DOI:
10.1039/C9QO00621D
(Research Article)
Org. Chem. Front., 2019,
6, 2788-2791
Direct methylation and carbonylation of in situ generated arynes via HDDA-Wittig coupling†
Received
11th May 2019
, Accepted 13th June 2019
First published on 28th June 2019
Abstract
A highly efficient HDDA-Wittig coupling strategy for the synthesis of fully functionalized benzenes, such as ethyl 2-methylbenzoates and o-tolylethanones, is reported. The formation of four new C–C bonds via a one-pot, multicomponent cascade proceeded through the formation of a benzyne intermediate by self-cyclization, which then reacted with a phosphorus ylide. The target bicyclic aromatic compounds were prepared by the reaction of tetraynes with (acetylmethylene)triphenylphosphorane/(carbomethoxymethylene)triphenylphosphorane, and trace water allowed direct methylation and played a pivotal role in the construction of the natural carbonylated 2,3-dihydro-1H-indene cores, which were highly substituted. This report describes a robust method for the production of fused polyfunctional aromatic hydrocarbons.
Wittig reagents or phosphorus ylides (P-ylides) are suitable tools for C
C bond formation and are frequently used in organic synthesis.1 The aryne intermediate is highly reactive and offers good regioselectivity.2 This intermediate has unique advantages in the construction of cyclic compounds, especially certain complex PAH compounds.3 The hexadehydro-Diels–Alder (HDDA) reaction is widely used in the conversion of functional groups to tune molecular functions.4 Yang et al. used a magnesium-catalyzed hemiacetal and bench-stable P-ylide for a step-economic asymmetric reaction.5 Hoye used benzynes with BF3 as a Lewis acid in cascade reactions to promote carbene-like reactivity.6 Stuart et al. presented the C–H deprotonation activation to avoid the necessity for difunctionalized aryne precursors.7 Ohmori et al. established dual benzyne monomer coupling methods for the natural product synthesis of Actinorhodin.8 Zhu reported the heteroannulation of arynes as precursors in the natural total synthesis of (+)-hinckdentine A.9 However, it is difficult to introduce methyl and carbonyl groups into benzene rings. We tested a novel cyclization method involving a benzyne intermediate with a Wittig reagent to prepare rare, fully substituted benzene cores.10 The synthesis of an aryne precursor system that is both methylated and carbonylated that does not utilize a stepwise bimolecular nucleophilic substitution reaction (Scheme 1) is very challenging for organic chemists.11,12 Surprisingly, the benzannulation of triynes and acetyl-methylenetriphenylphosphorane/carbomethoxymethylenetriphenylphosphorane and trace water in toluene typically yields fused poly-functional aromatic hydrocarbon derivatives as the major products via waste-free, green transformations that also exhibit high atom economy.13 The fully substituted benzene derivatives were compared with general aromatic hydrocarbon derivatives, and the former, with multiple rings and sophisticated and diverse structures, were prepared by the present reaction method, high-lighting its great potential for chemical production and pharmaceutical synthesis.14
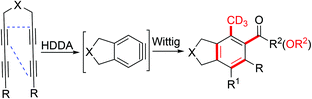 |
| Scheme 1 Target carbonylated 2,3-dihydro-1H-indene structures. | |
Results and discussion
Herein, we developed a mild, catalyst-free procedure for the synthesis of polyfunctional fused aromatics in good to excellent yields. This method was simple and met the requirements of atom economy for green chemistry. It was verified that H2O played a crucial role in this reaction. With this novel method, the simultaneously methylation and carbonylation of the aromatic ring was achieved. The polyfunctionalized fused aromatic derivatives were generated by cascade15 HDDA reactions of different P-ylide and tetrayne substrates. We proposed a possible mechanism for the reaction: the tetrayne substrates form benzyne intermediates by self-cyclization and then react with the P-ylides to yield the fused aromatics compounds via an HDDA reaction. This method, which does not require a directing group, exhibits precise regioselectivity and can generate fused methyl 4-methyl-2,3-dihydro-1H-indene-5-carboxylates and ethyl 4-methyl-2,3-dihydro-1H-indene-5-carboxylates via a one-pot, multiterminal cycloaddition reaction (1a–1q) with excellent yields (Table 1). Consequently, this reaction provides an economical, efficient, and direct method for the synthesis of highly substituted ethyl 2-methylbenzoate and o-tolylethanone compounds. A reaction scheme for accessing carbon-bridged tetraynes with ylides in a catalyst-free manner was designed. The optimum reaction conditions in terms of water addition, temperature, solvent, and reaction time are discussed. Initially, with dry toluene as the solvent, substrate 1a was reacted with (carbomethoxymethylene)triphenylphosphorane at 100 °C. TLC was performed to monitor the reaction, and the starting material disappeared after 9 h. After separation and purification of the products, the reaction with a toluene
:
water ratio of 100
:
1 using (carbomethoxymethylene)triphenylphosphorane for 9 h at 100 °C yielded 10% of the desired product, and when the temperature was 90 °C (with a reaction time of 9 h), the yield was 80%. Because TLC did not fully reflect raw materials, the actual yield was 72%. Then, the temperature was increased to 110 °C and 120 °C with the same 9 h reaction time. TLC-based monitoring showed that the raw materials had been consumed, and the reaction had reached completion. We investigated the reactions in acetonitrile, toluene and cyclohexane and found that toluene was the most effective. The optimum reaction conditions for the tetrayne substrate (1 equiv.) with (carbomethoxymethylene)triphenylphosphorane (1.05 equiv.) and water (2 equiv.) were as follows: toluene 1.5 mL, 100 °C, 9 h.
Table 1 Preparation of ethyl 2-methylbenzoatesa,b
Reaction scale: tetraynes 1 (1.0 equiv.), (carbomethoxymethyl ene)triphenylphosphorane 2 (1.05 equiv.), water (2.0 equiv.), toluene 1.5 mL, 100 °C.
Isolated yield.
|
|
First, the effect of the structure of the tetrayne substrate on the yield was investigated. As shown in Table 1, a series of products (3a–3p) were obtained from the reactions of tetraynes with (carbomethoxymethylene)triphenylphosphorane or ethyl 2-(triphenylphosphoranylidene)acetate, and the yields ranged from 75% to 85%. The effect of different tetrayne substrate on the product yield was examined. When OiPr, OEt and OMe were connected to the carbonyl carbon in the alkyne substrates, the yields were almost the same (3a (80%) and 3d (80%), 3i (79%), 3n (77%) and 3p (77%)). While the yields of 3c and 3h were 78% and 75%, respectively. Compounds containing benzene rings with fluorine, chlorine or other electron-withdrawing substituents exhibited lower yields than those with unsubstituted rings, for example, the yield of chloride-containing substrate 3c was 78%, while that of the molecule with an unsubstituted benzene ring was 80% (3a); the yield of the substrate with a benzene ring bearing a fluoride (3o) was 73%, while the molecule with an unsubstituted benzene ring has offered a yield of 77% (3n).
As shown in Table 2, yields of 76–87% were obtained from the reactions of tetraynes with (acetylmethylene)triphenylphosphorane. When OiPr, OEt and OMe were connected to carbonyl carbon of the tetrayne substrates, the yields were similar, such as those of 3q (80%), 3x (81%), 3r (82%) and 3v (81%). The yields with benzene ring-containing tetraynes with alkyl groups as electron donors were slightly higher than those of tetraynes directly bearing alkyl groups as electron donors. For example, the yields of 3r and 3s were 82% and 85%, respectively (Table 2), while the yields of 3t and 3u were 80% and 79%, respectively. The yields of benzene rings with alkyl substituents were higher than those of benzene rings without substituents. For example, the yield of 3s, with an n-propyl group on the benzene ring, was 87%, and the yield of 3r, with a methyl group on the benzene ring, was 82%, while the yield of the unsubstituted benzene ring was 80% (3a). The structures of 3h and 3y were confirmed by X-ray diffraction.16
Table 2 Preparation of fused 1-(o-tolyl)ethanonesa,b
Reaction scale: tetraynes 1 (1.0 equiv.), (acetylmethylene) triphenylphosphorane 2 (1.05 equiv.), water (2.0 equiv.), toluene 1.5 mL, 100 °C.
Isolated yield.
|
|
Scheme 2 shows a possible mechanism of the reaction. Tetrayne substrate 1 formed a benzyne intermediate by self-cyclization and then reacted with a Wittig reagent to yield fused aromatic compounds Bvia nucleophilic addition reaction. The tetrayne substrate first undergoes an HDDA reaction to form benzyne intermediate A, and then, the carbanion in the phosphorus ylide attacks benzyne intermediate A to form four-membered ring intermediate B.17 Triphenyl oxyphosphorous C is produced after the self-cyclization performance of intermediate B, affording the intermediate Dvia a 4π-electrocyclic ring opening process.18 With the elimination of phosphine oxide,19E, which is the different resonance structure of D then transformed to product 3f. Deuterated benzene was initially used as the solvent to explore the source of the methyl hydrogen in the product. 1H NMR spectroscopy confirmed that deuterated benzene was not the source of the hydrogen in the product, demonstrating the importance of D2O in this process.
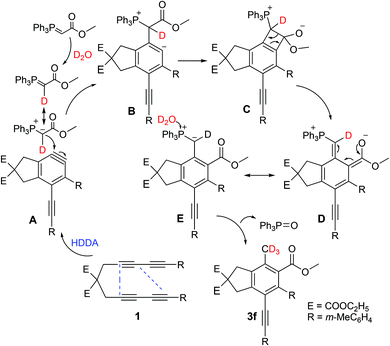 |
| Scheme 2 Tentative mechanism of the HDDA-Wittig coupling 3f. | |
Conclusions
In summary, we describe the first method for the synthesis of fully substituted benzenes, such as ethyl 2-methylbenzoates and o-tolylethanones, via the reaction of acetylmethylenetriphenylphosphorane/carbomethoxymethylenetriphenylphosphorane and trace water with polyynes. The formation of four new C–C bonds via a one-pot, multicomponent cascade led to the formation of benzyne intermediates by self-cyclization, and these intermediates then reacted with phosphorus ylides. The reactions produced highly substituted targets with excellent regioselectivity. Rare, fully substituted benzene derivatives were obtained in high yields under aerobic conditions. Future studies will be focused on the construction of directly methylated and carbonylated aryne precursors and on the development of a highly efficient pathway for the production of the ubiquitous carbonylated 2,3-dihydro-1H-indene and polyfunctional aromatic hydrocarbons.
Conflicts of interest
There are no conflicts to declare.
Acknowledgements
The authors thank the National Natural Science Foundation of China (21572002, 21272005), the Research Culture Funds of Anhui Normal University (2019XJJ020), and Department of Human Resources of Anhui Province for financial support.
Notes and references
- For reviews on this topic, see:
(a) H. Guo, Y. Fan, Z. Sun, Y. Wu and O. Kwon, Chem. Rev., 2018, 118, 10049–10293 CrossRef CAS PubMed;
(b)
R. Sanz and A. Suarez, Arene Chemistry, Wiley, Hoboken, 2015, pp. 299–336 Search PubMed;
(c)
V. Iaroshenko, Phosphorus Ylides and Related Compounds, Wiley-VCH Verlag GmbH & Co. KGaA, 2019 Search PubMed;
(d) S. Han, P. Chakrasali, J. Park, H. Oh, S. Kim, K. Kim, A. Pandey, S. H. Han, S. B. Han and I. S. Kim, Angew. Chem., Int. Ed., 2018, 57, 12737–12740 CrossRef CAS PubMed.
- For reviews on this topic, see:
(a) Y. M. Li, C. Mück-Lichtenfeld and A. Studer, Angew. Chem., Int. Ed., 2016, 55, 14435–14438 CrossRef CAS PubMed;
(b) J. A. Garcia-Lopez, M. Cetin and M. F. Greaney, Angew. Chem., Int. Ed., 2015, 54, 2156–2159 CrossRef CAS PubMed;
(c) R. Karmakar, A. Le, P. Xie, Y. Xia and D. Lee, Org. Lett., 2018, 20, 4168–4172 CrossRef CAS PubMed;
(d) B. Michel and M. F. Greaney, Org. Lett., 2014, 16, 2684–2684 CrossRef CAS PubMed;
(e) M. H. Feng, B. Q. Tang, N. Z. Wang, H. X. Xu and X. F. Jiang, Angew. Chem., Int. Ed., 2015, 54, 14960–14964 CrossRef CAS PubMed;
(f) D. Zhao, J. J. Zhang and Z. W. Xie, Angew. Chem., Int. Ed., 2014, 53, 8488–8491 CrossRef CAS PubMed;
(g) M. Pawliczek, L. K. B. Garvea and D. B. Werz, Chem. Commun., 2015, 51, 9165–9168 RSC;
(h) T. Roya and A. T. Biju, Chem. Commun., 2018, 54, 2580–2594 RSC.
-
(a) R. Gleiter, G. Haberhauer, D. B. Werz, F. Rominger and C. Bleiholder, Chem. Rev., 2018, 118, 2010–2041 CrossRef CAS PubMed;
(b) M. Murai, T. Ogita and K. Takai, Chem. Commun., 2019, 55, 2332–2335 RSC;
(c) A. Narita, X. Y. Wang, X. L. Feng and K. Müllen, Chem. Soc. Rev., 2015, 44, 6616–6643 RSC;
(d) H. Takikawa, A. Nishii, T. Sakaib and K. Suzuki, Chem. Soc. Rev., 2018, 47, 8030–8056 RSC;
(e) R. Karmakara and D. Lee, Chem. Soc. Rev., 2016, 45, 4459–4470 RSC;
(f) J. R. Shi, Y. Y. Li and Y. Li, Chem. Soc. Rev., 2017, 46, 1707–1719 RSC.
-
(a) C. Holden and M. F. Greaney, Angew. Chem., Int. Ed., 2014, 53, 5746–5749 CrossRef CAS PubMed;
(b) Y. M. Hu, Y. D. Hu, Q. Hu, J. Ma, S. Lv, B. H. Liu and S. Wang, Chem. – Eur. J., 2017, 23, 4065–4072 CrossRef CAS PubMed;
(c) W. B. Li, L. J. Zhou and J. L. Zhang, Chem. – Eur. J., 2016, 22, 1558–1571 CrossRef CAS PubMed;
(d) J. T. Zhang, A. C. S. Page, V. Palani, J. H. Chen and T. R. Hoye, Org. Lett., 2018, 20, 5550–5553 CrossRef CAS PubMed.
-
(a) L. Q. Wang, D. X. Yang, D. Li, X. H. Liu, P. X. Wang, K. Z. Wang, H. Y. Zhu, L. T. Bai and R. Wang, Angew. Chem., Int. Ed., 2018, 57, 9088–9092 CrossRef CAS PubMed;
(b) M. Petzold, P. G. Jones and D. B. Werz, Angew. Chem., Int. Ed., 2019, 58, 6225–6229 CrossRef CAS PubMed.
- H. Shen, X. Xiao, M. Haj, P. Willoughby and T. R. Hoye, J. Am. Chem. Soc., 2018, 140, 15616–15620 CrossRef CAS PubMed.
- S. K. Sundalam, A. Nilova, T. L. Seidl and D. R. Stuart, Angew. Chem., Int. Ed., 2016, 55, 8431–8434 CrossRef CAS PubMed.
- M. Ninomiya, Y. Ando, F. Kudo, K. Ohmori and K. Suzuki, Angew. Chem., Int. Ed., 2019, 58, 4264–4270 CrossRef CAS PubMed.
- R. Torres-Ochoa, T. Buyck, Q. Wang and J.-P. Zhu, Angew. Chem., Int. Ed., 2018, 57, 5679–5683 CrossRef CAS PubMed.
-
(a) Y. M. Hu, J. Ma, L. D. Li, Q. Hu, S. Lv, B. H. Liu and S. Wang, Chem. Commun., 2017, 53, 1542–1545 RSC;
(b) X. Meng, S. Lv, D. Cheng, Q. Hu, J. Ma, B. Liu and Y. Hu, Chem. – Eur. J., 2017, 23, 6264–6271 CrossRef CAS PubMed.
- S. P. Ross and T. R. Hoye, Nat. Chem., 2017, 9, 523–530 CrossRef CAS PubMed.
- Y. L. Liu, Y. Liang, S. F. Pi and J. H. Li, J. Org. Chem., 2009, 74, 5691–5694 CrossRef CAS PubMed.
-
(a) S. S. Bhojgude, A. Bhunia and A. T. Biju, Acc. Chem. Res., 2016, 49, 1658–1670 CrossRef CAS PubMed;
(b) I. Triandafillidi and C. G. Kokotos, Org. Lett., 2017, 10, 107–109 Search PubMed;
(c) N. Hall, Science, 1994, 266, 32–34 CrossRef CAS PubMed.
-
(a) S. Yang, D. F. Lu, H. R. Huo, F. Luo and Y. F. Gong, Org. Lett., 2018, 20, 6943–6947 CrossRef CAS PubMed;
(b) S. Dubbu and Y. D. Vankar, Eur. J. Org. Chem., 2018, 5060–5064 CrossRef CAS.
-
(a) T. S. Maskrey, M. C. Frischling, M. L. Rice and P. Wipf, Front. Chem., 2018, 24, 201800376 Search PubMed;
(b) T. Schitter, N. J. Roy, P. G. Jones and D. B. Werz, Org. Lett., 2019, 21, 640–643 CrossRef CAS PubMed.
- CCDC 1903151 (3h) and 1888961 (3y) contain the supplementary crystallographic data for this paper.†.
- Y. Uchiyama, N. Kano and T. Kawashima, J. Org. Chem., 2006, 71, 659–670 CrossRef CAS PubMed.
-
(a) Z. Chen, Y. Nieves-Quinones, J. R. Waas and D. A. Singleton, J. Am. Chem. Soc., 2014, 136, 13122–13125 CrossRef CAS PubMed;
(b) J. C. Deng, F. W. Chan, C. W. Kuo, C. A. Cheng, C. Y. Huang and S. C. Chuang, Eur. J. Org. Chem., 2012, 5738–5747 CrossRef CAS.
-
(a) M. Mehta, I. G. Arada, M. Perez, D. Porwal, M. Oestreich and D. W. Stephan, Organometallics, 2016, 35, 1030–1035 CrossRef CAS;
(b) H. Zhu, Z. W. Qu and S. Grimme, Chem. – Eur. J., 2019, 25, 4670–4672 CrossRef CAS PubMed.
Footnotes |
† Electronic supplementary information (ESI) available: Preparation of substrates, characterization data, 1H and 13C NMR, MS and IR spectra. CCDC 1888961 and 1903151. For ESI and crystallographic data in CIF or other electronic format see DOI: 10.1039/c9qo00621d |
‡ These authors contributed equally to this work. |
|
This journal is © the Partner Organisations 2019 |
Click here to see how this site uses Cookies. View our privacy policy here.