Anthracene–rhodium complexes with metal coordination at the central ring – a new class of catalysts for reductive amination†
Received
15th October 2018
, Accepted 28th November 2018
First published on 29th November 2018
Abstract
A new class of anthracene complexes with a metal coordinated at the central ring was applied in catalysis for the first time. As a result, a simple and efficient protocol for reductive amination that involves CO as a reducing agent has been developed. The rhodium complex [(cyclooctadiene)Rh(C10H4Me2(OMe)4)]+ (1 mol%) catalyses such reactions under mild conditions (40–130 °C) and produces a variety of amines in good yields (74–95%) without affecting the functional groups. The protocol is acceptable for all combinations of aldehydes (aromatic and aliphatic), ketones (aromatic and aliphatic) and amines (aromatic and aliphatic; primary and secondary).
Introduction
Arene complexes of transition metals are widely used in many fields of chemistry. Most of them are air-stable and easily available, which facilitate their application. They have emerged as a perspective class of compounds, for applications in medicine1 and various catalytic processes, providing a new manifold of synthetic options (such as asymmetric catalysis, organic synthesis, polymerization, antitumor agents, etc.).2 In particular, Hintermann and Bolm have used naphthalene complex 1 (Chart 1) for anti-Markovnikov hydration of terminal alkynes to give aldehydes.3 Lacour used it to promote enantioselective Carroll rearrangement.4 Meggers employed ruthenium–pyrene complex 2 for allylcarbamate cleavage in biological environments.5 Wender et al. and Chung et al. utilized rhodium complex 3 to effectively catalyze cycloaddition of alkynes to vinylcyclopropanes or dienes.6 Bringmann used CpRu complex 4 for the synthesis of potential chiral ligands/organocatalysts.7 Metal–arene complexes are widely used in biochemistry as anti-cancer agents (complex 5).8 Complex 1 was very useful for the selective labeling of the tryptophan residue in peptides 6.9
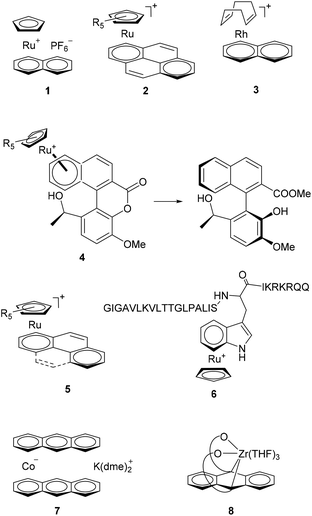 |
| Chart 1 Arene complexes previously used as catalysts, reagents, antitumor agents, and selective labels for peptides. | |
As far as we are aware, the application of anthracene–metal complexes as catalysts is extremely limited so far. Wolf and Jacobi von Wangelin et al. have only recently shown that the bis(anthracene)cobalt anion 7 is a good precursor of active metal species for hydrogenation of alkenes, ketones and imines.10 In 2018 Agapie et al. described zirconium complex 8 with a non-innocent anthracene ligand, which catalyses the co-trimerization of alkynes and nitriles.11
In 2017 we described a general method for the synthesis of metal complexes with anthracenes coordinated at the central ring.12 Herein we report the first investigation of the catalytic activity of this new class of compounds.
Catalytic amination reactions meet the requirements of modern society as a cost- and resource-efficient method for the preparation of pharmaceutically important amines.13 According to statistical analysis,14 reductive amination is one of the top 10 most used reactions in the pharmaceutical industry. Among the reductive agents used for amination, CO15 is potentially the most selective due to the absence of extra hydrogen atoms.16,17 Its high selectivity was indeed demonstrated by comparing its performance to those of NaBH3CN and other common reductants.17a Therefore we decided to study the catalytic activity of our new anthracene complexes in reductive amination in the presence of CO.
Results and discussion
To date, CO-assisted reductive amination has been usually conducted at high temperatures of 120–180 °C.16 There has been only one reported catalyst for such a reaction which works at 90–130 °C.17 Therefore our studies were aimed at the elaboration of milder reaction conditions.
We tested two representatives of a new family of catalysts129 and 10 in comparison with the previously best performing catalysts, namely the cyclobutadiene–rhodium complex [(C4Et4)Rh(p-xylene)]PF6 (11) and Rh2(OAc)4 (Chart 2).16,17 We have used pyrrolidine and morpholine as amines because they are known to add valuable pharmacological properties to the molecules.18 In addition, pyrrolidine and morpholine scaffolds are used for the construction of organocatalysts as well as ligands for highly efficient metal containing catalysts.19
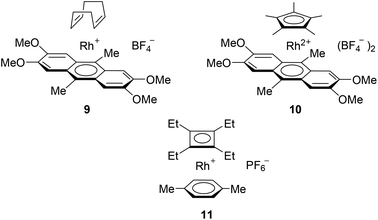 |
| Chart 2 Anthracene and cyclobutadiene complexes of rhodium used in this study. | |
In the model reaction of 2-phenylpropionic aldehyde with pyrrolidine at 40 °C, the catalyst 9 showed the best performance to give the corresponding amine 12 in 47% yield (Table 1, entry 1). The catalyst 10 gave only traces of the product; its poor activity was in accordance with the generally low performance of the Cp*Rh species in CO-mediated reduction processes. The best catalysts reported previously, complex 11 and Rh2(OAc)4, gave low yields of the product 12 (23 and 18% respectively), so further experiments were conducted with the catalyst 9.
Table 1 Optimization of the model reaction
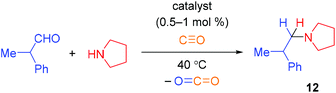
|
Entry |
Catalyst |
Solvent |
Catalyst loading, mol% |
Yield of 12, % |
0.2 mmol of the aldehyde, 0.6 mmol of the amine, 21 hours, 30 bar CO. Variation of conditions: 75 °C. 3 bar of CO. 1.2 equiv. of amine. |
1 |
9
|
t
BuOH |
1 |
47 |
2 |
10
|
t
BuOH |
1 |
Traces |
3 |
11
|
t
BuOH |
1 |
23 |
4 |
Rh2(OAc)4 |
t
BuOH |
1 |
18 |
5a |
9
|
t
BuOH |
1 |
>90 |
6a |
9
|
t
BuOH |
0.5 |
80 |
7a |
9
|
THF |
0.5 |
3 |
8a |
9
|
Et2O |
0.5 |
2 |
9a |
9
|
MeOH |
0.5 |
52 |
10a,b |
9
|
t
BuOH |
0.5 |
43 |
11a,c |
9
|
t
BuOH |
0.5 |
35 |
Increasing the temperature to 75 °C allowed the improvement of the yield of the product to 90% (Table 1). Screening of solvents allowed us to identify tBuOH as the best choice (Table 1, entries 6–9). Decreasing the amine equivalents as well as the pressure of CO and the catalyst loading negatively affected the yield of the product (entries 6, 10 and 11).
The scope of the method was demonstrated by using various combinations of carbonyl and amine components to give the corresponding coupling products in good yields of 60–95% (Scheme 1). Pyrrolidine reacted at 75 °C or even at 40 °C, which are the mildest conditions for CO-assisted reductive amination, to the best of our knowledge. Morpholine typically required harsher conditions (100 °C). Less nucleophilic amines required even higher temperature; the protocol was applied to the synthesis of Ladasten (27). The reaction worked well for aromatic (14, 16–18, 20–26) and aliphatic (12, 19) aldehydes. Noteworthily, the reductive amination of much less reactive ketones, such as 4-phenyl-2-butanone (13) and acetophenone (15), was also possible. Before this work aromatic ketones such as acetophenone were converted into an amine by CO-mediated reductive amination only once.16a Finally, N-methylpiperazine was transformed into derivative 26 at 100 °C in a 86% yield and the benzyloxy group was not affected. The reaction between m-nitrobenzaldehyde and aniline led to the product 30 with a low yield. However, the nitro group was untouched; the rest of the reaction mixture was the corresponding Schiff base. Since such a Schiff base has a good conjugated system with a nitro group, the stability of the crystals increases and the Schiff base precipitates. Fine tuning with the solvent and the concentration is needed. The usage of rhodium acetate is better for such products.16a The activity of rhodium acetate might be lower since the oxidation state is two whereas the complex 9 has rhodium with an oxidation state of one.
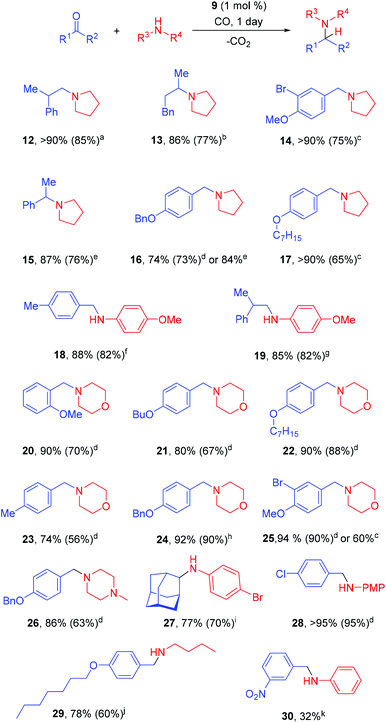 |
| Scheme 1 Scope of aldehydes and ketones in the reaction. NMR yields are given; isolated yields are given in parentheses. 3 eq. of amine were used a 60 °C, 30 bar; b 75 °C, 30 bar; c 75 °C, 50 bar; d 100 °C, 50 bar; e 90 °C, 50 bar; f 90 °C, 30 bar, 1 eq. of amine, 0.5% 9; g 90 °C, 50 bar, 2 eq. of amine; h 90 °C, 50 bar, 48 h; i 120 °C, 50 bar, 2% 9; j 130 °C, 50 bar, 2 eq. of amine. k 100 °C, 50 bar, THF was used as solvent. | |
The reaction mechanism is not yet clear. However, current and previous observations make it possible to propose the catalytic cycle as shown in Scheme 2. The complex 9 can exchange either the cyclooctadiene or anthracene ligand for CO to produce the active species [LmRh(CO)x]+. They can insert into the activated C–OH bond of the hemiaminal intermediate to provide the hydroxo complex A. An intramolecular hydroxylation of the Rh-bound CO then leads to the intermediate B. Its decarboxylation gives the Rh-hydride species C, which upon reductive elimination leads to the amine product and the regenerated catalyst. It should be noted that there are other possible mechanisms which are under discussion in the literature.15a
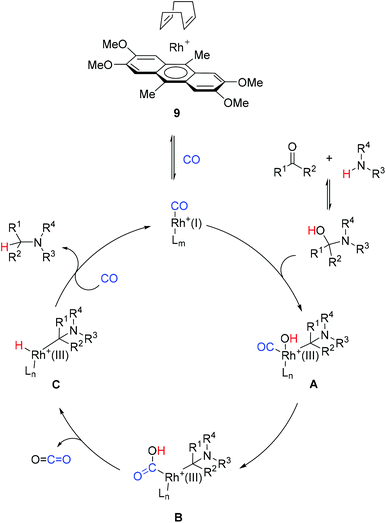 |
| Scheme 2 Plausible mechanism. | |
Conclusions
A new class of anthracene–metal complexes with central ring coordination was tested in catalysis. Rhodium complex 9 with substituted anthracene and cyclooctadiene ligands demonstrated catalytic activity in CO-assisted reductive amination under the mildest conditions (40–130 °C). The protocol is acceptable for all combinations of aldehydes (aromatic and aliphatic), ketones (aromatic and aliphatic) and amines (aromatic and aliphatic; primary and secondary).
Conflicts of interest
There are no conflicts to declare.
Acknowledgements
The work was financially supported by the Russian Science Foundation (grant # 16-13-10393). The contribution of the Center for Molecule Composition Studies of INEOS RAS is gratefully acknowledged.
Notes and references
- For arene complexes as antitumor agents see:
(a) B. S. Murray, M. V. Babak, C. G. Hartinger and P. J. Dyson, Coord. Chem. Rev., 2016, 306, 86–114 CrossRef CAS;
(b) S. Adhikari, O. Hussain, R. M. Phillips, W. Kaminsky and M. R. Kollipara, Appl. Organomet. Chem., 2018, 32(9), 1–13 CrossRef;
(c) A. Weiss, R. H. Berndsen, M. Dubois, C. Müller, R. Schibli, A. W. Griffioen, P. J. Dyson and P. Nowak-Sliwinska, Chem. Sci., 2014, 5, 4742–4748 RSC;
(d) Y. K. Yan, M. Melchart, A. Habtemariam and P. J. Sadler, Chem. Commun., 2005, 4764–4776 RSC.
- For arene complexes in catalysis see:
(a) J. H. Rigby and M. A. Kondratenko, Top. Organomet. Chem., 2004, 7, 181–204 CrossRef CAS. For applications of naphthalene complexes in catalysis see:
(b) L. Li and S. B. Herzon, Nat. Chem., 2013, 1–6 Search PubMed;
(c) D. S. Perekalin, E. E. Karslyan, E. A. Trifonova, A. I. Konovalov, N. L. Loskutova, Y. V. Nelyubina and A. R. Kudinov, Eur. J. Inorg. Chem., 2013, 481–493 CrossRef CAS;
(d) L. Hintermann, L. Xiao, A. Labonne and U. Englert, Organometallics, 2009, 28, 5739–5748 CrossRef CAS;
(e) D. Linder, M. Austeri and J. Lacour, Org. Biomol. Chem., 2009, 7, 4057–4061 RSC. For a review see:
(f) D. S. Perekalin and A. R. Kudinov, Coord. Chem. Rev., 2014, 276, 153–173 CrossRef CAS. For applications of polyarene complexes see:
(g) P. K. Sasmal, S. Carregal-Romero, W. J. Parak and E. Meggers, Organometallics, 2012, 31, 5968–5970 CrossRef CAS;
(h) Y. Hiroi, N. Komine, S. Komiya and M. Hirano, Org. Lett., 2013, 9–12 Search PubMed.
-
(a) A. Labonne, T. Kribber and L. Hintermann, Org. Lett., 2006, 8(25), 5853–5856 CrossRef CAS PubMed;
(b) A. Labonne, L. Zani, L. Hintermann and C. Bolm, J. Org. Chem., 2007, 72(15), 5704–5708 CrossRef CAS PubMed.
-
(a) D. Linder, M. Austeri and J. Lacour, Org. Biomol. Chem., 2009, 7, 4057–4061 RSC;
(b) D. Linder, F. Buron, S. Constant and J. Lacour, Eur. J. Org. Chem., 2008, 5778–5785 CrossRef CAS.
- P. K. Sasmal, S. Carregal-Romero, W. J. Parak and E. Meggers, Organometallics, 2012, 31(16), 5968–5970 CrossRef CAS.
-
(a) P. A. Wender, L. I. Sirois, R. T. Stemmler and T. J. Williams, Org. Lett., 2010, 12, 1604–1607 CrossRef CAS PubMed;
(b) P. A. Wender and T. J. Williams, Angew. Chem., Int. Ed., 2002, 41, 4550–4553 CrossRef CAS;
(c) S.-J. Paik, S. U. Son and Y. K. Chung, Org. Lett., 1999, 1(13), 2045–2047 CrossRef CAS.
-
(a) G. Bringmann, A. Wuzik, R. Stowasser, C. Rummey, L. Göbel, D. Stalke, M. Pfeiffer and W. A. Schenk, Organometallics, 1999, 18(24), 5017–5021 CrossRef CAS;
(b) K. Kamikawa, K. Norimura, M. Furusyo, T. Uno, Y. Sato, A. Konoo, G. Bringmann and M. Uemura, Organometallics, 2003, 22(5), 1038–1046 CrossRef CAS.
-
(a) B. T. Loughrey, B. V. Cunning, P. C. Healy, C. L. Brown, P. G. Parsons and M. L. Williams, Chem. – Asian J., 2012, 7, 112–121 CrossRef CAS PubMed;
(b) D. S. Perekalin, A. P. Molotkov, Y. V. Nelyubina, N. Yu. Anisimova and A. R. Kudinov, Inorg. Chim. Acta, 2014, 409(Part B), 390–393 CrossRef CAS.
- D. S. Perekalin, V. V. Novikov, A. A. Pavlov, I. A. Ivanov, N. Yu. Anisimova, A. N. Kopylov, D. S. Volkov, I. F. Seregina, M. A. Bolshov and A. R. Kudinov, Chem. – Eur. J., 2015, 21(13), 4923–4925 CrossRef CAS PubMed.
- M. Sc. D. Gartner, A. Welther, B. Rezaei Rad, R. Wolf and A. Jacobi von Wangelin, Angew. Chem., Int. Ed., 2014, 53, 3722–3726 CrossRef PubMed.
- C. H. Low, J. N. Rosenberg, M. A. Lopez and T. Agapie, J. Am. Chem. Soc., 2018, 140, 11906–11910 CrossRef CAS PubMed.
- E. E. Karslyan, A. O. Borissova and D. S. Perekalin, Angew. Chem., Int. Ed., 2017, 56, 5584–5587 CrossRef CAS PubMed.
-
(a) N. Mršić, A. J. Minnaard, B. L. Feringa and J. G. de Vries, J. Am. Chem. Soc., 2009, 131, 8358–8359 CrossRef PubMed;
(b) O. V. Zatolochnaya and V. Gevorgyan, Nat. Chem., 2014, 6(8), 661–663 CrossRef CAS PubMed;
(c) A. de la Torre, V. Tona and N. Maulide, Angew. Chem., Int. Ed., 2017, 56, 12416–12423 CrossRef CAS PubMed;
(d) V. Tona, A. de la Torre, M. Padmanaban, S. Ruider, L. González and N. Maulide, J. Am. Chem. Soc., 2016, 138(27), 8348–8351 CrossRef CAS PubMed.
- S. D. Roughley and A. M. Jordan, J. Med. Chem., 2011, 54, 3451–3479 CrossRef CAS PubMed.
- For the state-of-the-art of CO-related processes see reviews:
(a) A. Ambrosi and S. E. Denmark, Angew. Chem., Int. Ed., 2016, 55, 2–28 CrossRef PubMed;
(b) A. A. Tsygankov, M. Makarova and D. Chusov, Mendeleev Commun., 2018, 28, 113–122 CrossRef CAS;
(c) F. Ragaini, Dalton Trans., 2009, 6251–6266 RSC; and recent examples:
(d) S. E. Denmark, Z. D. Matesich, S. T. Nguyen and S. M. Sephton, J. Org. Chem., 2018, 83, 23–48 CrossRef CAS PubMed;
(e) P. Zhou, C. Yu, L. Jiang, K. Lv and Z. Zhang, J. Catal., 2017, 352, 264–273 CrossRef CAS;
(f) S. E. Denmark, M. Y. S. Ibrahim and A. Ambrosi, ACS Catal., 2017, 7, 613–630 CrossRef CAS;
(g) S. E. Denmark and Z. D. Matesich, J. Org. Chem., 2014, 79, 5970–5986 CrossRef CAS PubMed;
(h) J. W. Park and Y. K. Chung, ACS Catal., 2015, 5, 4846–4850 CrossRef CAS;
(i) F. Ferretti, M. A. EL-Atawy, S. Muto, M. Hagar, E. Gallo and F. Ragaini, Eur. J. Org. Chem., 2015, 5712–5715 CrossRef CAS.
-
(a) D. Chusov and B. List, Angew. Chem., Int. Ed., 2014, 53, 5199–5201 CAS;
(b) A. A. Tsygankov, M.-S. Chun, A. D. Samoylova, S. Kwon, Y. M. Kreschenova, S. Kim, E. Shin, J. Oh, T. V. Strelkova, V. S. Kolesov, F. I. Zubkov, S. E. Semenov, I. V. Fedyanin and D. Chusov, Synlett, 2017, 28(05), 615–619 CAS;
(c) A. P. Moskovets, D. L. Usanov, O. I. Afanasyev, V. A. Fastovskiy, A. P. Molotkov, K. M. Muratov, G. L. Denisov, S. S. Zlotskii, A. F. Smol'yakov, D. A. Loginov and D. Chusov, Org. Biomol. Chem., 2017, 15, 6384–6387 RSC.
-
(a) O. I. Afanasyev, A. A. Tsygankov, D. L. Usanov, D. S. Perekalin, N. V. Shvydkiy, V. I. Maleev, A. R. Kudinov and D. Chusov, ACS Catal., 2016, 6, 2043–2046 CrossRef CAS;
(b) O. I. Afanasyev, A. A. Tsygankov, D. L. Usanov, D. S. Perekalin, A. D. Samoylova and D. Chusov, Synthesis, 2017, 49, 2640–2651 CrossRef CAS.
- For some recent applications of pyrrolidines see:
(a) C. Bello, J. Bai, B. K. Zambron, P. E. Rodríguez, C. Gajate, I. Robina, I. Caffa, M. Cea, F. Montecucco, A. Nencioni, A. Nahimana, D. Aubry, C. Breton, M. A. Duchosal, F. Mollinedo and P. Vogel, Eur. J. Med. Chem., 2018, 150, 457–478 CrossRef CAS PubMed;
(b) A. Ayati, R. Esmaeili, S. Moghimi, T. O. Bakhshaiesh, Z. Eslami-S, K. Majidzadeh-A, M. Safavi, S. Emami and A. Foroumadi, Eur. J. Med. Chem., 2018, 145, 404–412 CrossRef CAS PubMed; and a review:
(c) D. O'Hagan, Nat. Prod. Rep., 2000, 17, 435–446 RSC. For some recent applications of morpholines see:
(d) S.-K. Xiang, B. Zhang, L.-H. Zhang, Y. Cui and N. Jiao, Chem. Commun., 2011, 47, 8097–8099 RSC;
(e) B. T. Hahn, R. Fröhlich, K. Harms and F. Glorius, Angew. Chem., Int. Ed., 2008, 47, 9985–9988 CrossRef CAS PubMed.
-
(a) J. Beament, M. F. Mahon, A. Buchard and M. D. Jones, Organometallics, 2018, 37, 1719–1724 CrossRef CAS;
(b) V. Poirier, T. Roisnel, J.-F. Carpentier and Y. Sarazin, Dalton Trans., 2011, 40, 523–534 RSC.
Footnote |
† Electronic supplementary information (ESI) available. See DOI: 10.1039/c8ob02561d |
|
This journal is © The Royal Society of Chemistry 2019 |
Click here to see how this site uses Cookies. View our privacy policy here.