Synthesis and characterization of propeller-shaped mono- to hexacationic quinolinium-substituted benzenes†
Received
13th February 2019
, Accepted 2nd April 2019
First published on 5th April 2019
Abstract
Diels–Alder reaction of 2-, 3- and 4-(phenylethynyl)quinolines and tetraphenylcyclopentadienone gave three regioisomeric 2,3,4,5,6-pentaphenyl-1-(quinolin-2-yl, -3-yl, and -4-yl)benzenes. Restricted rotation of the 3-yl and 4-yl substituted derivatives is observed between the central core and the substituents, resulting in propeller-shaped molecules. Likewise, 1,2-diquinolinyl-3,4,5,6-tetraphenylbenzenes with 3-yl,3-yl and 3-yl,4-yl connectivity were prepared. As evidenced by NMR spectroscopy, they form two diasteromers due to their restricted rotation. A cobalt-catalyzed [2 + 2 + 2]-cyclotrimerization of 2-(phenylethynyl)quinoline resulted in the formation of triphenyl-2,4,6- and -3,5,6-tri(quinolin-2-yl)benzenes. The same reaction was applied to 3,3′-ethyne-1,2-diyldiquinoline which formed hexa(quinolin-3-yl)benzene. N-Methylation gave the title compounds. Among those, the hexacationic hexa(N-methylquinolinio-3-yl)benzene is described. Stereochemical aspects are predominantly discussed by means of results of NMR experiments. DFT-calculations on the most stable conformations and the frontier orbital profiles of the hexacation as well as of its neutral precursor have been carried out.
Introduction
Considerable attention has been directed toward heterocycle polycations as they are interesting in natural product chemistry, heterocyclic chemistry, and materials chemistry. Thus, polypyridinium alkaloids (halitoxin,1 cyclostellettamin C,2 amphitoxin,3 viscosamine,4 viscosaline,5 pachychalines A–C6) are polycationic molecules from nature. Streitwieser et al. reported on series of polypyridinium salts and betaines which have adjacent heteroarenium rings in conjugation.7 Thus, the five-fold pyridinium substituted cyclopentadiene anion 18 possesses structure elements of conjugated mesomeric betaines. The SASAPOS protocol (
elf-
ctivated
ilyl-
ssisted
oly
nio
ubstitution) by Weiss et al. allowed for the synthesis of a variety of heteroarenium substituted substrates, for which 2 is given as an example here.9 This widely applicable protocol10 takes advantage of the fact that a substrate which bonds neutral ligands such as chloride undergoes a substitution with heteroaromatic nucleophiles equivalent to the quantity of the bonding ligands in the presence of the same number of equivalents of trimethylsilyltriflate (TMSOTf). We reported on heterocycle polycations with heteroaromatic central cores such as pyridine,11 pyrimidine,12 pyrazine,13 pyridazine,13 1,3,5-triazine,13 and purine.13 An example is pentacation 3.14 Pyridine polycations proved to be versatile starting materials for the synthesis of highly substituted pyridines with various substitution patterns.11 Apart from their synthetic applicabilities, heterocycle polycations are of interest as potential semiconductors,15 photosensitive materials,16 oxidants,17 and biologically active compounds like herbicides,18 acetylcholinesterase reactivators,19 and cholinesterase inhibitors.20 A recent review article summarizes results achieved so far (Scheme 1).21
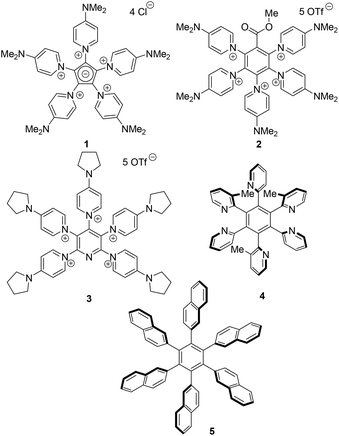 |
| Scheme 1 Examples of heterocycle polycations and of propeller-shaped molecules. | |
Steric hindrance exerted by the peripheral ligands cause propeller-shaped molecules. Recently, the sterically congested hexa(heteroaryl)benzene (HHAB) 4 was formed as two isolable isomers which differ only in the rotation of one methyl-pyridine group.22 In general, propeller-shaped hexaarylbenzenes (HABs) such as hexa(β-naphthyl)benzene 523 have numerous applications in materials sciences as they play roles as liquid crystals,24 microporous organic solids,25 molecular capsules,26 supramolecular electronic materials,27 molecular rotors,28 nonlinear optical materials,29 metal sensors,30 redox materials,31 and molecular wires.32 We report here on quinolinium-substituted benzenes which combine the features of HHABs and polycations as they have to adopt propeller-shaped configurations due to the additional benzo-annulation in comparison to their pyridinium derivatives.
Results and discussion
First, Sonogashira–Hagihara coupling reactions were used to prepare three isomeric ethynyl-substituted quinolines as starting materials for the synthesis of monoquinolinyl-substituted pentaphenylbenzenes, which are of potential interest as ligands of organic electroluminescent device materials.33 Thus, the 2-, 3-, and 4-halosubstituted quinolines 6a–c and phenylacetylene 7 were reacted to give the quinolines 8a–c in good yields (Scheme 2). With these compounds in hand, we examined the [4 + 2]-cycloaddition with tetraphenylcyclopentadione 9. The reaction did not proceed under a variety of different conditions that were tested, among those reflux temperature in benzene, toluene, and xylene, respectively. On increasing the reaction temperature to 305 °C by using benzophenone as a solvent, however, the quinolines 10a–c were finally prepared within 30 min in moderate yields.
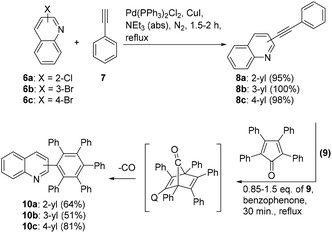 |
| Scheme 2 Synthesis of monoquinoline-substituted HHABs. | |
Similar to hexaphenylbenzene, the six peripheral rings of 10a–c cannot lie in the plane of the central benzene ring. Whereas in solution, on the NMR time scale, the peripheral rings are perpendicular to the plane of the central ring in the absence of appropriate substitutions, the X-ray structure of hexaphenylbenzene itself showed a propeller conformation with angles around the Ph–Ph bonds of approximately 65°.34 It is known that methyl and methoxy groups in C6Ar6 systems in ortho position cause a barrier of rotation of approximately 33 kcal mol−1, whereas this value is considerably decreased to approximately 17 kcal mol−1 for the case of meta-substitutions.23 Hexaphenylbenzene C6Ph6 consequently displays one set of signals in the 13C NMR spectra and one overlapped signal with a center of gravity at 6.83 ppm (30H) in the proton resonance spectra.35 The chemical shifts of 10a–c are different as a consequence of their isomerism and, in addition, intermolecular interactions (Fig. 1). The broadening of the signals at approximately 7.00 ppm in the spectrum of 10a (blue) can be attributed to N⋯H–C interactions to the nitrogen atom, which is not possible in 10b,c. These interactions are thought to be within the limits of hydrogen bonds and classical van der Waals contacts.36 The upfield shift of phenyl ring signals due to intramolecular π-interactions caused by the quinolin-4-yl substitution of 10c can clearly be seen (black), which are less in 10a,b.
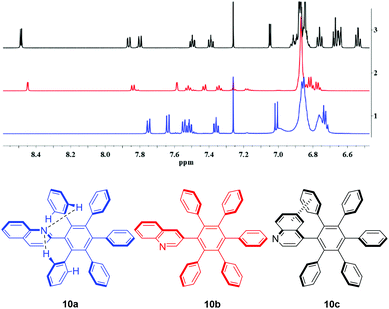 |
| Fig. 1 Comparison of 1H NMR spectra of HHABs 10a (1), 10b (2), and 10c (3) (DMSO-d6, 25 °C). | |
The steric hindrance of the quinoline rings can be compared with 1-(3,4-dimethylphenyl)-2,3,4,5,6-pentaphenylbenzene37 for 10a,b and 1-(2,3-dimethylphenyl)-2,3,4,5,6-pentaphenylbenzene, which seems to be unknown, for 10c. However, the quinolin-4-yl-substitution pattern of 10c imitates the steric hindrance of the methyl group in the model compound 1-(2-methylphenyl)-2,3,4,5,6-pentaphenylbenzene which has a propeller-type topology in the solid38 and a slow rotation of the o-tolyl group 25 °C in solution.39 For the case of free rotation under the measurement conditions, 25 distinct 13C NMR resonance frequencies can be expected for 10a–c, among those 15 signals of CH groups. For the case of restricted rotation, this number is increased to 29 13C NMR signals in total, and 19 signals of CH groups, because the ortho- and meta-positions of the 2- and 3-phenyl substituents become non-isochronous. The least sterically hindered compound 10a displays 22 distinct signals due to overlapping in DMSO-d6 at 25 °C. Compound 10b shows the expected 29 signals for a restricted rotation, and 10c displays in total 27 distinct resonance frequencies under the same measurement conditions, presumably due to overlapping of two signals.
The same protocol was applied to the reaction of quinoline 14 with two alkynyl residues which was prepared in 21% yield in three steps from 1,4-dibromobenzene 11via12 and 13 as shown (Scheme 3). Quinoline 14 reacted with an excess of cyclopentadienone 9 to give compound 15 which precipitated as exclusive reaction product during the work-up procedure in good yield.
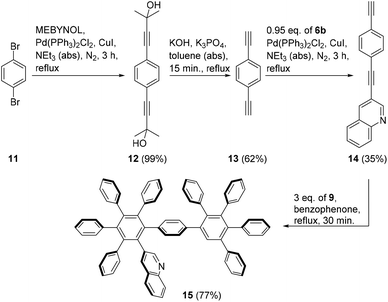 |
| Scheme 3 Synthesis of a bulky monoquinoline HHAB. | |
The method is also applicable to prepare isomeric diquinolinyl substituted tetraphenylbenzenes (Scheme 4). Thus, under analogous conditions, the compound 19a with quinolin-3-yl/3-yl connectivity and the isomeric quinolin-3-yl/4-yl derivative 19b were prepared in 90% and 78% yield, respectively, starting from 18a,b which are available starting from 6b,c and 17 by standard procedures. The quinolin-3-yl,3-yl compound 19a can exist in two diastereomeric forms due to restricted rotation, a Cs conformation and a racemic pair with C2 symmetry, similar to hexaarylbenzenes with two meta-substituents at 0 °C on the NMR time scale.34,40 The 1H NMR spectrum of 19a showed 37 of expectable 48 13C NMR signals. Two sets of partially overlapped signals can be identified in a ratio of 1
:
0.8 under the measuring conditions (DMSO-d6, 25 °C). The 4-H hydrogen atom of the quinoline residues of one isomer appears at 7.97 ppm, whereas the other is detectable at 7.93 ppm. The 13C NMR spectrum of isomer 19b, which possesses a quinolin-3-yl,4-yl connectivity, shows 72 distinct signals of expectable 96. This number is due to the fact, that the 3-yl,4-yl connectivity causes an additional non-symmetry of the molecule and consequently non-isochronous substituents of the two magnetically inequivalent isomers. Under the same measuring conditions, two isochronous rotameric forms are present in a ratio of 1
:
1 in the NMR spectrum of 19b.
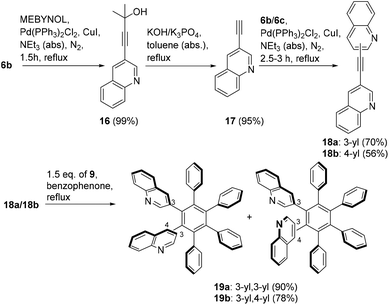 |
| Scheme 4 Synthesis of diquinoline-substituted HHABs. | |
For the synthesis of HHABs with three quinoline residues another approach was applied. Thus, the asymmetric acetylene 8a was subjected to a cobalt-catalyzed [2 + 2 + 2]-trimerization reaction which has already been applied for the synthesis of HABs before (Scheme 5).41 The trimerization of 8a gave a mixture of two separable regioisomers 20a and 20b (1
:
3) with a total yield of 40%. Compound 20a is a heteroaromatic analogue of 1,3,5-tri(α-naphthyl)-benzene, a propeller-like, non-planar molecule that is known to interlock in the melt.42 It displays 15 13C NMR signals, similar to the corresponding number of signals of D3h symmetric oligophenylenes which were expected within the fast exchange limit for all single bond rotations.43 Similar to the spectra of the mono(quinolin-2-yl)derivative 10a, the signals of the ortho- and meta-protons appear as broad singlets due to N⋯H–C interactions. Assignment of all atoms using 2D spectra was possible; one set of proton signals for all three quinoline residues of 20a was found. It is known that ortho-substitutions, which cannot be taken as a model for the quinolin-2-yl substitution, can prevent rapid interconversion of the C3v and Cs isomeric forms which are the result of the formation of syn- and anti-conformations.44 The 1,2,4-substituted compound 20b displays 36 of expectable 45 distinct 13C NMR resonance frequencies in DMSO-d6 at 25 °C, if free rotation under the measurement conditions is presumed. Similar to compound 10a, a considerable broadening of the 1H NMR signals of the quinolines in DMSO-d6 at 25 °C as well as the phenyl rings is observable.
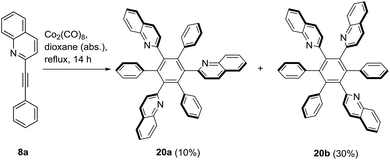 |
| Scheme 5 Cobalt-catalyzed [2 + 2 + 2]-cyclotrimerization. | |
Trimerization of the acetylene 18a was tested to prepare a hexaquinolin-3-yl substituted benzene. Indeed, HHAB 21 was formed in 85% yield (Scheme 6). Unfortunately, due to the rather limited solubility of 21 NMR analyses were not possible. Nonetheless, the corresponding HRMS (m/z = 863.2896) is in accord with the structure of 21 which can exist in eight rotameric forms. Literature-known23 calculated minimized energies of hexa(β-naphthyl)benzene, the non-heteroaromatic analogue of 21, revealed that the most stable rotamer is the one in which all β-naphthyl residues are twisted onto the same side (6,0). The X-ray crystal analysis of hexa(2-pyridyl)benzene, however, revealed the α,β,α,β,α,β arrangement of pyridine rings and dihedral angles between the pyridyl substituent and the benzene ring of approximately 90°.22
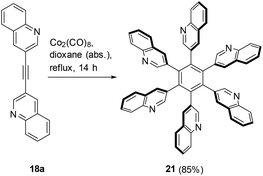 |
| Scheme 6 Cobalt-catalyzed [2 + 2 + 2]-cyclotrimerization of 18a. | |
Synthesis of polycationic HABs
Based on former experiences of our group,45 dimethyl sulfate was used as a methylation agent to convert the quinoline derivatives into cationic species. First, the monoquinoline substituted HABs 10a–c were successfully methylated in anhydrous toluene under reflux conditions (Scheme 7). The N-methyl group of 22a interlocks the molecule and 19 distinct 13C NMR signals of CH groups are detectable plus the signals of the anion and the methyl group at 52.8 and 42.5 ppm, respectively. Thus, in contrast to 10a–c, 22a–c all display the same number of signals. The N-methylquinolinium salts of 15 with methylsulfate (23) and hexafluorophosphate anions (23PF6) were prepared as well, and the diquinoline HABs 19a,b were also successfully methylated. An immediate anion exchange reaction during work-up yielded the dihexafluorophosphate salts 24a,b. Similar to the non-methylated precursors 19a and 19b, the number of 13C NMR resonance frequencies of 24a and 24b is 38 and 72, respectively. The 3-yl,3-yl salt 24a has an almost identical ratio of rotamers (1
:
0.9) as its non-methylated precursor, and the corresponding ratio of the salt 24b in DMSO-d6 is 1
:
1.27. In summary, except for 10a, no spectroscopically detectable changes of the symmetry of the molecules is caused by the methylations. On methylation, the UV/Vis absorption maxima display bathochromic shifts. The spectra are shown in the ESI.†
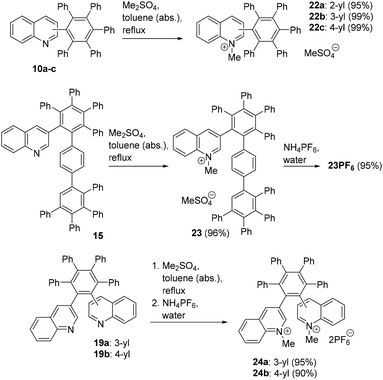 |
| Scheme 7 Methylation of quinoline-based HHABs. | |
Finally, N-methylation of 21 with an excess of dimethyl sulfate followed by precipitation with NH4PF6 successfully gave the fully methylated hexacationic HHAB 25 (Scheme 8) which is soluble in DMSO-d6 and which could be characterized completely.
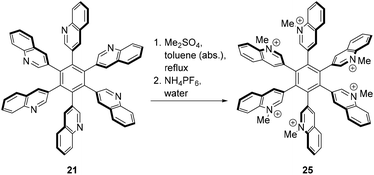 |
| Scheme 8 Methylation of perquinoline-substituted HAB 21. | |
Theoretically, the salt 25 can exist in eight rotameric forms A–H. Similar to hexa(β-naphthyl)benzene,23 solving the simple combinatoric problem created by propeller-like compounds such as 21 or 25 results in the following ratio: A
:
B
:
C
:
D
:
E
:
F
:
G
:
H = 1
:
6
:
3
:
6
:
6
:
3
:
6
:
1. Fig. 2 shows possible interconversions between the forms A–H, which we calculated in form of the hexacationic species (DFT, 6-31G*/PBE0). Schematically, the rotamers can be classified as those in which N-methylquinolinium residues are 6
:
0 (all-syn), 5-up/1-down, 4 up/2-down (1,2; 1,3; 1,4), and 3-up/3-down (1,2,3; 1,2,4; 1,3,5) (Fig. 2). The calculated energy differences between the eight rotamers of 25 are extremely small, and so are the differences between the rotamers of 21. The lowest energy rotamer of 25 is the 3-up-3-down (1,3,5) isomer, whereas the all-syn isomer (6,0) has an energy of 7.65 kJ mol−1 and is thus the less stable. Similarly, the (6,0) rotamer of the neutral precursor 21 is by only 3.8 kJ mol−1 less stable than the (3,3)-1,3,5 rotamer. As a matter of fact, the rotamers of 25 cause signal overlaps in the 1H NMR spectrum at ambient temperature (Fig. 1, spectrum 1, ESI†). The spectra change reversibly on heating of the NMR sample successively from rt to 100 °C (Fig. 1, spectra 2–9); on cooling, the original spectrum is reconstituted (Fig. 1, spectrum 10). Obviously, no other ratio of sets of isochronous rotamers is formed during this temperature experiment. Calculations of all true minimum structures of 25 show that in all rotamers the dihedral angles between the quinoline and the phenyl rings are in the range from 71° to 88°.
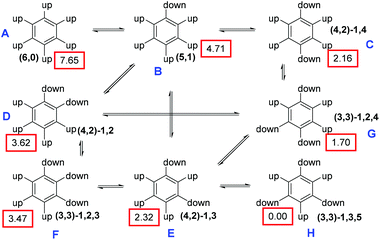 |
| Fig. 2 Eight energy-minimized rotamers of 25, showing which can be directly interconverted by rotation of a single substituent. Relative energies (in kJ mol−1) are shown in red boxes. | |
Fig. 3 shows the calculated most stable conformer of 25 and the HOMO/LUMO profiles of the 25 (3,3)-1,3,5 rotamer. In contrast to 22b,46 in which the HOMO is separated from the LUMO, the HOMO (–19.41 eV) as well as LUMO (−15.48 eV) of 25 are located individually on the six N-methylquinolinium-3-yl residues. Considerable parts of the LUMO are located on the nitrogen atoms as well as on the C2/C4 carbon atoms of the quinolinium residues, whereas significant parts of the HOMO are located on their fused benzene rings. The fraction of the HOMO located at C3 of the (3,3)-1,3,5- as well as of the all-syn (6,0) rotamer (cf. ESI†) is lying in one plane and is orientated to the opposite phases of neighboring C3, similar to the in-plane-aromatic stabilizations due to interactions between the in-plane ethynyl π-orbitals in hexaethynylbenzene.47 This may contribute to the stability of 25. The frontier orbitals of the neutral precursor 21 are shown in the ESI.†
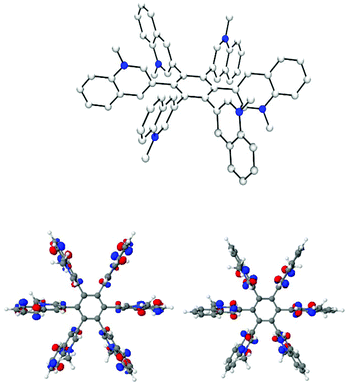 |
| Fig. 3 Most stable conformation of 25 (calculated) (above). HOMO (left) and LUMO (right) of 25 (3,3)-1,3,5. | |
Conclusions
Series of propeller-shaped quinolinium-substituted benzenes as well as their electrostatically neutral precursors have been prepared under variation of the substitution site of the quinoline ring (2-yl, 3-yl, 4-yl), covering the range from the monocationic quinolinium-2,3,4,5,6-pentaphenylbenzene to the hexacationic hexakis(1-methylquinolinium-3-yl)benzene. The latter can exist in eight different rotamers, the (3,3)-1,3,5-isomer of which was calculated to be the most stable one. Due to the propeller-shape geometry which suppresses conjugation throughout the entire π-electron system, the frontier orbitals are located in the individual quinolinium rings.
Experimental
All reactions were carried out under an atmosphere of nitrogen in flame or oven-dried glassware. All chemicals were purchased and used without further purification unless otherwise mentioned. Anhydrous solvents were dried according to standard procedures before usage. Melting points are uncorrected and were determined in an apparatus according to Dr Tottoli (Büchi). The ATR-IR spectra were obtained on a Bruker Alpha in the range of 400 to 4000 cm−1. 1H NMR spectra were recorded at 600 MHz. 13C NMR spectra were recorded at 150 MHz, with the solvent peak used as the internal reference. Multiplicities are described by using the following abbreviations: s = singlet, d = doublet, t = triplet, q = quartet, and m = multiplet. Spectroscopic atom numberings are shown in the ESI.† Signal orientations in DEPT experiments were described as follows: o = no signal; + = up (CH, CH3); − = down (CH2). The mass spectra (ESIMS) were measured with a Varian 320 MS Triple Quad GC/MS/MS (EIMS) or with an Agilent LCMSD series HP 1100 with APIES at fragmentor voltages as indicated. Samples were sprayed from MeOH at 4000 V capillary voltage and fragmentor voltages of 30 V unless otherwise noted. The HRMS spectra were obtained with a Bruker Impact II, a Bruker Daltonik Tesla-Fourier transform-ion cyclotron resonance mass spectrometer, or with a Waters Micromass LCT with the direct inlet. Chromatography: The reactions were traced by thin layer chromatography with silica gel 60 (F254, company MERCK KGAA). For the detection of substances, quenching was used at either 254 nm or 366 nm with a mercury lamp. The preparative column chromatography was conducted through silica gel 60 (230–400 mesh) of the company MERCK KGAA. Yields are not optimized.
Calculations
All density-functional theory (DFT)-calculations were carried out by using the Firefly 8.2.0 QC package,48 which is partially based on the GAMESS (US) source code,49 running on Linux 2.6.18-238.el5 SMP (x86_64) on five AMD Phenom II X6 1090T processor workstations (Beowulf-cluster) with Infiniband interconnect and parallelized with MPICH 1.2.7p1. MM2 optimized structures were used as starting geometries. Complete geometry optimizations were carried out on the implemented 6-31G* basis set and with the PBE0 density functional. All calculated structures were proven to be true minima by the absence of imaginary frequencies. Partial charges were obtained with NBO 5.950 from the results of the DFT calculations. Orbital plots were obtained using Jmol 14.27.2.
Synthesis
General procedure of Sonogashira–Hagihara coupling (Procedure 1)
The reactions were carried out under a nitrogen atmosphere. A mixture of 5 mmol of corresponding haloquinoline 1, 1 mol% of Pd(PPh3)2Cl2, and 2 mol% of CuI was suspended in 7 mL of dry NEt3 with stirring. A sample of the corresponding ethynylbenzene 2 (1.05 equiv.) in dry NEt3 was added dropwise at ambient temperature. The resulting solutions were then stirred at reflux temperature until complete conversion was monitored by TLC. The mixtures were then allowed to cool to rt. The solvents were removed in vacuo. The resulting residues were finally purified by column chromatography (petroleum ether
:
ethyl acetate) to afford the products.
2-(Phenylethynyl)quinoline (8a).
According to Procedure 1, a solution of 0.491 g (3.00 mmol) of 2-chloroquinoline 6a, 0.042 g (0.06 mmol) of Pd(PPh3)2Cl2, 0.012 g (0.06 mmol) CuI and 0.367 g (3.6 mmol) of ethynylbenzene 7 in 20 mL of anhydrous NEt3 was heated over the period of 2 h under reflux temperature. Finally, a purification by column chromatography (petroleum ether
:
ethyl acetate = 5
:
1) gave 2-(phenylethynyl)quinoline 8a. Yield 0.653 g, 95%, a yellow solid, m.p. 75 °C (72–75 °C (ref. 51)). 1H NMR (600 MHz, CDCl3): δ = 8.44 (d, J = 8.3 Hz, 1H, 4-H), 8.03–8.01 (m, 2H, 5-H, 8-H), 7.82 (ddd, J = 1.5, 6.7, 8.5 Hz, 1H, 7-H), 7.74 (d, J = 8.3 Hz, 1H, 3-H), 7.70–7.68 (m, 2H, 2′-H, 6′-H), 7.66 (ddd, J = 1.2, 6.9, 8.1 Hz, 1H, 6-H), 7.53–7.48 (m, 3H, 3′-H, 4′-H, 5′-H) ppm. 13C NMR (150 MHz, CDCl3): δ = 147.7 (o, C8a), 142.6 (o, C2), 136.7 (+, C4), 131.9 (+, C2′, C6′), 129.7 (+, C4′), 128.9 (+, C3′, C5′), 128.6 (+, C5), 128.0 (+, C8), 127.4 (+, C6), 126.9 (o, C4a), 124.3 (+, C3), 121.2 (o, C1′), 89.5 (o, Cα), 89.1 (o, Cβ) ppm. IR (ATR): 3053, 2208, 2161, 1616, 1591, 1552, 1498, 1441, 1425, 1310, 1295, 1211, 1142, 1115, 1070, 989, 829, 788, 769, 760, 693, 620, 548, 526, 478 cm−1. HRMS (ESI): m/z calcd for C17H12N [M + H]+ 230.0965, found 230.0969; m/z calcd for C17H11NNa [M + Na]+ 252.0789, found 252.0789.
3-(Phenylethynyl)quinoline (8b).
According to Procedure 1, a solution of 2.080 g (10.00 mmol) of 3-bromoquinoline 6b, 0.070 g (0.10 mmol) of Pd(PPh3)2Cl2, 0.038 g (0.20 mmol) CuI and 1.071 g (10.50 mmol) of ethynylbenzene 7 in 30 mL of anhydrous NEt3 was heated over the period of 1.5 h under reflux temperature. Finally, a purification by column chromatography (petroleum ether
:
ethyl acetate = 3
:
1) gave 3-(phenyl-ethynyl)quinoline 8b. Yield 2.290 g, 100%, a white solid, m.p. 83 °C. 1H NMR (600 MHz, CDCl3): δ = 9.00 (d, J = 2.0 Hz, 1H, 2-H), 8.30 (d, J = 2.0 Hz, 1H, 4-H), 8.10 (d, J = 8.3 Hz, 1H, 8-H), 7.79 (d, J = 8.3 Hz, 1H, 5-H), 7.72 (ddd, J = 1.5, 6.9, 8.3 Hz, 1H, 7-H), 7.63–7.53 (m, 3H, 6-H, 2′-H, 6′-H), 7.42–7.35 (m, 3H, 3′-H, 4′-H, 5′-H) ppm. 13C NMR (150 MHz, CDCl3): δ = 152.1 (+, C2), 146.8 (o, C8a), 138.3 (+, C4), 131.8 (+, C2′, C6′), 130.1 (+, C7), 129.4 (+, C8), 128.9 (+, C4′), 128.5 (+, C3′, C5′), 127.6 (+, C6), 127.3 (+, C5), 127.3 (o, C4a), 122.6 (o, C1′), 117.5 (o, C3), 92.7 (o, Cβ), 86.7 (o, Cα) ppm. IR (ATR): 3008, 2162, 1619, 1599, 1564, 1484, 1443, 1409, 1368, 1294, 1196, 1124, 1113, 1072, 980, 905, 860, 784, 756, 691, 656, 640, 539, 515, 498, 474, 429 cm−1. HRMS (ESI): m/z calcd for C17H11NNa [M + Na]+ 252.0789, found 252.0796.
4-(Phenylethynyl)quinoline (8c).
According to Procedure 1, a solution of 0.624 g (3.00 mmol) of 4-bromoquinoline 6c, 0.042 g (0.06 mmol) of Pd(PPh3)2Cl2, 0.012 g (0.06 mmol) CuI and 0.367 g (3.6 mmol) of ethynylbenzene 7 in 20 mL of anhydrous NEt3 was heated over the period of 2 h under reflux temperature. Finally, a purification by column chromatography (petroleum ether
:
ethyl acetate = 5
:
1) gave 4-(phenylethynyl)quinoline 8c. Yield 0.674 g, 98%, of a yellow solid, m.p. 45 °C. 1H NMR (600 MHz, CDCl3): δ = 8.90 (d, J = 4.4 Hz, 1H, 2-H), 8.38 (ddd, J = 0.6, 1.4, 8.3 Hz, 1H, 5-H), 8.14 (d, J = 8.1 Hz, 1H, 8-H), 7.76 (ddd, J = 1.4, 6.9, 8.4 Hz, 1H, 7-H), 7.68–7.66 (m, 2H, 2′-H, 6′-H), 7.64 (ddd, J = 1.4, 6.9, 8.2 Hz, 1H, 6-H), 7.57 (d, J = 4.4 Hz, 1H, 3-H), 7.44–7.42 (m, 3H, 3′-H, 4′-H, 5′-H) ppm. 13C NMR (150 MHz, CDCl3): δ = 149.9 (+, C2), 148.3 (o, C8a), 132.1 (+, C2′, C6′), 130.0 (+, C7, C8), 129.9 (o, C4), 129.5 (+, C4′), 128.7 (+, C3′, C5′), 127.9 (o, C4a), 127.3 (+, C6), 126.1 (+, C5), 123.7 (+, C3), 122.4 (o, C1′), 98.8 (o, Cβ), 85.2 (o, Cα) ppm. IR (ATR): 3085, 3051, 3029, 2998, 2212, 1961, 1919, 1595, 1576, 1505, 1487, 1441, 1418, 1390, 1363, 1310, 1277, 1217, 1192, 1175, 1134, 1068, 1029, 919, 871, 846, 815, 756, 687, 641, 579, 550, 529, 506, 485, 442 cm−1. HRMS (ESI): m/z calcd for C17H12N [M + H]+ 230.0965, found 230.0965.
General procedure of preparation of propeller-like compounds (Procedure 2)
Benzophenone (10 g) was melted in a 50 mL round-bottomed flask fitted with an air condenser. Corresponding phenylethynylquinoline 8 (2.00 mmol) and tetraphenylcyclopentadienone 9 (2.50 mmol) were added to the flask, which was heated for 0.5 h using a heat gun. The solution was cooled to rt and toluene (10 mL) was added to prevent the solidification of the benzophenone. After cooling, n-hexane (50 mL) was added, resulting in the precipitation of a product, which was collected by vacuum filtration.
2,3,4,5,6-Pentaphenyl-1-(quinolin-2-yl)benzene (10a).
2,3,4,5,6-Pentaphenyl-1-(quinoline-2-yl)benzene 10a was prepared by Procedure 2 using 2-(phenylethynyl)quinoline 8a (0.158 g, 0.690 mmol) and tetraphenylcyclopentadienone 9 (0.394 g, 1.035 mmol) in benzophenone (5 g) in 10 mL round-bottomed flask in 0.75 h. Yield 0.260 g, 64%, a white solid, m.p. >360 °C. 1H NMR (600 MHz, CDCl3): δ = 7.75 (d, J = 8.6 Hz, 1H, 8i-H), 7.64 (d, J = 8.5 Hz, 1H, 4i-H), 7.55 (dd, J = 1.2, 8.2 Hz, 1H, 5i-H), 7.51 (ddd, J = 1.5, 6.9, 8.5 Hz, 1H, 7i-H), 7.36 (ddd, J = 1.1, 7.0, 8.1 Hz, 1H, 6i-H), 7.01 (d, J = 8.5 Hz, 1H, 3i-H), 7.00–6.96 (m, 2H, Ph), 6.93–6.83 (m, 16 H, 2ii-H, 6ii-H, 2iii-H, 6iii-H, 2v-H, 6v-H, 2vi-H, 6vi-H, 3ii-H, 5ii-H, 3iii-H, 5iii-H, 3v-H, 5v-H, 3vi-H, 5vi-H), 6.79–6.72 (m, 7H, Ph) ppm. 13C NMR (150 MHz, CDCl3): δ = 160.2 (o, C2i), 147.1 (o, C8ai), 141.6 (o, C1iv), 140.8/140.4/140.3/140.1 (o, C2, C3, C5, C6, C1ii, C1iii, C1v, C1vi), 140.6 (o, C4), 140.0 (o, C1), 134.2 (+, C4i), 131.7/131.5/131.4/130.7 (+, Ph), 129.05 (+, C7i), 129.00 (+, C8i), 127.3 (+, C5i), 126.8 (+, Ph), 126.0 (+, C6i), 125.7 (o, C4ai), 125.51/125.45 (+, Ph) ppm. IR (ATR): 3055, 3025, 1597, 1558, 1501, 1441, 1403, 1323, 1300, 1221, 1154, 1073, 1029, 948, 839, 812, 753, 718, 695, 617, 583, 555, 531, 478 cm−1. HRMS (ESI): m/z calcd for C45H32N [M + H]+ 586.2530, found 586.2531.
2,3,4,5,6-Pentaphenyl-1-(quinolin-3-yl)benzene (10b).
2,3,4,5,6-Pentaphenyl-1-(quinoline-3-yl)benzene 10b was prepared by Procedure 2 using 3-(phenylethynyl)quinoline 8b (0.458 g, 2.00 mmol) and tetraphenylcyclopentadienone 9 (0.961 g, 2.50 mmol) in benzophenone (10 g) in 50 mL round-bottomed flask in 0.5 h. Yield 0.590 g, 51%, a white solid, m.p. 334 °C. 1H NMR (600 MHz, CDCl3): δ = 8.45 (d, J = 2 Hz, 1H, 2i-H), 7.84 (d, J = 8.4 Hz, 1H, 8i-H), 7.59 (d, J = 2 Hz, 1H, 4i-H), 7.53–7.51 (m, 1H, 7i-H), 7.43 (d, J = 8.0 Hz, 5i-H), 7.35–7.33 (m, 1H, 6i-H), 6.88–6.85 (m, 20H, 2ii-H, 6ii-H, 2iii-H, 6iii-H, 2iv-H, 6iv-H, 2v-H, 6v-H, 2vi-H, 6vi-H, 3ii-H, 5ii-H, 3iii-H, 5iii-H, 3iv-H, 5iv-H, 3v-H, 5v-H, 3vi-H, 5vi-H), 6.83–6.78 (m, 4H, 4ii-H, 4iii-H, 4v-H, 4vi-H), 6.78–6.77 (m, 1H, 4iv-H) ppm. 13C NMR (150 MHz, CDCl3): δ = 152.9 (+, C2i), 145.6 (o, C8ai), 141.4 (o, C4), 141.07 (o, C2, C6), 140.88 (o, C3, C5), 140.44 (o, C1iv), 140.34 (o, C1ii, C1vi), 139.96 (o, C1iii, C1v), 137.8 (+, C4i), 136.51 (o, C1), 134.2 (o, C3i), 131.51/131.48/131.45/131.41/131.37 (+, C2ii, C6ii, C2iii, C6iii, C2iv, C6iv, C2v, C6v, C2vi, C6vi), 129.0 (+, C8i), 128.9 (+, C7i), 127.6 (+, C5i), 127.4/127.0/126.85/126.83/126.78 (+, C3ii, C5ii, C3iii, C5iii, C3iv, C5iv, C3v, C5v, C3vi, C5vi), 127.1 (o, C4ai), 126.2 (+, C6i), 125.87 (+, C4ii, C4vi), 125.54 (+, C4iii, C4v), 125.52 (+, C4iv) ppm. IR (ATR): 3055, 3024, 1662, 1600, 1495, 1440, 1401, 1354, 1311, 1275, 1261, 1127, 1070, 1029, 966, 906, 838, 817, 779, 747, 738, 719, 695, 647, 638, 555, 544, 535, 480, 443, 413 cm−1. HRMS (APCI): m/z calcd for C45H32N [M + H]+ 586.2529, found 586.2530.
2,3,4,5,6-Pentaphenyl-1-(quinolin-4-yl)benzene (10c).
2,3,4,5,6-Pentaphenyl-1-(quinoline-4-yl)benzene 10c was prepared by Procedure 2 using 4-(phenylethynyl)quinoline 8c (0.302 g, 1.319 mmol) and tetraphenylcyclopentadienone 9 (0.430 g, 1.121 mmol, 0.85 equiv.) in benzophenone (10 g) in 50 mL round-bottomed flask in 0.5 h (total conversion of dienone). Yield 0.529 g, 81%, a white solid, m.p. 323 °C. 1H NMR (600 MHz, CDCl3): δ = 8.49 (d, J = 4.4 Hz, 1H, 2-H), 7.86 (dd, J = 0.5, 7.9 Hz, 1H, 5-H), 7.80 (d, J = 8.3, 1H, 8-H), 7.50 (ddd, J = 1.5, 6.9, 8.4 Hz, 1H, 7-H), 7.39 (ddd, J = 1.3, 6.9, 8.3 Hz, 1H, 6-H), 7.05 (d, J = 4.4 Hz, 1H, 3-H), 6.93–6.82 (m, 17H, Ph), 6.77–6.75 (m, 2H, Ph), 6.68–6.64 (m, 4H, Ph), 6.55–6.52 (m, 2H, Ph) ppm. 13C NMR (150 MHz, CDCl3): δ = 148.8 (+, C2), 147.9 (o, C4), 147.7 (o, C8a), 141.5 (o), 140.74 (o), 140.70 (o), 140.4 (o), 140.2 (o), 139.7 (o), 136.0 (o, Cα), 131.53 (+), 131.46 (+), 131.44 (+), 130.9 (+), 130.2 (+), 129.3 (+, C8), 128.8 (+, C7), 128.0 (o, C4a), 127.2 (+, C5), 126.84 (+), 126.81 (+), 126.7 (+), 126.6 (+), 125.8 (+), 125.7 (+, C6), 125.5 (+), 124.4 (+, C3) ppm. IR (ATR): 3052, 3025, 1598, 1496, 1440, 1385, 1291, 1071, 1028, 905, 852, 817, 759, 736, 718, 695, 616, 586, 562, 551, 542, 463, 449, 425 cm−1. HRMS (ESI): m/z calcd for C45H32N [M + H]+ 586.2530, found 586.2522.
3-((4-Ethynylphenyl)ethynyl)quinoline (14).
According to Procedure 1, a solution of 2.080 g (10.00 mmol) of 3-bromoquinoline 6b, 0.070 g (0.10 mmol) of Pd(PPh3)2Cl2, 0.038 g (0.20 mmol) of CuI and 1.197 g (9.50 mmol) of 1,4-diethynylbenzene 13 in 50 mL of anhydrous NEt3 was heated (3.5 h) under reflux temperature. Finally, a purification by column chromatography (petroleum ether
:
ethyl acetate = 3
:
1) gave 3-((4-ethynylphenyl)ethynyl)quinoline 14. Yield 0.889 g, 37%, a brown solid, m.p. 113 °C. 1H NMR (600 MHz, CDCl3): δ = 8.99 (d, J = 2.1 Hz, 1H, 2-H), 8.30 (d, J = 2.1 Hz, 1H, 4-H), 8.10 (d, J = 8.5 Hz, 1H, 8-H), 7.70 (d, J = 8.1 Hz, 1H, 5-H), 7.73 (ddd, J = 1.5, 7.0, 8.5 Hz, 1H, 7-H), 7.57 (ddd, J = 1.5, 7.0, 8.1 Hz, 1H, 6-H), 7.55–7.53 (m, 2H, 2′-H, 6′-H), 7.51–7.49 (m, 2H, 3′-H, 5′-H), 3.20 (s, 1H, CCH) ppm. 13C NMR (150 MHz, CDCl3): δ = 152.1 (+, C2), 147.1 (o, C8a), 138.5 (+, C4), 132.3 (+, C3′, C5′), 131.7 (+, C2′, C6′), 130.4 (+, C7), 129.6 (+, C8), 127.8 (+, C5), 127.5 (+, C6), 127.4 (o, C4a), 123.2 (o, C1′), 122.6 (o, C4′), 117.2 (o, C3), 92.1 (o, Cβ), 88.7 (o, Cα), 83.2 (o, Cγ), 79.4 (o, Cδ) ppm. IR (ATR): 3265, 3060, 3034, 2101, 1969, 1710, 1699, 1602, 1566, 1487, 1404, 1351, 1266, 1145, 1105, 1010, 981, 958, 906, 861, 838, 782, 752, 691, 653, 622, 548, 471, 419 cm−1. HRMS (ESI): m/z calcd for C19H12N [M + H]+ 254.0964, found 254.0972; m/z calcd for C19H11NNa [M + Na]+ 276.0789, found 276.0785.
1-(Quinolin-3-yl)-2,3,4,5,-tetraphenyl-6-(4-(1,2,3,4-tetraphenyl)phenyl)-benzene (15).
1-(Quinolin-3-yl)-2,3,4,5,-tetraphenyl-6-(4-(1,2,3,4-tetraphenyl)phenyl)benzene 15 was prepared by Procedure 2 using 3-((4-ethynylphenyl)ethynyl)quinoline 14 (0.290 g, 1.146 mmol) and tetraphenylcyclopentadienone 9 (1.320 g, 3.438 mmol, 3.0 equiv.) in benzophenone (10 g) in 50 mL round-bottomed flask in 0.5 h. Yield 0.885 g, 77%, a brown solid, m.p. 368 °C (decomp.). 1H NMR (600 MHz, CDCl3): δ = 8.42 (d, J = 2.0 Hz, 1H), 7.90 (d, J = 8.3 Hz, 1H), 7.57 (ddd, J = 1.4, 6.8, 8.3 Hz, 1H), 7.55 (d, J = 2.0 Hz, 1H), 7.46–7.45 (m, 1H), 7.41–7.38 (m, 1H), 7.23 (s, 1H), 7.15–1.13 (m, 2H), 7.07–7.06 (m, 2H), 6.94–6.55 (m, 40H) ppm. 13C NMR (150 MHz, CDCl3): δ = 152.9 (+), 145.7 (o), 141.8 (o), 141.7 (o), 141.3 (o), 141.1 (o), 140.86 (o), 140.82 (o), 140.80 (o), 140.73 (o), 140.48 (o), 140.43 (o), 140.34 (o), 140.27 (o), 140.21 (o), 140.07 (o), 139.95 (o), 139.7 (o), 139.3 (o), 139.1 (o), 138.8 (o), 137.9 (o), 137.8 (+), 136.5 (o), 134.2 (o), 132.5 (+), 131.6 (+), 131.5 (+), 131.39 (+), 131.35 (+), 131.0 (+), 130.9 (+), 130.2 (+), 130.0 (+), 129.1 (+), 128.89 (+), 128.87 (+), 128.77 (+), 128.4 (+), 127.69 (+), 127.62 (+), 127.3 (+), 127.1 (+), 127.0 (+), 126.96 (o), 126.82 (+), 126.76 (+), 126.66 (+), 126.31 (+), 126.2 (+), 125.8 (+), 125.64 (+), 125.55 (+), 125.50 (+), 125.46 (+), 125.36 (+) ppm. IR (ATR): 3024, 1599, 1490, 1441, 1398, 1275, 1071, 1021, 907, 851, 763, 696, 638, 615, 566, 554, 480, 425 cm−1. HRMS (ESI): m/z calcd for C75H51NNa [M + Na]+ 988.3914, found 988.3901.
3,3′-Ethyne-1,2-diyldiquinoline (18a).
According to Procedure 1, a solution of 0.208 g (1.00 mmol) of 3-bromoquinoline 6b, 0.007 g (0.01 mmol) of Pd(PPh3)2Cl2, 0.0038 g (0.02 mmol) CuI and 0.2184 g (1.05 mmol) of 3-ethynylquinoline 17 in 10 mL of anhydrous NEt3 was heated (2 h) under reflux temperature. Finally, a purification by column chromatography (petroleum ether
:
ethyl acetate = 3
:
1) gave 3,3′-ethyne-1,2-diyldiquinoline 18a. Yield 0.196 g, 70%, a brownish solid, m.p. 173 °C. 1H NMR (600 MHz, CDCl3): δ = 9.05 (d, J = 2 Hz, 2H, 2-H, 2′-H), 8.36 (d, J = 2 Hz, 2H, 4-H, 4′-H), 8.12 (d, J = 8.1 Hz, 2H, 8-H, 8′-H), 7.82 (d, J = 8.3 Hz, 2H, 5-H, 5′-H), 7.74 (ddd, J = 1.4, 6.9, 8.4 Hz, 2H, 7-H, 7′-H), 7.58 (ddd, J = 1.1, 6.9, 8.0 Hz, 2H, 6-H, 6′-H) ppm. 13C NMR (150 MHz, CDCl3): δ = 152.0 (+, C2, C2′), 147.2 (o, C8a, C8a′), 138.8 (+, C4, C4′), 130.5 (+, C7, C7′), 129.6 (+, C8, C8′), 127.8 (+, C5, C5′), 127.6 (+, C6, C6′), 127.3 (o, C4a, C4a′), 116.9 (o, C3, C3′), 90.0 (o, Cα) ppm. IR (ATR): 3061, 2169, 1839, 1616, 1565, 1487, 1468, 1413, 1357, 1291, 1226, 1192, 1122, 991, 955, 906, 878, 869, 786, 770, 761, 748, 737, 640, 614, 594, 553, 527, 491, 479, 472, 461, 445, 415 cm−1. MS (ESI): m/z = 281.1 [M + H]+. HRMS (ESI): m/z calcd for C20H13N2 [M + H]+ 281.1074, found 281.1072.
3-(Quinolin-4-ylethynyl)quinoline (18b).
According to Procedure 1, a solution of 0.208 g (1.00 mmol) of 4-bromoquinoline 6c, 0.070 g (0.10 mmol) of Pd(PPh3)2Cl2, 0.038 g (0.20 mmol) CuI and 0.218 g (1.05 mmol) of 3-ethynylquinoline 17 in 10 mL of anhydrous NEt3 was heated (3.5 h) under reflux temperature. Finally, a purification by column chromatography (petroleum ether
:
ethyl acetate = 3
:
1) gave 3-(quinolin-4-ylethynyl)quinoline 18b. Yield 0.160 g, 57%, a yellow solid, m.p. 134 °C. 1H NMR (600 MHz, CDCl3): δ = 9.11 (d, J = 2.1 Hz, 1H, 2-H), 8.94 (d, J = 4.5 Hz, 1H, 2′-H), 8.45 (d, J = 2.0 Hz, 1H, 4-H), 8.40 (dd, J = 0.9, 8.3 Hz, 1H, 5′-H), 8.16 (d, J = 7.8, 1H, 8′-H), 8.14 (d, J = 7.8 Hz, 1H, 8-H), 7.85 (d, J = 7.9 Hz, 1H, 4-H), 7.80–7.76 (m, 2H, 7-H, 7′-H), 7.68 (ddd, J = 1.2, 6.9, 8.2 Hz, 1H, 6′-H), 7.63 (d, J = 4.3 Hz, 1H, 3′-H), 7.61 (ddd, J = 1.2, 7.1, 8.2 Hz, 1H, 6-H) ppm. 13C NMR (150 MHz, CDCl3): δ = 151.9 (+, C2), 149.9 (+, C2′), 148.3 (o, C8a′), 147.5 (o, C8a), 139.2 (+, C4), 130.9 (+, C7), 130.2 (+, C7′), 130.2 (+, C8′), 129.7 (+, C8), 129.1 (o, C4′), 127.9 (+, C5), 127.7 (+, C6), 127.6 (o, C4a′), 127.6 (+, C6′), 127.3 (o, C4a), 126.0 (+, C5′), 123.9 (+, C3′), 116.5 (o, C3), 95.7 (o, Cα), 88.3 (o, Cβ) ppm. IR (ATR): 3033, 2209, 2203, 1615, 1572, 1484, 1419, 1282, 1220, 1192, 1123, 1011, 976, 905, 845, 787, 758, 651, 609, 542, 514, 491, 476, 445 cm−1. MS (ESI): m/z = 281.1 [M + H]+. HRMS (ESI): m/z calcd for C20H13N2 [M + H]+ 281.1074, found 281.1062.
1,2-Di(quinolin-3-yl)-3,4,5,6-tetraphenylbenzene (19a).
According to Procedure 2, benzophenone (5 g) was melted in a 50 mL round-bottomed flask fitted with an air condenser. 3,3′-Ethyne-1,2-diyldiquinoline 18a (0.280 g, 1.00 mmol) and tetraphenyl-cyclopentadienone 9 (0.576 g, 1.50 mmol) were added to the flask, which was heated for 1 h using an open flame. The solution was cooled to rt and toluene (3 mL) was added to prevent the solidification of the benzophenone. After cooling, n-hexane (200 mL) was added, resulting in the precipitation of 19a as a white powder, which was washed with n-hexane and toluene, and collected by vacuum filtration. Yield 0.570 g, 90%, a white solid, m.p. 344 °C. 1H NMR (600 MHz, DMSO-d6), two sets of isochronous rotameric forms (1
:
0.8): δ = 8.52 (d, J = 2.1 Hz, 3.6H, 2-H, 2′-H), 7.97 (d, J = 1.7 Hz, 2H, 4-H), 7.93 (d, J = 1.7 Hz, 1.6H, 4′-H), 7.64–7.61 (m, 3.6H, 8-H, 8′-H), 7.59 (d, J = 7.9 Hz, 2H, 5-H), 7.52 (d, J = 8.3 Hz, 1.6H, 5′-H), 7.50–7.47 (m, 3.6H, 7-H, 7′-H), 7.38–7.36 (m, 2H, 6-H), 7.35–7.32 (m, 1.6H, 6′-H), 7.01–6.74 (m, 36H, Ph) ppm. 13C NMR (150 MHz, DMSO-d6): δ = 152.0 (+, C2), 151.8 (+, C2′), 144.99 (o, C8a′), 144.98 (o, C8), 141.06 (o), 141.04 (o), 140.9 (o), 139.5 (o), 139.29 (o), 139.25 (o), 137.4 (+, C4′), 137.0 (+, C4), 136.95 (o, Cα), 136.93 (o, Cβ), 132.97 (o), 132.94 (o), 130.93 (+), 130.89(+), 130.77 (+), 130.72 (+), 130.70 (+), 129.66 (+), 129.51 (+), 129.18 (+, C7 or C7′), 129.16 (+, C7 or C7′), 128.34 (+, C8 or C8′), 128.31 (+, C8 or C8′), 127.8 (+, C5), 127.6 (+, C5′), 127.0 (+), 126.8 (+), 126.7 (+), 126.5 (+, C6, C6′), 126.3 (o, C4a or C4a′), 126.20 (o, C4a or C4a′), 125.9 (+), 125.7 (+) ppm. IR (ATR): 3055, 3025, 1600, 1552, 1490, 1401, 1314, 1241, 1127, 1071, 966, 907, 812, 784, 747, 696, 632, 536, 476 cm−1. HRMS (ESI): m/z calcd for C48H33N2 [M + H]+ 637.2639, found 637.2637.
1-(Quinolin-3-yl)-2-(quinolin-4-yl)-3,4,5,6-tetraphenylbenzene (19b).
1-(Quinoline-3-yl)-2-(quinoline-4-yl)-3,4,5,6-tetraphenylbenzene 19b was prepared by Procedure 2 using 4-(quinolin-3-ylethynyl)quinoline 18b (0.264 g, 0.943 mmol) and tetraphenylcyclopentadienone 9 (0.543 g, 1.414 mmol) in benzophenone (5 g) in 10 mL round-bottomed flask in 0.5 h. The solution was cooled to rt and toluene (4 mL) was added to prevent the solidification of the benzophenone. Then solvents were evaporated with silica gel and obtained solid was purified by column chromatography with EE
:
PE (1
:
1) as eluents to give 19b. Yield 0.469 g, 78%, a white solid, m.p. 300 °C (decomp.). 1H NMR (600 MHz, DMSO-d6): δ = 8.58 (d, J = 2.2 Hz, 1H), 8.46–8.44 (m, 2H), 8.25 (d, J = 2.2 Hz, 1H), 7.97–7.96 (m, 1H), 7.91–7.87 (m, 2H), 7.61–7.49 (m, 10H), 7.47 (ddd, J = 1.6, 6.8, 8.4 Hz, 2H), 7.45 (ddd, J = 1.5, 6.6, 8.2 Hz, 1H), 7.41–7.39 (m, 2H), 7.36 (ddd, J = 1.2, 6.8, 8.1 Hz, 1H), 7.26–7.24 (m, 1H), 7.21–7.19 (m, 1H), 7.13–7.00 (m, 8H), 6.96–6.81 (m, 22H), 6.79–6.72 (m, 3H), 6.71–6.67 (m, 4H), 6.55 (t, J = 7.6 Hz, 2H) ppm. 13C NMR (150 MHz, DMSO-d6): δ = 151.50 (+), 150.81 (+), 148.76 (+), 148.58 (+), 146.85 (o), 146.78 (o), 145.98 (o), 145.89 (o), 145.00 (o), 144.90 (o), 141.21 (o), 141.18 (o), 141.07 (o), 141.01 (o), 140.86 (o), 140.70 (o), 140.29 (o), 140.20 (o), 139.52 (o), 139.45 (o), 139.17 (o), 139.15 (o), 139.05 (o), 138.94 (o), 136.72 (+), 136.35 (o), 136.29 (o), 135.89 (o), 135.82 (o), 135.61 (+), 132.66 (o), 132.55 (o), 131.11 (+), 130.98 (+), 130.94 (+), 130.90 (+), 130.81 (+), 130.76 (+), 130.71 (+), 130.63 (+), 130.60 (+), 130.58 (+), 130.53 (+), 130.42 (+), 129.36 (+), 129.07 (+), 129.01 (+), 128.95 (+), 128.75 (+), 128.68 (+), 128.21 (+), 127.63 (+), 127.31 (+), 126.93 (+), 126.89 (+), 126.81 (+), 126.76 (+), 126.72 (+), 126.67 (+), 126.63 (+), 126.49 (+), 126.45 (+), 126.40 (+), 126.33 (+), 126.24 (+), 125.89 (+), 125.83 (+), 125.73 (+), 125.65 (+), 124.37 (+), 123.82 (+) ppm. IR (ATR): 3055, 3027, 1699, 1600, 1585, 1567, 1505, 1490, 1463, 1442, 1384, 1359, 1126, 1072, 1027, 965, 908, 854, 814, 761, 696, 617, 533, 480 cm−1. HRMS (ESI): m/z calcd for C48H33N2 [M + H]+ 637.2639, found 637.2629.
General procedure for the synthesis of substituted benzenes (Procedure 3)
2-(Phenylethynyl)quinoline 8a (0.21 g, 0.92 mmol) was dissolved in anhydrous dioxane (4 mL) in an oven dried Schlenk flask under a nitrogen atmosphere and the flask was evacuated and filled with nitrogen repeatedly (3×). Then, Co2(CO)8 (0.015 g) was added to the flask under a nitrogen atmosphere and the flask was evacuated and filled with nitrogen again (3×). The resulting mixture was refluxed for 14 h and dioxane was evaporated. The resulting residue was dissolved in dichloromethane and filtered through a short pad of silica gel. Evaporation of the solvent afforded a dark colored solid which was purified by column chromatography with PE–EE as an eluent.
1,3,5-Triphenyl-2,4,6-tri(quinolin-2-yl)benzene (20a).
Yield 0.021 g, 10%, a white solid, m.p. 337 °C. 1H NMR (600 MHz, DMSO-d6): δ = 7.83 (d, J = 8.5 Hz, 3H, 4′-H), 7.72 (d, J = 8.5 Hz, 3H, 8′-H), 7.67 (d, J = 7.9 Hz, 3H, 5′-H), 7.57 (t, J = 7.3 Hz, 3H, 7′-H), 7.41 (t, J = 7.3 Hz, 3H, 6′-H), 7.22 (d, J = 8.5 Hz, 3H, 3′-H), 7.05 (br s, 6H, 2′′-H, 6′′-H), 6.70 (br s, 6H, 3′′-H, 5′′-H), 6.63 (t, J = 7.3 Hz, 3H, 4′′-H) ppm. 13C NMR (150 MHz, DMSO-d6): δ = 158.9 (o, C2′), 146.4 (o, 8a′), 139.9 (o, C2, C4; C6), 139.8 (o, C1, C3, C5), 138.7 (o, C1′′), 134.1 (+, C4′), 130.6 (+, C2′′, C6′′), 129.0 (+, C7′), 128.6 (+, C8′), 127.4 (+, C5′), 126.3 (+, C3′′, C5′′), 126.1 (+, C6′), 125.8 (+, C4′′), 125.4 (o, C4a′), 124.2 (+, C3′) ppm. IR (ATR): 3058, 3038, 3007, 1617, 1595, 1557, 1501, 1443, 1424, 1332, 1305, 1253, 1223, 1156, 1139, 1113, 1073, 1031, 959, 906, 838, 753, 698, 619, 587, 515, 476, 431, 423, 412 cm−1. MS (ESI): m/z = 688.5 [M + H]+. HRMS (ESI): m/z calcd for C51H34N3 [M + H]+ 688.2745, found 688.2743.
1,2,4-Triphenyl-3,5,6-tri(quinolin-2-yl)benzene (20b).
Yield 0.063 g, 30%, a white solid, m.p. 318 °C. 1H NMR (600 MHz, DMSO-d6): δ = 7.85 (d, J = 7.5 Hz, 1H), 7.76–7.68 (m, 4H), 7.57–7.53 (m, 5H), 7.45–7.42 (m, 3H), 7.32–7.29 (m, 2H), 7.22–7.20 (m, 3H), 7.04–6.99 (m, 6H), 6.83–6.67 (m, 9H) ppm. 13C NMR (150 MHz, DMSO-d6): δ = 159.1 (o), 159.0 (o), 158.8 (o), 146.4 (o), 146.3 (o), 146.2 (o), 140.5 (o), 140.4 (o), 140.2 (o), 139.8 (o), 139.6 (o), 139.3 (o), 139.0 (o), 138.9 (o), 138.6 (o), 134.1 (+), 133.6 (+), 130.8 (+), 130.3 (br, +), 129.0 (+), 128.8 (+), 128.6 (+), 128.3 (+), 127.4 (+), 127.3 (+), 126.6 (+), 126.5 (+), 126.1 (+), 125.92 (+), 125.85 (+), 125.81 (+), 125.7 (+), 125.3 (o), 125.2 (o), 124.3 (+), 124.2 (+) ppm. IR (ATR): 3056, 3023, 1737, 1597, 1559, 1501, 1441, 1424, 1294, 1160, 1142, 1294, 1160, 1142, 1111, 1072, 1029, 942, 849, 835, 818, 751, 722, 699, 606, 531, 514, 479, 423, 417 cm−1. MS (ESI): m/z = 688.3 [M + H]+. HRMS (ESI): m/z calcd for C51H34N3 [M + H]+ 688.2745, found 688.2739.
Hexakis(quinolin-3-yl)benzene (21).
Hexakis(quinoline-3-yl)benzene 11 was prepared by an identical procedure (Procedure 3) using bis (quinoline-3-yl)acetylene 18a (0.295 g, 0.35 mmol) as above. A dark colored solid was purified by column chromatography with EE, then MeOH–CHCl3 (1
:
3) as eluents. Yield 0.295 g, 85%, a white solid, m.p. >370 °C. The compound is insoluble in all NMR solvents which we tested. IR (ATR): 3025, 1619, 1602, 1567, 1489, 1355, 1317, 1260, 1195, 1126, 1110, 1043, 1017, 974, 953, 905, 858, 785, 744, 696, 605, 527, 474, 439 cm−1. HRMS (ESI): m/z calcd for C60H36N6Na [M + Na]+ 863.2899, found 863.2896.
General procedure for the preparation of the salts (Procedure 4)
Samples of 0.50 mmol of the corresponding quinolines were dissolved in toluene containing 1 drop of nitrobenzene. Then 0.75 mmol of dimethyl sulfate was added with stirring. Thereafter the resulting mixture was stirred under reflux temperature. After completion of the reaction (controlled by TLC), the solution was cooled, the crude product was filtered off, washed with ethyl acetate (3 × 10 mL), and dried to afford the product.
2,3,4,5,6-Pentaphenyl-1-(1-methylquinolinium-2-yl)benzene methylsulfate (22a).
According to Procedure 4, a solution of 0.100 g (0.171 mmol) of 2,3,4,5,6-pentaphenyl-1-(quinoline-2-yl)benzene 10a, 1 drop of nitrobenzene and 0.06 mL (0.63 mmol) of dimethyl sulfate in 5 mL of anhydrous toluene was heated for 2 h under reflux temperature to give 2,3,4,5,6-pentaphenyl-1-(1-methylquinolinium-2-yl)benzene methylsulfate 22a. Yield 0.115 g, 95%, a white solid, m.p. 190 °C. 1H NMR (600 MHz, DMSO-d6): δ = 8.88 (d, J = 8.5 Hz, 1H, 4i-H), 8.31 (d, J = 9.0 Hz, 1H, 8i-H), 8.24–8.23 (m, 2H, 3i-H, 5i-H), 8.14 (t, J = 8.0 Hz, 7i-H), 7.93 (t, J = 7.4 Hz, 6i-H), 7.10–6.84 (m, 25H, Ph), 4.41 (s, 3H, NiCH3), 3.38 (s, 3H, CH3SO4) ppm. 13C NMR (150 MHz, DMSO-d6): δ = 159.1 (o, C2i), 144.2 (+, C4i), 143.9 (o, C1), 141.2 (o), 139.1 (o), 138.9 (o), 138.3 (o), 137.5 (o, C8ai), 137.4 (o), 136.0 (+, C7i), 131.7 (+), 130.9 (+), 130.6 (+), 130.4 (+), 130.4 (+, C6i), 130.3 (+, C5i), 130.26 (+), 130.0 (+), 129.3 (+), 126.7 (+), 127.43 (o, C4ai), 127.39 (+, C3i), 127.2 (+), 126.95 (+), 126.91 (+), 126.86 (+), 126.2 (+), 126.0 (+), 119.1 (+, C8i), 52.8 (+, CH3SO4), 42.5 (+, NiCH3) ppm. IR (ATR): 3056, 3023, 1619, 1600, 1577, 1519, 1497, 1442, 1409, 1347, 1252, 1224, 1176, 1152, 1073, 1059, 1013, 918, 849, 817, 770, 756, 723, 698, 644, 608, 576, 554, 532, 428 cm−1. HRMS (ESI): m/z calcd for C46H34N [M]+ 600.2686, found 600.2692.
2,3,4,5,6-Pentaphenyl-1-(1-methylquinolinium-3-yl)benzene methylsulfate (22b).
According to Procedure 4, a solution of 0.100 g (0.171 mmol) of 2,3,4,5,6-pentaphenyl-1-(quinoline-3-yl)benzene 10b, 1 drop of nitrobenzene and 0.06 mL (0.63 mmol) of dimethyl sulfate in 5 mL of anhydrous toluene was heated for 2 h under reflux temperature to give 2,3,4,5,6-pentaphenyl-1-(1-methylquinolinium-3-yl)benzene methyl-sulfate 22b. Yield 0.122 g, 99%, a light green solid, m.p. 321 °C (decomp.). 1H NMR (600 MHz, DMSO-d6): δ = 9.35 (d, J = 1.4 Hz, 1H, 2-H), 8.84 (s, 1H, 4-H), 8.24 (d, J = 8.1 Hz, 1H, 8-H), 8.14 (ddd, J = 1.5, 7.1, 8.8 Hz, 1H, 7-H), 8.06 (dd, J = 1.1, 8.4 Hz, 1H, 5-H), 7.92–7.90 (m, 1H, 6-H), 7.05 (d, J = 7.7 Hz, 2H, Ph), 7.02 (d, J = 7.7 Hz, 2H, Ph), 6.96–6.82 (m, 21H, Ph), 4.34 (s, 3H, NCH3), 3.37 (s, 3H, CH3SO4) ppm. 13C NMR (150 MHz, DMSO-d6): δ = 150.6 (+, C2), 147.5 (+, C4), 142.3 (o), 140.7 (o), 140.5 (o), 139.3 (o), 139.0 (o), 138.4 (o), 135.53 (o, C8a), 135.48 (+, C7), 134.4 (o, C3), 132.8 (o, C1′), 131.0 (+), 130.7 (+), 130.64 (+), 130.60 (+), 130.54 (+), 130.5 (+, C6), 129.9 (+, C5), 127.7 (o, C4a), 127.4 (o), 126.89 (+), 126.87 (+), 126.80 (+), 126.77 (+), 126.4 (+), 125.92 (+), 125.85 (+), 118.9 (+, C8), 52.8 (+, CH3SO4), 44.8 (+, NCH3) ppm. IR (ATR): 3051, 3023, 2961, 1600, 1524, 1496, 1442, 1378, 1254, 1215, 1063, 1012, 760, 697, 618, 577, 558, 441 cm−1. HRMS (ESI): m/z calcd for C46H34N [M]+ 600.2686, found 600.2687.
2,3,4,5,6-Pentaphenyl-1-(1-methylquinolinium-4-yl)benzene methylsulfate (22c).
According to Procedure 4, a solution of 0.154 g (0.263 mmol) of 2,3,4,5,6 pentaphenyl-1-(quinoline-4-yl)benzene 10c, 1 drop of nitrobenzene and 0.06 mL (0.63 mmol) of dimethyl sulfate in 5 mL of anhydrous toluene was heated for 2 h under reflux temperature to give 2,3,4,5,6-pentaphenyl-1-(1-methylquinolinium-4-yl)benzene methylsulfate 22c. Yield 0.185 g, 99%, a brownish solid, m.p. 355 °C (decomp.). 1H NMR (600 MHz, DMSO-d6): δ = 9.14 (d, J = 6.0 Hz, 1H, 2-H), 8.27 (dd, J = 1.0, 8.4 Hz, 1H, 5-H), 8.20 (d, J = 8.9 Hz, 1H, 8-H), 8.15 (d, J = 6.0 Hz, 1H, 3-H), 8.11 (ddd, J = 1.4, 7.1, 8.7 Hz, 1H, 7-H), 7.96–7.94 (m, 1H, 6-H), 7.06 (d, J = 7.7 Hz, 2H, Ph), 7.01 (d, J = 7.7 Hz, 2H, Ph), 6.95–6.83 (m, 14H, Ph), 6.74–6.71 (m, 4H, Ph), 6.60–6.57 (m, 2H, Ph), 4.42 (s, 3H, NCH3), 3.37 (s, 3H, CH3SO4) ppm. 13C NMR (150 MHz, DMSO-d6): δ = 158.5 (o, C4), 147.5 (+, C2), 142.3 (o), 140.6 (o), 139.36 (o), 139.34 (o), 138.9 (o), 138.3 (o), 137.1 (o, C8a), 135.2 (+, C7), 133.1 (o, Cα), 131.0 (+), 130.8 (+), 130.52 (+), 130.49 (+), 129.83 (+, C6), 128.80 (+), 129.3 (+, C5), 128.3 (o, C4a), 126.83 (+), 126.78 (+), 126.74 (+), 126.71 (+), 126.67 (+), 126.4 (+), 125.81 (+), 125.77 (+), 125.3 (+, C3), 118.8 (+, C8), 52.8 (+, CH3SO4), 45.0 (+, NCH3) ppm. IR (ATR): 3051, 3025, 1622, 1615, 1599, 1586, 1577, 1531, 1495, 1441, 1401, 1389, 1375, 1335, 1265, 1216, 1118, 1059, 1015, 869, 820, 766, 726, 695, 609, 576, 553, 542, 464, 430 cm−1. HRMS (ESI): m/z calcd for C46H34N [M]+ 600.2686, found 600.2673.
1-(1-Methylquinolinium-3-yl)-2,3,4,5,-tetraphenyl-6-(4-(1,2,3,4-tetraphenyl)-phenyl)benzene methylsulfate (23).
According to Procedure 4, a solution of 0.481 g (0.5 mmol) of 1-(quinolin-3-yl)-2,3,4,5,-tetraphenyl-6-(4-(1,2,3,4-tetraphenyl)phenyl)benzene 15, 1 drop of nitrobenzene and 0.06 mL (0.63 mmol) of dimethyl sulfate in 5 mL of anhydrous toluene was heated for 2 h under reflux temperature to give 1-(1-methylquinolinium-3-yl)-2,3,4,5,-tetraphenyl-6-(4-(1,2,3,4-tetraphenyl)phenyl)benzene methylsulfate 23. Yield 0.521 g, 96%, a khaki solid, m.p. 222 °C (decomp.). 1H NMR (600 MHz, DMSO-d6): δ = 9.28 (d, J = 1.3 Hz, 1H, Q), 8.73 (s, 1H, Q), 8.35 (d, J = 6.7 Hz, 1H, Q), 8.20 (ddd, J = 1.6, 7.0, 8.7 Hz, 1H, Q), 8.05–8.04 (m, 1H, Q), 7.98–7.95 (m, 1H, Q), 7.26–7.23 (m, 1H, Ph), 7.19–7.13 (m, 4H, Ph), 7.02–6.99 (m, 3H, Ph), 6.95–6.74 (m, 28H, Ph), 6.71–6.64 (m, 5H, Ph), 6.61–6.53 (m, 3H, Ph), 6.49–6.47 (m, 1H, Ph), 4.33 (s, 3H, NCH3), 3.36 (s, 3H, CH3SO4) ppm. 13C NMR (150 MHz, DMSO-d6): δ = 150.6 (+), 147.5 (+), 142.2 (o), 141.5 (o), 140.9 (o), 140.7 (o), 140.6 (o), 140.4 (o), 140.3 (o), 140.0 (o), 139.6 (o), 139.3 (o), 139.01 (o), 139.00 (o), 138.96 (o), 138.8 (o), 138.6 (o), 138.4 (o), 137.3 (o), 136.5 (o), 135.6 (o), 135.5 (+), 134.4 (o), 132.8 (o), 131.0 (+), 130.9 (+), 130.8 (+), 130.7 (+), 130.64 (+), 130.60 (+), 130.55 (+), 130.53 (+), 130.44 (+), 130.36 (+), 130.28 (+), 130.0 (+), 129.4 (+), 128.9 (+), 128.6 (+), 128.4 (+), 128.2 (+), 127.73 (+), 127.66 (+), 127.4 (+), 126.85 (+), 126.81 (+), 126.51 (+), 126.46 (+), 126.4 (+), 125.91 (+), 125.85 (+), 125.8 (+), 125.44 (+), 125.37 (+), 125.31 (+), 118.9 (+), 52.7 (+), 44.8 (+) ppm. IR (ATR): 3055, 3023, 1600, 1524, 1442, 1378, 1249, 1222, 1178, 1157, 1138, 1058, 1008, 911, 852, 797, 766, 697, 565, 496, 432 cm−1. HRMS (ESI): m/z calcd for C76H54N [M]+ 980.4251, found 980.4257.
1-(1-Methylquinolinium-3-yl)-2,3,4,5,-tetraphenyl-6-(4-(1,2,3,4-tetraphenyl)phenyl)benzene hexafluorophosphate (23PF6).
A suspension of 0.050 g (0.046 mmol) of 1-(1-methylquinolinium-3-yl)-2,3,4,5,-tetraphenyl-6-(4-(1,2,3,4-tetraphenyl)phenyl)benzene methylsulfate 23 and 0.008 g (0.049 mmol) of NH4PF6 in 4 mL of water was stirred for 1 day at rt to give 1-(1-methylquinolinium-3-yl)-2,3,4,5,-tetraphenyl-6-(4-(1,2,3,4-tetraphenyl)phenyl)benzene hexafluorophosphate 23PF6. Yield 0.049 g, 95%, a yellow solid, m.p. 220 °C (decomp.). 1H NMR (600 MHz, DMSO-d6): δ = 9.28 (s, 1H, Q), 8.73 (s, 1H, Q), 8.35 (d, J = 8.9 Hz, 1H, Q), 8.20 (t, J = 7.7 Hz, 1H, Q), 8.05 (d, J = 7.9 Hz, 1H, Q), 7.96 (t, J = 7.5 Hz, 1H, Q), 7.26 (m, 1H, Ph), 7.17–7.14 (m, 4H, Ph), 7.02–6.47 (m, 40H, Ph), 4.33 (s, 3H, NCH3) ppm. 13C NMR (150 MHz, DMSO-d6): δ = 150.6 (+), 147.5 (+), 142.2 (o), 141.5 (o), 140.9 (o), 140.7 (o), 140.6 (o), 140.4 (o), 140.3 (o), 140.0 (o), 139.6 (o), 139.3 (o), 139.01 (o), 139.00 (o), 138.96 (o), 138.8 (o), 138.6 (o), 138.4 (o), 137.3 (o), 136.5 (o), 135.6 (o), 135.5 (+), 134.4 (o), 132.8 (o), 131.0 (+), 130.9 (+), 130.8 (+), 130.7 (+), 130.64 (+), 130.60 (+), 130.55 (+), 130.53 (+), 130.44 (+), 130.36 (+), 130.28 (+), 130.0 (+), 129.4 (+), 128.9 (+), 128.6 (+), 128.4 (+), 128.2 (+), 127.73 (+), 127.66 (+), 127.4 (+), 126.85 (+), 126.81 (+), 126.51 (+), 126.46 (+), 126.4 (+), 125.91 (+), 125.85 (+), 125.8 (+), 125.44 (+), 125.37 (+), 125.31 (+), 118.9 (+), 44.8 (+) ppm. IR (ATR): 3055, 3027, 1600, 1521, 1495, 1442, 1379, 1072, 1028, 835, 766, 697, 557, 427 cm−1. HRMS (ESI): m/z calcd for C76H54N [M]+ 980.4251, found 980.4238.
1,2-Di(1-methylquinolinium-3-yl)-3,4,5,6-tetraphenylbenzene dihexafluorophosphate (24a).
A solution of 0.050 g (0.079 mmol) of 1,2-di(quinoline-3-yl)-3,4,5,6-tetraphenylbenzene 19a, 1 drop of nitrobenzene and 0.02 mL (0.21 mmol) of dimethyl sulfate in 5 mL of anhydrous toluene was heated for 3 h under reflux temperature, cooled to rt and extracted with water (3 × 5 mL) and precipitated with excess of NH4PF6 (1.3 equiv.) to give 1,2-di(1-methylquinolinium-3-yl)-3,4,5,6-tetraphenylbenzene dihexafluorophosphate 24a. Yield 0.068 g, 90%, a white solid, m.p. 240 °C. 1H NMR (600 MHz, DMSO-d6), two sets of isochronous rotameric forms (0.9
:
1).: δ = 9.43 (d, J = 1.3 Hz, 0.9H, 2-H), 9.40 (d, J = 1.3 Hz, 1H, 2′-H), 9.05 (s, 1H, 4′-H), 8.88 (s, 0.9H, 4-H), 8.30 (d, J = 8.8 Hz, 1H, 8′-H), 8.27 (d, J = 8.9 Hz, 0.9H, 8′-H), 8.16–8.09 (m, 3.8H, 5-H, 5′-H, 7-H, 7′-H), 7.93–7.90 (m, 1.9H, 6-H, 6′-H), 7.05–6.84 (m, 48H, Ph), 4.38 (s, 6H, N′CH3), 4.32 (s, 5.4H, NCH3) ppm. 13C NMR (150 MHz, DMSO-d6): δ = 150.6 (+, C2), 149.8 (+, C2′), 147.9 (+, C4′), 147.6 (+, C4), 142.8 (o), 142.7 (o), 141.9 (o), 141.8 (o), 138.5 (o), 137.6 (o), 136.5 (o, C8a′), 136.4 (o, C8a), 135.94 (+, C7 or C7′), 135.85 (+, C7 or C7′), 133.10 (o, Cβ), 132.99 (o, Cα), 132.4 (o), 132.2 (o), 130.89 (+), 130.75 (+), 130.69 (+), 130.60 (+), 130.48 (+), 130.44 (+), 130.42 (+), 130.38 (+), 130.33 (+), 130.31 (+), 130.22 (+, C5), 130.07 (+, C5′), 127.92 (o, C4a′), 127.89 (o, C4a), 127.6 (+), 127.0 (+), 126.7 (+), 126.3 (+), 119.00 (+, C8′), 118.90 (+, C8), 45.4 (+, N′CH3), 45.2 (+, NCH3) ppm. IR (ATR): 3057, 3027, 1631, 1602, 1583, 1523, 1497, 1443, 1380, 1356, 1334, 1230, 1174, 1141, 1115, 1073, 1024, 936, 830, 769, 744, 702, 619, 556, 524, 504, 419 cm−1. HRMS (ESI): m/z calcd for C50H38N2 [M]2+ 333.1512, found 333.1518.
1-(1-Methylquinolinium-3-yl)-2-(1-methylquinolinium -4-yl)-3,4,5,6-tetraphenylbenzene dihexafluorophosphate (24b).
A solution of 0.050 g (0.079 mmol) of 1-(quinoline-3-yl)-2-(quinoline-4-yl)-3,4,5,6-tetraphenylbenzene 19b, 1 drop of nitrobenzene and 0.02 mL (0.21 mmol) of dimethyl sulfate in 5 mL of anhydrous toluene was heated for 3 h under reflux temperature, cooled to rt and extracted with water (3 × 5 mL) and then precipitated with excess of NH4PF6 (1.3 equiv.) to give 1-(1-methylquinolinium-3-yl)-2-(1-methylquinolinium-4-yl)-3,4,5,6-tetraphenylbenzene dihexafluorophosphate 24b. Yield 0.067 g, 89%, a yellow solid, m.p. 245 °C (decomp.). 1H NMR (600 MHz, DMSO-d6), two sets of isochronous rotameric forms (24b*/24b# = 1
:
1.27) with close chemical shifts, exact description of the coupling constants is not possible: δ = 9.54# (d, J = 1.3 Hz, 1H, 2-H), 9.23* (d, J = 1.5 Hz, 1H, 2-H), 9.20# (d, J = 6.3 Hz, 1H, 2′-H), 9.18* (d, J = 6.4 Hz, 1H, 2′-H), 9.02* (d, J = 1.5 Hz, 1H, 4-H), 8.81# (s, 1H, 4-H), 8.34# (dd, J = 1.1, 8.6 Hz, 1H), 8.26–8.20 (m, 6H), 8.16# (d, J = 6.3 Hz, 1H, 3′-H), 8.15–8.07 (m, 7H), 8.04–8.01# (m, 2H), 7.92* (ddd, J = 1.2, 6.8, 8.1 Hz, 1H), 7.85–7.79# (m, 2H), 7.21–6.62*# (m, 40H, Ph), 4.37# (s, 3H, N′CH3), 4.346# (s, 3H, NCH3), 4.343* (s, 3H, N′CH3), 4.10* (s, 3H, NCH3) ppm. 13C NMR (150 MHz, DMSO-d6): δ = 155.64*/# (o, C4′), 155.59*/# (o, C4′), 149.53# (+, C2), 149.31* (+, C2), 148.47# (+, C2′), 148.42* (+, C2′), 147.14* (+, C4), 146.81# (+, C4), 142.92 (o), 142.90 (o), 142.89 (o), 142.84 (o), 141.63 (o), 141.49 (o), 140.37 (o), 140.21 (o), 138.56 (o), 138.48 (o), 138.35 (o), 138.31 (o), 137.54 (o), 137.52 (o), 137.48 (o), 137.43 (o), 136.38# (o, C8a), 136.10* (o, C8a), 135.96 (+), 135.88 (+), 135.46 (+), 135.38 (+), 133.36* (o, Cβ), 133.23# (o, Cβ), 132.26 (o), 132.21 (o), 132.05 (o), 131.97 (o), 131.16 (+), 130.88 (+), 130.73 (+), 130.63 (+), 130.61 (+), 130.58 (+), 130.55 (+), 130.51 (+), 130.42 (+), 130.30 (+), 130.26 (+), 130.23 (+), 130.22 (+), 129.71 (+), 129.63 (+), 129.50 (+), 128.60 (+), 128.32 (+), 127.88 (o), 127.71 (o), 127.61 (+), 127.59 (+), 127.44 (+), 127.37 (+), 127.15 (+), 127.07 (+), 127.03 (+), 126.98 (+), 126.93 (+), 126.85 (+), 126.79 (+), 126.64 (+), 126.30 (+), 126.26 (+), 126.24 (+), 125.24 (+), 124.78 (+), 119.34 (+), 119.02 (+), 118.86 (+), 45.37 (+), 45.33 (+), 45.30 (+), 44.67* (+, NCH3) ppm. IR (ATR): 3057, 1619, 1605, 1588, 1529, 1498, 1443, 1234, 1175, 1115, 1073, 1025, 1001, 918, 827, 765, 702, 619, 556, 492, 434 cm−1. HRMS (ESI): m/z calcd for C50H38N2 [M]2+ 333.1512, found 333.1512.
Hexakis(1-methylquinolinium-3-yl)benzene hexakis hexafluorophosphate (25).
According to Procedure 4, a solution of 0.084 g (0.10 mmol) of hexakis(quinoline-3-yl)benzene 21, 1 drop of nitrobenzene and 0.10 mL (1.00 mmol) of dimethyl sulfate in 5 mL of anhydrous toluene was heated for 3 h under reflux temperature. Then the obtained salt was dissolved in water and precipitated with 1.05 equiv. of NH4PF6 to give hexakis(1-methylquinolinium-3-yl)benzene hexakis-hexafluorophosphate 25. Yield 0.171 g, 95%, a white solid, m.p. 195 °C (decomp.). 1H NMR (600 MHz, DMSO-d6): δ = 9.41–8.89 (m, 12H), 8.34–8.16 (m, 18H), 8.00–7.92 (m, 6H), 4.44–4.28 (m, 18H) ppm. 13C NMR (150 MHz, DMSO-d6): δ = 148.7, 148.2, 137.9, 137.8, 137.7, 137.5, 137.0, 131.0, 130.4, 128.6, 128.0, 119.4, 45.97, 45.89, 45.85, 45.79 ppm. IR (ATR): 3084, 1631, 1582, 1524, 1451, 1382, 1228, 1173, 1040, 952, 920, 827, 771, 753, 740, 614, 556, 494, 440, 413 cm−1.
Conflicts of interest
There are no conflicts to declare.
References
- F. J. Schmitz, K. H. Hollenbeak and D. C. Campbell, J. Org. Chem., 1978, 43, 3916 CrossRef CAS.
-
(a) N. Fusetani, N. Asai and S. Matsunaga, Tetrahedron Lett., 1994, 23, 3967 CrossRef;
(b) H. Anan, N. Seki, O. Noshiro, K. Hona, K. Yasumuro, T. Ozasa and N. Fusetani, Tetrahedron, 1996, 52, 10849 CrossRef CAS.
- S. Albrizio, P. Ciminiello, E. Fattorusso, S. Magno and J. R. Pawlik, J. Nat. Prod., 1995, 58, 647 CrossRef CAS.
- C. A. Volk and M. Köck, Org. Lett., 2003, 5, 3567 CrossRef CAS PubMed.
- C. A. Volk and M. Köck, Org. Biomol. Chem., 2004, 2, 1827 RSC.
- R. Laville, O. P. Thomas, F. Berrue, F. Reyes and P. Amade, Eur. J. Org. Chem., 2008, 121 CrossRef CAS.
-
(a) K. A. Smith and A. Streitwieser Jr., J. Org. Chem., 1983, 48, 2629 CrossRef CAS;
(b) K. C. Waterman and A. Streitwieser Jr., J. Am. Chem. Soc., 1984, 106, 3874 CrossRef CAS;
(c) K. A. Smith, K. C. Waterman and A. Streitwieser Jr., J. Org. Chem., 1985, 50, 3360 CrossRef CAS;
(d) K. C. Waterman, D. V. Speer, A. Streitwieser Jr., G. C. Look, K. O. Nguyen and J. G. Stack, J. Org. Chem., 1988, 53, 583 CrossRef CAS;
(e) A. S. Koch, K. C. Waterman, K. Banks and A. Streitwieser, J. Org. Chem., 1990, 55, 6166 CrossRef CAS;
(f) A. S. Feng, D. V. Speer, S. G. DiMagno, M. S. Konings and A. Streitwieser, J. Org. Chem., 1992, 57, 2902 CrossRef CAS.
- A. S. Koch, A. S. Feng, T. A. Hopkins and A. Streitwieser, J. Org. Chem., 1993, 58, 1409 CrossRef CAS.
- F. G. Pühlhofer and R. Weiss, Eur. J. Org. Chem., 2004, 1002 CrossRef.
-
(a) R. Weiss, R. Roth, R. H. Lowack and H. Bremer, Angew. Chem., 1990, 102, 1164 (
Angew. Chem., Int. Ed. Engl.
, 1990
, 29
, 1132
) CrossRef CAS;
(b) R. Weiss, B. Pomrehn, F. Hampel and W. Bauer, Angew. Chem., 1995, 107, 1446 (
Angew. Chem., Int. Ed. Engl.
, 1995
, 34
, 1319
) CrossRef;
(c) R. Weiss, R. May and B. Pomrehn, Angew. Chem., 1996, 108, 1319 (
Angew. Chem., Int. Ed. Engl.
, 1996
, 35
, 1232
) CrossRef;
(d) R. Weiss, S. M. Huber and F. G. Pühlhofer, Eur. J. Org. Chem., 2005, 3530 CrossRef CAS;
(e) R. Weiss, S. M. Huber, F. W. Heinemann, P. Audebert and F. G. Pühlhofer, Angew. Chem., 2006, 118, 8228 (
Angew. Chem. Int. Ed.
, 2006
, 45
, 8059
) CrossRef;
(f) R. Weiss and F. G. Pühlhofer, J. Am. Chem. Soc., 2007, 129, 547 CrossRef CAS PubMed.
-
(a) A. Schmidt, T. Mordhorst and M. Nieger, Synthesis, 2006, 3987 CrossRef CAS;
(b) A. Schmidt, J. C. Namyslo and T. Mordhorst, Tetrahedron, 2006, 62, 6893 CrossRef CAS;
(c) A. Schmidt, T. Mordhorst and M. Nieger, Tetrahedron, 2006, 62, 1667 CrossRef CAS;
(d) A. Schmidt and T. Mordhorst, Synthesis, 2005, 781 CrossRef CAS;
(e) A. Schmidt, J. Heterocycl. Chem, 2002, 39, 949 CrossRef CAS.
-
(a) A. Schmidt and A. Hetzheim, Tetrahedron, 1997, 53, 1295 CrossRef CAS;
(b) A. Schmidt and M. K. Kindermann, J. Org. Chem., 1998, 63, 4636 CrossRef CAS;
(c) A. Schmidt and M. Nieger, J. Chem. Soc., Perkin Trans. 1, 1999, 1325 RSC.
- A. Schmidt and T. Mordhorst, Heterocycles, 2006, 68, 1393 CrossRef CAS.
-
(a) A. Schmidt, T. Mordhorst and M. Nieger, Org. Biomol. Chem., 2005, 3, 3788 RSC;
(b) A. Schmidt, T. Mordhorst and T. Habeck, Org. Lett., 2002, 4, 1375 CrossRef CAS PubMed.
-
(a) S. G. DiMagno, K. C. Waterman, D. V. Speer and A. Streitwieser, J. Am. Chem. Soc., 1991, 113, 4679 CrossRef CAS;
(b) A. S. Koch, A. S. Feng, T. A. Hopkins and A. Streitwieser, J. Org. Chem., 1993, 58, 1409 CrossRef CAS.
-
(a) K. Akiyama, S. Tero-Kubota and Y. Ikegami, J. Am. Chem. Soc., 1983, 105, 3601 CrossRef CAS;
(b) Y. Ikegami, T. Muramatsu and K. Hanaya, J. Am. Chem. Soc., 1989, 111, 5782 CrossRef CAS;
(c) K. Okada, K. Matsumoto, M. Oda, H. Murai, K. Akiyama and Y. Ikegami, Tetrahedron Lett., 1995, 36, 6689 CAS.
- R. Weiss, R. May and B. Pohmrehn, Angew. Chem., 1996, 108, 1319 (
Angew. Chem., Int. Ed. Engl.
, 1996
, 35
, 1232
) CrossRef.
-
(a) W. R. Boon, Chem. Ind., 1965, 782 CAS;
(b) A. L. Black and L. A. Summers, J. Chem. Soc. C, 1969, 610 RSC;
(c) E. C. Campbell, E. E. Glover and G. Trenholm, J. Chem. Soc., 1969, 1987 RSC;
(d) A. D. Dodge, Endeavour, 1970, 111, 130 Search PubMed.
-
(a) C. N. Corder and J. L. Way, J. Med. Chem., 1966, 9, 638 CrossRef CAS PubMed;
(b) Y. Ashani and S. Cohen, J. Med. Chem., 1971, 14, 621 CrossRef CAS PubMed;
(c) C. F. Barfknecht, F. W. Benz and J. P. Long, J. Med. Chem., 1971, 14, 1003 CrossRef CAS PubMed.
- M. Komloova, K. Musilek, A. Horova, O. Holas, V. Dohnal, F. Gunn-Moore and K. Kuca, Bioorg. Med. Chem. Lett., 2011, 21, 2505 CrossRef CAS PubMed.
- J. Hiller, M. Liu and A. Schmidt, Heterocycles, 2017, 94, 821 CrossRef CAS.
- M. Drev, U. Grošelj, B. Ledinek, F. Perdih, J. Svete, B. Štefane and F. Požgan, Org. Lett., 2018, 20, 5268 CrossRef CAS PubMed.
- L. E. Harrington, J. F. Britten, K. Nikitin and M. J. McGlinchey, ChemPlusChem, 2017, 82, 433 CrossRef CAS.
- Y. Geng, A. Fechtenkötter and K. Müllen, J. Mater. Chem., 2001, 11, 1634 RSC.
- K. Kobayashi, A. Sato, S. Sakamoto and K. Yamaguchi, J. Am. Chem. Soc., 2003, 125, 3035 CrossRef CAS PubMed.
- S. Hiraoka, T. Nakamura, M. Shiro and M. Shionoya, J. Am. Chem. Soc., 2010, 132, 13223 CrossRef CAS PubMed.
- Z. Tomović, J. van Dongen, S. J. George, H. Xu, W. Pisula, P. Leclère, M. M. J. Smulders, S. De Feyter, E. W. Meijer and A. P. H. J. Schenning, J. Am. Chem. Soc., 2007, 129, 16190 CrossRef PubMed.
- S. Hiraoka, Y. Hisanaga, M. Shiro and M. Shionoya, Angew. Chem., 2010, 122, 1713 (
Angew. Chem., Int. Ed.
, 2010
, 49
, 1669
) CrossRef.
- B. Traber, J. J. Wolff, F. Rominger, T. Oeser, R. Gleiter, M. Goebel and R. Wortmann, Chem. – Eur. J., 2004, 10, 1227 CrossRef CAS PubMed.
- R. Shukla, S. V. Lindeman and R. Rathore, J. Am. Chem. Soc., 2006, 128, 5328 CrossRef CAS PubMed.
- M. Steeger and C. Lambert, Chem. – Eur. J., 2012, 18, 11937 CrossRef CAS PubMed.
- Y. Tanaka, T. Koike and M. Akita, Chem. Commun., 2010, 46, 4529 RSC.
-
S. H. Shin and N. Y. Sim, Korean Kongkae Taeho Kongbo, KR2016072868A20160624, 2016.
-
(a) D. Gust, J. Am. Chem. Soc., 1977, 99, 6980 CrossRef CAS;
(b) J. C. J. Bart, Acta Crystallogr., Sect. B: Struct. Sci., 1968, 24, 1277 CrossRef CAS;
(c) A. Almenningen, O. Bastiansen and P. N. Skancke, Acta Chem. Scand., 1958, 12, 1215 CrossRef CAS.
- G. Hilt, T. Vogler, W. Hess and F. Galbiati, Chem. Commun., 2005, 11, 1474 RSC.
-
(a) M. Mazik, D. Bläser and R. Boese, Tetrahedron Lett., 2000, 41, 5827 CrossRef CAS;
(b) R. Taylor and O. Kennard, J. Am. Chem. Soc., 1982, 104, 5063 CrossRef CAS;
(c) F. A. Cotton, L. M. Daniels, G. T. Jordan IV and C. A. Murillo, Chem. Commun., 1997, 1673 RSC;
(d)
G. R. Desiraju, Crystal Engineering. The Design of Organic Solids, Elsevier, Amsterdam, 1989; p. 166 Search PubMed.
-
(a) K. Ghosh and A. J. Bhattacharya, Indian J. Chem., Sect. B: Org. Chem. Incl. Med. Chem., 1977, 15, 678 CAS;
(b) P. K. Banerjee, S. R. Samanta and A. J. Bhattacharya, Curr. Sci., 1976, 45, 254 CAS.
- E. Gagnon, T. Maris, P.-M. Arseneault, K. E. Maly and J. D. Wuest, Cryst. Growth Des., 2010, 10, 648 CrossRef CAS.
- E. Gagnon, S. D. Halperin, V. Métivaud, K. E. Maly and J. D. Wuest, J. Org. Chem., 2010, 75, 399 CrossRef CAS PubMed.
- D. Gust and A. Patton, J. Am. Chem. Soc., 1978, 100, 8175 CrossRef CAS.
-
(a) M. A. Ogliaruso, M. G. Romanelli and E. I. Becker, Chem. Rev., 1965, 65, 261 CrossRef CAS;
(b) S. Saito and Y. Yamamoto, Chem. Rev., 2000, 10, 2901 CrossRef.
-
(a) J. H. Magill and A. R. Ubbelohde, Trans. Faraday Soc., 1958, 54, 1811 RSC;
(b) D. J. Plazek and J. H. Magill, J. Chem. Phys., 1966, 45, 3038 CrossRef CAS.
- D. Wasserfallen, G. Mattersteig, V. Enkelmann and K. Müllen, Tetrahedron, 2006, 62, 5417 CrossRef CAS.
- H. D. Katz, J. Org. Chem., 1987, 52, 3932 CrossRef CAS.
-
(a) A. Schmidt, S. Batsyts, A. Smeyanov, T. Freese, E. G. Hübner and M. Nieger, J. Org. Chem., 2016, 81, 4202 CrossRef CAS PubMed;
(b) S. Batsyts, F. J. Ramírez, J. Casado, J. C. Namyslo and A. Schmidt, Z. Naturforsch., 2018, 73, 481 CAS;
(c) A. Dreger, R. Cisneros Camuña, N. Münster, T. A. Rokob, I. Pápai and A. Schmidt, Eur. J. Org. Chem., 2010, 4296 CrossRef CAS;
(d) M. Liu, M. Nieger, E. Hübner and A. Schmidt, Chem. – Eur. J., 2016, 22, 5416 CrossRef CAS PubMed.
- S. Batsyts, R. Vedmid, J. C. Namyslo, M. Nieger and A. Schmidt, Eur. J. Org. Chem., 2019, 1301 CrossRef CAS.
- M. S. El-Shall and K. P. C. Vollhardt, J. Mol. Struct.: THEOCHEM, 1989, 183, 175 CrossRef.
-
A. A. Granovsky, Firefly version 8, http://www.classic.chem.msu.su/gran/firefly/index.html Search PubMed.
- M. W. Schmidt, K. K. Baldridge, J. A. Boatz, S. T. Elbert, M. S. Gordon, J. H. Jensen, S. Koseki, N. Matsunaga, K. A. Nguyen, S. Su, T. L. Windus, M. Dupuis and J. A. Montgomery, J. Comput. Chem., 1993, 14, 1347 CrossRef CAS.
-
E. D. Glendening, J. K. Badenhoop, A. E. Reed, J. E. Carpenter, J. A. Bohmann, C. M. Morales and F. Weinhold, NBO 5.9, Theoretical Chemistry Institute, University of Wisconsin, Madison, WI, 2012 Search PubMed.
- M.-H. Son, J. Y. Kim, E. J. Lim, D.-J. Beak, K. Choi, J. K. Lee, A. N. Pee, S.-J. Min and Y. S. Cho, Bioorg. Med. Chem. Lett., 2013, 23, 1472 CrossRef CAS PubMed.
Footnote |
† Electronic supplementary information (ESI) available: Calculations, NMR spectra. See DOI: 10.1039/c9ob00357f |
|
This journal is © The Royal Society of Chemistry 2019 |