Lipid vesicle-loaded meso-substituted chlorins of high in vitro antimicrobial photodynamic activity†
Received
19th June 2018
, Accepted 25th October 2018
First published on 31st October 2018
Abstract
Photodynamic inactivation potential against bacteria of four chlorin derivatives with phenyl or fluorophenyl substituents was evaluated. The quantum yield values of singlet oxygen formation were in the range of 0.16–0.86. Compounds were characterized by high quantum yields of fluorescence (0.15–0.44) and moderate photostability in DMF solutions. Irradiation of chlorins in DMSO resulted in their phototransformation and then photodecomposition. Photodynamic inactivation of bacteria was performed after the compounds had been loaded into lipid vesicles. The following log reductions of growth values were obtained: Enterococcus faecalis >5.44; Staphylococcus aureus 2.74–5.34; Escherichia coli 0.01–2.14. No activity of meso-substituted chlorins was noticed against Pseudomonas aeruginosa and fungi Candida albicans and Trichophyton mentagrophytes.
Introduction
Chlorins constitute a large family of dye compounds, and they reveal absorption bands within the entire UV-vis range; this property makes them fascinating materials for applications in medicine and industry. Chlorins are known to play an essential role in nature as well. Many vital natural dyes such as chlorophylls and bacteriochlorins belong to this group, and they are critical agents in photosynthesis. Because of light absorption in the “therapeutic window,” they are good candidates as photosensitizers for photodynamic therapy (PDT).1 Photodynamic therapy is a method of cancer treatment, but it is effective against many other diseases including bacterial infections. PDT is based on the interactive application of three agents: molecular oxygen, a photosensitizer, and light. There are some limitations of PDT treatment resulting from its fundamental principles. Light is necessary for photosensitizer activation. Therefore, it should be delivered effectively to the target. Relatively high penetration of tissues by red light has been proved. Therefore, chlorins, which reveal high absorption in the red region, are promising photosensitizers.2–4
PDT focused on combating microbial infections is called photodynamic antimicrobial chemotherapy (PACT). PACT is an excellent treatment modality for superficially localized infections.5 Singlet oxygen formed during the photodynamic process is responsible for therapeutic effect. It reacts with bacterial membrane components and internal structures, disrupting their functions. Therefore, bacteria can be combated in a multi-targeted way. Taking into account the above facts, it can be concluded that the formation of resistance against PACT by bacteria seems difficult.6–8 Another crucial point of PACT protocol is delivery of the photosensitizer to the targeted area. Photosensitizers based on the porphyrinoid group mostly reveal hydrophobic character. Thus, many research groups have developed different strategies for photosensitizer delivery. An interesting approach has been presented in the review article published by Kryjewski and co-workers. The authors have shown usability of cyclodextrins as nanocarriers for photodynamic therapy.9 Another possibility is liposome-based carriers, which seem to be the best carriers for photosensitizers.10 Applications of liposome carriers have been presented by Jeong and co-workers, who studied liposomes loaded with erythromycin and pullulanpheophorbide as a photosensitizer against Propionibacterium acnes. The obtained results indicate high potential of this approach for the treatment of skin infections.11 Lipid vesicles are convenient for modifications. Additions of penetration enhancers such as ethanol and terpenes make them more elastic and allow formation of a new subclass called invasomes. Skin penetration studies of different liposomes and invasomes have been conducted by Dragicevic-Curic and co-workers. These authors concluded that liposomes modified with a small amount of ethanol as well as invasomes can be used as a highly efficient delivery system.12 Invasomes have also been used by Ossmann and co-workers, who incorporated chlorin derivatives into them and tested the obtained species against Enterococcus faecalis. E. faecalis is the primary cause of re-endodontic treatment in dentistry. Ossmann and co-workers proved that the studied chlorin, i.e., temoporfin is effective in bacteria eradication from dental roots.13
In the current paper, we present studies on photostability, singlet oxygen formation, and bacteria inactivation potential of a series of chlorins. Our studies were performed for four pyrrolidine-fused chlorins having phenyl and fluorophenyl substituents in meso positions and a nitro group at the β position (Fig. 1). The studied chlorin derivatives were synthesized following the multistep procedure previously described by Sniechowska and co-workers.14
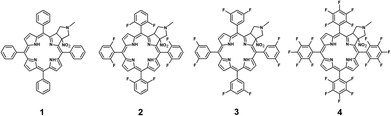 |
| Fig. 1 Chemical structures of chlorins 1–4. | |
Materials and methods
General
Chlorins were synthesized in a multistep procedure. Chemical structures of obtained compounds were confirmed by NMR and MS techniques.14 Chemical purity was assessed by the HPLC method (ESI†).
Spectral properties
Absorption spectra were recorded using a Shimadzu UV-160A spectrophotometer. Emission spectra were recorded with a Jasco 6200 spectrofluorometer in DMF and DMSO solutions. Fluorescence quantum yields were determined according to the methods described previously.15–19
Photostability studies
Photostability studies were performed at ambient temperature in DMF and DMSO according to methods previously described.16,20,21 A high-pressure xenon lamp (150 W, Optel) was used as a source of visible irradiation (21.6 mW m−2 at 640 nm). Samples were monitored between irradiation intervals with an OceanOptics system consisting of a Flame spectrometer and a source DT-MINI-2-GS. OceanOptics system was coupled with OceanView software. Mass spectra before and after irradiation were recorded with CombiFlash EZ Prep PurIon S (Teledyne-ISCO).
Singlet oxygen formation studies
Singlet oxygen formation quantum yields were determined in solution (DMF, DMSO) at ambient temperature. Studies were performed with a comparative method using a chemical quencher of singlet oxygen (rubrene, Aldrich) and a reference compound (zinc phthalocyanine, Aldrich). The mixture of rubrene and photosensitizer was irradiated with monochromatic light at the wavelength fixed to the maximum absorbance in Qy band region of chlorin spectrum. A high-pressure xenon lamp (150 W, Optel) was used as the irradiation source. A monochromator (M250/1200/U with 2 nm mm−1 (Dk = 4 nm) dispersion, Optel) was used to select an appropriate wavelength (corresponding to a Q band at maximum absorbance) and to avoid direct photodegradation of rubrene under UV light irradiation. The light intensity was measured by RD 0.2/2 with TD probe (Optel) radiometer and adjusted to 1 mW cm−2. Samples were monitored after irradiation intervals with an OceanOptics system consisting of a Flame spectrometer and a source DT-MINI-2-GS. OceanOptics system was coupled with OceanView software. Finally, the obtained kinetic data were compared with reference ones, and quantum yield was calculated.16,17,20,22–24
Lipid vesicle preparation
Lipid vesicles were prepared according to the method described by Dragicevic-Curic and co-workers.12 They employed phospholipids supplied by Avanti Polar Lipids Inc. (Alabaster, AL, USA): POPC (1-palmitoyl-2-oleoyl-sn-glycero-3-phosphocholine) and DOTAP (N-[1-(2,3-dioleoyloxy)propyl]-N,N,N-trimethylammonium chloride, 25 mg mL−1). POPC and DOTAP dissolved in chloroform (Aldrich) were mixed in a molar ratio of 8
:
2. Then, chlorins chloroform solution at the concentration 1 mg mL−1 was added in a proper amount to achieve final concentrations in the vesicles of 100, 10 and 1 μM. The obtained samples were evaporated under reduced pressure. To the obtained lipid-chlorin film, ethanol (3.3% w/v) was added and the mixture was vortexed for 5 min. Saline buffer was added and vortexed for another 5 min. The formed vesicles were stored in a fridge at 2–8 °C protected from light. Size of obtained vesicles was measured with Zetasizer Nano ZS (Malvern).
In vitro photodynamic activity against bacteria and fungi
Bacterial cultures.
Gram-positive bacteria Enterococcus faecalis (ATCC 29212) and Staphylococcus aureus (ATCC 25923), Gram-negative bacteria Pseudomonas aeruginosa (ATCC 6749) and Escherichia coli (ATCC 25922) and fungi Trichophyton mentagrophytes (ATCC 9533) and Candida albicans (ATCC 10231) were purchased from American Type Culture Collection. Bacterial strains were cultured aerobically in BHI broth (bioMerieux, France) at 36 °C ± 1 °C for 18–20 h, C. albicans in Sabouraud dextrose broth (Oxoid, UK) at 35 °C ± 1 °C for 24 h and T. mentagrophytes in Sabouraud dextrose agar (Oxoid, UK) at 35 °C ± 1 °C until adequate sporulation (ca. three weeks). Then, microorganisms were harvested by centrifugation (3000 rpm for 15 min), re-suspended in 10 mM phosphate buffered saline (PBS, pH = 7.0) and diluted in PBS to final concentrations of ca. 107 CFU mL−1 for bacteria, 106 CFU mL−1 for C. albicans and 105 CFU mL−1 for T. mentagrophytes.
Dark activity.
Aliquots of a standardized microbial suspension were placed in the wells of a microtitre plate; then, solutions containing different concentrations of a studied compound incorporated in the lipid formulation were added to each well except controls and were incubated for 20 min (incubation time). Simultaneously, a control experiment was conducted to assess the antimicrobial activity of the empty lipid vesicle (without photosensitizer). Also, the increase in the number of bacteria without added liposomes and photosensitizer was evaluated. Microbial suspensions from each well, after dilution, were grown on tryptic soy agar (TSA) plates. After an incubation period (20 h for bacteria and C. albicans; 3–5 days for T. mentagrophytes at 36 °C ± 1 °C), the viability of microbes was calculated by counting the number of colony forming units (CFUs). The log reduction of living bacteria in each sample was determined. Experiments were performed in triplicate.
Light-dependent activity.
Light activity evaluation was performed in a similar manner to the dark one, with irradiation of the culture after an incubation time of 20 min. Bacteria were irradiated at room temperature with high power LED multichip emitters (60 high-efficiency AlGaAs diode chips, Roithner LaserTechnik GmbH, Vienna, Austria) with maximum wavelength 640 nm, corresponding to the Q band of the chlorin at a fixed total light dose 30 J cm−2. The control experiments were performed analogous to dark conditions including empty vesicles and assessment of bacteria growth. Experiments were performed in triplicate.
Statistical analysis.
Statistical analyses were performed using the STATISTICA software v.13.0. Experiments were performed in triplicate. The quantitative variables were characterized by the arithmetic mean of standard deviation.
Microtox® analysis.
Experiments were performed according to 81.9% Microtox® test protocol with Microtox Model 500 analyzer and MicrotoxOmni software (Modern Water, Inc.).25,26
Results and discussion
Spectral properties
The recorded spectra of studied compounds reveal typical profiles for chlorins with high absorption in the Soret region27,28 (Fig. 2). The Soret band (By) consists of a single band at ca. 410 nm dependent on compound and solvent (Fig. 2, ESI†). Similarly, the Q band is divided into three bands at ca. 510 nm (Qx), ca. 535 nm (Qx) and ca. 640 nm (Qy). Hirohara and co-workers have presented properties of compound VI29 (first synthesized by Silva and co-workers,30Fig. 3) analogous to that of compound 4 studied here. Chlorin 4 in comparison to VI has a nitro group attached to the macro ring (Fig. 1 and 3). This difference in chemical structures causes a significant hypsochromic shift of the Qy band from 650 nm (VI) to 640 nm (4) in the absorption spectra recorded in DMSO. Thus, it can be concluded that the electron-withdrawing group linked directly to the chlorin core provides a hypsochromic shift in comparison to the compound with no electron-withdrawing group attached. Within the studied group of compounds, only slight differences are noticed in their absorption spectra (Fig. 2). These observations are in agreement with the results of Pandey and co-workers published previously.31 The electron-withdrawing character of fluorine atoms causes decrease in fluorescence quantum yields from 0.19 (1) to 0.15 (4) in DMF and from 0.44 (1) to 0.22 (4) in DMSO (Table 1). In comparison with porphyrin, the chlorin core causes slightly increased quantum yield, as reported by Pineiro and co-workers. These authors concluded that fluorochlorin (II, Fig. 3, ΦFL = 0.124) in comparison to fluoroporphyrin (I, Fig. 3, ΦFL = 0.069) reveals ca. 2-fold higher quantum yield of fluorescence in toluene solutions. Moreover, the introduction of a chlorine atom into molecule instead of fluorine causes decrease in the fluorescence ability (II, Fig. 3, ΦFL = 0.124; IV, Fig. 3, ΦFL = 0.049). Interestingly, increasing the amount of chlorine atoms in the molecule causes a decrease in the quantum yield (III, Fig. 3, ΦFL = 0.089; IV, Fig. 3, ΦFL = 0.049).32 In summary, in the case of chlorine photosensitizers, the reduction of fluorescence capacity is observed after the introduction of withdrawing substituents into the molecule. When the electron-withdrawing potential of the substituent is higher, the fluorescence quantum yield of the substituted molecule increases in comparison to that of the molecule bearing low electron-withdrawing groups. This dependency is observed in the series of chlorins II, III and IV. Moreover, it should be noted that fluorescence spectra of fluorinated chlorins do not change in comparison to those of non-fluorinated compounds (Fig. 2). Pandey and co-workers observed the same phenomenon in their study.31 Moreover, when chlorins 4 and VI studied here were analysed by Mesquita and co-workers, they obtained similar values of fluorescence quantum yields, i.e., 0.15 and 0.16 in DMF, respectively.33 Thus, it can be supposed that the introduction of the nitro group to the macrocyclic ring has negligible influence on fluorescence ability.
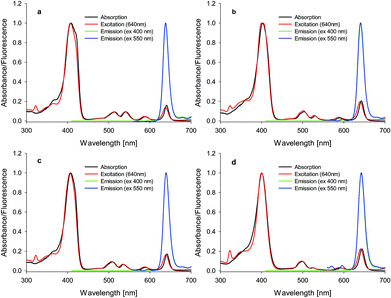 |
| Fig. 2 Absorption, emission, and excitation spectra of chlorins (a) 1, (b) 2, (c) 3, and (d) 4 in DMF. | |
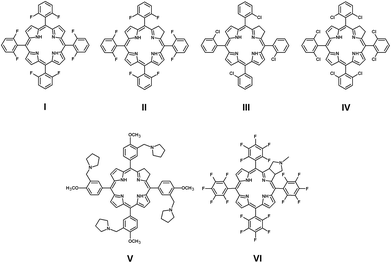 |
| Fig. 3 Chemical structures of compounds I–VI. | |
Table 1 Values of quantum yields, singlet oxygen, photodecomposition, fluorescence, lipid vesicle size, size distribution and polydispersity index (PDI)
Compound |
Φ
P
|
Φ
FL
|
Φ
Δ
|
DMF [106] |
DMSO [105] |
DMF |
DMSO |
DMF |
DMSO |
1
|
5.42 |
2.52 |
0.19 |
0.44 |
0.46 |
0.16 |
2
|
1.69 |
12.20 |
0.21 |
0.35 |
0.63 |
0.41 |
3
|
1.28 |
12.30 |
0.17 |
0.27 |
0.59 |
0.32 |
4
|
2.09 |
13.70 |
0.15 |
0.22 |
0.86 |
0.54 |
Lipid vesicle size |
Size distribution [nm] |
Compound |
Mean diameter ± SD [nm] |
PDI index |
Dv90 |
Dv50 |
Dv10 |
Error ≤0.02.
|
1
|
197 ± 1 |
0.29 |
419 |
209 |
110 |
2
|
209 ± 1 |
0.27 |
454 |
224 |
118 |
3
|
170 ± 1 |
0.22 |
316 |
179 |
106 |
4
|
260 ± 9 |
0.33 |
679 |
266 |
145 |
Photostability studies
As previously reported in our studies, photodecomposition processes during irradiation of phthalocyanines and porphyrazines were recognized as photobleaching. Decomposition processes were assigned as fragmentation ones. Photodegradation occurs rapidly in DMF solutions in comparison to that in DMSO ones.16,34 Interestingly, irradiation of studied chlorins demonstrated a different pathway of photodecomposition in contrast to phthalocyanines and porphyrazines. The studied chlorins dissolved in DMF revealed moderate photostability (Table 1, Fig. 4). The same observation was reported by Pedrosa and co-workers, who noticed that irradiation of chlorin derivative solutions (DMF/water) did not cause significant decomposition of compounds.35 It should be noted that irradiated DMSO solutions of 1–4 revealed an interesting phenomenon, which was assigned as a phototransformation process (Fig. 4). The presence of DMSO as a solvent initiated the phototransformation process. Photodecomposition of chlorin derivatives has been studied by only few groups so far. Especially, photodecomposition of temoporfin (Foscan®), a common photosensitizer in clinical use, has been investigated. After the irradiation period, Jones and co-workers as well as Bonnett and co-workers detected a macrocycle derivative and low-weight molecules simultaneously. On the basis of mass spectroscopy results, they proposed a modified chlorin derivative or porphyrin depending on irradiation conditions.36,37 Moreover, Hadjur and co-workers proved that the key role for photodecomposition process is played by singlet oxygen formed by the parent compound.38 Similar to Foscan®, the studied chlorins 1–4 might undergo phototransformation process, further resulting in modified macrocycle formation. This possibility was suggested after the analysis of mass spectra recorded before and after irradiation (Fig. 5). Moreover, color changes were observed in DMSO solutions after light exposure (Fig. 6). The mass spectra after irradiation showed new signals at slightly lower m/z values than those observed for the parent compound. Zhang and co-workers described the phototransformation of magnesium(II) meso-tetraphenylporphyrin, which upon dissolution in dichloromethane was irradiated in the absence and presence of molecular oxygen. It was noticed that irradiation of the porphyrin in the presence of molecular oxygen resulted in a new absorption band in the UV-vis spectrum and change in the colour of the sample. Also, in the MS spectra of the irradiated mixture, the authors noticed new signals corresponding with higher masses than those of the parent compound.39 In the IR analysis, signals assigned to N–O bond formation appeared at 1272 and 762 cm−1. Thus, Zhang and co-workers suggested the formation of porphyrin–oxygen adduct in 1
:
1 molar ratio.39
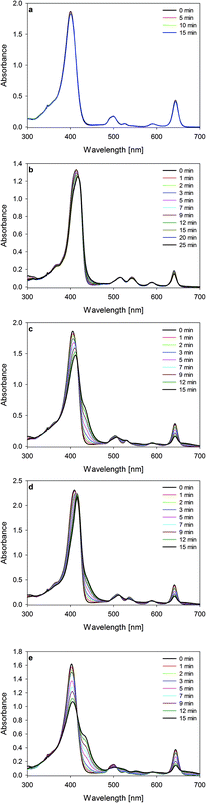 |
| Fig. 4 Spectra of studied chlorins during irradiation with visible light: (a) 4 in DMF, (b) 1 in DMSO, (c) 2 in DMSO, (d) 3 in DMSO, and (e) 4 in DMSO. | |
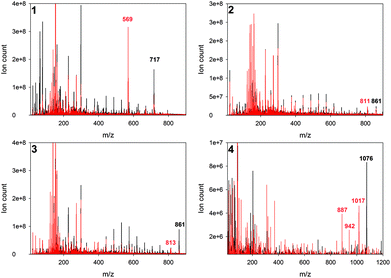 |
| Fig. 5 Mass spectra before (black) and after irradiation (red) of studied chlorins. | |
 |
| Fig. 6 Color change after irradiation in comparison to parent sample (before,’ after) in DMSO. | |
Singlet oxygen formation studies
As mentioned above, singlet oxygen plays an essential role in photodynamic therapy and should thus be generated in good yield by a potential photosensitizer. For clinical use, porphyrins were introduced over 40 years ago. Because of their low absorption in the red region of the light spectrum, chlorin-based photosensitizers were developed; they are compounds with higher absorption in the desirable range.3,40,41 Pineiro and co-workers performed singlet oxygen formation studies of porphyrins and related chlorins. Summarizing their study, it can be concluded that halogenated porphyrins (excited in the blue region) reveal similar singlet oxygen quantum yield as chlorins excited in the red region.32 Studied compounds 1–4 revealed relatively high quantum yields of singlet oxygen formation. Fig. 7 shows typical changes in the absorption spectrum of compound 2 while measuring the formation of singlet oxygen using rubrene as a singlet oxygen scavenger. It can be seen that by increasing the number of fluorine atoms, the increase in quantum yield was 1 < 2 ≈ 3 < 4 (Table 1). This tendency may be related to the electron-withdrawing properties of fluorine atoms. Similar regularities were reported by Tang and co-workers for porphyrins.42 Compounds 2 and 3 possess similar abilities for singlet oxygen formation. Therefore, it can be concluded that the positions of fluorine atoms in the phenyl substituents do not influence the singlet oxygen formation process. Chlorin 1 has no fluorine atoms in its structure and in comparison with twenty-fluorine-atom chlorin 4, it reveals approx. 2-fold lower quantum yield of singlet oxygen generation. Non-fluorinated chlorin 1 in comparison with photosensitizers registered for clinical use, i.e., Foscan® (ΦΔ = 0.68 in DMF)43 and chlorin e6 (ΦΔ = 0.63 in DMF)43 reveals lower singlet oxygen formation quantum yield. On the other hand, chlorins 2 and 3 form singlet oxygen with similar yields, and 4 is more efficient. Based on the research results previously published by Piskorz and co-workers, it can be concluded that when the fluorine atom is localized outside the macrocyclic ring (i.e., it is a part of the aliphatic chain), it does not increase singlet oxygen quantum yield.44
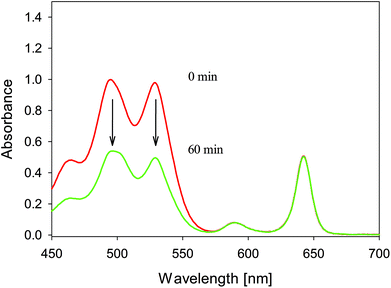 |
| Fig. 7 UV-vis spectra of 2 during singlet oxygen formation measurements with rubrene as a singlet oxygen scavenger. | |
Moreover, the quantum yield of singlet oxygen formation in DMF for compound 1 (0.46) studied here is at the same level as that for Zhang and co-workers’ compound V (0.48, Fig. 3).27 Both compounds 1 and V are demetallated chlorins with substituents having weak electron-donating character. Mesquita and co-workers studied similar structures; the compound VI (Fig. 3) presented by this group is especially interesting.33
In comparison to compound VI, our chlorin 4 possesses a nitro group attached to the chlorin ring. The nitro group possesses electron-withdrawing properties; thus, significant improvement in singlet oxygen formation for 4 (0.86 in DMF) is observed in comparison to that for VI (0.41 in DMF).33
Lipid vesicle preparation
As a lipid formulation, liposomes consisting of phospholipids, ethanol (3.3%) and terpenes, called invasomes, were chosen.45 Because of dark activity of “empty” invasomes against studied microbes (data not shown), it was decided to use ethanol-modified liposomes. There are known liposomes named ethosomes, which consist of phospholipids and 20% or more ethanol.46 Thus, it was not appropriate to use both names, and they were named lipid vesicles (modified liposomes). Ethanol makes lipid vesicles more elastic and enables efficient transport through lipid membranes.46 Moreover, it was proven that elastic vesicles enable delivery of the bacteria-killing agent inside dentinal tubules up to 300 μm, where Enterococcus faecalis can persist despite conventional treatment.13,47 Obtained liposome vesicles were of approx. 200 nm diameter (Table 1) and revealed polydispersity index ca. 0.30.
In vitro photodynamic activity against bacteria and fungi
The photodynamic microbe inactivation potential was evaluated against Gram-positive and negative bacteria as well as fungi. As a member of the first group, Enterococcus faecalis was chosen; it is known as a significant pathogen in periapical lesions and can penetrate dentinal tubules, simultaneously forming biofilms.48,49 Moreover, it is known to show resistance to many antibiotics including vancomycin.13 Studied compounds 1–4 revealed high potential against E. faecalis. Chlorins 1–3 at concentration of 1 μM achieved reduction rates of bacterial growth >5 logs and for 4, the result of ca. 5 logs was obtained (Table 2). According to FDA regulations, reduction in bacterial growth of 3 logs classifies an agent as bactericidal.50 It should be noted that 1 works against bacteria slightly better than 4 although 4 generates singlet oxygen over 2-fold more intensively. A similar phenomenon has been described by our group for the anticancer photodynamic activity of some phthalocyanine derivatives.34 The above observations enable the conclusion that the amount of formed singlet oxygen is not directly correlated with the biological effect. Quaternalized photosensitizers are often recognized as being the most active. Interestingly, a previously published paper by Dlugaszewska and co-workers reported no activity at 1 μM of quaternalized phthalocyanine against this bacterium.51 Recently, Ossmann and co-workers reported reduction in E. faecalis in the root canal system of 3.6 log by Temoporfin incorporated into invasomes at concentration of 50 μM and light dose of 100 J cm−2,13 whereas chlorins 1–4 presented here revealed much higher activity at 1 μM and 30 J cm−2. In our studies, Staphylococcus aureus was chosen as a second example of Gram-positive bacteria; it easily colonizes open wounds and can acquire the methicillin-resistance mechanism (MRSA).52 Within studied chlorins group, the most active against S. aureus was 1 at the concentration of 1 μM (Table 2). The FDA proposed limit of 3 logs was also crossed by 2. Chlorins 3 and 4 revealed inhibitions above 3 log at higher concentrations (10 μM, Table 2). In comparison, Dlugaszewska and co-workers noticed high reduction of S. aureus growth only at the concentration of 100 μM.51 As a Gram-negative bacteria, Escherichia coli was chosen. It is the best-described bacteria and some strains exhibit specific virulence.53 Studied chlorins 1–3 achieved photodynamic inactivation activity at the level of 2 log and 4 at ca. 1 log. Mesquita and co-workers subjected chlorin VI to photodynamic inactivation experiments. The studied compound at concentration of 5 μM revealed only 0.57 log reduction in bacterial growth.33 As examined here, chlorin 4 with nitro group attached to the ring revealed no activity at concentrations of 1 and 10 μM. However, increasing the concentration up to 100 μM gave 0.94 log reduction in E. coli growth. It should be mentioned that quaternalization of VI performed by Mesquita and co-workers resulted only in slight increase of its activity.33 Interestingly, immobilization of these kinds of compounds on silica and Morrifield resin pushed the activity level up to 3 log reduction of E. coli growth.54 Another example of Gram-negative bacteria studied here is Pseudomonas aeruginosa. This pathogen causes serious, life-threatening infections, which are difficult to treat due to the intrinsic resistance of the species to a variety of antimicrobials. P. aeruginosa possesses remarkable ability of acquiring novel mechanisms of resistance to multiple classes of antimicrobial agents (multidrug resistance).55,56P. aeruginosa does not reveal susceptibility to the treatment neither do similarly studied species of fungi Trichophyton mentagrophytes (causing dermatophytosis57) and Candida albicans (a common fungus associated with humans, which can cause serious health problems58) (ESI†). It is well-known that Gram-negative bacteria and fungi are often more difficult to treat with PACT. The reason for this is the specific cell wall structure of these microbes.59
Table 2 Log reduction values of S. aureus, E. faecalis, and E. coli obtained for studied compounds
Log reduction of bacterial growth |
1
|
Bacteria strain |
ATCC |
Conditions |
100 μM |
10 μM |
1 μM |
Staphylococcus aureus
|
25923 |
Light |
— |
5.17 ± 0.07 |
3.93 ± 0.66 |
Dark |
— |
0.21 ± 0.08 |
0.34 ± 0.09 |
Enterococcus faecalis
|
29212 |
Light |
— |
>5.44 ± 0.19 |
>5.44 ± 0.19 |
Dark |
— |
0,1 ± 0.06 |
0.08 ± 0.08 |
Eschericha coli
|
25922 |
Light |
2.14 ± 0.33 |
−0.07 ± 0.1 |
— |
Dark |
0.26 ± 0.07 |
0.02 ± 0.14 |
— |
2
|
Bacteria strain |
ATCC |
Conditions |
100 μM |
10 μM |
1 μM |
Staphylococcus aureus
|
25923 |
Light |
— |
5.34 ± 0.06 |
3.08 ± 0.04 |
Dark |
— |
0.11 ± 0.05 |
0.03 ± 0.01 |
Enterococcus faecalis
|
29212 |
Light |
— |
>5.44 ± 0.19 |
>5.44 ± 0.19 |
Dark |
— |
0.04 ± 0.04 |
0.10 ± 0.2 |
Eschericha coli
|
25922 |
Light |
1.77 ± 0.3 |
−0.05 ± 0.13 |
— |
Dark |
0.15 ± 0.08 |
−0.10 ± 0.11 |
— |
3
|
Bacteria strain |
ATCC |
Conditions |
100 μM |
10 μM |
1 μM |
Staphylococcus aureus
|
25923 |
Light |
— |
3.78 ± 0.07 |
2.75 ± 0.17 |
Dark |
— |
0.19 ± 0.02 |
0.06 ± 0.5 |
Enterococcus faecalis
|
29212 |
Light |
— |
>5.44 ± 0.19 |
>5.44 ± 0.19 |
Dark |
— |
0.03 ± 0.04 |
0.040.09 |
Eschericha coli
|
25922 |
Light |
2.04 ± 0.17 |
−0.03 ± 0.13 |
— |
Dark |
−0.09 ± 0.14 |
0.01 ± 0.1 |
— |
4
|
Bacteria strain |
ATCC |
Conditions |
100 μM |
10 μM |
1 μM |
Staphylococcus aureus
|
25923 |
Light |
— |
3.64 ± 0.2 |
2.71 ± 0.15 |
Dark |
— |
0.16 ± 0.09 |
0.17 ± 0.06 |
Enterococcus faecalis
|
29212 |
Light |
— |
4.33 ± 0.31 |
5.44 ± 0.19 |
Dark |
— |
0.06 ± 0.07 |
0.03 ± 0.08 |
Eschericha coli
|
25922 |
Light |
0.94 ± 0.16 |
−0.07 ± 0.12 |
— |
Dark |
−0.05 ± 0.12 |
0.06 ± 0.07 |
— |
Empty liposomes |
Staphylococcus aureus
|
25923 |
Light |
0.06 ± 0.14 |
Dark |
0.14 ± 0.14 |
Enterococcus faecalis
|
29212 |
Light |
0.08 ± 0.22 |
Dark |
0.13 ± 0.04 |
Eschericha coli
|
25922 |
Light |
0.09 ± 0.13 |
Dark |
0.12 ± 0.12 |
Microtox® analysis
Microtox® bioassay is a fast tool for sample toxicity determination. The test is based on bioluminescence of marine bacteria Vibrio fischeri. The intensity of emitted light is directly connected with living bacteria. Therefore, with 81.9% screening test, a general level of sample toxicity can be assessed. The 81.9% test indicates that the parent sample is diluted with sodium chloride solution, which is necessary for V. fischeri growth.25,26,60 Compounds 2–4 revealed very low toxicity (Fig. 8). Chlorin 1 exhibited 2-fold higher toxicity in comparison with 2 and 3. Interestingly, chlorin 4 caused hormesis phenomenon during the Microtox® test, especially after 15 min of incubation. Hormesis phenomenon is the stimulation of growth at a low dose of an agent, which causes inhibition at higher doses.61 Therefore, the dosage of 4 should be carefully studied to choose the optimal value.
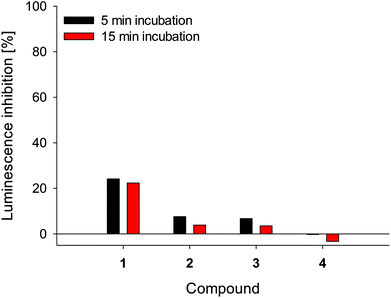 |
| Fig. 8
Vibrio fischeri luminescence inhibition in Microtox® analysis. | |
Conclusions
Presented chlorin derivatives revealed high fluorescence ability and were very efficient singlet oxygen generators. The introduction of electron-withdrawing fluorine atoms caused decrease in fluorescence quantum yields, whereas the introduction of the nitro group to the macro-ring did not change these values. Chlorins dissolved in DMF revealed moderate photostability, whereas in DMSO solutions, they revealed an interesting phenomenon assigned as the phototransformation process. Similar to Foscan®, studied chlorins 1–4 might undergo phototransformation process, further resulting in modified macrocycle formation. Such phenomenon was suggested after the analysis of mass spectra recorded before and after irradiation. The mass spectra after irradiation showed new signals at slightly lower m/z values than those observed for the parent compounds. Studied compounds 1–4 revealed relatively high quantum yields of singlet oxygen formation. It can be noted that by increasing the number of fluorine atoms, the increase in quantum yield of singlet oxygen values was as follows: 1 < 2 ≈ 3 < 4. This tendency can be associated with the electron-withdrawing properties of fluorine atoms. Considering the above facts, compounds 1–4 can be considered as potential photosensitizers for photodynamic therapy. Their insolubility in water environment can be easily overcome by incorporation into lipid vesicles. Presented compounds are active under light against Enterococcus faecalis and reveal low general toxicity. For chlorins 1–3 at the concentration of 1 μM, reduction rates of bacterial growth above 5 log were achieved and the result for 4 was ca. 5 log. The above-mentioned bacteria are the key factor in recurrent apical lesions in dentistry. Therefore, PDT with chlorin photosensitizer can be considered as an adjuvant or even the method of choice for endodontic treatment. Within the studied chlorins group, the most active against S. aureus was 1 at the concentration of 1 μM. Chlorins 2, 3 and 4 revealed high activity at higher concentrations. Gram-negative bacteria Escherichia coli revealed much lower susceptibility to phototreatment with chlorins 1–4. No activity of meso-substituted chlorins was noticed against other Gram-negative bacteria such as Pseudomonas aeruginosa and fungi Candida albicans and Trichophyton mentagrophytes. Microtox® bioassay, a fast tool for toxicity determination, revealed that compounds 2–4 exhibited very low toxicity, chlorin 1 exhibited moderate toxicity, and chlorin 4 caused hormesis phenomenon. All the above aspects of studied chlorins make them suitable for photodynamic antimicrobial chemotherapy.
Conflicts of interest
There are no conflicts to declare.
Acknowledgements
This research has been financially supported by the Polish National Centre of Sciences (Grant No. 2013/11/N/ST5/02040).
Notes and references
- K. T. de Oliveira, P. B. Momo, F. F. de Assis, M. AB Ferreira and T. J. Brocksom, Chlorins: natural Sources, synthetic developments and main applications, Curr. Org. Synth., 2014, 11, 42–58 CrossRef CAS.
- P. Agostinis, K. Berg, K. A. Cengel, T. H. Foster, A. W. Girotti, S. O. Gollnick, S. M. Hahn, M. R. Hamblin, A. Juzeniene, D. Kessel, M. Korbelik, J. Moan, P. Mroz, D. Nowis, J. Piette, B. C. Wilson and J. Golab, Photodynamic therapy of cancer: An update, CA-Cancer J. Clin., 2011, 61, 250–281 CrossRef PubMed.
- R. R. Allison, G. H. Downie, R. Cuenca, X.-H. Hu, C. J. Childs and C. H. Sibata, Photosensitizers in clinical PDT, Photodiagn. Photodyn. Ther., 2004, 1, 27–42 CrossRef CAS PubMed.
- R. R. Allison and C. H. Sibata, Oncologic photodynamic therapy photosensitizers: A clinical review, Photodiagn. Photodyn. Ther., 2010, 7, 61–75 CrossRef CAS PubMed.
- M. Wainwright, T. Maisch, S. Nonell, K. Plaetzer, A. Almeida, G. P. Tegos and M. R. Hamblin, Photoantimicrobials—are we afraid of the light?, Lancet Infect. Dis., 2017, 17, e49–e55 CrossRef PubMed.
- M. R. Hamblin, Antimicrobial photodynamic inactivation: a bright new technique to kill resistant microbes, Curr. Opin. Microbiol., 2016, 33, 67–73 CrossRef CAS PubMed.
- N. Sung, S. Back, J. Jung, K.-H. Kim, J.-K. Kim, J. H. Lee, Y. Ra, H. C. Yang, C. Lim, S. Cho, K. Kim and S. Jheon, Inactivation of multidrug resistant (MDR)- and extensively drug resistant (XDR)-Mycobacterium tuberculosis by photodynamic therapy, Photodiagn. Photodyn. Ther., 2013, 10, 694–702 CrossRef CAS PubMed.
- K. Winkler, C. Simon, M. Finke, K. Bleses, M. Birke, N. Szentmáry, D. Hüttenberger, T. Eppig, T. Stachon, A. Langenbucher, H.-J. Foth, M. Herrmann, B. Seitz and M. Bischoff, Photodynamic inactivation of multidrug-resistant Staphylococcus aureus by chlorin e6 and red light (λ = 670 nm), J. Photochem. Photobiol., B, 2016, 162, 340–347 CrossRef CAS PubMed.
- M. Kryjewski, T. Goslinski and J. Mielcarek, Functionality stored in the structures of cyclodextrin–porphyrinoid systems, Coord. Chem. Rev., 2015, 300, 101–120 CrossRef CAS.
- P. Skupin-Mrugalska, J. Piskorz, T. Goslinski, J. Mielcarek, K. Konopka and N. Düzgüneş, Current status of liposomal porphyrinoid photosensitizers, Drug Discovery Today, 2013, 18, 776–784 CrossRef CAS PubMed.
- S. Jeong, J. Lee, B. N. Im, H. Park and K. Na, Combined photodynamic and antibiotic therapy for skin disorder via lipase-sensitive liposomes with enhanced antimicrobial performance, Biomaterials, 2017, 141, 243–250 CrossRef CAS PubMed.
- N. Dragicevic-Curic, D. Scheglmann, V. Albrecht and A. Fahr, Development of different temoporfin-loaded invasomes—novel nanocarriers of temoporfin: Characterization, stability and in vitro skin penetration studies, Colloids Surf., B, 2009, 70, 198–206 CrossRef CAS PubMed.
- A. Ossmann, S. Kranz, G. Andre, A. Völpel, V. Albrecht, A. Fahr and B. W. Sigusch, Photodynamic killing of Enterococcus faecalis in dentinal tubules using mTHPC incorporated in liposomes and invasomes, Clin. Oral Investig., 2015, 19, 373–384 CrossRef PubMed.
- J. Śniechowska, P. Paluch and M. J. Potrzebowski, Structure and dynamics processes in free-base chlorins controlled by chemical modifications of macroring and aryl groups in meso-positions, RSC Adv., 2017, 7, 24795–24805 RSC.
- V. Chauke, A. Ogunsipe, M. Durmuş and T. Nyokong, Novel gallium(III) phthalocyanine derivatives – Synthesis, photophysics and photochemistry, Polyhedron, 2007, 26, 2663–2671 CrossRef CAS.
- L. Sobotta, P. Fita, W. Szczolko, M. Wrotynski, M. Wierzchowski, T. Goslinski and J. Mielcarek, Functional singlet oxygen generators based on porphyrazines with peripheral 2,5-dimethylpyrrol-1-yl and dimethylamino groups, J. Photochem. Photobiol., A, 2013, 269, 9–16 CrossRef CAS.
- A. Ogunsipe, D. Maree and T. Nyokong, Solvent effects on the photochemical and fluorescence properties of zinc phthalocyanine derivatives, J. Mol. Struct., 2003, 650, 131–140 CrossRef CAS.
- S. Silva, P. M. R. Pereira, P. Silva, F. A. Almeida Paz, M. A. F. Faustino, J. A. S. Cavaleiro and J. P. C. Tomé, Porphyrin and phthalocyanine glycodendritic conjugates: synthesis, photophysical and photochemical properties, Chem. Commun., 2012, 48, 3608 RSC.
- T. H. Tran-Thi, J. F. Lipskier, P. Maillard, M. Momenteau, J. M. Lopez-Castillo and J. P. Jay-Gerin, Effect of the exciton coupling on the optical and photophysical properties of face-to-face porphyrin dimer and trimer: a treatment including the solvent stabilization effect, J. Phys. Chem., 1992, 96, 1073–1082 CrossRef CAS.
- I. Seotsanyana-Mokhosi, N. Kuznetsova and T. Nyokong, Photochemical studies of tetra-2, 3-pyridinoporphyrazines, J. Photochem. Photobiol., A, 2001, 140, 215–222 CrossRef CAS.
- N. A. Kuznetsova, D. A. Makarov, O. A. Yuzhakova, L. I. Solovieva and O. L. Kaliya, Study on the photostability of water-soluble Zn(II) and Al(III) phthalocyanines in aqueous solution, J. Porphyrins Phthalocyanines, 2010, 14, 968–974 CrossRef CAS.
- X. Shen, W. Lu, G. Feng, Y. Yao and W. Chen, Preparation and photoactivity of a novel water-soluble, polymerizable zinc phthalocyanine, J. Mol. Catal. A: Chem., 2009, 298, 17–22 CrossRef CAS.
- A. Ogunsipe, M. Durmuş, D. Atilla, A. G. Gürek, V. Ahsen and T. Nyokong, Synthesis, photophysical and photochemical studies on long chain zinc phthalocyanine derivatives, Synth. Met., 2008, 158, 839–847 CrossRef.
- A. Sivéry, F. Anquez, C. Pierlot, J. M. Aubry and E. Courtade, Singlet oxygen () generation upon 1270 nm laser irradiation of ground state oxygen () dissolved in organic solvents: Simultaneous and independent determination of production rate and reactivity with chemical traps, Chem. Phys. Lett., 2013, 555, 252–257 CrossRef.
- T. X. H. Le, T. V. Nguyen, Z. Amadou Yacouba, L. Zoungrana, F. Avril, D. L. Nguyen, E. Petit, J. Mendret, V. Bonniol, M. Bechelany, S. Lacour, G. Lesage and M. Cretin, Correlation between degradation pathway and toxicity of acetaminophen and its by-products by using the electro-Fenton process in aqueous media, Chemosphere, 2017, 172, 1–9 CrossRef CAS PubMed.
- R. Weltens, K. Deprez and L. Michiels, Validation of Microtox as a first screening tool for waste classification, Waste Manage., 2014, 34, 2427–2433 CrossRef CAS PubMed.
- L.-J. Zhang, L.-X. Wang, W.-L. Zhang, Y.-J. Yan and Z.-L. Chen, Anti-tumor activities of a novel chlorin derivative for photodynamic therapy in vitro and in vivo, J. Innovative Opt. Health Sci., 2015, 8, 1540003 CrossRef CAS.
- K. Aravindu, H.-J. Kim, M. Taniguchi, P. L. Dilbeck, J. R. Diers, D. F. Bocian, D. Holten and J. S. Lindsey, Synthesis and photophysical properties of chlorins bearing 0–4 distinct meso-substituents, Photochem. Photobiol. Sci., 2013, 12, 2089 RSC.
- S. Hirohara, M. Obata, H. Alitomo, K. Sharyo, T. Ando, M. Tanihara and S. Yano, Synthesis, photophysical properties and sugar-dependent in vitro photocytotoxicity
of pyrrolidine-fused chlorins bearing S-glycosides, J. Photochem. Photobiol., B, 2009, 97, 22–33 CrossRef CAS PubMed.
- A. M. G. Silva, A. C. Tomé, M. G. P. M. S. Neves, A. M. S. Silva and J. A. S. Cavaleiro, meso-Tetraarylporphyrins as dipolarophiles in 1,3-dipolar cycloaddition reactions, Chem. Commun., 1999, 1767–1768 RSC.
- S. K. Pandey, A. L. Gryshuk, A. Graham, K. Ohkubo, S. Fukuzumi, M. P. Dobhal, G. Zheng, Z. Ou, R. Zhan, K. M. Kadish, A. Oseroff, S. Ramaprasad and R. K. Pandey, Fluorinated photosensitizers: synthesis, photophysical, electrochemical, intracellular localization, in vitro photosensitizing efficacy and determination of tumor-uptake by 19F in vivo NMR spectroscopy, Tetrahedron, 2003, 59, 10059–10073 CrossRef CAS.
- M. Pineiro, M. M. Pereira, A. d'A. R. Gonsalves, L. G. Arnaut and S. J. Formosinho, Singlet oxygen quantum yields from halogenated chlorins: potential new photodynamic therapy agents, J. Photochem. Photobiol., A, 2001, 138, 147–157 CrossRef CAS.
- M. Q. Mesquita, J. C. J. M. D. S. Menezes, M. G. P. M. S. Neves, A. C. Tomé, J. A. S. Cavaleiro, Â. Cunha, A. Almeida, S. Hackbarth, B. Röder and M. A. F. Faustino, Photodynamic inactivation of bioluminescent Escherichia coli by neutral and cationic pyrrolidine-fused chlorins and isobacteriochlorins, Bioorg. Med. Chem. Lett., 2014, 24, 808–812 CrossRef CAS PubMed.
- L. Sobotta, M. Wierzchowski, M. Mierzwicki, Z. Gdaniec, J. Mielcarek, L. Persoons, T. Goslinski and J. Balzarini, Photochemical studies and nanomolar photodynamic activities of phthalocyanines functionalized with 1,4,7-trioxanonyl moieties at their non-peripheral positions, J. Inorg. Biochem., 2016, 155, 76–81 CrossRef CAS PubMed.
- L. F. Pedrosa, M. C. de Souza, M. A. F. Faustino, M. G. P. M. S. Neves, A. M. S. Silva, A. C. Tomé, V. F. Ferreira and J. A. S. Cavaleiro, Porphyrin - Phosphoramidate Conjugates: Synthesis, Photostability and Singlet Oxygen Generation, Aust. J. Chem., 2011, 64, 939 CrossRef CAS.
- R. M. Jones, Q. Wang, J. H. Lamb, B. D. Djelal, R. Bonnett and C. K. Lim, Identification of photochemical oxidation products of 5, 10, 15, 20-tetra (m-hydroxyphenyl) chlorin by on-line high-performance liquid chromatography-electrospray ionization tandem mass spectrometry, J. Chromatogr., A, 1996, 722, 257–265 CrossRef CAS.
- R. Bonnett and G. Martínez, Photobleaching of Compounds of the 5,10,15,20-Tetrakis(m -hydroxyphenyl)porphyrin Series (m-THPP, m-THPC, and m-THPBC), Org. Lett., 2002, 4, 2013–2016 CrossRef CAS PubMed.
- C. Hadjur, N. Lange, J. Rebstein, P. Monnier, H. van den Bergh and G. Wagnières, Spectroscopic studies of photobleaching and photoproduct formation of meta (tetrahydroxyphenyl) chlorin (m-THPC) used in photodynamic therapy. The production of singlet oxygen by m-THPC, J. Photochem. Photobiol., B, 1998, 45, 170–178 CrossRef CAS.
- J. Zhang, P. Zhang, Z. Zhang and X. Wei, Spectroscopic and Kinetic Studies of Photochemical Reaction of Magnesium Tetraphenylporphyrin with Oxygen, J. Phys. Chem. A, 2009, 113, 5367–5374 CrossRef CAS PubMed.
- M. Ethirajan, Y. Chen, P. Joshi and R. K. Pandey, The role of porphyrin chemistry in tumor imaging and photodynamic therapy, Chem. Soc. Rev., 2011, 40, 340–362 RSC.
- J. F. Lovell, T. W. B. Liu, J. Chen and G. Zheng, Activatable Photosensitizers for Imaging and Therapy, Chem. Rev., 2010, 110, 2839–2857 CrossRef CAS PubMed.
- J. Tang, J.-J. Chen, J. Jing, J.-Z. Chen, H. Lv, Y. Yu, P. Xu and J.-L. Zhang, β-Lactonization of fluorinated porphyrin enhances LDL binding affinity, cellular uptake with selective intracellular localization, Chem. Sci., 2014, 5, 558–566 RSC.
- H. Mojzisova, S. Bonneau, P. Maillard, K. Berg and D. Brault, Photosensitizing properties of chlorins in solution and in membrane-mimicking systems, Photochem. Photobiol. Sci., 2009, 8, 778 RSC.
- J. Piskorz, P. Skupin, S. Lijewski, M. Korpusinski, M. Sciepura, K. Konopka, S. Sobiak, T. Goslinski and J. Mielcarek, Synthesis, physical–chemical properties and in vitro photodynamic activity against oral cancer cells of novel porphyrazines possessing fluoroalkylthio and dietherthio substituents, J. Fluorine Chem., 2012, 135, 265–271 CrossRef CAS.
- P. Lakshmi, B. Kalpana and D. Prasanthi, Invasomes-novel Vesicular Carriers for Enhanced Skin Permeation, Syst. Rev. Pharm., 2013, 4, 26 CrossRef CAS.
- E. Touitou, N. Dayan, L. Bergelson, B. Godin and M. Eliaz, Ethosomes—novel vesicular carriers for enhanced delivery: characterization and skin penetration properties, J. Controlled Release, 2000, 65, 403–418 CrossRef CAS PubMed.
- I. B. Jurič, V. Plečko, D. G. Pandurić and I. Anić, The antimicrobial effectiveness of photodynamic therapy used as an addition to the conventional endodontic re-treatment: A clinical study, Photodiagn. Photodyn. Ther., 2014, 11, 549–555 CrossRef PubMed.
- Z. Lim, J. Cheng, T. Lim, E. Teo, J. Wong, S. George and A. Kishen, Light activated disinfection: an alternative endodontic disinfection strategy, Aust. Dent. J., 2009, 54, 108–114 CrossRef CAS PubMed.
- I. Portenier, T. M. Waltimo and M. Haapasalo,
Enterococcus faecalis–the root canal survivor and ‘star'in post-treatment disease, Endod. Topics, 2003, 6, 135–159 CrossRef.
- FDA Briefing Document: Anti-Infective Drugs Advisory Committee Meeting October 17, 2013.
- J. Dlugaszewska, W. Szczolko, T. Koczorowski, P. Skupin-Mrugalska, A. Teubert, K. Konopka, M. Kucinska, M. Murias, N. Düzgüneş, J. Mielcarek and T. Goslinski, Antimicrobial and anticancer photodynamic activity of a phthalocyanine photosensitizer with N -methyl morpholiniumethoxy substituents in non-peripheral positions, J. Inorg. Biochem., 2017, 172, 67–79 CrossRef CAS PubMed.
- G. Y. Liu, Molecular Pathogenesis of Staphylococcus aureus Infection, Pediatr. Res., 2009, 65, 71R–77R CrossRef PubMed.
- J. B. Kaper, J. P. Nataro and H. L. T. Mobley, Pathogenic Escherichia coli, Nat. Rev. Microbiol., 2004, 2, 123–140 CrossRef CAS PubMed.
- M. Q. Mesquita, J. C. J. M. D. S. Menezes, S. M. G. Pires, M. G. P. M. S. Neves, M. M. Q. Simões, A. C. Tomé, J. A. S. Cavaleiro, Â. Cunha, A. L. Daniel-da-Silva, A. Almeida and M. A. F. Faustino, Pyrrolidine-fused chlorin photosensitizer immobilized on solid supports for the photoinactivation of Gram negative bacteria, Dyes Pigm., 2014, 110, 123–133 CrossRef CAS.
- M. Buhl, S. Peter and M. Willmann, Prevalence and risk factors associated with colonization and infection of extensively drug-resistant Pseudomonas aeruginosa: a systematic review, Expert Rev. Anti-Infect. Ther., 2015, 13, 1159–1170 CrossRef CAS PubMed.
- K. Poole,
Pseudomonas Aeruginosa: Resistance to the Max, Front. Microbiol. DOI:10.3389/fmicb.2011.00065.
- N. Zaias and G. Rebell, Clinical and mycological status of the Trichophyton mentagrophytes (interdigitale) syndrome of chronic dermatophytosis of the skin and nails, Int. J. Dermatol., 2003, 42, 779–788 CrossRef PubMed.
- F. L. Mayer, D. Wilson and B. Hube, Candida albicans pathogenicity mechanisms, Virulence, 2013, 4, 119–128 CrossRef PubMed.
- T. G. St. Denis, T. Dai, L. Izikson, C. Astrakas, R. R. Anderson, M. R. Hamblin and G. P. Tegos, All you need is light: Antimicrobial photoinactivation as an evolving and emerging discovery strategy against infectious disease, Virulence, 2011, 2, 509–520 CrossRef PubMed.
- V. R. Urbano, M. G. Maniero, M. Pérez-Moya and J. R. Guimarães, Influence of pH and ozone dose on sulfaquinoxaline ozonation, J. Environ. Manage., 2017, 195, 224–231 CrossRef CAS PubMed.
- E. J. Calabrese, Hormesis: principles and applications, Homeopathy, 2015, 104, 69–82 CrossRef PubMed.
Footnote |
† Electronic supplementary information (ESI) available. See DOI: 10.1039/c8pp00258d |
|
This journal is © The Royal Society of Chemistry and Owner Societies 2019 |
Click here to see how this site uses Cookies. View our privacy policy here.