv. Drug Delivery Rev., 2012, 64, 836–851 CrossRef CAS PubMed.
- L. Zhang and A. Eisenberg, Multiple morphologies of “crew-cut” aggregates of polystyrene-b-poly(acrylic acid) block copolymers, Science, 1995, 268, 1728–1731 CrossRef CAS PubMed.
- G. Widawski, M. Rawiso and B. Francois, Self-organized honeycomb morphology of star-polymer polystyrene films, Nature, 1994, 369, 387–389 CrossRef CAS.
- S. Jain and F. S. Bates, On the origins of morphological complexity in block copolymer surfactants, Science, 2003, 300, 460–464 CrossRef CAS PubMed.
- Y. Cai, J. Zhang, N. G. Chen, Z. Shi, J. Qiu, C. He and M. Chen, Recent Advances in Anticancer Activities and Drug Delivery Systems of Tannins, Med. Res. Rev., 2017, 37, 668–701 CrossRef PubMed.
- F. Zhou, Z. Song, Y. Wen, H. Xu, L. Zhu and R. Feng, Transdermal delivery of curcumin-loaded supramolecular hydrogels for dermatitis treatment, J. Mater. Sci.: Mater. Med., 2019, 30, 11 CrossRef PubMed.
- N. Ghayour, S. M. H. Hosseini, M. H. Eskandari, S. Esteghlal, A.-R. Nekoei, H. H. Gahruie, M. Tatar and F. Naghibalhossaini, Food Hydrocolloids, 2019, 87, 394–403 CrossRef CAS.
- S. Kobayashi and S. Kimura, Enzymatic Polymerization, Chem. Rev., 2001, 101, 3793–3818 CrossRef CAS.
- R. A. Gross, A. Kumar and B. Kalra, Polymer synthesis by in vitro enzyme catalysis, Chem. Rev., 2001, 101, 2097–2124 CrossRef CAS PubMed.
- S. Kadokawa, Polymer synthesis by enzymatic catalysis, Curr. Opin. Chem. Biol., 2010, 14, 145–153 CrossRef PubMed.
- P. Wang, B. D. Martin, S. Parida, D. G. Rethwisch and J. S. Dordick, Multienzymic Synthesis of Poly(hydroquinone) for Use as a Redox Polymer, J. Am. Chem. Soc., 1995, 117, 12885–12886 CrossRef CAS.
- P. Wang, S. Amarasinghe, J. Leddy, M. Arnold and J. S. Dordick, Enzymatically prepared poly(hydroquinone) as a mediator for amperometric glucose sensors, Polymer, 1998, 39, 123–127 CrossRef CAS.
- T. Kiso, S. Watase, Y. Kobayashi, M. Shizuma, H. Murakami, T. Kiryu and H. Nakano, Oxidative Polymerization of Phenolic Glycosides by Peroxidase, J. Appl. Glycosci., 2007, 54, 157–161 CrossRef CAS.
- T. Kiso, M. Shizuma, H. Murakami, T. Kiryu, K. Hozono, T. Terai and H. Nakano, Oxidative coupling reaction of arbutin and gentisate catalyzed by horseradish peroxidase, J. Mol. Catal. B: Enzym., 2007, 45, 50–56 CrossRef CAS.
- H. Nakano, M. Shizuma, H. Murakami, T. Kiryu and T. Kiso, One-pot synthesis of glycosyl poly(arbutin) by enzymatic glycosylation followed by polymerization with peroxidase, J. Mol. Catal. B: Enzym., 2005, 33, 1–8 CrossRef CAS.
- T. Kiso, T. Kiryu, H. Murakami and H. Nakano, Polymerization of 4'-Hydroxyphenyl α-Glucoside and 4'-Hydroxyphenyl β-Glucoside Catalyzed by Horseradish Peroxidase, J. Appl. Glycosci., 2007, 54, 181–186 CrossRef CAS.
- M. Hashimoto, S. Iwabuchi, Y. Oishi and Y. Shibasaki, Water-soluble polyphenol synthesis via oxidative coupling polymerisation of β-arbutin with copper catalyst in alkaline media, Eur. Polym. J., 2016, 81, 152–160 CrossRef CAS.
- K. Grundke and A. Augsburg, On the determination of the surface energetics of porous polymer materials, J. Adhes. Sci. Technol., 2000, 14, 765–775 CrossRef CAS.
- J. Israelachvili, Intermolecular & Surface Forces, Academic Press, London, 2nd edn, 1991 Search PubMed.
- N. Sakai, T. Satoh and T. Kakuchi, Rod-Like Amphiphile of Diblock Polyisocyanate Leading to Cylindrical Micelle and Spherical Vesicle in Water, Macromolecules, 2014, 47, 1699–1704 CrossRef CAS.
- H. E. Miller, A Simplified Method for the Evaluation of Antioxidants, J. Am. Oil Chem. Soc., 1971, 48, 91 CrossRef CAS.
Footnote |
† Electronic supplementary information (ESI) available: Scheme 1s, Fig. 1s–10s. See DOI: 10.1039/c8ra09090d |
|
This journal is © The Royal Society of Chemistry 2019 |