DOI:
10.1039/C9MO00061E
(Research Article)
Mol. Omics, 2019,
15, 331-339
Reduced sialyl-Lewisx on salivary MUC7 from patients with burning mouth syndrome†
Received
26th March 2019
, Accepted 16th July 2019
First published on 16th July 2019
Abstract
We analysed and compared MUC7 O-glycosylation and inflammatory biomarkers in saliva from female patients with burning mouth syndrome (BMS) and gender/age-matched controls. Oligosaccharides from salivary MUC7 from BMS and controls were released. Inflammatory mediators were measured by multiplex proximity extension assay. Presence of sialyl-Lewisx (Si-Lex) epitope on MUC7 was confirmed using Western blot. MUC7 O-glycans and measured inflammatory biomarkers were found to be similar between BMS and controls. However, oligosaccharides sialyl-Lewisx (Si-Lex) was found to be reduced in samples from BMS patients. Positive correlation (combined patients and controls) was found between levels of C–C motif chemokine 19 (CCL-19) and the amount of core-2 oligosaccharides on MUC7 as well as fractalkine (CX3CL1) and level of sialylation. Patients with BMS were shown to represent a heterogeneous group in terms of inflammatory biomarkers. This indicates that BMS patients could be further stratified on the basis of low-level inflammation. The results furthermore indicate that reduced sialylation of MUC7, particularly Si-Lex, may be an important feature in patients with BMS. However, the functional aspects and potential involvement in immune regulation of Si-Lex remains unclear. Our data suggests a chemokine driven alteration of MUC7 glycosylation.
Introduction
Burning mouth syndrome (BMS) is a condition characterised by chronic oral mucosal pain without any visible mucosal changes in the oral cavity.1 In addition to an oral burning sensation in the mucosa, patients with BMS also experience subjective dry mouth feelings accompanied by taste abnormalities.2,3 Females are more commonly affected by BMS than males and the prevalence of BMS increases with age.4,5 Postmenopausal women from 50–89 are reported to have the highest BMS incidence.4 Currently there are no objective diagnostic criteria for BMS.
Saliva is a complex biological secretion containing components from major and minor salivary glands that is of significance in oral and systemic diseases,6,7 and its constituents is known to vary with age.8 Aging also leads to a low-grade inflammatory chronic condition known as inflammaging or immunosenescence.9 Inflammaging is known to have consequences for diseases within the elderly population. Aging changes the immune system10 and different individuals age in a different rate, leading to that the biological age of a person may be different from the chronological age.11
Salivary glycoproteins such as mucins are the major components in saliva. Mucins aid in the maintenance of physical and functional integrity of normal mucosa.12 MUC5B and MUC7 are two highly glycosylated mucins found in saliva that help in moistening and lubrication.13 Salivary O-linked glycosylation is an important post-translational process, whose role in addressing different diseases and disorders such as Sjögren's syndrome,14 dry mouth condition15 and recurrent aphthous stomatitis,16 have been studied. The role of salivary O-linked glycosylation in BMS is unexplored. Given the complex nature and the diversity of oral mucosal symptoms observed in patients with BMS, qualitative analysis of salivary O-linked oligosaccharides is a potential pathway to discover connections to the disease etiology and pathology. The aim of this study was to identify changes of MUC7 O-linked glycosylation between BMS patients and controls, and to compare the level of inflammatory mediators in patients with BMS with the controls.
Experimental
Patients and ethical approval
The study was approved by the Regional ethical review board in Gothenburg (Dnr. 36. 8–10), and followed the guidelines of the Helsinki declaration. Informed consent was obtained from all the participants. Patients with BMS were diagnosed according to the International Headache society criteria.17 The controls included in the study were clinically examined and did not report any burning mouth sensation in the oral mucosa. Complete blood count for the presence of haematological deficiencies and microbiological examination for the presence of opportunistic microorganisms in higher numbers were carried out for all BMS patients and controls, in order to exclude anyone suffering from these conditions, which could mimic the BMS symptoms. The clinical characteristics of patients with BMS and the controls are presented in Table 1.
Table 1 Clinical characteristics of patients with BMS and controls used in PEA and glycosylation analysis
BMS patients |
Controls |
No. |
Age (years) |
Blood group |
SWS (mL min−1) |
Xerostomia (yes/no) |
Total no. of drugs |
No. |
Age (years) |
Blood group |
SWS (mL min−1) |
Xerostomia (yes/no) |
Total no. of drugs |
Samples included for MUC7 O-glycosylation.
Samples used for both glycosylation and PEA assay.
Samples included in PEA assay only.
|
1a |
54 |
O Rh+ |
0.4 |
Yes |
7 |
1b |
67 |
O Rh+ |
2.50 |
Yes |
4 |
2a |
76 |
O Rh+ |
1.60 |
Yes |
4 |
2b |
85 |
O Rh+ |
2.50 |
No |
2 |
3a |
54 |
B Rh+ |
2 |
Yes |
8 |
3b |
68 |
A Rh+ |
1.67 |
No |
1 |
4b |
66 |
O Rh+ |
1.60 |
No |
6 |
4b |
58 |
A Rh+ |
1.25 |
No |
1 |
5b |
70 |
A Rh+ |
1.20 |
Yes |
6 |
5b |
68 |
A Rh+ |
2.0 |
No |
2 |
6b |
73 |
A Rh+ |
2.30 |
No |
1 |
6b |
66 |
A Rh+ |
1.8 |
No |
0 |
7b |
84 |
A Rh+ |
0.80 |
Yes |
0 |
7c |
52 |
O Rh+ |
2.0 |
No |
0 |
8b |
69 |
A Rh+ |
2.30 |
Yes |
0 |
|
|
|
|
|
|
9b |
54 |
O Rh+ |
2.10 |
Yes |
4 |
|
|
|
|
|
|
10c |
80 |
B Rh+ |
0.20 |
Yes |
7 |
|
|
|
|
|
|
ELISA
Stimulated whole saliva (SWS) was collected from the patients with BMS and the controls for the duration of five minutes, the total volume was measured, and the flow rate (mL min−1) was calculated. An enzyme-linked immunosorbent assay (ELISA) was performed to determine the concentration (pg mL−1) of interleukin 8 (IL-8), vascular endothelial growth factor (VEGF), and epidermal growth factor (EGF) in the saliva of patients with BMS, and the controls according to the manufacturers' instructions (R&D systems, Minneapolis, MN). The total secretory outputs (pg min−1) of IL-8, EGF and VEGF were calculated from the SWS flow rate (mL min−1) and the measured concentration of IL-8, EGF or VEGF (pg mL−1). Concentration of salivary immunoglobulin A (IgA) in patients with BMS and the controls was determined by sandwich ELISA as described previously by Krzywkowskiet et al.18
Multiplex proximity extension assay
Multiplex proximity extension assays (PEA) technology was utilised to measure inflammatory mediators in saliva from BMS patients (n = 7) and controls (n = 6) using the Inflammation panel (Olink Proteomics, Uppsala, Sweden), consisting of 92 inflammatory-related biomarkers and four controls.19 Briefly, one μL sample is mixed with 3 μL incubation mix containing a set of probe pairs, each consisting of an antibody conjugated to single-stranded DNA oligonucleotide. Upon the target recognition, when a probe pair is in proximity, the DNA oligonucleotides are allowed to hybridized to each other and subsequently extended by enzymatic polymerization. The resulted double-stranded DNA molecules are pre-amplified using a pair of universal primers before each specific DNA reporter molecule, representing a target protein, is amplified, decoded and quantified by a specific pair of primers in a 96 Dynamic Array Integrated Fluidic Circuit on a BioMark HD system (Fluidgm CA, USA). The assay includes internal controls, incubation controls (non-human antigens), extension control (a molecule with both DNA oligonucleotides conjugated to one antibody), and amplification control (a double stranded DNA molecule amplicon) allowing monitoring of each step in the assay. A triplicate negative control was used to define the limit of detection for each protein. In the current format, the multiplex PEA technology, is a relative quantification method, the values are expressed in normalized protein expression (NPX), an arbitrary unit on log2 scale.
Release and analysis of oral mucin oligosaccharides
Stimulated saliva samples from BMS (n = 9) and control women (n = 6) were collected, centrifuged at 1700 × g for 7 min and the supernatants were stored at −80 °C until further analysis. MUC7 was isolated from saliva using sodium dodecyl sulfate-agarose/polyacrylamide composite gel electrophoresis (SDS-AgPAGE) or SDS-PAGE. After electrophoresis, MUC5B and MUC7 were blotted onto polyvinyl difluoride (PVDF) membranes and stained with alcian blue. MUC7 glycans were released from membranes by reductive β-elimination,20 and subjected to liquid chromatography mass spectrometry (LC-MS/MS). The experimental parameters for the analysis of MUC7 glycans is presented in ESI,† Table S1. Glycans were annotated from their MS/MS spectra manually and further validated by available structures stored in UniCarb-DR database (http://unicarb-dr.org). Their abundances were calculated as the intensity of individual glycan to the sum of the total glycan MS intensity.
Western blot analyses
Stimulated whole saliva samples were separated by electrophoresis in 4–12% gradient NuPAGE Bis–Tris (Novex, ThermoFisher, Waltham, MA) gel, blotted onto PVDF membranes and blocked with TBS-T buffer (Tris-buffered saline, pH 7.4, with 0.01% Tween 20) containing 1% BSA. The membranes were incubated for 1 h at room temperature with mouse anti-Si-Lex (CD15s, BD Biosciences, San Jose, CA) (1
:
1000). The presence of MUC7 was confirmed by the use of polyclonal antiserum LUM7-1 (kindly provided by Dr Claes Wickström, Lund University) diluted 1
:
500 and incubated for 1 h. The membranes were subsequently treated with horseradish peroxidase (HRP)-conjugated goat anti-rabbit IgG (1
:
2000) (Fc-specific, Merck, Kenilworth, NJ) and rabbit anti-mouse immunoglobulins (1
:
2000) (Dako Cytomation, Glostrup, Denmark), respectively. The membranes were washed subsequently and visualized by SuperSignal West Femto maximum sensitivity substrate (Thermo Scientific).
Statistical analysis
Statistical analysis was performed using Prism (version 7.0) software (GraphPad, San Diego, CA.). Non-parametric Mann–Whitney U test was performed to investigate significant differences between individual glycans, the overall glycosylation matrix and the proteins measured by PEA assay. The differences between BMS patients and controls for the levels of proteins and different growth factors measured using ELISA were calculated by unpaired two-tailed Student's t-test. For correlation analysis, Spearman correlation coefficient was used. A p-value <0.05 was regarded as being statistically significant.
Results
Concentration versus secretory output of proteins in BMS
Since xerostomia (the subjective experience of dry mouth) is a co-morbidity of BMS, we wanted to investigate if secretion rate of proteins in saliva rather than concentration of saliva was a constituting factor in biomarker discovery for BMS. We have previously found that BMS patients more often experience hyposalivation due to very low stimulated whole saliva (SWS) secretion rate.21 We selected growth factors (EGF and VEGF), pro-inflammatory cytokine IL-8 and sIgA in whole saliva as well as total protein, and corrected for secretion rate (concentration time salivary flow rate) (Fig. 1). We could not find statistical significance, neither for concentration nor secretory output between BMS patients and controls for any of the measured parameters, suggesting that secretion rate was not an important factor in biomarker discovery in BMS. The descriptive statistics for the cytokines and growth factors are presented in ESI,† Table S2.
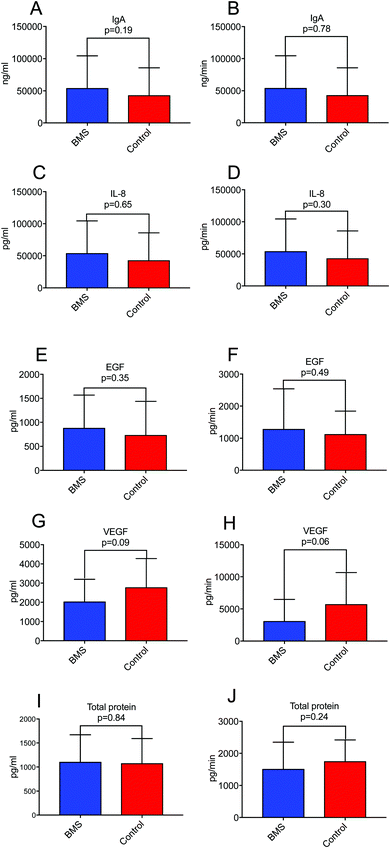 |
| Fig. 1 A representative figure of salivary levels of growth factors, total protein and cytokines in BMS and controls detected by ELISA. Columns on left represent concentration (mean ± SD) and on right represent secretory output of (A and B) IgA; (C and D) IL-8; (E and F) EGF; (G and H) VEGF, and (I and J) Total protein. | |
Inflammatory markers and BMS
Cytokines are known to work in networks, and it is therefore important to measure multiple cytokines in a single sample.22 We expanded our investigation of inflammatory mediators in saliva and their involvement in BMS by adopting an array used for plasma samples. For this analysis we selected only a limited number of patients (n = 8) with age- and gender-matched controls (n = 8). Out of 92 different inflammatory mediators analysed, 57 were detectable in the saliva samples (ESI†). Fig. 2A illustrates representative examples of inflammatory mediators, Interleukin 7 (IL-7), Cystatin-5, Fibroblast growth factor 19 (FGF-19), C–C motif chemokine 19 (CCL-19) tested with a p ≤ 0.1. These inflammatory biomarkers were close to being significantly elevated in BMS saliva. This suggested that low-level inflammation, as has been proposed in aging and immunosenescence, could play a role in BMS. It should be stressed that statistical analyses based on the complete panel of inflammatory biomarkers did not reveal any differences between the BMS patients and controls. However, one interesting finding was the increased heterogeneity in the level of each of the measured inflammatory biomarker comparing the BMS patients with the controls (Fig. 2B). These data suggested that BMS patients are a heterogeneous cohort, where stratification based on the level of inflammatory markers may generate more homogenous patient populations, despite the fact that the levels are low and not significantly distinguished from those in the control samples.
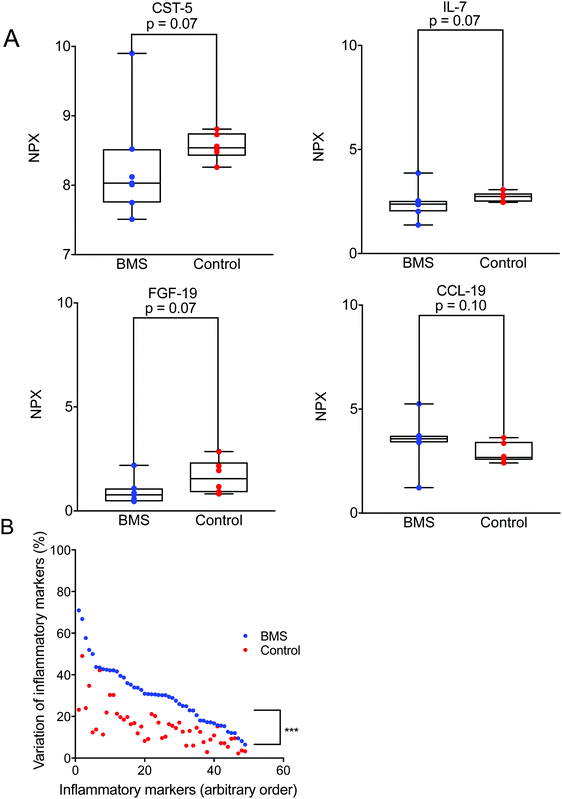 |
| Fig. 2 (A) A representative figure of inflammatory biomarkers such as Cystatin-5, (CST-5), Interleukin-7 (IL-7), Fibroblast Growth Factor-19 (FGF-19), and C–C motif chemokine 19 (CCL-19) comparison between BMS (N = 7) and controls (N = 6) detected by PEA. The X-axis corresponds to the normalized protein expression values denoted as NPX. (B) Plot showing variation of inflammatory markers between BMS and the controls organized by highest to lowest variation of the BMS patients. The level of significance of higher heterogeneity in BMS of the level of all measure biomarker is denoted by p < 0.001. | |
Same glycans are found on both patients and control MUC7
Since inflammation has been reported to influence glycosylation,23 the role of BMS and oral O-linked mucin oligosaccharides was investigated, specifically targeting MUC7. Separation of saliva using SDS-AgPAGE gel, revealed two clearly visible bands corresponding to the molecular masses of MUC5B (∼1 MDa) and MUC7 (∼150 kDa) in both BMS patients and control samples as shown in Fig. 3A. Following separation, individual MUC7 bands were excised and further processed for the LC-MS/MS analysis of O-linked glycans. The annotation of the spectra led to the identification of 53 peaks, showing the complexity of these O-glycans. A representative LC-MS profile of BMS is depicted in Fig. 3D. The list of O-glycans identified by LC-MS/MS is presented in ESI,† Table S3. The majority of the glycans detected in MUC7 has already been described.20 The detected glycans were based on core 1 (Galβ1-3GalNAc-ol) and core 2 (GlcNAcβ1-6(Galβ1-3) GalNAc-ol) both in BMS patients and control samples. The core 1 structures were found in all the subjects and were mostly terminating with one or two sialic acids. The core 2 glycans were found to be bi- and tri-antennary, and in addition to termination with sialic acid (sialic acid type 2) terminating epitopes also included fucose making up the structure Si-Lex. The presence of Si-Lex on MUC7 and epitope was confirmed by Western blot analysis in both controls and patients (Fig. 3B). A matrix to overview the LC-MS glycosylation based on the relative mass spectrometry abundance was applied to investigate an average composition of detected oligosaccharides (Fig. 3C). This data showed that there was no difference in the type of glycosylation in BMS and control females providing a reliable dataset for further investigation of specific quantitative aspects of MUC7 oligosaccharides.
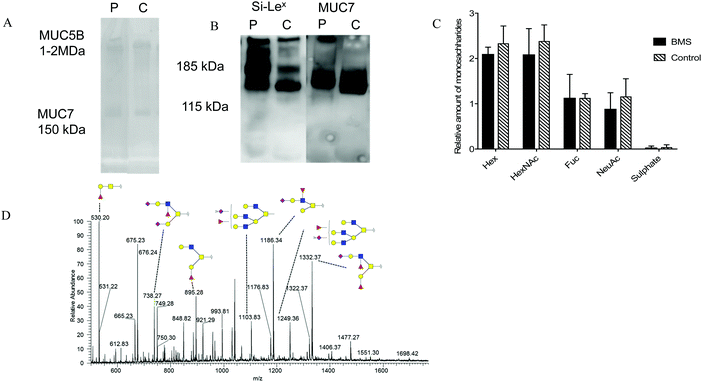 |
| Fig. 3 A representative figure showing salivary mucins and MUC7 glycosylation in patients with BMS. (A) Alcian blue stained PVDF membranes of salivary mucins detected using Ag-PAGE gel electrophoresis from representative BMS patient and control (B) MUC7 and Si-Lex epitope detected in patient and control using Western blot analysis run in a SDS-PAGE 4-12% Bis–Tris gel. (C) Monosaccharide comparison between BMS (n = 9) and controls (n = 6) based upon the relative mass spectrometer intensities showing no significant difference in the overall glycosylation (D) Liquid Chromatography Mass Spectrometry (LC-MS) and annotated major oligosaccharides identified on MUC7 from a BMS patient. Structures identified by LC-MS/MS were annotated using the Symbol Nomenclature for Glycans (SNFG). Here, Hex corresponds to the hexose i.e. galactose in this case. HexNAc could be GalNAc or GlcNAc (N-acetylgalactosamine or N-acetylglucosamine). NeuAc is corresponding to sialic acid and Fuc to fucose. | |
Lowered sialylation of core 2 structures in BMS
Since the overview matrix showed no major difference in the glycosylation between BMS patients and controls, quantitative statistical analysis of individual oligosaccharides was carried out. This analysis, based on the relative intensity of individual glycans, showed a trend of decrease in sialylation in general for the BMS patients. In particular, sialylated and fucosylated structures with [M − H] ions of m/z 11861− (corresponding to NeuNAc1Hex2HexNAc2Fuc1), (Fig. 4A), [M − 2H]2− ions of m/z 12492− (Hex4HexNAc4Fuc1NeuAc3), (Fig. 4B) and with [M − 2H]2− ions of m/z 11032− (Hex4HexNAc4Fuc1NeuAc2) (Fig. 4C), all of them containing Si-Lex epitopes, were significantly reduced in patients suffering from BMS. Combining the level based on the signal of the [M − nH]n- ions of all the Si-Lex containing oligosaccharides confirmed the result of a relative lower amount of Si-Lex in BMS patients (Fig. 4D).
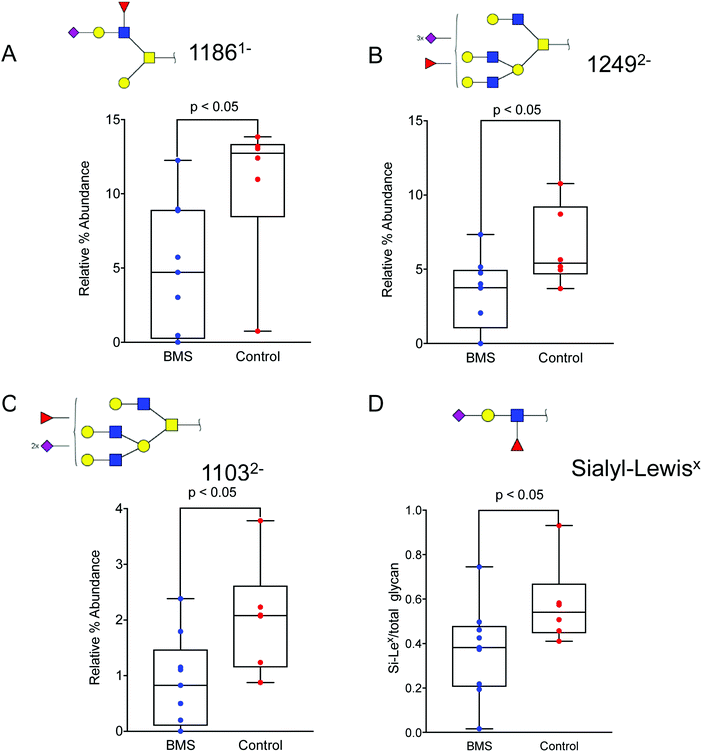 |
| Fig. 4 Altered oligosaccharide in patients with BMS and controls. Sialylated and fucosylated structures with (A) [M − H] ions of m/z 11861− (corresponding to NeuNAc1Hex2HexNAc2Fuc1), (B), with [M − 2H]2- ions of m/z 12492− (Hex4HexNAc4Fuc1NeuAc3), and (C) with [M − 2H]2− ions of m/z 11032− (Hex4HexNAc4Fuc1NeuAc2) were significantly reduced in patients suffering from BMS. All of the above oligosaccharides contain a specific epitope, Si-Lex. (D) The total amount of Si-Lex was also found to be reduced in BMS patients. The glycan structures are denoted using SNFG notation (Fig. 3). The data is analysed using box plots using a non-parametric Mann Whitney U test. The level of significance corresponding to statistically significance is shown using p value <0.05. | |
Inflammatory markers correlate with core 2 and sialylation of MUC7
Little is known about the association between inflammation and glycosylation. We hypothesized that one pathway could be that extracellular signaling of inflammatory mediators via their cellular receptors can trigger intracellular signaling leading to altered transcription of proteins/enzymes involved in the glycosylation machinery. If this is true, the levels of some of the analysed inflammatory mediators from the panel found in saliva combining both BMS patients and controls could correlate with features of the glycosylation. We tested this hypothesis by calculating the correlation between individual MUC7 oligosaccharides from LC-MS as well as combined features (relative amount of monosaccharides (Fig. 2C) and the amount of core-1 and core-2) with the signal from the inflammatory panel. Extensive evaluation of the correlation only showed significant correlation between the total amount of core-2 oligosaccharides and the level of C–C motif chemokine 19 (CCL-19). We also found a correlation between the level of sialylation of MUC7 and fractalkine (CX3CL1) (Fig. 5). These glycosylation features, core 2 and sialylation are related to the biosynthesis of the Si-Lex containing structures, which we identified here to be significantly down in BMS patients. CCL-19 was also one of the chemokines that was close to being significant for BMS as mentioned above. The connection between increased Si-Lex and the correlation with CX3CL1 with sialylation that is part of the Si-Lex epitope is more complex, since the trend was that this chemokine was lower in BMS (not significant).
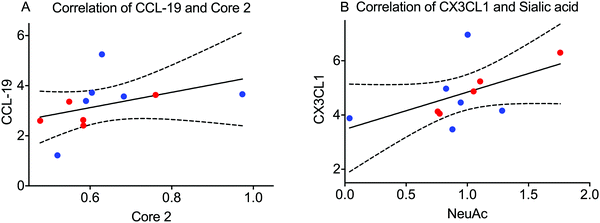 |
| Fig. 5 Salivary C motif chemokine 19 (CCL-19) and fractalkine (CX3CL1) is correlated with MUC7 glycosylation features. (A) CCL-19 correlates with the level of core 2 structures identified on MUC7 (spearman r = 0.78; p < 0.01). (B) Fractalkine correlates with the level of sialylation of MUC7 (spearman r = 0.63; p < 0.05). BMS patients are labeled with blue dots and the controls with red dots. The dash lines represent the error of equations. | |
Discussion
The current study ascertained altered oligosaccharide classes, particularly Si-Lex epitope in saliva from BMS patients. We chose to study MUC7 O-linked glycosylation, since the glycosylation of this mucin is reported to be consistent between healthy individuals,20 while the other major salivary mucin MUC5B glycosylation is heterogeneous and is affected by blood group and the secretory status.24 Hence, we would be more likely to identify if there are specific BMS related glycosylation changes on MUC7.
BMS has been characterized as a condition where the pain in the oral mucosa persists without any visible laboratory or clinical findings.25 Patients with BMS often suffer from dry mouth and can have reduced whole saliva secretion rates.21 Results from the PEA analysis shows the spread of the standard deviation between BMS and controls revealed patients with BMS being a heterogeneous group. Chronological age per se did not affect the individual inflammatory biomarkers in our study (data not shown). However, the cumulative effects of aging, medication and comorbid conditions of patients (and also controls) included in the study are likely to produce a spectrum of low-grade chronic inflammation. The increased heterogeneity of BMS inflammatory marker levels detected in this report may be a consequence of the multifactorial etiology of BMS, where the sense of oral burning could have several causes; either depending upon on a low level of chronic inflammation or non-inflammatory driven causes. Additionally, BMS itself is based upon diagnosis of exclusion, which further complicates the overall biomarker analysis.
The Si-Lex epitope has been found to be expressed on MUC7.20 Glycosylation changes have been investigated in conditions such as aphthous stomatitis, inflammation and cancer.16,26 Altered terminal sugar residue may have regulatory functions and confer biological consequences, which might be the case also in BMS patients. The reduction in the Si-Lex could result in reduced ability in clearing the bacteria from the oral cavity.27 In addition, reduction in the sialylated residues may negatively affect the hydration of mucosal surfaces leading to the sensation of dry mouth in these BMS patients. Reduced sialylation has been confirmed in dry mouth patients and Sjögren's syndrome previously.14,15
Overall reduced sialylated structure particularly, Si-Lex could be an important functional change observed in patients with BMS. The results from the inflammatory marker analysis suggest that patients with BMS to exhibit a heterogeneous but low-grade inflammation. Proteomic analysis of unstimulated whole saliva in another study suggested patients with BMS to have an ongoing low-level inflammation evidenced by increased level of the marker cystatin SN in unstimulated whole saliva.28 With a low-grade inflammation, the data suggest that inflammatory screening should be performed on a larger BMS patient cohort to investigate its significance. This in turn also allows stratification of the BMS patients to more homogeneous groups. In this report, an effect of inflammaging is proposed in BMS. To assess the existence of inflammaging subtypes of BMS, longitudinal and/or including also additional control groups of younger individuals may be necessary. The existence of low Si-Lex expression associated with BMS would help the physicians identify BMS risk group and allow further investigation of BMS subtypes (e.g. low-inflammatory and non-inflammatory), which hopefully would result in better treatment strategies.
The correlation of MUC7 glycosylation with the chemokines CCL-19 and CX3CL1 indicates a complex relation between the detected Si-Lex epitope and its regulation in low-grade inflammation. The correlation between CCL-19 and core-2 oligosaccharides suggest an involvement of the core 2 beta 1,6 N-acetylglucosaminyltransferase (C2GnT). The transcription regulation of the C2GnT-1 in human has been shown to require the transcription factor Sp-1 both in lymphocyte and epithelial derived cells.29 CCL-19 and its receptor chemokine receptor 7 (CCR7), has been linked to several salivary related diseases including increased transcription of CCR7 in malignant salivary gland tumors.30 Increased expression of CCL-19/CCR7 due to formation of germinal centre in primary Sjögren's syndrome has also been reported.31 The CCL19/CCR7 pathway has been suggested to up-regulate the expression of heparanase via Sp1 in lung cell line A54932 indicating a connection between CCL19 and Sp1 transcription regulation that could also be relevant for how CCL19 is regulating C2GnT. However, to date, no cellular connection between CCL-19 and C2GnT has been demonstrated.
The correlation of between fractalkine (CX3CL1) and sialylation is even more difficult to dissect. Expression of fractalkine and its receptor CX3CR1 receptor has shown to be up-regulated in salivary glands in patients with primary Sjögren's syndrome.33 Relevant sialyl transferases, ST3GAL1 for core 1 and core 2 smaller structures and ST3GAL4 for branched Si-Lex containing structures have suggested to be regulated by transcription factors such as Sp1, USF, NF-kB, AP-2 and c-Ets.34–36 Synovial fibroblasts have been shown to activate NF-kB37via fractalkine, suggesting a pathway for fractalkine regulation of ST3GAL1 and ST3GAL4. Only limited information is available about other sialyltransferase related transcription factors and how/if they are controlled by fractalkine stimulation. Fractalkine can also stimulate local secretion of inflammatory mediators, providing the possibility of secondary intercellular communication for sialyltransferase induction and not a direct intracellular transcriptional regulation.
Conclusions
The observed correlation between chemokines and glycosylation related to the identified BMS associated Si-Lex still need to be explored. Our data indicates a complex indirect relation, where potentially altered levels of sialyl-transferase and C2GnT activity promote elongation and branching of MUC7 oligosaccharides generating altered level of Si-Lex. Hence, for a multifaceted disease such as BMS, a marker that senses multiple pathways and combination of genes such as glycosylation may be beneficiary.
Conflicts of interest
The authors declare that they have no competing interest.
Acknowledgements
The authors are thankful to the patients and controls that participated in the study. We would also like to thank the TUA Research Funding Grant TUAGBG-66851, TUAGBG-289931, TUAGBG-627791, Wilhelm and Martina Lundgren Foundation, and Swedish Research Council (621-2013-5895). QC was supported by the Marie Curie ITN grant agreement Nr 316929 (GastricGlycoExplorer). PEA was performed by the PLA Proteomics Facility, Uppsala University, Science for Life Laboratory.
References
- M. Grushka, Clinical features of burning mouth syndrome, Oral Surg., Oral Med., Oral Pathol., 1987, 63, 30–36 CrossRef CAS.
- S. Acharya, A. Carlén and B. Wenneberg,
et al., Clinical characterization of women with burning mouth syndrome in a case-control study, Acta Odontol. Scand., 2017, 76, 1–8 Search PubMed.
- H. Imura, M. Shimada, Y. Yamazaki and K. Sugimoto, Characteristic changes of saliva and taste in burning mouth syndrome patients, J. Oral Pathol. Med., 2016, 45, 231–236 CrossRef PubMed.
- J. J. Kohorst, A. J. Bruce and R. R. Torgerson,
et al., A population-based study of the incidence of burning mouth syndrome, Mayo Clin. Proc., 2014, 89, 1545–1552 CrossRef PubMed.
- M. Bergdahl and J. Bergdahl, Burning mouth syndrome: prevalence and associated factors, J. Oral Pathol. Med., 1999, 28, 350–354 CrossRef CAS PubMed.
- M. A. Javaid, A. S. Ahmed, R. Durand and S. D. Tran, Saliva as a diagnostic tool for oral and systemic diseases, J. Oral. Biol. Craniofac. Res., 2016, 6, 66–75 Search PubMed.
- H. Jasim, A. Carlsson and B. Hedenberg-Magnusson,
et al., Saliva as a medium to detect and measure biomarkers related to pain, Sci. Rep., 2018, 8, 3220, DOI:10.1038/s41598-018-21131-4.
- R. M. Nagler and O. Hershkovich, Age-related changes in unstimulated salivary function and composition and its relations to medications and oral sensorial complaints, Aging: Clin. Exp. Res., 2005, 17, 358–366 Search PubMed.
- T. Fulop, A. Larbi and G. Dupuis,
et al., Immunosenescence and Inflamm-Aging As Two Sides of the Same Coin: Friends or Foes?, Front. Immunol., 2018, 8, 1960 CrossRef PubMed.
- C. Castelo-Branco and I. Soveral, The immune system and aging: a review, Gynecol. Endocrinol., 2014, 30, 16–22 CrossRef CAS PubMed.
- M. Moreno-Villanueva and A. Burkle, Epigenetic and redox biomarkers: Novel insights from the MARK-AGE Study, Mech. Ageing Dev., 2018, 177, 128–134 CrossRef PubMed.
- L. A. Tabak, M. J. Levine, I. D. Mandel and S. A. Ellison, Role of salivary mucins in the protection of the oral cavity, J. Oral Pathol., 1982, 11, 1–17 CrossRef CAS.
- L. A. Tabak, Structure and function of human salivary mucins, Crit. Rev. Oral Biol. Med., 1990, 1, 229–234 CrossRef CAS.
- N. M. Chaudhury, G. B. Proctor and N. G. Karlsson,
et al., Reduced Mucin-7 (Muc7) Sialylation and Altered Saliva Rheology in Sjogren's Syndrome Associated Oral Dryness, Mol. Cell. Proteomics, 2016, 15, 1048–1059 CrossRef CAS PubMed.
- N. M. Chaudhury, P. Shirlaw and R. Pramanik,
et al., Changes in Saliva Rheological Properties and Mucin Glycosylation in Dry Mouth, J. Dent. Res., 2015, 94, 1660–1667 CrossRef CAS PubMed.
- M. Zad, S. A. Flowers and M. Bankvall,
et al., Salivary mucin MUC7 oligosaccharides in patients with recurrent aphthous stomatitis, Clin. Oral Investig., 2015, 19, 2147–2152 CrossRef PubMed.
-
The International Classification of Headache Disorders, 3rd edition (beta version), Cephalalgia, 2013, 33, 629–808.
- K. Krzywkowski, E. W. Petersen and K. Ostrowski,
et al., Effect of glutamine and protein supplementation on exercise-induced decreases in salivary IgA, J. Appl. Physiol., 2001, 91, 832–838 CrossRef CAS PubMed.
- E. Assarsson, M. Lundberg and G. Holmquist,
et al., Homogenous 96-plex PEA immunoassay exhibiting high sensitivity, specificity, and excellent scalability, PLoS One, 2014, 9(4), e95192 CrossRef PubMed.
- N. G. Karlsson and K. A. Thomsson, Salivary MUC7 is a major carrier of blood group I type O-linked oligosaccharides serving as the scaffold for sialyl Lewis x, Glycobiology, 2009, 19, 288–300 CrossRef CAS PubMed.
- S. Acharya, C. Hägglin and M. Jontell,
et al., Saliva on the oral mucosa and whole saliva in women diagnosed with burning mouth syndrome, Oral Dis., 2018, 24, 1468–1476 CrossRef PubMed.
- J. A. Stenken and A. J. Poschenrieder, Bioanalytical chemistry of cytokines--a review, Anal. Chim. Acta, 2015, 853, 95–115 CrossRef CAS PubMed.
- E. Rodrigues and M. S. Macauley, Hypersialylation in Cancer: Modulation of Inflammation and Therapeutic Opportunities, Cancers, 2018, 10(10), 207 CrossRef PubMed.
- K. A. Thomsson, B. L. Schulz, N. H. Packer and N. G. Karlsson, MUC5B glycosylation in human saliva reflects blood group and secretor status, Glycobiology, 2005, 15, 791–804 CrossRef CAS PubMed.
- S. K. Jaaskelainen and A. Woda, Burning mouth syndrome, Cephalalgia, 2017, 37, 627–647 CrossRef PubMed.
- A. Sebastian, M. A. Alzain and C. O. Asweto,
et al., Glycan Biomarkers for Rheumatoid Arthritis and Its Remission Status in Han Chinese Patients, OMICS, 2016, 20, 343–351 CrossRef CAS PubMed.
- A. Prakobphol, K. Tangemann and S. D. Rosen,
et al., Separate oligosaccharide determinants mediate interactions of the low-molecular-weight salivary mucin with neutrophils and bacteria, Biochemistry, 1999, 38, 6817–6825 CrossRef CAS PubMed.
- T. Cabras, B. Manconi and M. Castagnola,
et al., Proteomics of the acid-soluble fraction of whole and major gland saliva in burning mouth syndrome patients, Arch. Oral Biol., 2019, 98, 148–155 CrossRef CAS PubMed.
- V. R. Falkenberg and N. Fregien, Control of core 2 beta 1,6-N-acetylglucosaminyltransferase-I transcription by Sp1 in lymphocytes and epithelial cells, Glycoconjugate J., 2007, 24, 511–519 CrossRef CAS PubMed.
- M. R. Haghshenas, M. J. Ashraf and B. Khademi,
et al., Chemokine and chemokine receptor patterns in patients with benign and malignant salivary gland tumors: a distinct role for CCR7, Eur. Cytokine Network, 2017, 28, 27–35 CAS.
- F. Carubbi, A. Alunno and P. Cipriani,
et al., Is minor salivary gland biopsy more than a diagnostic tool in primary Sjogrens syndrome? Association between clinical, histopathological, and molecular features: a retrospective study, Semin. Arthritis Rheum., 2014, 44, 314–324 CrossRef PubMed.
- Q. Zhang, L. Sun and L. Yin,
et al., CCL19/CCR7 upregulates heparanase via specificity protein-1 (Sp1) to promote invasion of cell in lung cancer, Tumour Biol., 2013, 34, 2703–2708 CrossRef CAS PubMed.
- J. H. Lee, S. K. Kwok and S. M. Jung,
et al., Role of fractalkine in the pathogenesis of primary Sjogren syndrome: increased serum levels of fractalkine, its expression in labial salivary glands, and the association with clinical manifestations, J. Rheumatol., 2014, 41, 2425–2438 CrossRef PubMed.
- A. Taniguchi, I. Yoshikawa and K. Matsumoto, Genomic structure and transcriptional regulation of human Gal beta 1,3 GalNAc alpha 2,3-sialyltransferase (hST3Gal I) gene, Glycobiology, 2001, 11, 241–247 CrossRef CAS PubMed.
- A. Taniguchi, M. Hioki and K. Matsumoto, Transcriptional regulation of human Gal beta 1,3 GalNAc/Gal beta 1, 4 GlcNAc alpha 2,3-sialyltransferase (hST3Gal IV) gene in testis and ovary cell lines, Biochem. Biophys. Res. Commun., 2003, 301, 764–768 CrossRef CAS PubMed.
- K. Higai, S. Ishihara and K. Matsumoto, NFkappaB-p65 dependent transcriptional regulation of glycosyltransferases in human colon adenocarcinoma HT-29 by stimulation with tumor necrosis factor alpha, Biol. Pharm. Bull., 2006, 29, 2372–2377 CrossRef CAS PubMed.
- X. Guo, Y. Pan and C. Xiao,
et al., Fractalkine stimulates cell growth and increases its expression via NF-kappaB pathway in RA-FLS, Int. J. Rheum. Dis., 2012, 15, 322–329 CrossRef CAS PubMed.
Footnote |
† Electronic supplementary information (ESI) available. See DOI: 10.1039/c9mo00061e |
|
This journal is © The Royal Society of Chemistry 2019 |
Click here to see how this site uses Cookies. View our privacy policy here.