DOI:
10.1039/C8RA08967A
(Paper)
RSC Adv., 2019,
9, 1147-1150
A FRET based ratiometric fluorescent probe for detection of sulfite in food†
Received
30th October 2018
, Accepted 23rd December 2018
First published on 11th January 2019
Abstract
A new fluorophore pyrido[1,2-a]benzimidazole based ratiometric fluorescent probe for the selective detection of sulfite ions in water was investigated. It shows large (pseudo) Stokes shifts (260 nm), high FRET efficiency, high selectivity and sensitivity. A distinct color change from red to colorless was observed and importantly, it proves to be a convenient and efficient tool to detect the sulfite levels in sugar samples.
Introduction
Sulfites are widely applied in the production of foods, pharmaceutical products and beverages as antimicrobial agents, antioxidants and enzyme inhibitors.1 However, the levels of sulfites have been strictly controlled because of the harmful effects towards the human body, such as allergic reactions and food intolerance symptoms.2–4 Therefore, for food quality control and quality assurance, it is important to develop a low-cost, selective and sensitive method for sulfite determination.
Compared with several traditional methods such as electrochemistry and chromatography, fluorescent probes are particularly attractive due to their simplicity, high selectivity and high sensitivity.5 Many fluorescent probes are reported to detect sulfites based on the changes of emission intensity.6 However, the signal output of these intensity-based fluorescent probes can be affected by instrumental factors, environmental effects and probe concentrations.7 Therefore, ratiometric fluorescent probes which are independent of the environmental effects are more desirable.8–11 Also, they have large Stokes shifts which can prevent serious self-quenching and fluorescence detection errors. So far, only a few well-behaved ratiometric probes for sulfite have been reported.12–26 Herein, a new FRET based ratiometric fluorescent probe was designed (Scheme 1). A pyrido[1,2-a]benzimidazole fluorophore, due to its favorable photophysical properties, was selected as the donor. While a hemicyanine fluorophore of which the absorption band overlapped well the emission band of the donor was chosen as the acceptor (Fig. S1†).
 |
| Scheme 1 Synthesis of probe PBI-S. | |
Experimental
Reagents and instrumentation
RF-5301PC luminescence spectrophotometer (Shimadzu) and UV-2600 spectrometer (Shimadzu) were used to measure the fluorescence and UV-vis absorption spectra, respectively. NMR spectra were recorded on a Bruker Avance 400 (400 MHz) spectrometer (in CDCl3, TMS as an internal standard). All commercial reagents and solvents were used without further purification.
Preparation of the probe PBI-S
Compound 1 and compound 2 were synthesized according to the literature.27,28 To a solution of compound 1 (580 mg, 2 mmol) in 20 mL CH2Cl2 was added DMAP (60 mg, 0.4 mmol) and EDC (576 mg, 3.0 mmol). Then, compound 2 (744 mg, 12 mmol) was added. The mixture was stirred for 12 h at room temperature. After removal of the solvent under reduced pressure, the crude probe was obtained, which was purified flash silica gel chromatography (CH3OH
:
CH2Cl2 = 1
:
100) to afford pure probe PBI-S (purity: 98.6%, 456 mg, yield: 71%). 1H NMR (400 MHz, CDCl3) δ 8.55 (d, J = 8.0 Hz, 1H), 8.00 (d, J = 8.0 Hz, 1H), 7.96 (d, J = 8.0 Hz, 1H), 7.87 (d, J = 8.0 Hz, 2H), 7.54 (m, 6H), 7.44 (m, 2H), 6.95 (d, J = 8.0 Hz, 1H), 6.79 (d, J = 16.0 Hz, 1H), 6.74 (d, J = 8.0 Hz, 2H), 3.92 (m, 1H), 3.52 (m, 2H), 3.24 (m, 1H), 3.12 (m, 2H), 2.98 (m, 1H), 2.18 (m, 1H), 1.75 (s, 6H). 13C NMR (100 MHz, CDCl3) δ 175.9, 174.2, 167.8, 153.2, 147.5, 146.7, 145.0, 131.7, 130.1, 129.4, 128.7, 126.1, 125.0, 124.2, 122.0, 120.6, 114.4, 111.5, 111.0, 110.7, 110.6, 109.9, 97.2, 96.3, 46.5, 46.2, 45.0, 41.0, 26.7. HRMS: 642.2590 ([M + H]+); calcd for C40H32N7O2: 642.2617.
Results and discussion
PBI-S was synthesized by the classical condensation of compound 1 with compound 2 in one step in good yield (Scheme 1).
The solvent and effect of water content on fluorescence spectra of the probe was investigated firstly (Fig. S2 and S3†). Considering its better peak shapes, we chose the solvent ratio (DMF/PBS = 3
:
7) as the vitro test solvent system.
The absorption band of PBI-S, attributing to hemicyanine and pyrido[1,2-a]benzimidazole moiety, centered at 556 nm and 334 nm. When sodium sulfite was added gradually to the solution of PBI-S (DMF/PBS = 3
:
7), the absorption peak at 334 nm increased while the peak at 556 nm decreased (Fig. 1). Concomitantly, the solution turned from red to colorless in color, which proves that PBI-S could act as a “naked-eye” probe for HSO3−. It was calculated that the ratio of the absorbance at 334 and 556 nm displayed an over 300-fold enhancement, which indicates that PBI-S is capable for detecting sulfite by UV-vis absorption.
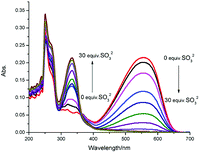 |
| Fig. 1 Absorption spectra of PBI-S (5 μM) upon addition of HSO3− (150 μM) in DMF–PBS buffer solution (3/7, v/v, pH = 7.20). | |
Next, the fluorescence titration was conducted. The free PBI-S displayed two obvious fluorescence bands at 465 nm and 640 nm, which attributed to pyrido[1,2-a]benzimidazole and hemicyanine unit, respectively. Upon addition of HSO3− incrementally, fluorescence emission band at 465 nm increased gradually while the fluorescence at 640 nm decreased (Fig. 2), which implies that the acceptor moiety was destroyed by the reaction of the probe with HSO3− and the FRET between the donor and the acceptor was switched off.
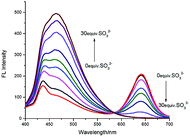 |
| Fig. 2 Fluorescence spectra of PBI-S (5 μM) upon addition of HSO3− (150 μM) in DMF–PBS buffer solution (3/7, v/v, pH = 7.20), (λex = 380 nm, slit = 10 nm/10 nm). | |
The ratio of emission intensities at 465 and 640 nm (I465/I640) changed from 0.52 in the absence of HSO3− to 123.75, a 238-fold variation in the ratios. Furthermore, the emission ratio (I465/I640) was found to increase linearly with the HSO3− concentration changed from 3 to 9 μM (Fig. S4†). The detection limit was determined to be 62 nM which is calculated by 3σ/k (“σ” is the standard deviation of 15 blank measurements, “k” is the slope of the fitting line).
Then the selectivity of PBI-S toward sulfite over other relevant anions was conducted and it was observed that only by the addition of sulfite could the absorption and fluorescence change. While with other anions, no change in the UV and FL spectra was noted (Fig. 3 and S5†). However, some spectral changes in GSH, Hcy and Cys were observed due to nucleophilic property of the SH group in them.
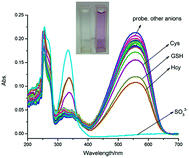 |
| Fig. 3 UV-vis spectral changes of probe PBI-S (5 μM) in the presence of various analytes (150 μM). | |
Furthermore, we studied the responses of PBI-S to the sulfite in the presence of the other competitive anions (including GSH, Hcy and Cys). It was observed that when the other anions were added subsequently, the fluorescence emission ratios (I465/I640) were not influenced (Fig. S6†).
As shown in Fig. S7,† the probe was stable and can function well over a wide range of pH (6.0–10.0). Time-dependent fluorescence response to SO32− was also carried out (Fig. S8†). The reaction could be completed within 2 min (50 eq. SO32−). Such a rapid response is appealing for real-time detection.
The supposed mechanism is shown in Scheme 2. For the probe alone, FRET between the pyrido[1,2-a]benzimidazole and hemicyanine moiety resulted in red emission from hemicyanine. Upon the addition of HSO3−, the energy transfer from pyrido[1,2-a]benzimidazole donor to hemicyanine acceptor was interrupted because of the breakage of C
C bond by the addition reaction with HSO3−. The MS spectra of the reaction product also supported the deduction (calcd for C40H34N7O5S: 723.2342, found 723.2334, Fig. S9 and S10†).
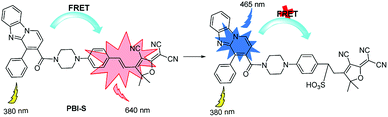 |
| Scheme 2 Proposed mechanism of probe. | |
Finally, PBI-S was applied to detect sulfite in sample analysis of commercially purchased granulated sugar, crystal sugar, and soft sugar. The sugar (5.0 g) was dissolved in deionized water and then was diluted to 10 mL. To the solution of the probe PBI-S (5 μM) in the DMF/PBS buffer (DMF/PBS = 3
:
7, pH 7.20, 10 mM), aliquots of the sugar solution were added. As shown in Table 1, the HSO3− concentration in sugar was successfully determined by the probe PBI-S with good recovery.
Table 1 Concentration of the sulfite in sugar samplesa
Sample |
HSO3− level ± SD (μmol L−1) |
Added (μmol L−1) |
Found ± SD (μmol L−1) |
Recovery ± SD (%) |
The results were presented as means ± SE with replicates n = 5. |
Crystal sugar |
0.63 ± 0.048 |
0.5 |
1.07 ± 0.031 |
94.69 ± 2.6% |
1 |
1.58 ± 0.036 |
96.93 ± 2.3% |
Granulated sugar |
1.21 ± 0.049 |
0.5 |
1.80 ± 0.026 |
105.26 ± 0.51% |
1 |
2.04 ± 0.030 |
92.31 ± 0.03% |
Soft sugar |
0.76 ± 0.045 |
0.5 |
1.28 ± 0.0140 |
101.16 ± 3.3% |
1 |
1.70 ± 0.0127 |
96.59 ± 3.5% |
It was calculated that the HSO3− concentration in the three sugar samples were 0.79, 1.52, and 0.96 mg kg−1, respectively. A traditional titration method was carried out to test the accuracy of this method and Table S1† showed that results of the two methods were equivalent.
Conclusions
In summary, we have developed a new fluorophore pyrido[1,2-a]benzimidazole based ratiometric fluorescent probe for selective detection of sulfite ion in water. It shows large (pseudo) Stokes shifts (260 nm), high FRET efficiency, high selectivity and sensitivity. A distinct color change from red to colorless was observed and importantly, it proves to be a convenient and efficient tool to detect the sulfite levels in sugar samples.
Conflicts of interest
There are no conflicts to declare.
Acknowledgements
This work was supported by the Science Fund of Shandong Province for Excellent Young Scholars (ZR2017JL015) and the Natural Science Foundation of China (21602153 and 11574009).
Notes and references
- J. L. Wang, Y. F. Hao, H. Wang, S. X. Yang, H. Y. Tian, B. G. Sun and Y. G. Liu, Rapidly responsive and highly selective fluorescent probe for bisulfite detection in food, J. Agric. Food Chem., 2017, 65, 2883–2887 CrossRef CAS PubMed.
- M. G. Choi, J. Y. Hwang, S. Y. Eor and S. K. Chang, Chromogenic and fluorogenic signaling of sulfite by selective deprotection of resorufin levulinate, Org. Lett., 2010, 24, 5624–5627 CrossRef PubMed.
- X. F. Gu, C. H. Liu, Y. C. Zhu and Y. Z. Zhu, A boron-dipyrromethene-based fluorescent probe for colorimetric and ratiometric detection of sulfite, J. Agric. Food Chem., 2011, 59, 11935–11939 CrossRef CAS PubMed.
- K. Y. Chen, Y. Guo, Z. H. Lu, B. Q. Yang and Z. Shi, Novel coumarin-based fluorescent probe for selective detection of bisulfite anion in water, Chin. J. Chem., 2010, 28, 55–60 CrossRef CAS.
- Y. M. Yang, Q. Zhao, W. Feng and F. Y. Li, Luminescent chemodosimeters for bioimaging, Chem. Rev., 2013, 113, 192–270 CrossRef CAS PubMed.
- V. S. Lin, W. Chen, M. Xian and C. J. Chang, Chemical probes for molecular imaging and detection of hydrogen sulfide and reactive sulfur species in biological systems, Chem. Soc. Rev., 2014, 44, 4596–4618 RSC.
- L. Yuan, W. Y. Lin, K. B. Zheng and S. S. Zhu, FRET-based small-molecule fluorescent probes: rational design and bioimaging applications, Acc. Chem. Res., 2013, 46, 1462–1473 CrossRef CAS PubMed.
- X. L. Zheng, R. X. Ji, X. Q. Cao and Y. Q. Ge, FRET-based ratiometric fluorescent probe for Cu2+ with a new indolizine fluorophore, Anal. Chim. Acta, 2017, 978, 48–54 CrossRef CAS PubMed.
- Y. Q. Ge, R. X. Ji, S. L. Shen and X. Q. Cao, A ratiometric fluorescent probe for sensing Cu2+ based on new imidazo[1,5-a]pyridine fluorescent dye, Sens. Actuators, B, 2017, 245, 875–881 CrossRef CAS.
- Y. Q. Ge, X. J. Xing, A. K. Liu, R. X. Ji, S. L. Shen and X. Q. Cao, A novel imidazo[1,5-a]pyridine-rhodamine FRET system as an efficient ratiometric fluorescent probe for Hg2+ in living cells, Dyes Pigm., 2017, 146, 136–142 CrossRef CAS.
- R. X. Ji, A. K. Liu, S. L. Shen, X. Q. Cao, F. Li and Y. Q. Ge, An indolizine–rhodamine based FRET fluorescence sensor for highly sensitive and selective detection of Hg2+ in living cells, RSC Adv., 2017, 7, 40829–40833 RSC.
- X. F. Yang, M. L. Zhao and G. Wang, A rhodamine-based fluorescent probe selective for bisulfite anion in aqueous ethanol media, Sens. Actuators, B, 2011, 152, 8–13 CrossRef CAS.
- Y. Q. Sun, P. Wang, J. Liu, J. Y. Zhang and W. Guo, A fluorescent turn-on probe for bisulfite based on hydrogen bond-inhibited C=N isomerization mechanism, Analyst, 2012, 137, 3430–3433 RSC.
- H. Y. Tian, J. H. Qian, Q. Sun, H. Y. Bai and W. B. Zhang, Colorimetric and ratiometric fluorescent detection of sulfite in water via cationic surfactant-promoted addition of sulfite to α,β-unsaturated ketone, Anal. Chim. Acta, 2013, 788, 165–170 CrossRef CAS PubMed.
- M. Y. Wu, K. Li, C. Y. Li, J. T. Hou and X. Q. Yu, A water-soluble near-infrared probe for colorimetric and ratiometric sensing of SO2 derivatives in living cells, Chem. Commun., 2014, 50, 183–185 RSC.
- Z. X. Liu, S. J. Guo, J. Y. Piao, X. Zhou and X. Wu, A reversible fluorescent probe for circulatory detection of sulfites through a redox-based tandem reaction, RSC Adv., 2014, 4, 54554–54557 RSC.
- W. Xu, C. L. Teoh, J. J. Peng, D. D. Su, L. Yuan and Y. T. Chang, A mitochondria-targeted ratiometric fluorescent probe to monitor endogenously generated sulfur dioxide derivatives in living cells, Biomaterials, 2015, 56, 1–9 CrossRef CAS PubMed.
- Y. Liu, K. Li, M. Y. Wu, Y. H. Liu, Y. M. Xie and X. Q. Yu, A mitochondria-targeted colorimetric and ratiometric fluorescent probe for biological SO2 derivatives in living cells, Chem. Commun., 2015, 51, 10236–10239 RSC.
- X. J. Liu, Q. W. Yang, W. Q. Chen, L. N. Mo, S. Chen, J. Kang and X. Z. Song, A ratiometric fluorescent probe for rapid, sensitive and selective detection of sulfur dioxide with large Stokes shifts by single wavelength excitation, Org. Biomol. Chem., 2015, 32, 8663–8668 RSC.
- B. Roubinet, L. Bailly, E. Petit, P. Y. Renard and A. Romieu, A FRET-based probe for fluorescence sensing of sulfide/sulfite analytes, using a novel long-wavelength water-soluble 7-hydroxycoumarin as reporter fluorophore, Tetrahedron Lett., 2015, 56, 1015–1019 CrossRef CAS.
- W. L. Wu, H. L. Ma, M. F. Huang, J. Y. Miao and B. X. Zhao, Mitochondria-targeted ratiometric fluorescent probe based on FRET for bisulfite, Sens. Actuators, B, 2017, 241, 239–244 CrossRef CAS.
- Z. Chen, F. Z. Chen, Y. C. Sun, H. Liu, H. P. He, X. H. Zhang and S. F. Wang, A novel ratiometric fluorescent probe for selective detection of bisulfite in living cells, RSC Adv., 2017, 7, 2573–2577 RSC.
- Y. Yao, Q. Sun, Z. Chena, R. Huang, W. Zhang and J. Qian, A mitochondria-targeted near infrared ratiometric fluorescent probe for the detection of sulfite in aqueous and in living cells, Talanta, 2018, 189, 429–436 CrossRef CAS PubMed.
- J. Xu, D. J. Zheng, M. M. Su, Y. C. Chen, Q. C. Jiao, Y. S. Yang and H. L. Zhu, A rapid cell-permeating turn-on probe for sensitive and selective detection of sulfite in living cells, Org. Biomol. Chem., 2018, 16, 6940–6946 CAS.
- K. Dou, G. Chen, F. Yu, Z. Sun, G. Li, X. Zhao, L. Chen and J. You, A two-photon ratiometric fluorescent probe for the synergistic detection of the mitochondrial SO2/HClO crosstalk in cells and in vivo, J. Mater. Chem. B, 2017, 5, 8389–8398 RSC.
- K. Dou, Q. Fu, G. Chen, F. Yu, Y. Liu, Z. Cao, G. Li, X. Zhao, L. Xia, L. Chen, H. Wang and J. You, A novel dual-ratiometric response fluorescent probe for SO2/ClO− detection in cells and in vivo and its application in exploring the dichotomous role of SO2 under the ClO− induced oxidative stress, Biomaterials, 2017, 133, 82–93 CrossRef CAS PubMed.
- Y. Q. Ge, X. L. Zheng, R. X. Ji, S. L. Shen and X. Q. Cao, A new pyrido[1,2-a]benzimidazole-rhodamine FRET system as an efficient ratiometric fluorescent probe for Cu2+ in living cells, Anal. Chim. Acta, 2017, 965, 103–110 CrossRef CAS PubMed.
- Y. Q. Ge, A. K. Liu, R. X. Ji, S. L. Shen and X. Q. Cao, Detection of Hg2+ by a FRET ratiometric fluorescent probe based on a novel pyrido[1,2-a]benzimidazole rhodamine system, Sens. Actuators, B, 2017, 251, 410–415 CrossRef CAS.
Footnotes |
† Electronic supplementary information (ESI) available: 1H NMR, 13C NMR and MS spectra of probe, and additional cell images. See DOI: 10.1039/c8ra08967a |
‡ Equal contribution. |
|
This journal is © The Royal Society of Chemistry 2019 |
Click here to see how this site uses Cookies. View our privacy policy here.