DOI:
10.1039/C9MD00405J
(Research Article)
Med. Chem. Commun., 2019,
10, 1966-1987
Methyl sulfonamide substituents improve the pharmacokinetic properties of bicyclic 2-pyridone based Chlamydia trachomatis inhibitors
Received
20th August 2019
, Accepted 20th September 2019
First published on 17th October 2019
Abstract
Chlamydia trachomatis infections are a global health problem and new approaches to treat C. trachomatis with drugs of high specificity would be valuable. A library of substituted ring fused 2-pyridones has been synthesized and evaluated for their ability to attenuate C. trachomatis infectivity. In vivo pharmacokinetic studies were performed, with the best candidates demonstrating that a C8-methylsulfonamide substituent improved pharmacokinetic properties important for oral administration. C8-Methyl sulfonamide analogue 30 inhibited C. trachomatis infectivity in low micromolar concentrations. Further pharmacokinetic evaluation at an oral dose of 10 mg kg−1 showed an apparent bioavailability of 41%, compared to C8-cyclopropyl and -methoxy analogues which had negligible oral uptake. In vitro ADME (absorption, distribution, metabolism and excretion) testing of solubility and Caco-2 cell permeability revealed that both solubility and permeability is greatly improved with the C8-methyl sulfonamide 30, effectively moving it from BCS (Biopharmaceutical Classification System) class IV to II.
Introduction
Chlamydia trachomatis is the most common cause of sexually transmitted disease and infectious blinding, infecting over one hundred million individuals globally every year.1 Chronic genital infections can cause infertility in women and inflammatory arthritis in both genders.2Chlamydia is a Gram negative obligate intracellular bacterium with a unique developmental cycle. The extracellular form, elementary body (EB), attaches and enters the host cell.3 Once intracellular, Chlamydia differentiates to the non-infectious replicative intracellular form, reticulate body (RB).4 RBs replicate within a vacuole-like structure termed inclusion for 36 to 72 hours. After replication, RBs differentiate back to EBs, which abandon the host cell by lysis or extrusion.5
Infections with C. trachomatis are routinely treated with doxycycline or azithromycin, with high efficacy.6 However, the treatment of uncomplicated infections with broad-spectrum antibiotics disturbs the commensal flora in both the short and long term.7 Moreover, exposure to antibiotics contributes to the overall selective pressure on bacterial resistance.8 Anti-virulence compounds with selective effect on Chlamydia would not only reduce the use of important broad-spectrum antibiotics but also reduce side effects on the normal flora and the resulting selection for antibiotic resistant strains.
In search for potent anti-virulence compounds targeting C. trachomatis we previously identified compound 1 (Fig. 1) as a possible candidate.9 When C. trachomatis was treated with this compound at 2.5 μM in vitro, progeny was produced but their ability to infect new cells was attenuated. It was observed that inhibition of the pathogenic ability of C. trachomatis was partly due to an effect on glucose uptake.9 Introduction of an amine substituent in the C6-position and saturation of the C2–C3 double bond resulted in compound 2, with higher activity and better physiochemical properties than its precursor.10 Further development by exchanging the hydrolysable C3-phenyl amide to non-hydrolysable amide isosteres resulted in the potent 1,2,3-triazole analogue 3 (Fig. 1).11 The ring-fused 2-pyridone analogues 2 and 3 inhibit Chlamydial infectivity (EC50 < 60 nM) in a cell based assay without effecting host microbiota and showed no mutagenic potential when assessed with the Ames test.11,12 Ideally a drug for the genital C. trachomatis infection would be administered orally. In vitro ADME (absorption, distribution, metabolism, excretion) testing regarding solubility and permeability was performed on 2 (not shown), yielding poor solubility and moderate permeability. Both compounds were also tested in vivo, via intravenous (IV) and per oral (PO) administration. The data showed very low blood concentrations especially of 2, while 3 had high enough blood concentrations to enable calculation of intravenous pharmacokinetic parameters indicating a high steady state volume of distribution (Vss) (Table 4, Fig. 2). Intending to improve pharmacokinetic properties, we decided to explore the possibility of modifying the C8-position of the central fragment. In our previous structure–activity relationship investigations, our focus was on ring-fused 2-pyridone analogues with structural changes at the C7-, C6-, and C3-positions, while the C8-cyclopropyl substituent remained unmodified. In this study, we have instead synthesized and evaluated a small library of C8-oxygen and nitrogen analogues with the overall aim to maintain anti-Chlamydial activity and improve oral availability. An earlier study10 showed that there was no discernable difference between the two enantiomers of compound 24 and therefore all compounds presented here were prepared and tested as racemic mixtures.
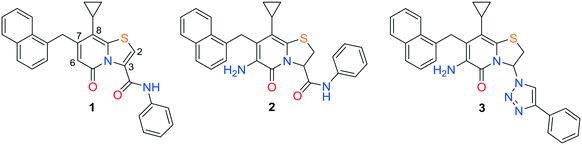 |
| Fig. 1 Chemical structures of compounds 1, 2, and 3. | |
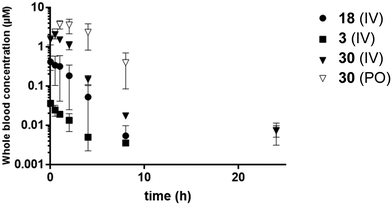 |
| Fig. 2 Blood concentration versus time of 3 (1.2 mg kg−1), 18 (0.9 mg kg−1), and 30 (1.0 mg kg−1 IV, 10 mg kg−1 PO). Error bars indicate SEM. | |
Results & discussion
By introducing heteroatom containing substituents in the C8-position we aimed to reduce the lipophilicity and improve the drug-like properties of the compounds. First a set of C8-hydroxyl or -methoxy substituted analogues were designed and for these the previously published carboxylic acid 4 (ref. 13) served as a key intermediate (Scheme 1). In our previous study10 the phenyl amide and p-methyl phenyl amide at the C3-position gave similar biological results. We therefore prepared C8-methoxy, C3-amide analogues 5 and 6 by amide coupling under basic conditions with the corresponding amine and propylphosphonic anhydride as coupling reagent.14 C3-Phenyl amide 7 was generated by demethylation of analogue 5 with boron tribromide (Scheme 1). Nitration and subsequent reduction with zinc in acetic acid15 starting from amides 5 and 6 gave the desired C6-amines 8 and 9 (Scheme 1).
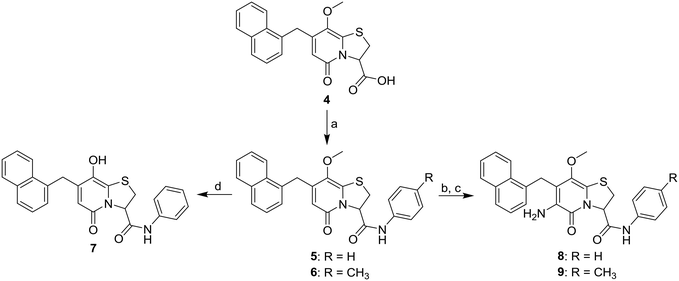 |
| Scheme 1 Synthesis of C8-methoxy amide analogues 5–7. Reagents and conditions: a) aniline or 4-methylaniline, propylphosphonic anhydride (50% in EtOAc), pyridine, MeCN/EtOAc (1 : 1), −10 °C to rt, 24–43 h, 5: 58%, 6: 38%; b) NaNO2, TFA, DCM, O2 atmosphere, rt, 1–7 h; c) activated Zn dust, AcOH, rt, 31–48 h, 8: 30% over two steps; 9: 35% over two steps; d) BBr3, DCM, −40 °C to rt, 6 h, 10%. | |
The previously observed potency of 3 (Fig. 1) also motivated us to synthesize the 1,2,3-triazole analogue. Thus, the carboxylic acid intermediate 4 underwent Curtius rearrangement in tert-butyl alcohol to acquire the BOC-protected amine 10, which upon acidic deprotection resulted in the primary amine 11 (Scheme 2). The final 1,2,3-triazole 12 was obtained by diazo transfer followed by Cu(I)-catalyzed azide–alkyne cycloaddition (CuAAC) (Scheme 2).
 |
| Scheme 2 Synthesis of C8-methoxy 1,2,3-triazole 12. Reagents and conditions: a) DPPA, Et3N, t-BuOH, 2 h, 85 °C, 60%; b) TFA, DCM, 2 h, rt, 32%; c) imidazole-1-sulfonyl azide H2SO4, CuSO4, K2CO3, MeOH, 40 h, 50 °C; d) phenyl acetylene, CuSO4, sodium ascorbate, DMF, H2O, rt, 4 h, 4% from 11. | |
Our previous study also revealed that the 1-naphthyl substituent could be exchanged for a 2,3-dimethyl phenyl substituent without loss of potency.10 This C7-substituent was incorporated into the ring-fused 2-pyridone scaffold using 2,3-dimethyl phenyl substituted Meldrum's acid 14 to give ring-fused 2-pyridone 15 (Scheme 3). The three C3-amides (16, 17, and 18) were obtained through methyl ester hydrolysis and then subsequent amide coupling of the generated acid using the corresponding aniline derivative. We have previously prepared and tested 1,2,4-oxadiazole substituted compounds which are easily accessible directly from the corresponding carboxylic acids.11 To further evaluate these moieties together with other C7- and C8-substituents we prepared two more of these amide isosteres via hydrolysis of methyl ester 15 and then amide coupling with benzamide oxime and TBTU followed by cyclization16 to generate the 1,2,4-oxadiazole 19. These analogues were C6-aminated via the established nitration-reduction route resulting in four C6-amine analogues 20, 21, 22, and 23 (Scheme 3).
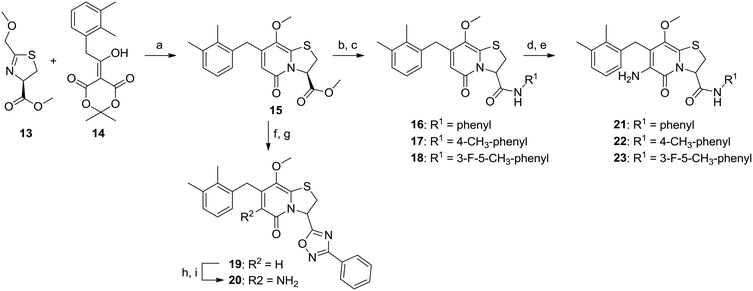 |
| Scheme 3 Synthesis of C8-methoxy analogues 16–23 with a C7-2,3-dimethyl phenyl substituent. Reagents and conditions: a) TFA, DCE, MWI, 120 °C, 3 min, 80%; b) 1 M LiOH(aq), THF, rt, 15 h; c) for 16 and 17: aniline, 4-methylaniline, propylphosphonic anhydride (50% in EtOAc), pyridine, MeCN/EtOAc (1 : 1), −10 °C to rt, 24 h, 16: 82%, 17: 39%; for 18: 3-fluoro-5-methylaniline, HATU, DIPEA, DCM, 2 h, 95%; d) NaNO2, TFA, DCM, O2 atmosphere, rt, 2.5–6 h; e) activated Zn dust, AcOH, rt, 20–23 h, 21: 30%, 22: 17%, 23: 38% over two steps; f) 1 M LiOH(aq), THF, rt, 15 h; g) benzamidoxime, TBTU, DIPEA, DMF, MWI, 170 °C,12 min, 46%; h) NaNO2, TFA, DCM, O2 atmosphere, rt, 1 h; i) activated Zn dust, AcOH, rt, 19 h, 60%. | |
The fourteen C8-oxygen analogues were evaluated for their ability to inhibit C. trachomatis infectivity by the previously described reinfect assay (Table 1). Reinfect values show inhibition of Chlamydia infectivity by the tested compound in relation to DMSO treated control infections.10,11 The reinfect assay is cell based and also provides preliminary information about the abilities of the compounds to penetrate biological membranes, attach to their target and exert their function on the intracellular bacteria. In brief, HeLa cells infected with C. trachomatis serovar L2 were treated with a compound (2.5 μM or 1 μM) and incubated for 48 h before Chlamydia was harvested by cell lysis. The cell lysate was used to reinfect fresh HeLa cells and the number of Chlamydia inclusion forming units (IFU) formed during the reinfection were enumerated after 48 hours incubation. The reinfect value is the reduction in percent of IFUs in a compound treated infection compared to DMSO treated controls. The cytotoxicity of the compounds at 10 μM was tested in a resazurin cell toxicity assay (HeLa cells) to assess that the anti-chlamydial effect of the compounds was not due to host cell toxicity.10,11
Table 1 C8-Oxygen analogues. Reinfect values indicate the % of inhibition of infectivity in the second cycle of infection at 2.5 μM or 1 μM. HeLa viability values express the % of cell growth when treated with 10 μM of compounds. Log
P is the logarithm of the partition coefficient between n-octanol and water
The C8-hydroxy (7) and -methoxy (5) analogues, both had slightly lower calculated log
P (ref. 17) value than the previously reported cyclopropyl analogue 24,10 but proved less active (Table 1). In addition, the synthesis of the hydroxyl analog required additional low yielding steps and therefore we decided not to continue with this series. In previous studies introduction of the C6-amine has been shown to improve activity10,11 but the corresponding C6-amine analogue 8 showed only 25% inhibition at 2.5 μM. Exchanging the 1-naphthyl group to a 2,3-dimethyl phenyl substituent at the C7-position resulted in a decreased log
P value as seen when comparing analogues 5 (5.0) and 13 (4.4), together with a small improvement in potency. However, the p-methyl phenyl amide 6 and the analogue 17 with a 2,3-dimethyl phenyl group at the C7-position demonstrated comparable anti-infective activity to phenyl amide 24 at 2.5 μM. Unfortunately, their effect dropped at 1 μM to 62% and 71% inhibition respectively. A more promising result was obtained with the 3-fluoro-5-methylaniline analogue 18, although the potency at 1 μM was still lower than 2 and 3. The 1,2,4-oxadiazole 19 and the 1,2,3-triazole 12 analogues were evaluated but proved less active than analogue 2 and the best C8 methoxy analogue 18. In contrast to previous studies, introduction of a C6-amine gave little benefit with a C8-methoxy substituent (Table 1). All the tested compounds showed low toxicity towards HeLa cells at 10 μM.
A general drop in potency of the C8-oxygen analogues compared with the cyclopropyl analogues was observed, with all C8-oxygen compounds losing activity at concentrations below 1 μM compared to the cyclopropyl analogues 2 and 3. However, analogue 18 was one of the most potent among the oxygen analogues and its synthesis in larger scale also proved straightforward resulting in a crystalline product suitable for various formulations. Therefore, this analogue was chosen for further evaluation together with the previously identified compounds 2 and 3. A formulation in lipid emulsion (for details about the vehicle see the Experimental section) enabled an oral dose of 10 mg kg−1. This formulation was used in an in vivo pharmacokinetic evaluation (Table 4, Fig. 2). Although the peak blood concentrations (Cmax) and AUC of compound 18 were higher compared to compound 3 after the intravenous administration, the oral uptake was unfortunately too low to allow for proper determination of pharmacokinetic parameters after oral administration. The concentrations of compound 2 after both intravenous and oral administration were too low to allow calculation of pharmacokinetic parameters. It was therefore clear that the oral bioavailability of these compounds was extremely poor.
We next considered that introduction of nitrogen based C8-substituents would potentially have a greater influence on drug-like properties. Therefore, a set of five analogues was designed for evaluation. The previously published primary amine intermediate 25 (ref. 13) served as the starting point and a collection of five compounds with different C8-nitrogen substituents were obtained (Scheme 4A). Methylation of the C8-primary amine, hydrolysis of the C3-methyl ester and following amide coupling using aniline resulted in dimethylamine 27. The secondary amine 26 was obtained through reductive alkylation of the C8-primary amine, followed by ester hydrolysis and amide coupling. Compounds 28 and 29 were synthesized by reaction with methyl chloroformate and acetyl chloride, respectively, followed by C3-methyl ester hydrolysis and amide coupling. The final methyl sulfonamide analogue was prepared by allowing the C8-amine intermediate 25 to react with methane sulfonyl chloride followed by hydrolysis and amide coupling using aniline to generate the C8-methyl sulfonamide 30 (Scheme 4A). Evaluation of their biological activity again showed a drop in potency compared to the cyclopropyl analogues but both 27 and 30 showed about 90% inhibition at 2.5 μM (Table 2). However, the calculated log
P was significantly lower for the methyl sulfonamide analogue 30 compared to the dimethylamine 27. To improve the potency a set of four additional methyl sulfonamide analogues were synthesized. Two of these were substituted phenyl amides (32 and 33, Scheme 4B and Table 2) chosen based on results of our previous study10 and prepared by amide coupling from the carboxylic acid 31.
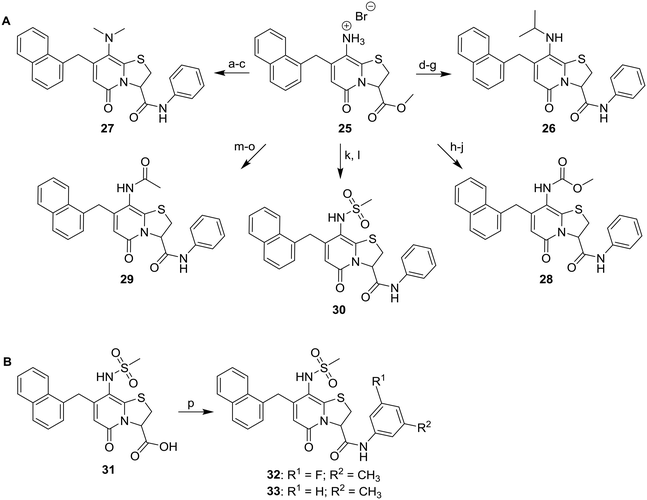 |
| Scheme 4 A. Synthesis of C8-nitrogen analogues 26–30. B. Synthesis of C8-methyl sulfonamide C3-amide derivatives 32 and 33. Reagents and conditions: a) CH3I, NaHCO3, DMF, 70 °C, 10 h; b) 1 M LiOH(aq), THF : MeOH (5 : 1), rt; c) aniline, HATU, DIPEA, DMF, 1 h, 55% from 25; d) NaBH(OAc)3, TFA, DCM, acetone, −15 °C to rt, overnight; e) 1 M LiOH(aq), THF : MeOH (4 : 1), rt, 1 h; f) suspended in DCM and dripped in to ethereal HCl, −20 °C, overnight; g) aniline, HATU, DIPEA, DMF, 1 h, 43% from 25; h) methyl chloroformate, pyridine, DCM, rt, 2 h; i) 1 M LiOH(aq), THF : MeOH (4 : 1), rt; j) aniline, HATU, DIPEA, DMF, 1 h, 38% from 25; k) methanesulfonyl chloride, pyridine, THF, rt, overnight; then: 1 M LiOH(aq), MeOH or THF : MeOH (3 : 1), rt; l) aniline, HATU, DIPEA, DMF, 1 h, 30: 52% from 25; m) acetyl chloride, pyridine, DCM, rt, overnight; n) 1 M LiOH(aq), THF : MeOH (5 : 1), rt, 2 h; o) aniline, HATU, DIPEA, DMF, 1 h, 50% from 25; p) 3-methylaniline or 3-fluoro-5-methylaniline, HATU, DIPEA, DMF, 4 h, 32: 49%, 33: 78%. The acid intermediates as isolated compounds are published previously.13 | |
Table 2 C8-Nitrogen analogues. Reinfect values indicate the % of inhibition of infectivity in the second cycle of infection at 2.5 μM or 1 μM. HeLa viability values express the % of cell growth when treated with 10 μM of compounds. Log
P is the logarithm of the partition coefficient between n-octanol and water
In addition, two methyl sulfonamide analogues with C3-amide isosteres instead of phenyl amides were synthesized. The first of these two, the 1,2,4-triazole 38 easily accessible from the corresponding methyl ester, was chosen based on results from our previous study11 and obtained as described in Scheme 5. A one-pot reaction18 was performed on the N-carboxybenzyl (Cbz) protected methyl ester 36 to give the 1,2,4-triazole 37. The desired methyl sulfonamide 38 was then prepared by acidic Cbz-deprotection followed by installment of the methyl sulfonamide functionality via a reaction with methane sulfonyl chloride (Scheme 5).
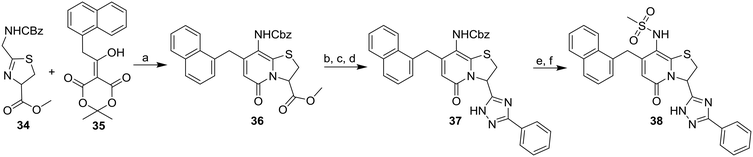 |
| Scheme 5 Synthesis of C8-methyl sulfonamide 1,2,4-triazole 38. Reagents and conditions: a) 35, TFA, DCM, 70 °C, 20 h, 85%; b) 1 M LiOH(aq), THF, rt, 2 h; c) benzamidine·HCl, HATU, DIPEA, DMF, 3.5 h; then (4-methoxybenzyl)hydrazine·HCl, AcOH, 80 °C, 20 h; d) TFA, DCM, 50 °C, 22 h, 39% from 36; e) 33% HBr/AcOH, DCM, rt, 2 h; f) methane sulfonyl chloride, pyridine, rt, 18 h; then: 1 M LiOH(aq), THF : MeOH (3 : 1), rt, 53% from 37. | |
Earlier studies showed that the 1,2,3-triazoles were the most potent amide bioisosteres and the 1,2,3-triazole 3 has previously successfully been obtained via diazo transfer with imidazole-1-sulfonyl-azide of the C3-primary amine.11 However, with a N-carboxybenzyl-protected amine at the C8-position, the diazo transfer reaction proved unsuccessful and was exchanged for a phthalimide as protecting group instead. The phthalimide substituted thiazoline derivative 40 was synthesized from 2-(1,3-dioxoisoindolin-2-yl)acetonitrile via the ethyl imidate 39 (Scheme 6). The ring-fused 2-pyridone methyl ester 41 was then obtained from 40 and 35 after 4 days at 80 °C and the following hydrolysis and re-closure of phthalimide gave the carboxylic acid 42. The BOC-protected 43 was generated by Curtius rearrangement in tert-butyl alcohol of intermediate 42 and subsequent acidic deprotection of 43 resulted in the C3-amine 44. Diazo transfer of intermediate 44 using imidazole-1-sulfonyl azide followed Cu(I)-catalyzed azide–alkyne cycloaddition (CuAAC) with phenyl acetylene resulted in the 1,2,3-triazole 45. The final compound 46 was obtained via deprotection, followed by reaction with methane sulfonyl chloride to generate the C8-methyl sulfonamide moiety.
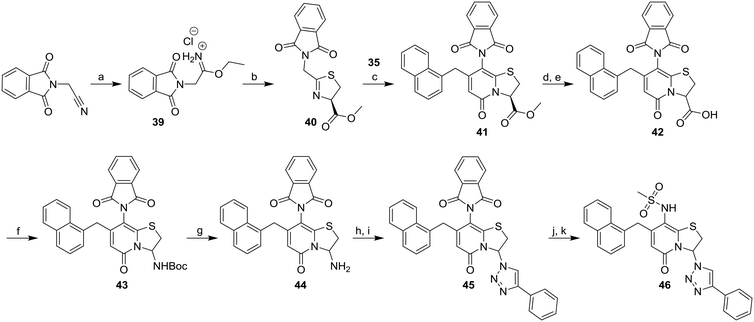 |
| Scheme 6 Synthesis of C8-methyl sulfonamide 1,2,3-triazole 46. Reagents and conditions: a) AcCl, EtOH/EtOAc (1 : 1), 0 °C to rt, 17 h, (see procedure); b) L-cysteine methyl ester·HCl, Et3N, DCM, rt, 3 days, 64%; c) 35, TFA, DCM, 80 °C, 4 days, 62%; d) 1 M LiOH(aq), THF, rt, 4 h; e) AcOH, DMF, 150 °C, 20 h, 78% from 41; f) DPPA, Et3N, t-BuOH, 2 h, 85 °C, 57%; g) TFA, DCM, rt, 2 h, 88%, h) imidazole-1-sulfonyl azide·H2SO4, CuSO4, K2CO3, MeOH, 24 h, 50 °C; i) phenyl acetylene, CuSO4, sodium ascorbate, DMF, H2O, rt, 3.5 h, 1% from 44; j) methyl amine, EtOH, rt, 20 h; k) methanesulfonyl chloride, pyridine, rt, 26 h; then: 1 M LiOH(aq), THF : MeOH (3 : 1), rt, 2 h, 99% from 45. | |
The four additional methyl sulfonamide analogues were evaluated in the cell-based infection assay but none of the new analogues showed any improvement compared to compound 30. Since the activity of 30 was still in the low μM range, with low toxicity and a lower calculated log
P compared to the analogues previously evaluated for pharmacokinetic properties, the methyl sulfonamide 30 was chosen together with analogues 3 and 18 for in vitro ADME determination (Table 3). Compounds 3 and 18 showed similar properties: very low solubility; low to moderate permeability with low or no efflux and low metabolic stability in both human and mouse liver microsomes (HLM, MLM). Analogue 30 on the other hand distinguished itself from 3 and 18 by improved solubility, much higher permeability and higher metabolic stability in both species. The mouse plasma protein binding was high, especially for analogues 3 and 18, which influences scaling (static well-stirred model) to hepatic clearance (CL) when using the plasma protein binding as a surrogate for the whole blood free fraction. Another limitation is that the blood to plasma ratio for these compounds are unknown, and it is known that in particular for high CL molecules the impact of the blood to plasma ratio may be large for these extrapolations.19 Thus, due to the high protein binding, the predicted unbound hepatic extraction (Eu) in mouse was low (Table 3).
Table 3
In vitro ADME properties of 3, 18 and 30
Assay |
3
|
18
|
30
|
Solubility (μM ± SD) |
0.31 ± 0.16 |
0.32 ± 0.04 |
1.3 ± 0.8 |
Caco-2 A-B Papp (×106 cm s−1 ± SD) |
0.33 ± 0.05 |
2.1 ± 0.04 |
30 ± 2.6 |
Caco-2 efflux ratio |
0.6 |
3.2 |
2.0 |
Mouse plasma protein binding (fraction unbound, %) |
<0.1 |
0.1 |
0.39 |
Intrinsic clearance (HLM, μl min−1 mg−1) |
187 |
184 |
39 |
Intrinsic clearance (MLM, μl min−1 mg−1) |
135 |
440 |
59 |
E
u (mouse unbound hepatic extraction) |
0.01 |
0.09 |
0.001 |
An in vivo pharmacokinetic validation to investigate the in vitro–in vivo correlation was then performed. The compounds were administered both intravenously and orally with the same formulation as previously used. The oral data for compound 3 and 18 was too low to be able to be quantified, therefore only intravenous is shown (Table 4, Fig. 2). Interestingly, a remarkable improvement was observed for analogue 30 compared to 3, 18 and the earlier evaluated candidates. Compound 30 yielded oral blood concentrations in the μM range, lower CL and an oral bioavailability of 41%. Analogue 18 showed very low AUC in comparison to 3 despite the similarity in in vitro ADME properties and it is likely that the increased membrane permeation contributed to the higher CL of compound 3. In comparison to the obtained in vitro data, the predicted CL is underestimated indicating erroneous assumptions in models or large extrahepatic contributions. Taken together, incorporation of a sulfonamide contributes to a lowered CL in vitro and in vivo, resulting in a longer in vivo half-life (t1/2). Further, the sulfonamide effectively shifted compound 30 into a Biopharmaceutical Classification System (BCS) category II compound (high permeability, low solubility), while compounds 3 and 18 were categorized as class IV compounds (low permeability, low solubility), which are difficult to handle in further drug development.
Table 4
In vivo mouse pharmacokinetics of compounds 3, 15 and 30. C0/Cmax: maximal measured plasma concentration; Tmax: time of C0/Cmax; AUC: calculated area under the curve, extrapolated to infinity; t1/2: elimination half-life; CL: clearance; Vss: volume of distribution at steady state; F: bioavailability
|
3
|
18
|
30
|
30
|
Variability in the compounds dose was caused by the different weight of mice between experiments.
|
Administration |
IV |
IV |
IV |
PO |
Dose (mg kg−1)a |
1.2 |
0.90 |
1.0 |
10 |
C
0/Cmax (MM) |
0.040 |
0.41 |
1.7 |
3.7 |
T
max (h) |
— |
— |
— |
1 |
AUC0−∞ (μM × h) |
0.10 ± 0.01 |
0.95 ± 0.28 |
4.8 ± 0.4 |
20.3 ± 4.5 |
t
1/2 (h) |
3.7 |
1.2 |
5.3 |
2.6 |
CL (L h−1) |
0.51 ± 0.05 |
0.040 ± 0.01 |
0.010 ± 0.001 |
— |
V
ss (L kg−1) |
27 ± 22 |
1.1 ± 0.6 |
0.62 ± 0.16 |
— |
F (%) |
— |
— |
— |
41 |
With these encouraging results in hand, a first treatment study was conducted. Although the solubility had improved slightly it was not possible to dissolve analogue 30 in the vehicle at a higher dose than 10 mg kg−1, which was given twice a day via oral gavage to nine C. trachomatis-infected mice. As a control, vehicle without active compound was given to nine mice, while six mice received doxycycline. The mice showed no negative vital signs from the treatment, but unfortunately the course of infection was not improved at this dose (Fig. 3).
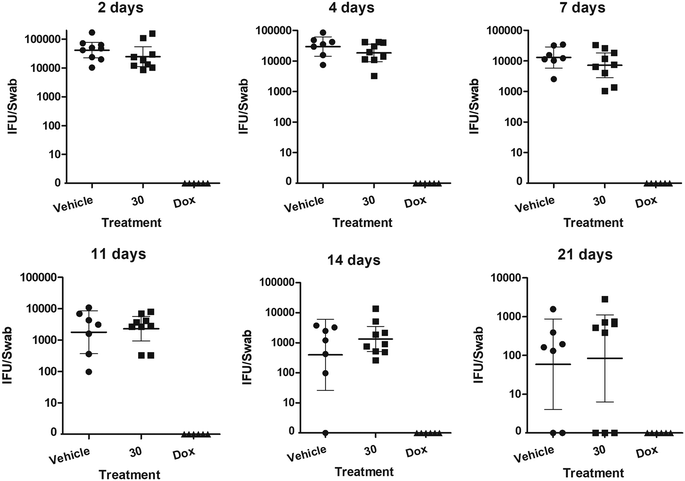 |
| Fig. 3 Oral treatment with compound 30 did not affect vaginal C. trachomatis infection in mice. The number of C. trachomatis IFUs per vaginal swab over time during the 7 days treatment and afterwards. C3H/HeJ mice were vaginally infected with C. trachomatis and treated with 10 mg kg−1 of compound 30, vehicle alone or 2 mg kg−1 doxycycline. | |
The pharmacokinetic improvements observed represent a great advance towards a proof of concept for an orally available drug to treat C. trachomatis infection. The finding that C8-methyl sulfonamides so strongly can influence important pharmacokinetic parameters in favor of oral administration is an important step forward. Although no treatment effect was observed at the tested dose, the compounds show very low toxicity and therefore higher doses of the active compound could be utilized in future trials. To accomplish this, improved vehicles will be required to deliver these analogues in higher dose. In parallel, additional fine tuning of the substitution pattern to improve the potency while retaining the important pharmacokinetic properties observed with the methyl sulfonamide 30 is ongoing in our laboratories.
Materials and methods
Biology
Cell culture and chlamydia propagation.
HeLa 229 cells (CCL-2.1; ATCC) were cultured at 37 °C (5% CO2) in RPMI-1640 medium (HyClone) with 25 mM HEPES and 2 mM L-glutamine, supplemented with 10% fetal bovine serum (FBS) (Sigma) and 10 μg mL−1 gentamicin. For the resazurin cytotoxicity experiments, colorless DMEM (Gibco) supplemented with 10% FBS (Sigma) was used. Chlamydia trachomatis serovar L2 454/Bu (VR902B; ATCC) was cultured in HeLa cells. EB stocks of C. trachomatis were purified as described by Caldwell et al. and stored at −80 °C in SPG buffer (0.25 M sucrose, 10 mM sodium phosphate and 5 mM L-glutamic acid).20
Reinfect assay.
Reinfection experiments were performed as previously described.10C. trachomatis serovar L2 EBs were mixed with Hank balanced salt solution (HBSS) and used to infect HeLa cells at a MOI of 0.3–0.5. After 1 h, the compounds were added mixed with RPMI at 2.5 μM or 1 μM. The cells were incubated for 44–48 h, then cold Milli-Q water was added in order to osmotically lyse the cells and harvest the EBs. 4× SPG was added to the EBs on MQ water to reach 1× SPG, and 10-fold serial dilutions were performed in HBSS. Fresh HeLa cells were infected with the diluted EBs, substituting the HBSS by fresh RPMI medium 1 h post infection. Cells were incubated for 44–46 h before fixation with methanol. Inclusions were stained with in-house rabbit anti-Chlamydia antibodies21 (1
:
1000), followed by secondary donkey anti-rabbit FITC-labeled antibody (1
:
1000) (Jackson ImmunoResearch). Cell nuclei were stained with DAPI (1
:
1000). Infected cells were observed and measured by Arrayscan automated microscopy (ArrayScan VTI HCS, Thermo Scientific). Reinfect value was calculated as the percentage of IFUs compared to a DMSO treated control. The presented value is the average of three independent experiments with duplicates for each condition.
Resazurin cytotoxicity assay.
HeLa cells were seeded in 96 well plates, leaving the outer wells filled with PBS to prevent edge effects. Compounds were tested at 10 μM in DMEM with 0.5% DMSO. Incubation proceeded for 48 h, whereafter 10 μL of resazurin (400 μM) was added to each well followed by 3 h incubation at 37 °C, 5% CO2 in the dark. After that, fluorescence in the wells was analyzed by Tecan Safire plate reader (excitation 535 nm, emission 595 nm). The results were calculated as the average from triplicate compound treated wells in percent of a DMSO treated control in Graph Pad Prism.
Compound formulation for in vivo administration.
For intravenous administration, the compound was dissolved in DMSO and diluted to 1 mM corresponding to 0.45–0.51 mg mL−1 compound in PBS with 10% cremophor, 5% EtOH and 4% DMSO. For oral administration the compound was dissolved in a lipid vehicle containing 55% capmul, 15% akomed, 15% etanol and 15% cremophor that was vigorously mixed with lukewarm water to form an emulsion. The final concentration in the oral dose solutions was 2.24 mg mL−1 compound, 6.8% capmul, 1.9% akomed, 1.9% cremophor, 1.9% EtOH in H2O.
In vitro ADME determination.
All quantitative analyses were performed using liquid chromatography tandem mass spectrometry (LC-MS/MS) on a Sciex QTRAP 6500 or a Waters XEVO TQ triple quadrupole mass spectrometer coupled to a Waters Acquity ultra-performance liquid chromatography (UPLC). Mobile phases (A): 0.1% formic acid, (B): 0.1% formic acid in acetonitrile were used with column HSS T3 50 × 2 mm and runtime was 2 minutes.
The thermodynamic solubility assay utilized solid weighed compound (0.2–0.5 mg) in a HPLC vial and PBS was added to yield a saturated solution. The vial was sealed and shaken (900 rpm) at room temperature for 48 h. After the incubation the suspension was transferred to conical glass inserts and centrifuged for 30 min at 10
000×g, at 23 °C. The supernatant was then diluted in acetonitrile/H2O (50/50) 10–1000× for LC-MS/MS analysis, in two steps.
The Caco-2 study was performed in accordance with published protocols.22 Caco-2 cell monolayers (passage 94–105) were grown on permeable filter support and used for transport study on day 21 after seeding. Prior to the experiment a drug solution of 1 μM was prepared and warmed to 37 °C. The Caco-2 filters were washed with pre-warmed HBSS prior to the experiment, and thereafter the experiment was started by applying the donor solution on the apical or basolateral side. The transport experiments were carried out at pH 7.4 in both the apical and basolateral chamber. The experiments were performed at 37 °C and with a stirring rate of 500 rpm. The receiver compartment was sampled at 15, 30 and 60 minutes, and at 60 minutes also a final sample from the donor chamber was taken in order to calculate the mass balance of the compound. The samples (100 μl) were transferred to a 96-well plate containing 100 μl acetonitrile and Warfarin as internal standard and was sealed until LC-MS/MS analysis.
The metabolic stability assay was determined in 0.5 mg ml−1 human or animal liver microsomes (XenoTech LLC, KS, USA) at a compound concentration of 1 μM in 100 mM phosphate buffer pH 7.4 in a total incubation volume of 500 μl. The reaction was initiated by addition of 1 mM NADPH (final concentration). At various incubation times, i.e. at 0, 5, 10, 20, 40 and 60 min, a sample was withdrawn from the incubation and the reaction was terminated by addition to 1.5 volumes of cold acetonitrile. After centrifugation the supernatant was analyzed for the amount of parent compound remaining by LC-MS/MS. To determine the in vitro half-life, the percent remaining compound (analyte area/IS area) was calculated using time 0 min sample as 100%. The natural logarithm of the % remaining compound was plotted against time followed by linear regression.25
The plasma protein binding assay utilized rapid equilibrium dialysis (RED, Thermo Scientific Inc., USA) device inserts which allow for short dialysis times (2–4 h) as compared to traditional methods (>8 h) and minimal drug consumption in a 96-well format. Pooled human plasma with citric acid as anti-coagulant from two healthy non-smoking blood donors was provided by Uppsala Academic Hospital. Human plasma was obtained from volunteered, healthy, regular blood donors and no ethical improvement was required. Informed consent was obtained from human donors. Plasma from CD-1 mice with EDTA or Heparin as anti-coagulant was purchased from Novakemi AB, Sweden. In brief, 0.2 ml 10% plasma, 90% isotonic buffer test solution was spiked 10 μM compound and 1% final DMSO concentration and transferred to the membrane tube in the RED insert. 0.35 ml isotonic phosphate buffer pH 7.4 was added to the other side of the membrane. The 96-well base plate was then sealed with an adhesive aluminum foil film to prevent evaporation. The sample was incubated with rapid rotation (900 rpm) on a Kisker rotational incubator (Kisker Biotech Gmbh & Co. KG, Germany) at 37 °C for 4 h to achieve equilibrium. A stability test of the test solution was also prepared to allow detection of drug degradation, 100 μL of the spiked plasma test solution (in a plastic vial or on a sealed plate) was incubated at 37 °C for 4 h (or as long as the dialysis time). The plasma test solution was frozen directly after the administration to prevent any degradation. Prior to LC-MS/MS analysis the plasma and buffer sample were treated with the addition of methanol (1
:
3) containing Warfarin as analytical internal standard to precipitate proteins. The standard curve was created using the previously frozen plasma standard. The volume in each well consisted of 25 μl plasma and 25 μl buffer to minimize any matrix related effects in interpretation. The plate was then sealed, centrifuged and the supernatant was analyzed by mass spectrometry (LC-MS/MS). To adjust the fraction unbound (fu) for the diluted plasma the following equation was used: undiluted fu = 1/DF/{[(1/fu) − 1] + 1/DF}, DF = dilution factor.23
Pharmacokinetics in mice.
Compounds were administered to mice either intravenously (IV) by a 50 μL tail vein injection or orally (PO) by a 100 μL oral gavage. Three mice per dose and route of administrations were used and the doses are indicated in Table 4. Compound 30 was given to 9 week old females C3H/HeOuJ (JAX stock #000635, Charles River, Germany), compounds 15 and 3 were given to 22-week-old females C3H/HeJ (JAX stock #000659, The Jackson laboratory, USA). Blood samples were collected from the tail vein at 10 minutes after intravenous administration and at 0.5, 1, 2, 4, 8, and 24 hours after both IV and PO administration using lithium heparin coated capillary blood collection tubes (Microvette CB300LH, Sarstedt, Nümbrecht, Germany). Twenty μL blood was mixed with 40 μL acetonitrile and stored at −20 °C until analysis. Whole blood samples were further diluted to 200 μl and dose solutions were diluted 500 times in acetonitrile/H2O (60/40) and extracted by addition of ice-cold methanol (1
:
4) followed by centrifugation at 3500
rpm prior to analysis. Standard curves were constructed using blank mouse blood matrix spiked with compound from 10 mM DMSO stocks. Calculations of non-compartmental pharmacokinetic properties utilized Microsoft Excel and Graphpad Prism 7. Errors are standard deviations of the AUC and nonlinear regression fitting.
Animal experiments were performed according with the Guidelines for Care and Use of Laboratory Animals of Umeå University and approved by Umeå Animal Research Ethical Committee, the Swedish Board of Agriculture (A4-14, A75-15).
C. trachomatis in vivo infection model.
Eight week old female C3H/HeJ mice (JAX stock #000659, The Jackson laboratory, USA) were injected subcutaneously with 2.4 mg of medroxiprogesteron acetate (Pfizer) 10 and 3 days prior to infection to synchronize their estrous cycles. A pediatric nylon flocked swab (Copan, Italia) pre-wet in SPG was used to remove vaginal mucus prior to intravaginal injection of 5000 CFU C. trachomatis serovar D (VR-885, ATCC) in 10 μL SPG. Mice were placed in an inverted position during 30 minutes under midazolam/ketamine anesthesia to allow the inoculum to gravitate to the uterine cervix. Treatment by oral gavage was initiated 10 hours after infection and repeated every 12 hours for 7 days. Nine mice were given 100 μL 2.33 mg mL−1 of compound 30 in a water emulsion containing 6.8% capmul, 1.9% akomed, 1.9% cremophor and 1.9% EtOH. Another nine mice received 100 μL of the same formulation without compound 30 and six mice were given 100 μL 2 mg mL−1 doxycycline in water. Day 2, 4, 7, 11, 14 and 21 after infection, vaginal swab samples were obtained by rotating a SPG-wet pediatric nylon flocked swab intravaginally during 10 seconds. Each swab was transported in a micro centrifuge tube with 200 μL ice cold SPG and vigorously vortexed prior to dilution of the SPG with HBSS 1
:
2, 1
:
20, 1
:
200 and 1
:
2000. Thirty μL of vaginal swab dilution was inoculated in duplicates in a 96 well plate with 20
000 HeLa cells per well. The plate was centrifuged at 900×g for 1 h at 37 °C. The inoculum was replaced by 100 μL preheated RPMI supplemented as above and with gentamicin (10 μg mL−1) per well and incubated for 42–46 h prior to methanol fixation, immunostaining and Chlamydia CFU quantification by Array Scan as described above.
Comp log
P
Computed log
P values were calculated in MOE.17
Chemistry
General.
All reagents and solvents were used as received from commercial suppliers, unless indicated otherwise. Triethylamine, N,N-diisopropylethylamine and pyridine were passed through activated alumina oxide and stored over activated 3 Å molecular sieves prior to use. Anhydrous solvents (THF, DMF, Et2O and DCM) were obtained from a solvent drying system and collected fresh prior to every reaction. All reactions were carried out in oven dried glassware and under a nitrogen atmosphere. TLC was performed on aluminum backed silica gel plates (median pore size 60 Å) and detected with UV light at 254 nm. Column chromatography was performed using silica gel with average particle diameter 50 μM (range 40–65 μM, pore diameter 53 Å) and eluents are given in brackets. Optical rotations were measured at 589 nm on a Polarimeter Autopol IV (Rudolph Research Analytical) at 20 °C and concentrations are given as g mL−1. High resolution mass spectra (HRMS) data was performed using a mass spectrometer, an Agilent 1290 binary LC System connected to a Agilent 6230 Accurate-Mass TOF LC/MS (ESI+); calibrated with Agilent G1969-85001 ES-TOF Reference Mix containing ammonium trifluoroacetate, purine and hexakis(1H,1H,3H-tetrafluoropropoxy)phosphazine in 90
:
10 MeCN
:
H2O. HPLC purifications were performed on a system (Gilson) equipped with a 250 × 21.5 mm Nucleodur® C18 HTEC (particle size 5 μM) column using a flow rate of 18–20 mL min−1 and detection at 214 nm. 1H and 13C NMR spectra were recorded on a Bruker Avance III 400 MHz or a Bruker Avance III HD 600 MHz spectrometer at 298 K unless otherwise stated and calibrated by using the residual peak of the solvent as the internal standard (CDCl3: δH = 7.26 ppm; δC = 77.16 ppm; DMSO-d6: δH = 2.50 ppm; δC = 39.52 ppm; CD3OD: δH = 3.31 ppm; δC = 49.00 ppm). 19F NMR spectra were recorded with proton decoupling on a 400 MHz spectrometer at 298 K with CF3CO2H as an internal standard (δF = −76.5 ppm). The asterisk * indicates peaks determined with two-dimensional proton–carbon correlation techniques (HSQC and HMBC), which were used to supplement and complete the 13C NMR spectra where signals were otherwise broadened or weak. Amide coupling reactions with propylphosphonic anhydride (T3P) were prepared by adaptation of the procedure reported by Dunetz et al.14 The following compounds were synthesized and characterized as described previously: 1,92,103,114,1313, 14, 24,1025,1331,1334,1335.24
Experimental
8-Methoxy-7-(naphthalen-1-ylmethyl)-5-oxo-N-phenyl-2,3-dihydrothiazolo[3,2-a]pyridine-3-carboxamide (5).
The carboxylic acid 4 (191 mg, 0.520 mmol) was suspended in anhydrous MeCN/EtOAc (1
:
1, 6 mL) and after cooling the suspension to ≃10 °C (NaCl/ice), pyridine (130 μL, 1.61 mmol) was added. The reaction mixture was stirred for 5 min, and aniline (71 μL, 0.79 mmol) was added, followed by dropwise addition of T3P (50% in EtOAc; 0.62 mL, 1.0 mmol). The reaction was stirred for 3 h, then allowed to warm to room temperature and stirred for 16.5 h. Additional aniline (36 μL, 0.40 mmol) was added and after 45 min additional pyridine (42 μL, 0.52 mmol) was added and stirred at room temperature for 23 h. The reaction mixture was cooled to 0 °C, quenched with 1 M HCl(aq) and extracted with DCM (×3). The organic extracts were washed successively with water and brine, dried (Na2SO4) and the solvent removed under reduced pressure. Purification by flash chromatography (SiO2, 15–100% EtOAc in heptane) and freeze-drying from H2O
:
MeCN (6
:
1) afforded the amide 5 as a white powder (134 mg, 58%). 1H-NMR (400 MHz, CDCl3) δ 10.35 (s, 1H), 7.91–7.79 (m, 3H), 7.52–7.41 (m, 5H), 7.37–7.32 (m, 1H), 7.24–7.17 (m, 2H), 7.06–6.99 (m, 1H), 5.78–5.74 (m, 1H), 5.71–5.66 (m, 1H), 4.36, 4.22 (ABq, JAB = 17.0 Hz, 2H), 4.04–3.97 (m, 1H), 3.77 (s, 3H), 3.54 (dd, J = 8.2, 11.4 Hz, 1H). 13C-NMR (100 MHz, CDCl3) δ 164.7, 161.3, 152.3, 140.5, 137.9, 137.8, 134.0, 133.1, 131.8, 128.9, 128.8, 128.04, 128.02, 126.5, 125.9, 125.6, 124.2, 123.8, 119.8, 114.4, 65.1, 60.8, 32.7, 30.5. HRMS (ESI+) (m/z): [M + H+]+ calcd. for C26H23N2O3S, 443.1423; found, 443.1432.
8-Methoxy-7-(naphthalen-1-ylmethyl)-5-oxo-N-(p-tolyl)-2,3-dihydrothiazolo[3,2-a]pyridine-3-carboxamide (6).
Carboxylic acid 4 (195 mg, 0.530 mmol) was suspended in anhydrous MeCN/EtOAc (1
:
1, 6 mL) and after cooling the suspension to ≃10 °C (NaCl/ice), pyridine (130 μL, 1.61 mmol) was added. After stirring for 5 min, p-methylaniline (70 mg, 0.65 mmol) was added, followed by dropwise addition of T3P (50% in EtOAc; 0.63 mL, 1.1 mmol). Stirred at −10 °C for 3 h and then allowed to return to rt and stirred for 16.5 h. Additional p-methylaniline (47 mg, 0.44 mmol) was added and stirred for 45 min and then pyridine (42 μL, 0.52 mmol) was added and stirred for another 4 h. The reaction mixture was cooled to 0 °C and quenched with 1 M HCl(aq) (10 mL), diluted with EtOAc and extracted with EtOAc (×3). The combined organic layers were washed successively with water and brine, then the precipitate was filtered off and the filtrate was dried (Na2SO4) and concentrated under reduced pressure. Purification by flash chromatography (SiO2, 30–100% EtOAc in heptane) and freeze-drying from H2O
:
MeCN (6
:
1) afforded the amide 6 as a white powder (107 mg, 44%). 1H-NMR (400 MHz, CDCl3) δ 10.23 (s, 1H), 7.91–7.77 (m, 3H), 7.51–7.37 (m, 5H), 7.36–7.32 (m, 1H), 7.08–7.03 (m, 2H), 5.79–5.77 (m, 1H), 5.73 (d, J = 7.9 Hz, 1H), 4.40–4.17 (m, 3H), 3.76 (s, 3H), 3.62 (dd, J = 7.9, 11.2 Hz, 1H), 2.28 (s, 3H). 13C-NMR (100 MHz, CDCl3) δ 164.3, 161.5, 152.3, 140.3, 138.1, 135.4, 134.1, 134.0, 133.1, 131.9, 129.5, 129.0, 128.12, 128.07, 126.6, 126.0, 125.6, 123.9, 119.9, 114.8, 65.2, 60.9, 32.8, 30.3, 21.0. HRMS (ESI+) (m/z): [M + H+]+ calcd. for C27H25N2O3S, 457.1579; found, 457.1590.
8-Hydroxy-7-(naphthalen-1-ylmethyl)-5-oxo-N-phenyl-2,3-dihydrothiazolo[3,2-a]pyridine-3-carboxamide (7).
BBr3 (0.035 mL, 0.020 mmol) was added slowly to a stirred solution of amide 5 (150 mg, 0.339 mmol) in DCM (5 mL) at −40 °C (dry ice/acetone) and was stirred for 6 h, meanwhile the reaction mixture was allowed to return to rt. The reaction mixture was quenched with NaHCO3 and then diluted with EtOAc. The aqueous layer was extracted with EtOAc (×2, 1 M HCl(aq) was added to acidify the aqueous layer). The combined organic layers were washed with brine, dried (Na2SO4) and the drying agent was filtered off. The drying agent (Na2SO4) was stirred in EtOAc (200 mL) for 1 h, then it was filtered off and the combined organic layers were concentrated under reduced pressure to obtain the crude product (45.6 mg). Purification by HPLC (H2O/MeCN, 0.75% formic acid, 30–100% MeCN, 25 min, 214 nm) and freeze-drying from H2O
:
MeCN afforded the amide 7 as a white powder (5.8 mg). A white precipitate in the combined aqueous layers was filtered off and after freeze-drying from H2O
:
MeCN afforded the amide 7 (8.0 mg). The total yield of the reaction: 14 mg, 10%. 1H-NMR (400 MHz, DMSO-d6) δ 10.39 (s, 1H), 8.56 (s, 1H), 8.00–7.84 (m, 3H), 7.59–7.47 (m, 5H), 7.42 (d, J = 6.9 Hz, 1H), 7.35–7.26 (m, 2H), 7.10–7.01 (m, 1H), 5.50–5.44 (m, 1H), 5.27 (s, 1H), 4.32, 4.23 (ABq, JAB = 16.8 Hz, 2H), 3.91 (dd, J = 11.8, 9.0 Hz, 1H), 3.62–3.54 (m, 1H). 13C-NMR (100 MHz, DMSO-d6) δ 166.0, 158.7, 149.8, 138.7, 136.2, 134.3, 133.5, 131.5, 131.4, 128.8, 128.6, 127.6, 127.3, 126.3, 125.8, 125.7, 124.0, 123.6, 119.0, 112.7, 64.3, 32.4, 32.2. HRMS (ESI+) (m/z): [M + Na+]+ calcd. for C25H21N2O3S, 429.1267; found, 429.1269.
6-Amino-8-methoxy-7-(naphthalen-1-ylmethyl)-5-oxo-N-phenyl-2,3-dihydrothiazolo[3,2-a]pyridine-3-carboxamide (8).
NaNO2 (27 mg, 0.39 mmol) was added to a stirred solution amide 5 (119 mg, 0.270 mmol) in DCM (5 mL). A balloon with O2 gas was connected via septum, TFA (352 μL, 4.58 mmol) was added dropwise and the reaction mixture was stirred at rt for 1 h. The reaction was quenched with sat. NaHCO3(aq) and extracted with DCM (×3). The combined organic layers were dried (Na2SO4) and concentrated under reduced pressure. The product was partially purified by flash chromatography (SiO2, 20–100% EtOAc in heptane) to afford the crude intermediate (82 mg). The crude (66 mg) was dissolved in acetic acid and freshly activated Zn dust (45 mg, 0.69 mmol) was added in portions to control the temperature of the reaction and stirred at rt for 15 h. Additional activated Zn dust (24 mg, 0.37 mmol) was added and the reaction mixture was stirred at rt for 24 h and additional zinc dust (26 mg, 0.40 mmol) was added. After 48 h, the reaction mixture was filtered through a pad of celite, washing with DCM, and quenched with sat. NaHCO3(aq). The mixture was extracted with DCM (×2), the combined organic layers were washed with NaHCO3 and then brine, dried (Na2SO4) and concentrated under reduced pressure. Purification by flash chromatography (SiO2, 30–100% EtOAc in heptane) and freeze-drying from H2O
:
MeCN (10
:
1) afforded the amide 8 as a grey powder (30 mg, 30%). 1H-NMR (400 MHz, CDCl3) δ 10.40 (s, br, 1H), 8.17 (d, J = 8.4 Hz, 1H), 7.93–7.88 (m, 1H), 7.77 (d, J = 8.3 Hz, 1H), 7.64–7.52 (m, 4H), 7.39–7.28 (m, 3H), 7.17–7.07 (m, 2H), 5.85 (d, J = 7.5 Hz, 1H), 4.38, 4.31 (ABq, JAB = 16.5 Hz, 2H), 4.20 (d, J = 11.3 Hz, 1H), 3.99 (s br, 2H), 3.68 (dd, J = 7.7, 11.3 Hz, 1H), 3.58 (s, 3H). 13C-NMR (100 MHz, CDCl3) δ 164.7, 156.3, 139.1, 138.1, 134.1, 133.2, 132.1, 131.8, 129.1*, 127.8, 126.6, 126.1, 125.7, 124.7, 124.6, 124.5, 124.1, 123.4, 120.1, 65.5, 61.4, 30.3, 28.2. HRMS (ESI+) (m/z): [M + H+]+ calcd. for C26H24N3O3S, 458.1532; found, 458.1523. *Note: overlap of two non-identical aromatic carbons, can be seen in HSQC.
6-Amino-8-methoxy-7-(naphthalen-1-ylmethyl)-5-oxo-N-(p-tolyl)-2,3-dihydrothiazolo[3,2-a]pyridine-3-carboxamide (9).
NaNO2 (18 mg, 0.26 mmol) was added to a stirred mixture of amide 6 (78 mg, 0.17 mmol), in DCM (5 mL). A balloon with O2 gas was connected via septum, TFA (224 μL, 2.92 mmol) was added dropwise and the reaction mixture was stirred at rt for 7 h. The reaction was quenched with sat. NaHCO3(aq) and extracted with DCM (×3). The combined organic layers were washed with sat. NaHCO3(aq) (×2), dried (Na2SO4) and concentrated under reduced pressure. The crude (86 mg) was dissolved in acetic acid (4 mL) and freshly activated Zn dust (58 mg, 0.89 mmol) was added in portions to control the temperature of the reaction and the reaction mixture was stirred at rt for 15 h. Additional Zn dust (56 mg, 0.86 mmol) were added and stirred for another 7 h. Additional Zn dust (28 mg, 0.43 mmol) was added and the reaction mixture was stirred at rt for another 19 h. The reaction mixture was filtered through a pad of celite, washed with DCM, and quenched with sat. NaHCO3(aq). The mixture was extracted with DCM (×2) and the combined organic layers were washed with sat. NaHCO3(aq) and then brine, dried (Na2SO4) and concentrated under reduced pressure. Purification by flash chromatography (SiO2, 30–100% EtOAc in Heptane) and freeze-drying from H2O
:
MeCN afforded the amine 9 as a grey powder (28 mg, 35%). 1H-NMR (400 MHz, CDCl3) δ 10.29 (br s, 1H), 8.17 (d, J = 8.3 Hz, 1H), 7.90 (d, J = 7.8 Hz, 1H), 7.77 (d, J = 8.2 Hz, 1H), 7.64–7.52 (m, 2H), 7.50–7.45 (m, 2H), 7.40–7.33 (m, 1H), 7.16–7.08 (m, 3H), 5.84 (d, J = 7.6 Hz, 1H), 4.38, 4.30 (ABq, JAB = 16.5 Hz, 2H), 4.20 (d, J = 11.2 Hz, 1H), 3.98 (br s, 2H), 3.67 (dd, J = 7.6, 11.2 Hz, 1H), 3.58 (s, 3H), 2.31 (s, 3H). 13C-NMR (100 MHz, CDCl3) δ 164.6, 156.3, 139.1, 135.5, 134.0, 133.1, 132.1, 131.8, 129.5, 129.0, 127.8, 126.6, 126.1, 125.7, 124.6, 124.1, 123.4, 120.1, 65.5, 61.4, 30.3, 28.2, 21.0. HRMS (ESI+) (m/z): [M + H+]+ calcd. for C27H26N3O3S, 472.1688; found, 472.1687.
Methyl (R)-7-(2,3-dimethylbenzyl)-8-methoxy-5-oxo-2,3-dihydrothiazolo[3,2-a]pyridine-3-carboxylate (15).
TFA (1.925 mL, 25.07 mmol) was added dropwise to a mixture of 2,3-dimethyl Meldrum's acid 14 (18.311 g, 63.074 mmol) and 13 (4.732 g, 25.01 mmol) in DCE (60 mL) and heated in MWI at 120 °C for 3 min (run in 6 batches). The reaction mixture was allowed to cool to rt, then diluted with EtOAc and quenched with sat. NaHCO3(aq). The aqueous layer was extracted with EtOAc (×3) and the combined organic layers were washed successively with H2O and brine, dried (Na2SO4), and concentrated under reduced pressure. Purification by flash chromatography (SiO2, EtOAc in heptane, 30–100%) afforded the methyl ester 15 as a yellow-orange powder (7.2 g, 80%). 1H-NMR (400 MHz, CDCl3) δ 7.09–7.01 (m, 2H), 6.98–6.94 (m, 1H), 5.69 (s, 1H), 5.55 (dd, J = 8.3, 2.2 Hz, 1H), 3.90–3.78 (m, 5H), 3.77–3.69 (m, 4H), 3.57 (dd, J = 11.7, 2.2 Hz, 1H), 2.28 (s, 3H), 2.10 (s, 3H). 13C-NMR (100 MHz, CDCl3) δ 168.4, 160.5, 152.1, 138.2, 137.4, 137.1, 135.3, 129.0, 128.4, 125.8, 114.9, 63.2, 60.7, 53.4, 34.0, 32.5, 20.8, 15.5. Optical rotation: [α]2D = −32 (c 0.001, CHCl3). HRMS (ESI+) (m/z): [M + H+]+ calcd. for C19H22NO4S, 360.1264; found, 360.1274.
7-(2,3-Dimethylbenzyl)-8-methoxy-5-oxo-N-phenyl-2,3-dihydrothiazolo[3,2-a]pyridine-3-carboxamide (16).
Methyl ester 15 (912 mg, 2.54 mmol) was dissolved in THF (50 mL) and 1 M LiOH(aq) (5 mL) was added and the reaction mixture was stirred at for 15 h. Diluted the reaction mixture with EtOAc extracted the with 1 M NaOH(aq) (×3). The combined aqueous layers were cooled on ice and acidified with 1 M and conc. HCl until ∼pH 1 and extracted with EtOAc (×4). The formed precipitate (product) was filtered off from the organic layer. The organic layer was dried washed with brine and concentrated under reduced pressure and then freeze dried the crude (1.299 g). The crude (149 mg) was suspended in anhydrous MeCN/EtOAc (1
:
1, 4 mL) and after cooling the suspension to ≃10 °C (NaCl/ice), pyridine (0.11 mL, 1.4 mmol) was added. After stirring for 5 min, aniline (60 μL, 0.66 mmol) was added, followed by dropwise addition of T3P (50% in EtOAc; 0.52 mL, 1.7 mmol). The reaction mixture was stirred at −10 °C for 1 h, then allowed to return to rt and stirred for 23 h. The reaction was cooled to 0 °C, then quenched with 1 M HCl(aq), diluted with EtOAc and extracted with EtOAc (×3). The combined organic layers were washed successively with H2O (1 mL 1 M HCl(aq) was added to aid separation) and brine, dried (Na2SO4), and concentrated under reduced pressure. Purification by flash chromatography (SiO2, 25–100% EtOAc in heptane) and freeze-drying from H2O
:
MeCN (6
:
1) afforded the amide 16 as a white powder (101 mg, 82%). 1H-NMR (600 MHz, CDCl3) δ 10.43 (s, 1H), 7.57–7.53 (m, 2H), 7.30–7.25 (m, 2H), 7.11–7.04 (m, 3H), 6.95 (d, J = 7.3 Hz, 1H), 5.81 (d, J = 8.0 Hz, 1H), 5.78 (s, 1H), 4.21 (d, J = 11.2 Hz, 1H), 3.90, 3.82 (ABq, JAB = 17.3 Hz, 2H), 3.71 (s, 3H), 3.66 (dd, J = 11.2, 8.0 Hz, 1H), 2.29 (s, 3H), 2.10 (s, 3H). 13C-NMR (151 MHz, CDCl3) δ 164.5, 161.6, 152.9, 140.3, 138.4, 138.0, 137.5, 135.3, 135.0, 129.2, 129.0, 128.4, 125.9, 124.5, 120.0, 114.2, 65.3, 60.7, 33.9, 30.4, 20.8, 15.6. HRMS (ESI+) (m/z): [M + H+]+ calcd. for C24H25N2O3S, 421.1580; found, 421.1589.
7-(2,3-Dimethylbenzyl)-8-methoxy-5-oxo-N-(p-tolyl)-2,3-dihydrothiazolo[3,2-a]pyridine-3-carboxamide (17).
Methyl ester 15 (912 mg, 2.54 mmol) was dissolved in THF (50 mL) and 1 M LiOH(aq) (5 mL) was added and the reaction mixture was stirred at for 15 h. Diluted the reaction mixture with EtOAc extracted the with 1 M NaOH(aq) (×3). The combined aqueous layers were cooled on ice and acidified with 1 M and conc. HCl until ∼pH 1 and extracted with EtOAc (×4). The formed precipitate (product) was filtered off from the organic layer. The organic layer was dried, washed with brine and concentrated under reduced pressure and freeze dried (1.299 g). A portion (149 mg) was suspended in anhydrous MeCN/EtOAc (1
:
1, 4 mL) and after cooling the suspension to ≃10 °C (NaCl/ice), pyridine (0.11 mL, 1.4 mmol) was added. After stirring for 15 min, p-methylaniline (70 mg, 0.65 mmol) was added and followed by dropwise addition of T3P (50% in EtOAc; 0.51 mL, 0.86 mmol). Stirred at −10 °C for 1 h and then allowed to return to rt and stirred for 23 h. The reaction mixture was cooled to 0 °C and quenched with 1 M HCl(aq), diluted with EtOAc and extracted with EtOAc (×3). The combined organic layers were washed successively with water and brine, dried (Na2SO4) and concentrated under reduced pressure. Purification by flash chromatography (SiO2, 25–100% EtOAc in heptane) and freeze-drying from H2O
:
MeCN afforded the amide 17 as an off-white powder (49 mg, 39%). 1H-NMR (400 MHz, CDCl3) δ 10.28 (s br, 1H), 7.45–7.40 (m, 2H), 7.12–7.03 (m, 4H), 6.98–6.93 (m, 1H), 5.80–5.73 (m, 2H), 4.21 (d, J = 11.2 Hz, 1H), 3.90, 3.82 (ABq, JAB = 17.3 Hz, 2H), 3.72 (s, 3H), 3.64 (dd, J = 7.9, 11.2 Hz, 1H), 2.31–2.27 (m, 6H), 2.10 (s, 3H). 13C-NMR (100 MHz, CDCl3) δ 164.3, 161.7, 152.6, 140.0, 138.2, 137.5, 135.4, 135.3, 135.0, 134.0, 129.5 (2C), 129.1, 128.4, 125.9, 120.0 (2C), 114.3, 65.1, 60.7, 33.9, 30.3, 20.9, 20.8, 15.6. HRMS (ESI+) (m/z): [M + Na+]+ calcd. for C25H26N2NaO3S, 457.1555; found, 457.1572.
7-(2,3-Dimethylbenzyl)-N-(3-fluoro-5-methylphenyl)-8-methoxy-5-oxo-2,3-dihydrothiazolo[3,2-a]pyridine-3-carboxamide (18).
Methyl ester 15 (4.705 g, 13.09 mmol) was dissolved in THF (130 mL) and 1 M LiOH(aq) (26 mL) was added and stirred at rt for 17 h. The reaction mixture was cooled on ice and quenched with 1 M HCl(aq) (35 mL), extracted with EtOAc (×4), and combined organic layers were washed with brine. The formed precipitate (product) was filtered off from the organic layer. The organic layer was dried (Na2SO4) and concentrated under reduced pressure to yield the crude (4.3 g). The crude (3.09 g) was suspended in DCM (60 mL) and 3-fluoro-5-methylaniline (1.20 mL, 10.7 mmol), N,N-diisopropyl ethyl amine (3.09 mL, 17.9 mmol) and HATU (4.25 g, 11.2 mmol) were added and stirred at rt under for 2 h. The reaction mixture was cooled on ice and 0.5 M HCl(aq) (100 mL) was added in portions. The mixture was diluted with EtOAc and the aqueous layer was extracted with EtOAc (×2). The combined organic layers were washed with 0.5 M HCl(aq) (×2), H2O (×2, added 1 M HCl(aq) to aid separation), and brine (×4), then dried (Na2SO4) and concentrated under reduced pressure. Purification by flash chromatography (SiO2, 20–100% EtOAc in heptane) afforded the product as a yellow foam (4.02 g, 95%). To obtain the product in crystalline form the product was recrystallized. The product was combined with a previous batch and the product (4.935 g) was recrystallized from boiling EtOH and dried under vacuum, which afforded the amide 18 as white crystals (3.329 g). 1H NMR (400 MHz, CDCl3) δ 10.50 (s br, 1H), 7.37–7.31 (m, 1H), 7.12–7.02 (m, 2H), 7.00–6.92 (m, 2H), 6.60 (d, J = 9.2 Hz, 1H), 5.82–5.74 (m, 2H), 4.21 (d, J = 11.2 Hz, 1H), 3.91, 3.82 (ABq, JAB = 17.3 Hz, 2H), 3.72 (s, 3H), 3.67 (dd, J = 8.0, 11.2 Hz, 1H), 2.30–2.26 (m, 6H), 2.10 (s, 3H). 13C NMR (100 MHz, CDCl3) δ 164.7, 162.9 (d, JCF = 246.3 Hz), 161.7, 152.9, 140.8 (d, JCF = 9.3 Hz), 140.0, 139.0 (d, JCF = 11.7 Hz), 138.4, 137.6, 135.3, 135.0, 129.2, 128.4, 125.9, 116.1 (d, JCF = 2.4 Hz), 114.4, 111.9 (d, JCF = 21.4 Hz), 104.7 (d, JCF = 26.7 Hz), 65.2, 60.8, 34.0, 30.2, 21.5 (d, JCF = 2.0 Hz), 20.8, 15.6. 19F NMR (376 MHz, CDCl3) δ −113.00. HRMS (ESI+) (m/z): [M + H+]+ calcd. for C25H26FN2O3S, 453.1642; found, 453.1656.
7-(2,3-Dimethylbenzyl)-8-methoxy-3-(3-phenyl-1,2,4-oxadiazol-5-yl)-2,3-dihydrothiazolo[3,2-a]pyridin-5-one (19).
Methyl ester 15 (578 mg, 1.61 mmol) was dissolved in THF and 1 M LiOH(aq) (3.3 mL) was added and stirred at rt for 22 h. 1 M HCl(aq) (5 mL) was added to the reaction mixture and then extracted with EtOAc (×3). The combined organic layers were washed with brine and the formed precipitate (product) was filtered off from the organic layer. The organic layer was dried (Na2SO4) and concentrated under reduced pressure to yield the acid (445 mg), which was used without purification. A portion (188 mg) was suspended in anhydrous DMF (4.3 mL) and benzamidoxime (89 mg, 0.65 mmol), TBTU (209 mg, 0.65 mmol), and diisopropyl ethyl amine (221 μL, 1.28 mmol) were added and the reaction mixture heated in MWI at 170 °C for 12 min. The reaction mixture was diluted with EtOAc and 0.5 M HCl(aq) and extracted with EtOAc (×3). The combined organic layers were washed successively with KHSO4(aq) (3% w/v) (×2), H2O and brine, dried (Na2SO4) and concentrated under reduced pressure. Purification by flash chromatography (SiO2, 30–100% EtOAc in Heptane) and freeze-drying from H2O
:
MeCN (6
:
1) afforded the 1,2,4-oxadiazole 19 as a yellow powder (139 mg, 46%). 1H-NMR (400 MHz, CDCl3) δ 8.06–8.01 (m, 2H), 7.53–7.43 (m, 3H), 7.11–7.04 (m, 2H), 7.02–6.97 (m, 1H), 6.40 (dd, J = 1.7, 7.8 Hz, 1H), 5.76–5.74 (m, 1H), 3.97 (dd, J = 7.8, 11.8 Hz, 1H), 3.94–3.83 (m, 2H), 3.77–3.71 (m, 4H), 2.30 (s, 3H), 2.13 (s, 3H). 13C-NMR (100 MHz, CDCl3) δ 175.1, 168.9, 160.4, 152.7, 137.6, 137.52, 137.49, 135.3, 135.2, 131.6, 129.2, 129.0, 128.4, 127.8, 126.4, 125.9, 115.4, 60.9, 58.3, 34.2, 34.1, 20.8, 15.6. HRMS (ESI+) (m/z): [M + H+]+ calcd. for C25H24N3O3S, 446.1532; found, 446.1531.
6-Amino-7-(2,3-dimethylbenzyl)-8-methoxy-3-(3-phenyl-1,2,4-oxadiazol-5-yl)-2,3-dihydrothiazolo[3,2-a]pyridin-5-one (20).
NaNO2 (18 mg, 0.26 mmol) was added to a stirred mixture of 1,2,4-oxadiazole 19 (110 mg, 0.247 mmol) in DCM (5 mL). A balloon with O2 gas was connected via septum, TFA (323 μL, 4.21 mmol) was added dropwise and the mixture was stirred at room temperature for 1 h. The reaction was quenched with sat. NaHCO3(aq) and extracted with DCM (×3). The combined organic layers were dried (Na2SO4) and concentrated under reduced pressure. The resulting intermediate (121 mg) was dissolved in acetic acid (4 mL) and freshly activated Zn dust (113 mg, 1.73 mmol) was added in portions to control the temperature of the reaction and it was stirred at rt for 19 h. The reaction mixture was filtered through a pad of celite, washed with DCM, and quenched with sat. NaHCO3(aq). The mixture was extracted with DCM (×2) and the combined organic layers were washed successively with sat. NaHCO3(aq) and brine, dried (Na2SO4) and concentrated under reduced pressure. Purification by flash chromatography (SiO2, 30–100% EtOAc in heptane) and freeze-drying from H2O
:
MeCN (10
:
1) afforded the amine 20 as a grey powder (68 mg, 60%). 1H-NMR (400 MHz, CDCl3) δ 8.09–8.04 (m, 2H), 7.53–7.43 (m, 3H), 7.09–6.98 (m, 2H), 6.86 (d, J = 7.4, 1H), 6.47 (dd, J = 1.8, 7.5 Hz, 1H), 4.07–3.81 (m, 5H), 3.73 (dd, J = 1.8, 11.8 Hz, 1H), 3.59 (s, 3H), 2.33 (s, 3H), 2.30 (s, 3H). 13C-NMR (100 MHz, CDCl3) δ 175.3, 168.8, 155.7, 138.4, 137.3, 135.4, 134.4, 133.2, 131.5, 128.9 (2C), 128.7, 127.7 (2C), 126.4, 125.9, 124.5, 124.0, 122.0, 61.3, 58.6, 34.1, 29.3, 20.8, 15.3. HRMS (ESI+) (m/z): [M + H+]+ calcd. for C25H25N4O3S, 461.1641; found, 461.1639.
6-Amino-7-(2,3-dimethylbenzyl)-8-methoxy-5-oxo-N-phenyl-2,3-dihydrothiazolo[3,2-a]pyridine-3-carboxamide (21).
NaNO2 (19 mg, 0.28 mmol) was added to a stirred mixture of amide 16 (80 mg, 0.19 mmol) in DCM (5 mL). A balloon with O2 gas was connected via septum, TFA (247 μL, 3.22 mmol) was added dropwise and the mixture was stirred at room temperature for 2.5 h. The reaction was quenched with sat. NaHCO3(aq) and extracted with DCM (×3). The combined organic layers were dried (Na2SO4) and concentrated under reduced pressure. The resulting intermediate (67 mg) was dissolved in acetic acid (4 mL) and freshly activated Zn dust (66 mg, 1.0 mmol) was added in portions to control the temperature of the reaction, and it was stirred at rt for 16 h. Additional Zn dust (32 mg, 0.49 mmol) was added and stirred at rt for 4 h. The reaction mixture was filtered through a pad of celite, washed with DCM, and quenched with sat. NaHCO3(aq). The mixture was extracted with DCM (×2) and the combined organic layers were washed successively with sat. NaHCO3(aq) and brine, dried (Na2SO4) and concentrated under reduced pressure. Purification by flash chromatography (SiO2, 30–100% EtOAc in heptane), and freeze-drying from H2O
:
MeCN (10
:
1) afforded the amine 21 as a grey powder (25 mg, 30%). 1H-NMR (400 MHz, CDCl3) δ 10.41 (bs, 1H) 7.61–7.55 (m, 2H), 7.34–7.28 (m, 2H), 7.12–7.04 (m, 2H), 7.03–6.97 (m, 1H), 6.80 (d, J = 7.5 Hz, 1H), 5.85 (d, J = 7.6 Hz, 1H), 4.19 (d, J = 11.2 Hz, 1H), 4.01–3.78 (m, 4H), 3.66 (dd, J = 7.6, 11.2 Hz, 1H), 3.57 (s, 3H), 2.33 (s, 3H), 2.29 (s, 3H). 13C-NMR (100 MHz, CDCl3) δ 164.8, 156.3, 139.1, 138.1, 137.4, 135.4, 134.3, 132.9, 129.0, 128.8, 126.0, 125.6, 124.5, 124.4, 120.1, 65.5, 61.3, 30.3, 29.3, 20.8, 15.3. HRMS (ESI+) (m/z): [M + H+]+ calcd. for C24H26N3O3S, 436.1689; found, 436.1691.
6-Amino-7-(2,3-dimethylbenzyl)-8-methoxy-5-oxo-N-(p-tolyl)-2,3-dihydrothiazolo[3,2-a]pyridine-3-carboxamide (22).
NaNO2 (10 mg, 0.14 mmol) was added to a stirred mixture of amide 17 (34 mg, 0.078 mmol) in DCM (5 mL). A balloon with O2 gas was connected via septum, TFA (101 μL, 1.32 mmol) was added dropwise and the mixture was stirred at room temperature for 4 h. The reaction was quenched with sat. NaHCO3(aq) and extracted with DCM (×3). The combined organic layers were washed with sat. NaHCO3(aq) (×2), dried (Na2SO4) and concentrated under reduced pressure. The resulting intermediate was dissolved in acetic acid (4 mL) and freshly activated Zn dust (40 mg, 0.61 mmol) was added in portions to control the temperature of the reaction and it was stirred at rt for 15 h. Additional Zn dust (45 mg, 0.69 mmol) were added and after 8 h of additional stirring, the reaction mixture was filtered through a pad of celite, washed with DCM, and quenched with sat. NaHCO3(aq). The mixture was extracted DCM (×2) and the combined organic layers were washed successively with sat. NaHCO3(aq) and brine, dried (Na2SO4) and concentrated under reduced pressure. Purification by flash chromatography (SiO2, 30–100% EtOAc in heptane) and freeze-drying from H2O
:
MeCN (10
:
1) afforded the amine 22 as an off-white powder (5.8 mg, 17%). 1H-NMR (400 MHz, CDCl3) δ 10.29 (br s, 1H), 7.49–7.43 (m, 2H), 7.14–7.08 (m, 2H), 7.08–7.03 (m, 1H), 7.03–6.96 (m, 1H), 6.79 (d, J = 7.5 Hz, 1H), 5.84 (d, J = 7.6 Hz, 1H), 4.20 (d, J = 11.2 Hz, 1H), 4.05–3.77 (m, 4H), 3.66 (dd, J = 7.6, 11.2 Hz, 1H), 3.57 (s, 3H), 2.34–2.27 (9H). 13C-NMR (100 MHz, CDCl3) δ 164.6, 156.4, 139.1, 137.4, 135.5, 135.4, 134.3, 134.1, 132.9, 129.6, 128.8, 126.0, 125.6, 124.61, 124.57, 120.1, 65.5, 61.3, 30.3, 29.4, 21.0, 20.9, 15.4. HRMS (ESI+) (m/z): [M + H+]+ calcd. for C25H28N3O3S, 450.1845; found, 450.1848.
6-Amino-7-(2,3-dimethylbenzyl)-N-(3-fluoro-5-methylphenyl)-8-methoxy-5-oxo-2,3-dihydrothiazolo[3,2-a]pyridine-3-carboxamide (23).
NaNO2 (13 mg, 0.19 mmol) was added to a stirred mixture of amide 18 (51 mg, 0.11 mmol) in DCM (5 mL). A balloon with O2 gas was connected and TFA (147 μL, 1.92 mmol) was added dropwise and the mixture was stirred at room temperature for 6 h. The reaction was quenched with sat. NaHCO3(aq) and extracted with DCM (×3). The combined organic layers were washed with sat. NaHCO3(aq) (×2), dried (Na2SO4) and concentrated under reduced pressure. The crude was dissolved in acetic acid (4 mL) and freshly activated Zn dust (59 mg, 0.90 mmol) was added in portions to control the temperature of the reaction and it was stirred at rt for 15 h. Additional Zn dust (23 mg, 0.35 mmol) were added and after 7 h of additional stirring, the reaction mixture was filtered through a pad of celite, washed with DCM, and quenched with sat. NaHCO3(aq). The mixture was extracted DCM (×2) and the combined organic layers were washed successively with sat. NaHCO3(aq) and brine, dried (Na2SO4) and concentrated under reduced pressure. Purification by flash chromatography (SiO2, 30–100% EtOAc in heptane) and freeze-drying from H2O
:
MeCN (10
:
1) afforded the amine 23 as a brown powder (20 mg, 39%). 1H-NMR (400 MHz, CDCl3) δ 10.48 (br s, 1H), 7.36–7.30 (m, 1H), 7.08–6.96 (m, 3H), 6.81–6.77 (m, 1H), 6.65–6.58 (m, 1H), 5.82 (d, J = 7.7 Hz, 1H), 4.17 (d, J = 11.3 Hz, 1H), 4.02–3.77 (m, 4H), 3.66 (dd, J = 7.7, 11.3 Hz, 1H), 3.57 (s, 3H), 2.32 (s, 3H), 2.31–2.28 (m, 6H). 13C-NMR (100 MHz, CDCl3) δ 164.9, 163.0 (d, JCF = 244.1 Hz), 156.4, 140.8 (d, JCF = 8.9 Hz), 139.3, 139.1 (d, JCF = 11.5 Hz), 137.5, 135.4, 134.2, 132.9, 128.8, 126.0, 125.8, 124.6, 124.5, 116.1 (d, JCF = 2.6 Hz), 111.9 (d, JCF = 21.2 Hz), 104.7 (d, JCF = 26.5 Hz), 65.5, 61.3, 30.2, 29.4, 21.5 (d, JCF = 2.0 Hz), 20.9, 15.4. 19F NMR (376 MHz, CDCl3) δ −113.29. HRMS (ESI+) (m/z): [M + H+]+ calcd. for C25H27FN3O3S, 468.1751; found, 468.1751.
tert-Butyl (8-methoxy-7-(naphthalen-1-ylmethyl)-5-oxo-3,5-dihydro-2H-thiazolo[3,2-a]pyridin-3-yl)carbamate (10).
Carboxylic acid 4 (500 mg, 1.36 mmol) was suspended in t-BuOH (10 ml). DPPA (308 μl, 1.43 mmol) and TEA (199 μl, 1.43 mmol) were added and the mixture was heated to 85 °C for 2 hours. Filtered and concentrated. The residue was purified using column chromatography on silica gel (heptane
:
EtOAc 83
:
17 ≥ 0
:
100) to give BOC-protected amine 10 as a yellow foam (358 mg, 60%). 1H-NMR (400 MHz, CDCl3) δ 7.87–7.78 (m, 2H), 7.77 (d, J = 7.9 Hz, 1H), 7.48–7.43 (m, 2H), 7.40 (dd, J = 8.4, 6.9 Hz, 1H), 7.30 (dd, J = 6.9, 0.9 Hz, 1H), 6.47 (td, J = 6.8, 0.9 Hz, 1H), 5.67 (s, 1H), 5.56 (d, J = 7.2 Hz, 1H), 4.23 (s, 2H), 3.71 (s, 3H), 3.63 (dd, J = 11.9, 6.5 Hz, 1H), 3.39 (dd, J = 11.9, 1.7 Hz, 1H), 1.42 (s, 9H); 13C-NMR (100 MHz, CDCl3) δ 159.6, 154.1, 151.6, 138.3, 136.4, 134.0, 133.4, 131.8, 128.8, 127.9, 127.8, 126.4, 125.8, 125.5, 123.8, 115.6, 80.8, 69.1, 60.7, 36.0, 32.7, 28.3; HRMS (ESI+) (m/z): [M + H+]+ calcd. for C24H27N2O4S+, 439.1686; found, 439.1691.
3-Amino-8-methoxy-7-(naphthalen-1-ylmethyl)-2H-thiazolo[3,2-a]pyridin-5(3H)-one (11).
BOC-protected amine 10 (345 mg, 0.787 mmol) was dissolved in a mixture of DCM (9 ml) and TFA (3 ml) and stirred at room temperature for 2 hours. Quenched with NaHCO3 (sat. aq) and extracted with EtOAc. Dried (Na2SO4), filtered and concentrated. Purified using column chromatography on silica gel (EtOAc
:
MeOH 99
:
1 ≥ 92
:
8) to give amine 11 as a yellow foam (85 mg, 32%). 1H-NMR (400 MHz, CDCl3) δ 7.89–7.80 (m, 2H), 7.78 (d, J = 8.0 Hz, 1H), 7.51–7.43 (m, 2H), 7.41 (t, J = 7.8 Hz, 1H), 7.32 (d, J = 6.8 Hz, 1H), 5.88 (dd, J = 7.3, 1.7 Hz, 1H), 5.69 (s, 1H), 4.26 (s, 2H), 3.73 (s, 3H), 3.66 (dd, J = 12.0, 7.1 Hz, 1H), 3.16 (dd, J = 12.0, 1.6 Hz, 1H), 2.48 (s, 2H); 13C-NMR (100 MHz, CDCl3) δ 160.6, 151.3, 137.3, 136.4, 134.0, 133.5, 131.9, 128.9, 127.9, 127.9, 126.4, 125.8, 125.5, 123.9, 115.3, 72.9, 60.8, 35.7, 32.7; HRMS (ESI+) (m/z): [M + H+]+ calcd. for C19H19N2O2S+, 339.1162; found, 339.1167.
8-Methoxy-7-(naphthalen-1-ylmethyl)-3-(4-phenyl-1H-1,2,3-triazol-1-yl)-2H-thiazolo[3,2-a]pyridin-5(3H)-one (12).
Amine 11 (60 mg, 0.18 mmol) was dissolved in dry MeOH (1 ml) and K2CO3 (54 mg, 0.39 mmol) followed by CuSO4 (5.7 mg, 0.036 mmol) were added. Imidazole-1-sulfonyl azide·H2SO4 (101 mg, 0.372 mmol) was added and the reaction was heated to 50 °C for 40 h. Diluted with EtOAc and washed with brine. The water layers were extracted with EtOAc and the organic layers were dried (Na2SO4), filtered and concentrated. The product was partially purified by column chromatography (SiO2, heptane
:
EtOAc 90
:
10 ≥ 20
:
80, Rf = 0.3), then dissolved in DMF (0.5 ml) and phenyl acetylene (4.2 μl, 0.038 mmol), sodium ascorbate (1.5 mg, 0.0077 mmol), CuSO4 (0.61 mg, 0.0038 mmol) and H2O (50 μl) were added. The mixture was stirred for 4 hours and then diluted with EtOAc and washed with brine. The organic layer was dried (Na2SO4), filtered and concentrated under reduced pressure. Purified using preparative HPLC (H2O
:
MeCN 90
:
10 ≥ 0
:
100 with 0.75% formic acid over 40 minutes) to give 1,2,3-triazole 12 as a yellow solid (4 mg, 45%). 1H-NMR (400 MHz, CDCl3) δ 8.20 (s, 1H), 7.89–7.84 (m, 1H), 7.82–7.77 (m, 4H), 7.49–7.45 (m, 2H), 7.44–7.37 (m, 3H), 7.35–7.28 (m, 3H), 5.68 (t, J = 1.1 Hz, 1H), 4.35 (d, J = 12.7 Hz, 1H), 4.33, 4.22 (ABq, JAB = 17.2 Hz, 2H), 4.05 (dd, J = 12.5, 7.1 Hz, 1H), 3.79 (s, 3H); 13C-NMR (100 MHz, CDCl3) δ 160.0, 153.4, 148.1, 138.6, 137.2, 134.1, 133.0, 131.9, 130.2, 129.1, 129.0, 128.9, 128.5, 128.2, 128.1, 126.6, 126.0, 125.7, 123.9, 121.0, 115.4, 73.0, 61.0, 33.9, 33.0; HRMS (ESI+) (m/z): [M + H+]+ calcd. for C27H23N4O2S+, 467.1536; found, 467.1540.
8-cyclopropyl-7-(naphthalen-1-ylmethyl)-5-oxo-N-phenyl-3,5-dihydro-2H-thiazolo[3,2-a]pyridine-3-carboxamide.
Prepared according to previously published procedures in ref. 10.
8-(Isopropylamino)-7-(naphthalen-1-ylmethyl)-5-oxo-N-phenyl-2,3-dihydrothiazolo[3,2-a]pyridine-3-carboxamide (26).
Ammonium salt 25 (70 mg, 0.16 mmol) was combined with NaBH(OAc)3 (100 mg, 0.47 mmol) and TFA (60 μL, 0.78 mmol) at −15 °C, then DCM (500 μL) added, followed by acetone (13.8 μL, 0.19 mmol). The mixture was stirred and allowed to warm to rt overnight. Additional acetone (1 μL, 0.01 mmol) and NaBH(OAc)3 (11 mg, 0.05 mmol) were added and the mixture stirred for another 2 h. The mixture was then acidified with 1 M HCl(aq) and extracted with EtOAc (×2), which was then washed with NaHCO3(aq). The combined organics were dried (MgSO4) and filtered, then the solvent removed under reduced pressure. The residue was re-dissolved in THF (1.48 mL) and MeOH (371 μL), then 1 M LiOH(aq) (800 μL, 0.80 mmol) added and the mixture stirred at rt for 1 h. Partitioned (EtOAc/1 M HCl(aq)) and the aqueous re-extracted (×2). The combined organics were dried over MgSO4, filtered and the solvent removed under reduced pressure. The residue was re-dissolved in DCM and a large excess of Eth. HCl(g) added. The mixture was allowed to stand overnight at −20 °C, then most of the Et2O removed by pipette, and the residue triturated with fresh Et2O. The material was dried under vacuum, then dissolved in DMF (500 μL) and HATU (51 mg, 0.13 mmol), aniline (19 μL, 0.21 mmol) and DIPEA (36 μL, 0.21 mmol 2.08) added. After stirring for 1 h at rt, the mixture was partitioned (EtOAc/dil. HCl(aq)) and the aqueous re-extracted (×2), dried (MgSO4), filtered and the solvent removed under reduced pressure. Purified by HPLC as described in the general procedure and freeze-dried from H2O
:
MeCN to give isopropyl amine 26 as a white solid (32 mg, 43%). 1H NMR (600 MHz, DMSO-d6) δ 10.36 (s, 1H), 7.99–7.93 (m, 1H), 7.90–7.82 (m, 2H), 7.56–7.48 (m, 5H), 7.43 (d, J = 7.0 Hz, 1H), 7.30 (apparent t, J = 7.7 Hz, 2H), 7.05 (apparent t, J = 7.4 Hz, 1H), 5.48 (d, J = 8.9 Hz, 1H), 5.16 (s, 1H), 4.41, 4.29 (ABq, JAB = 17.1 Hz, 2H), 3.87 (dd, J = 11.9, 9.1 Hz, 1H), 3.62 (br d, J = 6.1 Hz, 1H), 3.50 (d, J = 12.0 Hz, 1H), 3.32–3.25 (m, 1H), 1.13 (d, J = 6.2 Hz, 3H), 1.11 (d, J = 6.2 Hz, 3H). 13C NMR (151 MHz, DMSO) δ 166.0, 159.3, 154.5, 146.0, 138.7, 134.7, 133.5, 131.5, 128.8, 128.6, 127.9, 127.3, 126.2, 125.8, 125.7, 124.2, 123.5, 120.8, 119.0, 112.8, 64.7, 48.9, 33.4, 31.5, 23.45, 23.42. HRMS (ESI+) (m/z): [M + H+]+ calcd. for C28H28N3O2S, 470.1897; found, 470.1895.
8-(Dimethylamino)-7-(naphthalen-1-ylmethyl)-5-oxo-N-phenyl-2,3-dihydrothiazolo[3,2-a]pyridine-3-carboxamide (27).
Ammonium salt 25 (70 mg, 0.16 mmol) was dissolved with stirring in DMF (500 μL) and NaHCO3 (92 mg, 1.10 mmol) was added, followed by iodomethane (49 μL, 0.78 mmol). The resulting mixture was heated to 70 °C and stirred for 10 h. The mixture was then partitioned (EtOAc/brine) and the aqueous re-extracted (×2). The combined organics were dried (MgSO4). The solvent was then removed and replaced with a mixture of THF (640 μL) and MeOH (140 μL), then 1 M LiOH(aq) (786 μL, 0.79 mmol) added. The mixture was stirred at rt and monitored by LC-MS. On completion, the mixture was partitioned (EtOAc/brine) and the aqueous re-extracted (×2), then the combined organics dried (MgSO4), filtered and the solvent removed under reduced pressure. The residue was re-dissolved in DMF (500 μL) and HATU (59 mg, 0.15 mmol), aniline (23.5 μL, 0.26 mmol) and DIPEA (45 μL 0.26 mmol) added. After stirring for 1 h at rt, the mixture was partitioned (EtOAc/dil. HCl(aq)) and the aqueous re-extracted (×2), then the combined organics dried (MgSO4), filtered and the solvent removed under reduced pressure. Purified by HPLC as described in the general procedure and freeze-dried from H2O
:
MeCN to give dimethylamine 27 as a white solid (39 mg, 55%). 1H NMR (600 MHz, DMSO-d6) δ 10.39 (s, 1H), 7.96 (d, J = 7.7 Hz, 1H), 7.91 (d, J = 7.9 Hz, 1H), 7.86 (d, J = 8.2 Hz, 1H), 7.59–7.45 (m, 5H), 7.40 (d, J = 7.0 Hz, 1H), 7.30 (apparent t, J = 7.8 Hz, 2H), 7.06 (apparent t, J = 7.4 Hz, 1H), 5.45 (d, J = 9.1 Hz, 1H), 5.31 (s, 1H), 4.35, 4.30 (ABq, JAB = 16.7 Hz, 2H), 3.92 (dd, J = 12.0, 9.2 Hz, 1H), 3.55 (d, J = 11.9 Hz, 1H), 2.71 (s, 6H). 13C NMR (151 MHz, DMSO) δ 166.0, 159.5, 156.1, 147.3, 138.7, 134.8, 133.5, 131.5, 128.8, 128.6, 127.6, 127.2, 126.3, 125.8, 125.6, 125.1, 124.0, 123.6, 119.0, 113.0, 63.8, 42.6, 41.8, 33.8, 31.9. HRMS (ESI+) (m/z): [M + Na+]+ calcd. for C27H25N3O2SNa+, 478.1565; found, 478.1557.
Methyl(7-[naphthalen-1-ylmethyl]-5-oxo-3-[phenylcarbamoyl]-2,3-dihydrothiazolo[3,2-a]pyridin-8-yl)carbamate (28).
Ammonium salt 25 (70 mg, 0.16 mmol) was dissolved with stirring in DCM (500 μL) and pyridine (51 μL, 0.63 mmol) was added, followed by methyl chloroformate (30 μL, 0.39 mmol). The resulting mixture was stirred for 2 h, then partitioned (EtOAc/1 M HCl(aq)) and the aqueous re-extracted (×2), dried (MgSO4), filtered and the solvent removed under reduced pressure. The residue was purified by chromatography (SiO2, DCM/MeOH with 1% Et3N), then the resulting material dissolved in THF MeOH (200 μL) and 1 M LiOH(aq) (784 μL, 0.784 mmol) were added and the reaction monitored by TLC until conversion was complete. The mixture was acidified with 1 M HCl(aq) and extracted with DCM, then partially purified by chromatography (SiO2, EtOAc/heptane). The resulting material was dissolved in THF (1.15 mL) and MeOH (287 μL) then 1 M LiOH(aq) (789 μL, 0.79 mmol) added. After stirring overnight the material had precipitated, and was triturated with additional THF then collected. The residue was re-dissolved in DMF (500 μL) and HATU (53 mg, 0.14 mmol), aniline (19.6 μL, 0.21 mmol) and DIPEA (37 μL, 0.21 mmol) added. After stirring for 1 h at rt, the mixture was partitioned (EtOAc/dil. HCl(aq)) and the aqueous re-extracted (×2), dried (MgSO4), filtered and the solvent removed under reduced pressure. Purified by HPLC as described in the general procedure and freeze-dried from H2O
:
MeCN to give carbamate 28 as a white solid (29 mg, 38%). 1H NMR (600 MHz, DMSO-d6) δ 10.40 (s, 1H), 8.89* (s, 0.8H), 8.62* (s, 0.2H), 8.01–7.93 (m, 1H), 7.89 (d, J = 8.2 Hz, 1H), 7.87–7.80 (m, 1H), 7.59–7.47 (m, 5H), 7.41 (d, J = 7.0 Hz, 1H), 7.30 (apparent t, J = 7.7 Hz, 2H), 7.06 (t, J = 7.4 Hz, 1H), 5.52 (dd, J = 9.1, 1.6 Hz, 1H), 5.22* (s, 0.2H), 5.15* (s, 0.8H), 4.17, 4.12 (ABq, JAB = 17.1 Hz, 2H), 3.96 (apparent t, J = 10.6 Hz, 1H), 3.65 (s, 3H), 3.56 (d, J = 11.9 Hz, 1H). 13C NMR (151 MHz, DMSO) δ 165.7, 159.6, 155.7, 154.8, 149.8, 138.6, 133.5, 131.5, 128.8, 128.6, 128.1, 127.5, 126.3, 125.8, 125.7, 124.1, 123.6, 119.0, 112.4, 110.9, 65.0, 52.1, 33.6, 31.9. HRMS (ESI+) (m/z): [M + Na+]+ calcd. for C27H23N3O4SNa+, 508.1301; found, 508.1298.*Note: rotameric signals.
8-Acetamido-7-(naphthalen-1-ylmethyl)-5-oxo-N-phenyl-2,3-dihydrothiazolo[3,2-a]pyridine-3-carboxamide (29).
Ammonium salt 25 (70 mg, 0.16 mmol) was dissolved with stirring in DCM (500 μL) and pyridine (51 μL, 0.63 mmol) was added, followed by acetyl chloride (28 μL, 0.39 mmol). The resulting mixture was stirred overnight. MeOH (200 μL) and 1 M LiOH(aq) (784 μL, 0.784 mmol) were added and the reaction monitored by TLC until conversion was complete. The mixture was acidified with 1 M HCl(aq) and extracted with DCM, then partially purified by chromatography (SiO2, EtOAc/heptane). The resulting material was dissolved in THF (1.42 mL) and MeOH (355 μL) then 1 M LiOH(aq) (783 μL, 0.78 mmol) added. After 2 h the material had precipitated, and was triturated with additional THF then collected. The residue was re-dissolved in DMF (500 μL) and HATU (61 mg, 0.16 mmol), aniline (22.7 μL, 0.25 mmol) and DIPEA (43 μL, 0.25 mmol) added. After stirring for 1 h at rt, the mixture was partitioned (EtOAc/dil. HCl(aq)) and the aqueous re-extracted (×2), dried (MgSO4), filtered and the solvent removed under reduced pressure. Purified by HPLC as described in the general procedure and freeze-dried from H2O
:
MeCN to give amide 29 as a white solid (37 mg, 50%). 1H NMR (600 MHz, DMSO-d6) δ 10.40 (s, 1H), 9.41 (s, 1H), 7.98–7.94 (m, 1H), 7.88 (d, J = 8.3 Hz, 1H), 7.87–7.83 (m, 1H), 7.59–7.45 (m, 5H), 7.41 (d, J = 7.0 Hz, 1H), 7.30 (apparent t, J = 7.7 Hz, 2H), 7.06 (apparent t, J = 7.4 Hz, 1H), 5.50 (d, J = 9.1 Hz, 1H), 5.14 (s, 1H), 4.14, 4.08 (ABq, JAB = 17.3 Hz, 2H), 3.94 (dd, J = 12.0, 9.1 Hz, 1H), 3.56 (d, J = 12.0 Hz, 1H), 2.02 (s, 3H). 13C NMR (151 MHz, DMSO) δ 169.5, 165.8, 159.6, 154.5, 148.7, 138.6, 133.6, 133.5, 131.5, 128.8, 128.6, 128.1, 127.5, 126.3, 125.8, 125.7, 124.2, 123.6, 119.0, 112.3, 111.3, 64.9, 33.8, 31.8, 22.4. HRMS (ESI+) (m/z): [M + H+]+ calcd. for C27H24N3O3S+, 470.1533; found, 470.1536.
8-(Methylsulfonamido)-7-(naphthalen-1-ylmethyl)-5-oxo-N-phenyl-2,3-dihydrothiazolo[3,2-a]pyridine-3-carboxamide (30).
Ammonium salt 25 (70 mg, 0.16 mmol) was dissolved with stirring in THF (500 μL) and pyridine (50 μL, 0.63 mmol) was added, followed by methanesulfonyl chloride (30 μL, 0.39 mmol). The resulting mixture was stirred overnight. MeOH (200 μL) and 1 M LiOH(aq) (784 μL, 0.784 mmol) were added and the reaction monitored by TLC until conversion was complete. The mixture was acidified with 1 M HCl(aq) and partitioned with IPA/brine. The aqueous was re-extracted (×2), and the combined organics were dried (MgSO4), filtered and the solvent removed under reduced pressure. The residue was re-dissolved in DMF (500 μL) and HATU (99 mg, 0.18 mmol), aniline (25.4 μL, 0.28 mmol) and DIPEA (48 μL, 0.28 mmol) added. After stirring for 1 h at rt, the mixture was partitioned (EtOAc/dil. HCl(aq)) and the aqueous re-extracted (×2), dried (MgSO4), filtered and the solvent removed under reduced pressure. Purified by HPLC as described in the general procedure and freeze-dried from H2O
:
MeCN to give methyl sulfonamide 30 as a white solid (41 mg, 52%). 1H NMR (600 MHz, DMSO-d6) δ 10.40 (s, 1H), 9.31 (s, 1H), 7.97 (d, J = 7.8 Hz, 1H), 7.90 (d, J = 8.2 Hz, 1H), 7.87 (d, J = 8.1 Hz, 1H), 7.58–7.47 (m, 5H), 7.43 (d, J = 7.0 Hz, 1H), 7.30 (apparent t, J = 7.8 Hz, 2H), 7.06 (apparent t, J = 7.4 Hz, 1H), 5.50 (d, J = 9.0 Hz, 1H), 5.04 (s, 1H), 4.47, 4.31 (ABq, JAB = 17.7 Hz, 2H), 4.04–3.99 (m, 1H), 3.60 (d, J = 11.9 Hz, 1H), 3.22 (s, 3H). 13C NMR (151 MHz, DMSO) δ 165.6, 159.4, 156.3, 151.6, 138.6, 133.8, 133.5, 131.5, 128.8, 128.6, 128.3, 127.6, 126.3, 125.9, 125.7, 124.2, 123.6, 119.0, 112.7, 109.9, 65.0, 43.3, 34.4, 32.1. HRMS (ESI+) (m/z): [M + H+]+ calcd. for C26H24N3O4S2+, 506.1203; found, 506.1200.
N-(3-Fluoro-5-methylphenyl)-8-(methylsulfonamido)-7-(naphthalen-1-ylmethyl)-5-oxo-2,3-dihydrothiazolo[3,2-a]pyridine-3-carboxamide (32).
Carboxylic acid 31 (70 mg, 0.16 mmol) was dissolved in anhydrous DMF (2.5 mL) and 3-fluoro-5-methylaniline (36 μL, 0.32 mmol), HATU (74 mg, 0.19 mmol) and diisopropyl ethyl amine (57 μL, 0.33 mmol) were added and stirred at rt for 4 h. The reaction mixture was cooled on ice and quenched by addition of 0.5 M HCl(aq) (4 mL) in portions, diluted with EtOAc and the aqueous layer was extracted with EtOAc (×2). The combined organic layers were washed successively with 0.5 M HCl(aq) and brine, dried (Na2SO4) and concentrated under reduced pressure. Purification by flash chromatography (SiO2, 40–100% EtOAc in heptane) and freeze-drying from H2O
:
MeCN (5
:
1) afforded the amide 32 as an orange powder (43 mg, 49%). 1H-NMR (600 MHz, DMSO-d6) δ 10.55 (s, 1H), 9.32 (s, 1H), 7.98–7.95 (m, 1H), 7.90 (d, J = 8.2 Hz, 1H), 7.88–7.85 (m, 1H), 7.54–7.49 (m, 3H), 7.43 (d, J = 6.9 Hz, 1H), 7.30–7.26 (m, 1H), 7.10 (s, 1H), 6.76–6.72 (m, 1H), 5.48 (dd, J = 9.3, 2.0 Hz, 1H), 5.04 (s, 1H), 4.46, 4.32 (ABq, JAB = 17.7 Hz, 2H), 4.01 (dd, J = 11.9, 9.3 Hz, 1H), 3.59 (dd, J = 11.9, 2.0 Hz, 1H), 3.21 (s, 3H), 2.27 (s, 3H). 13C-NMR (151 MHz, DMSO-d6) δ 165.9, 162.0 (d, JCF = 141.0 Hz), 159.4, 156.3, 151.6, 140.6 (d, JCF = 9.6 Hz), 139.8 (d, JCF = 12.1 Hz), 133.8, 133.5, 131.5, 128.6, 128.3, 127.6, 126.3, 125.9, 125.7, 124.2, 115.4 (CF broad peak), 112.7, 110.8 (d, JCF = 21.2 Hz), 109.9, 103.2 (d, JCF = 26.4 Hz), 65.0, 43.3, 34.4, 21.0 (d, JCF = 2.0 Hz). HRMS (ESI+) (m/z): [M + H+]+ calcd. for C27H25FN3O4S2, 538.1265; found, 538.1273.
8-(Methylsulfonamido)-7-(naphthalen-1-ylmethyl)-5-oxo-N-(m-tolyl)-2,3-dihydrothiazolo[3,2-a]pyridine-3-carboxamide (33).
Carboxylic acid 31 (70 mg, 0.16 mmol) was dissolved in anhydrous DMF (2.5 mL) and 3-methylaniline (34 μL, 0.32 mmol), HATU (74 mg, 0.19 mmol), and diisopropyl ethyl amine (57 μL, 0.33 mmol) were added and stirred under an inert atmosphere at rt for 4 h. The reaction mixture was cooled on ice and quenched by addition of 0.5 M HCl(aq) (4 mL) in portions, diluted with EtOAC and aqueous layer was extracted with EtOAc (×2). The combined organic layers were washed successively with 0.5 M HCl(aq) and brine (2 mL 0.5 M HCl(aq) was added to aid separation), dried (Na2SO4) and concentrated under reduced pressure. Purification by flash chromatography (SiO2, EtOAc in heptane, 70–100%) and freeze-drying from H2O
:
MeCN (5
:
1) afforded the amide 33 as beige powder (66 mg, 78%). 1H-NMR (600 MHz, DMSO-d6) δ 10.32 (s, 1H), 9.32 (s, 1H), 7.99–7.95 (m, 1H), 7.92–7.85 (m, 2H), 7.55–7.49 (m, 3H), 7.44–7.42 (m, 1H), 7.41–7.39 (m, 1H), 7.31–7.27 (m, 1H), 7.18 (t, J = 7.8 Hz, 1H), 6.90–6.86 (m, 1H), 5.49 (dd, J = 9.2, 2.0 Hz, 1H), 5.04 (s, 1H), 4.46, 4.32 (ABq, JAB = 17.7 Hz, 2H), 4.01 (dd, J = 11.8, 9.2 Hz, 1H), 3.58 (dd, J = 11.8, 2.0 Hz, 1H), 3.21 (s, 3H), 2.25 (s, 3H). 13C-NMR (151 MHz, DMSO-d6) δ 165.6, 159.5, 156.2, 151.6, 138.5, 138.1, 133.9, 133.5, 131.5, 128.7, 128.6, 128.3, 127.6, 126.3, 125.9, 125.7, 124.33, 124.25, 119.6, 116.2, 112.7, 109.9, 65.0, 43.3, 34.4, 32.1, 21.1. HRMS (ESI+) (m/z): [M + H+]+ calcd. for C27H26N3O4S2, 520.1359; found, 520.1362.
Methyl 8-(((benzyloxy)carbonyl)amino)-7-(naphthalen-1-ylmethyl)-5-oxo-3,5-dihydro-2H-thiazolo[3,2-a]pyridine-3-carboxylate (36).
Thiazoline 34 (5.86 g, 19.0 mmol) and acyl meldrum's acid derivative 35 (19.6 g, 62.7 mmol) were dissolved in DCM (73 ml) and TFA (1.61 ml, 20.9 mmol) was added. The solution was split into 7 vials which were then capped and heated to 70 °C for 20 h. Quenched with NaHCO3 (sat. aq). The water layer was extracted with EtOAc and the organic layers were dried (Na2SO4), filtered and concentrated. Purified using column chromatography on silica gel (heptane
:
EtOAc 60
:
40 ≥ 0
:
100) to give methyl ester 36 as a yellow foam (8.12 g, 85%). 1H-NMR (400 MHz, CDCl3) δ 7.90–7.73 (m, 3H), 7.52–7.41 (m, 2H), 7.41–7.28 (m, 6H), 7.24–7.18 (m, 1H), 5.93 (s, 1H), 5.80–5.65 (m, 1H), 5.54 (dd, J = 8.4, 2.4 Hz, 1H), 5.20–5.10 (m, 2H), 4.27–4.05 (m, 2H), 3.76 (s, 3H), 3.73–3.63 (m, 1H), 3.55–3.45 (m, 1H); 13C-NMR (100 MHz, CDCl3) δ 168.3, 161.0, 154.9, 154.8, 148.4, 135.9, 134.1, 132.9, 132.0, 128.9, 128.7, 128.5, 128.3, 128.1, 127.7, 126.5, 126.0, 125.6, 124.0, 115.5, 111.5, 67.8, 64.0, 53.5, 34.9, 32.2; HRMS (ESI+) (m/z): [M + H+]+ calcd. for C28H25N2O5S+, 501.1479; found, 501.1476.
Benzyl(7-(naphthalen-1-ylmethyl)-5-oxo-3-(3-phenyl-1H-1,2,4-triazol-5-yl)-3,5-dihydro-2H-thiazolo[3,2-a]pyridin-8-yl)carbamate (37).
Methyl ester 36 (300 mg, 0.600 mmol) was dissolved in THF (2.2 ml) and 1 M LiOH(aq) (1.2 ml) was added. Stirred at room temperature for 2 h. Diluted with EtOAc and washed with 1 M HCl. The organic layer was dried (Na2SO4), filtered and concentrated. The residue was dissolved in dry DMF (3.6 ml) and benzamidine hydrochloride hydrate (154 mg, 0.88 mmol), DIPEA (305 μl, 1.76 mmol) and HATU (257 mg, 0.676 mmol) were added. Stirred at room temperature for 3.5 h (4-methoxybenzyl)hydrazine hydrochloride (166 mg, 0.882 mmol) and AcOH (337 μl, 5.88 mmol) were added and the mixture was heated to 80 °C for 20 h. Cooled to room temperature and quenched with NaHCO3 (aq). Extracted with EtOAc. The organic layers were washed with NaHCO3 (sat. aq), 5% LiCl, water, and brine and then dried (Na2SO4), filtered, and concentrated. The residue was suspended in DCM (3.6 ml) and TFA (3.6 ml) was added. Heated to 50 °C for 22 h. Concentrated, dissolved in EtOAc and washed with NaHCO3 (sat. aq). The water layer was extracted with EtOAc and the organic layers were then washed with water and brine. Dried (Na2SO4), filtered and concentrated. Purified using column chromatography on silica gel (heptane
:
EtOAc 25
:
75 ≥ 0
:
100) followed by preperative HPLC (H2O
:
MeCN 90
:
10 ≥ 0
:
100 with 0.75% formic acid over 40 minutes) to give 1,2,4-triazole 37 as a yellow solid (135 mg, 39%). 1H-NMR (400 MHz, CDCl3) δ 7.87–7.85 (m, 4H), 7.72 (d, J = 8.4 Hz, 1H), 7.46–7.36 (m, 2H), 7.35–7.21 (m, 9H), 7.11 (d, J = 7.1 Hz, 1H), 6.48 (s, 1H), 6.20 (d, J = 7.3 Hz, 1H), 5.68 (s, 1H), 5.13 (s, 2H), 4.17, 3.95 (ABq, JAB = 17.6 Hz, 2H), 3.78–3.66 (m, 2H); 13C-NMR (100 MHz, CDCl3) δ 161.7, 158.3, 157.6, 155.7, 155.1, 157.7, 136.0, 134.0, 132.9, 131.9, 130.0, 128.8, 128.8, 128.7, 128.7, 128.4, 128.2, 128.0, 127.8, 126.6, 126.5, 125.9, 125.6, 124.1, 115.0, 112.4, 67.7, 60.8, 34.7, 33.7; LRMS (m/z): [M + H+]+ calcd. for C34H28N5O3S, 586.2+; found, 586.3.
N-(7-(Naphthalen-1-ylmethyl)-5-oxo-3-(3-phenyl-1H-1,2,4-triazol-5-yl)-3,5-dihydro-2H-thiazolo[3,2-a]pyridin-8-yl)methanesulfonamide (38).
1,2,4-Triazole 37 (135 mg, 0.231 mmol) was dissolved in DCM (5 ml) and 33% HBr in AcOH (5 ml) was added. Stirred at room temperature for 2 h. Et2O was added to precipitate the product (127 mg), which was collected by filtration and washed with cold Et2O. 15 mg of this residue was dissolved in pyridine (0.5 ml) and MsCl (6.6 μl, 0.085 mmol) was added. Stirred at room temperature for 18 h. Concentrated and redissolved in a mixture of THF (1 ml) and MeOH (0.4 ml). LiOH (aq) (0.28 ml, 1 M in H2O) was added and the mixture was stirred at room temperature until completion (indicated by TLC). Diluted with EtOAc and washed with water. Dried (Na2SO4), filtered and concentrated. Purified using preperative HPLC (H2O
:
MeCN 90
:
10 ≥ 0
:
100 with 0.75% formic acid over 40 minutes) to give methyl sulfonamide 38 as a yellow powder (8 mg, 53%). 1H-NMR (400 MHz, DMSO-d6) δ 14.27 (br s, 1H), 9.34 (br s, 1H), 8.01–7.95 (m, 1H), 7.94–7.86 (m, 4H), 7.57–7.42 (m, 7H), 6.17 (dd, J = 8.0, 1.1 Hz, 1H), 5.09 (s, 1H), 4.48, 4.34 (ABq, JAB = 17.2 Hz, 2H), 4.10 (dd, J = 11.5, 8.2 Hz, 1H), 3.55 (dd, J = 11.5, 1.1 Hz, 1H), 3.22 (s, 3H); 13C-NMR (150 MHz, DMSO-d6) δ 161.6, 158.4, 157.5, 150.6, 134.1, 133.0, 132.0, 130.2, 128.9 (2C), 128.8, 128.1, 128.1, 126.6, 126.6, 126.0, 125.6, 124.4, 115.7, 111.8, 60.8, 43.7, 35.5, 33.5;. HRMS (ESI+) (m/z): [M + H+]+ calcd. for C27H24N5O3S2+, 530.1315; found, 530.1314.
Ethyl 2-(1,3-dioxoisoindolin-2-yl)acetimidate hydrochloride (39).
2-(1,3-Dioxoisoindolin-2-yl)acetonitrile (3.84 g, 20.6 mmol) was dissolved in a mixture of EtOH (60 ml) and EtOAc (60 ml). AcCl (14.7 ml, 206 mmol) was then added dropwise over 30 minutes at 0 °C. Allowed to slowly reach room temperature and stirring continued for 17 h, then concentrated to give 5.29 g of a mixture containing 39 (1.93 g, 35%) and ethyl 2-(1,3-dioxoisoindolin-2-yl)acetate as a white solid. 1H-NMR (400 MHz, CDCl3) δ 7.96–7.92 (m, 2H), 7.83–7.78 (m, 2H), 4.92 (s, 2H), 4.75 (q, J = 7.1 Hz, 2H), 1.43 (t, J = 7.1 Hz, 3H); 13C-NMR (100 MHz, CDCl3) δ 173.1, 166.9, 134.8, 131.9, 124.2, 72.6, 38.2, 13.7.
(R)-Methyl 2-((1,3-dioxoisoindolin-2-yl)methyl)-4,5-dihydrothiazole-4-carboxylate (40).
5.29 g of the mixture containing 39 (1.93 g, 7.18 mmol) and ethyl 2-(1,3-dioxoisoindolin-2-yl)acetate was dissolved in DCM (80 ml) and L-cysteine methyl ester hydrochloride (3.90 g, 22.7 mmol) followed by Et3N (4.32 ml, 31.0 mmol) were added. The reaction was stirred at room temperature for 3 days. The mixture was washed with NaHCO3 (aq) and the aqueous phase was extracted with EtOAc and DCM. The organic layers were dried (Na2SO4), filtered and concentrated. Purified using column chromatography on silica gel (heptane
:
EtOAc 88
:
12 ≥ 0
:
100) to give 40 as a colorless oil (1.39 g, 64%) [α]D 2° (c 0.0035, DCM). 1H-NMR (400 MHz, CDCl3) δ 7.86–7.80 (m, 2H), 7.71–7.66 (m, 2H), 5.10–5.03 (m, 1H), 4.66 (t, J = 1.8 Hz, 2H), 3.72 (s, 3H), 3.58 (dd, J = 11.3, 8.6 Hz, 1H), 3.48 (dd, J = 11.3, 9.6 Hz, 1H). 13C-NMR (100 MHz, CDCl3) δ 170.8, 170.0, 167.4, 134.4, 132.1, 123.9, 78.1, 53.0, 39.8, 35.9. HRMS (ESI+) (m/z): [M + Na+]+ calcd. for C14H12N2O4SNa+, 327.0410; found, 327.0411.
(R)-Methyl 8-(1,3-dioxoisoindolin-2-yl)-7-(naphthalen-1-ylmethyl)-5-oxo-3,5-dihydro-2H-thiazolo[3,2-a]pyridine-3-carboxylate (41).
Substituted thiazoline 40 (740 mg, 2.43 mmol) and 5-(1-hydroxy-2-(naphthalen-1-yl)ethylidene)-2,2-dimethyl-1,3-dioxane-4,6-dione (1.67 g, 5.35 mmol) were dissolved in DCM (9.35 ml). TFA (205 μl, 2.68 mmol) was added and the mixture was heated in a sealed vial at 80 °C for 4 days. More 5-(1-hydroxy-2-(naphthalen-1-yl)ethylidene)-2,2-dimethyl-1,3-dioxane-4,6-dione (1.67 g, 5.35 mmol) was added after 24 h, after 48 h, and after 72 h. The reaction was then quenched with NaHCO3(aq) and extracted with EtOAc. The organic phase was dried (Na2SO4), filtered and concentrated. Purified using column chromatography on silica gel (heptane
:
EtOAc 88
:
12 ≥ 0
:
100) to give methyl ester 41 as a brown foam (742 mg, 62%). [α]D −4° (c 0.0025, DCM); 1H-NMR (400 MHz, CDCl3) δ 7.98–7.91 (m, 2H), 7.76–7.69 (m, 4H), 7.67 (d, J = 8.3 Hz, 1H), 7.40–7.32 (m, 2H), 7.29 (t, J = 7.4 Hz, 1H), 7.22 (d, J = 6.9 Hz, 1H), 5.79 (s, 1H) 5.54 (dd, J = 8.5, 2.6 Hz, 1H), 4.07, 3.99 (ABq, JAB = 17.1 Hz, 2H), 3.78 (s, 3H), 3.73 (dd, J = 11.9, 8.4 Hz, 1H), 3.53 (dd, J = 11.9, 2.7 Hz, 1H). 13C-NMR (100 MHz, CDCl3) δ 167.9, 166.5, 166.4, 160.8, 154.2, 149.4, 134.7, 134.6, 133.8, 132.0, 131.6, 131.4, 131.3, 128.6, 128.1, 128.0, 126.4, 125.7, 125.4, 123.9, 123.9, 123.8, 115.5, 106.7, 63.9, 53.4, 34.7, 32.3. HRMS (ESI+) (m/z): [M + H+]+ calcd. for C28H21N2O5S+, 497.1166; found, 497.1166.
8-(1,3-Dioxoisoindolin-2-yl)-7-(naphthalen-1-ylmethyl)-5-oxo-3,5-dihydro-2H-thiazolo[3,2-a]pyridine-3-carboxylic acid (42).
Methyl ester 41 (1.26 g, 2.54 mmol) was dissolved in THF (10.3 ml) and LiOH (5.1 ml, 1 M in H2O) was added. The reaction was stirred for 4 h, then acidified using 1 M HCl and extracted with EtOAc. The organic phase was dried (Na2SO4), filtered and concentrated. Redissolved in DMF (15 ml) and AcOH (146 μl) added. Heated to 150 °C for 20 h. Concentrated and purified using column chromatography on silica gel (DCM
:
MeOH 99
:
1 ≥ 88
:
12 with 1% AcOH) to give carboxylic acid 42 as a brown solid (955 mg, 78%). 1H-NMR (400 MHz, DMSO-d6) δ 13.60 (br s, 1H), 7.98–7.86 (m, 5H), 7.83–7.73 (m, 2H), 7.48–7.34 (m, 3H), 7.33–7.28 (m, 1H), 5.50 (s, 1H), 5.46 (dd, J = 9.0, 2.4 Hz, 1H), 4.13, 4.07 (ABq, JAB = 17.4 Hz, 2H), 3.95 (dd, J = 11.9, 9.2 Hz, 1H), 3.59 (dd, J = 11.9, 2.1 Hz, 1H). 13C-NMR (100 MHz, DMSO-d6) δ 169.0, 166.4, 166.2, 159.6, 154.0, 150.8, 135.2, 135.2, 133.4, 132.7, 131.2, 130.9, 128.6, 128.0, 127.6, 126.2, 125.7, 125.4, 123.9, 123.8, 123.7, 113.4, 105.3, 63.7, 33.8, 32.0. HRMS (ESI+) (m/z): [M + H+]+ calcd. for C27H19N2O5S+, 483.1009; found, 483.1007.
tert-Butyl (8-(1,3-dioxoisoindolin-2-yl)-7-(naphthalen-1-ylmethyl)-5-oxo-3,5-dihydro-2H-thiazolo[3,2-a]pyridin-3-yl)carbamate (43).
Carboxylic acid 42 (940 mg, 1.95 mmol) was suspended in dry t-BuOH (20 ml) and DPPA (442 μl, 2.05 mmol) followed by Et3N (285 μl, 2.05 mmol) were added. 3 Å molecular sieves (enough to cover approximately one third of the solvent volume) was added to the suspension and the reaction vessel was sealed. The reaction was heated to 85 °C for 2 h and then filtered through wool and concentrated. Purified using column chromatography on silica gel (heptane
:
EtOAc 80
:
20 ≥ 0
:
100, the column was prewashed with heptane
:
EtOAc
:
TEA 80
:
20
:
2 to neutralize the silica gel) to give BOC-protected amine 43 as a pale yellow foam (610 mg, 57%). 1H-NMR (600 MHz, CDCl3) δ 7.82–7.76 (m, 2H), 7.71–7.67 (m, 4H), 7.63 (d, J = 8.3 Hz, 1H), 7.34–7.29 (m, 2H), 7.24 (t, J = 7.9 Hz, 1H), 7.17 (d, J = 7.0 Hz, 1H), 6.60 (t, J = 7.4 Hz, 1H), 5.77 (s, 1H), 5.73 (d, J = 7.8 Hz, 1H), 3.97 (s, 2H), 3.66 (dd, J = 11.8, 6.6 Hz, 1H), 3.28 (d, J = 11.8 Hz, 1H), 1.41 (s, 9H). 13C-NMR (151 MHz, CDCl3) δ 166.7, 166.4, 160.0, 153.9, 153.9, 149.0, 134.6, 133.7, 131.9, 131.5, 131.3, 131.2, 128.6, 128.0, 127.9, 126.3, 125.7, 125.3, 123.9, 123.9, 123.7, 115.8, 106.2, 80.8, 69.7, 36.2, 34.6, 28.2. HRMS (ESI+) (m/z): [M + H+]+ calcd. for C31H28N3O5S+, 554.1744; found, 554.1742.
2-(3-Amino-7-(naphthalen-1-ylmethyl)-5-oxo-3,5-dihydro-2H-thiazolo[3,2-a]pyridin-8-yl)isoindoline-1,3-dione (44).
BOC-protected amine 43 (280 mg, 0.51 mmol) was dissolved in DCM (6 ml) and TFA (2 ml) was added. The reaction was stirred at room temperature for 2 h. Neutralized with NaHCO3 (sat. aq) and extracted with EtOAc. The organic phase was dried (Na2SO4), filtered and concentrated. Purified using column chromatography on silica gel (EtOAc
:
MeOH 99
:
1 ≥ 90
:
10) to give amine 44 as a pale yellow foam (201 mg, 88%). 1H-NMR (600 MHz, CDCl3) δ 7.85–7.79 (m, 2H), 7.74–7.68 (m, 4H), 7.68 (d, J = 8.4 Hz, 1H), 7.36–7.30 (m, 2H), 7.29 (t, J = 7.5 Hz, 1H), 7.22 (d, J = 7.0 Hz, 1H), 5.93 (dd, J = 7.3, 1.9 Hz, 1H), 5.81 (s, 1H), 4.01 (s, 2H), 3.67 (dd, J = 11.9, 7.5 Hz, 1H), 3.12 (d, J = 11.9, 1.9 Hz, 1H), 2.59 (s, 2H). 13C-NMR (151 MHz, CDCl3) δ 166.7, 166.5, 161.3, 154.1, 148.1, 134.6, 133.8, 132.1, 131.6, 131.4, 131.3, 128.6, 128.1, 127.9, 126.4, 125.7, 125.4, 123.9, 123.8, 115.5, 106.2, 74.2, 35.5, 34.6. HRMS (ESI+) (m/z): [M + H+]+ calcd. for C26H20N3O3S+, 454.1220; found, 454.1222.
2-(7-(Naphthalen-1-ylmethyl)-5-oxo-3-(4-phenyl-1H-1,2,3-triazol-1-yl)-3,5-dihydro-2H-thiazolo[3,2-a]pyridin-8-yl)isoindoline-1,3-dione (45).
Amine 44 (500 mg, 1.10 mmol) was dissolved in dry MeOH (10 ml). K2CO3 (335 mg, 2.43 mmol) and CuSO4 (35.3 mg, 0.22 mmol) followed by imidazole-1-sulfonyl azide (628 mg, 2.32 mmol) were added. The mixture was heated to 50 °C for 24 h. Diluted with EtOAc and washed with brine. The water layer was extracted with EtOAc. The combined organic layers were dried (Na2SO4), filtered and concentrated. Rough purification using column chromatography on silica gel (heptane
:
EtOAc 90
:
10 ≥ 20
:
80) to give a yellow oil containing the azide. Redissolved in a mixture of DMF (1.86 ml) and H2O (0.186 ml) and added sodium ascorbate (61.5 mg, 0.310 mmol), CuSO4 (24.8 mg, 0.155 mmol) and phenyl acetylene (28.4 μl, 0.257 mmol). The reaction was stirred at room temperature for 3.5 hours. Diluted with EtOAc and washed with brine. EDTA (50 eq.) was added and the water phase was then acidified to pH 5 and left to stir overnight. Extracted with EtOAc. The combined organic layers were dried (Na2SO4), filtered and concentrated. Purified using column chromatography on silica gel (heptane
:
EtOAc 88
:
12 ≥ 0
:
100) to give 1,2,3-triazole 45 as a colorless solid (9 mg, 1.4%). 1H-NMR (400 MHz, DMSO-d6) δ 8.70 (s, 1H), 8.01–7.89 (m, 4H), 7.89–7.82 (m, 3H), 7.81–7.73 (m, 2H), 7.52–7.38 (m, 5H), 7.36 (t, J = 7.4 Hz, 2H), 7.29 (d, J = 7.4 Hz, 1H), 5.52 (s, 1H), 4.32 (dd, J = 13.1, 7.6 Hz, 1H), 4.17, 4.10 (ABq, JAB = 17.4 Hz, 2H), 3.87 (d, J = 12.8 Hz, 1H). 13C-NMR (100 MHz, DMSO-d6) δ 166.3, 166.2, 159.1, 155.3, 151.4, 145.9, 135.3, 135.2, 133.4, 132.4, 131.1, 130.9, 130.1, 129.0, 128.6, 128.2, 128.1, 127.6, 126.3, 125.8, 125.5, 125.3, 124.0, 123.9, 123.6, 121.8, 113.6, 105.8, 74.1, 35.0, 33.9. HRMS (ESI+) (m/z): [M + H+]+ calcd. for C34H24N5O3S+, 582.1594; found, 582.1593.
N-(7-(Naphthalen-1-ylmethyl)-5-oxo-3-(4-phenyl-1H-1,2,3-triazol-1-yl)-3,5-dihydro-2H-thiazolo[3,2-a]pyridin-8-yl)methanesulfonamide (46).
1,2,3-Triazole 45 (8 mg, 0.014 mmol) was suspended in EtOH (10 ml) and methyl amine (2.5 ml, 40% in H2O) was added. The reaction was stirred at room temperature for 20 hours. Most of the EtOH was removed under vacuum and the remaining solution was extracted with EtOAc. The organic phase was dried (Na2SO4), filtered and concentrated. The residue was redissolved in pyridine (0.28 ml) and MsCl (3.2 μl, 0.04 mmol) was added. Stirred at room temperature for 26 h. Additional MsCl was added after 19 h (3.2 μl, 0.04 mmol) and after 22 h (6.4 μl, 0.08 mmol). The reaction was concentrated and redissolved in MeOH (250 μl) and THF (600 μl). LiOH (700 μl, 1 M in H2O) was added and the reaction was stirred at room temperature for 2 h. The mixture was diluted with EtOAc and washed with brine. The organic phase was dried (Na2SO4), filtered and concentrated. Purified using HPLC (H2O
:
MeCN 95
:
5 ≥ 0
:
100 with 0.75% formic acid over 40 minutes) and then freeze dried to give amine 46 as a colorless solid (7 mg, 99%). 1H-NMR (600 MHz, CDCl3) δ 8.20 (s, 1H), 7.87–7.75 (m, 5H), 7.48–7.35 (m, 5H), 7.34–7.30 (m, 2H), 7.24 (d, J = 7.1 Hz, 1H), 6.10 (s, 1H), 5.66 (s, 1H), 4.50, 4.33 (ABq, JAB = 17.7 Hz, 2H), 4.27 (d, J = 12.5 Hz, 1H), 4.06 (dd, J = 12.6, 7.3 Hz, 1H), 3.23 (s, 3H); 13C-NMR (151 MHz, CDCl3) δ 160.3, 157.9, 149.8, 148.1, 134.1, 132.6, 132.0, 129.9, 129.0, 128.9, 128.7, 128.4, 128.1, 126.7, 126.1, 126.0, 125.7, 124.2, 121.3, 116.1, 111.3, 73.8, 44.0, 35.7, 33.6. HRMS (ESI+) (m/z): [M + H+]+ calcd. for C27H24N5O3S2+, 530.1315; found, 530.1313.
Author contributions
S. B., Å. G., F. A. initiated the project. M. K., A. G. C., J. A. D. G., and A. E. G. L. developed the synthetic routes. M. K., A. G. C., J. A. D. G., A. E. G. L., and P. S. performed chemical synthesis and structural analysis of compounds. C. N.-O. tested the compounds in reinfect and cytotoxicity experiments. J. S. and W. B. did reinfect preliminary testing of first compounds. Å. G., J. S. and E. W. performed animal experiments. R. S. measured and calculated pharmacokinetic data. M. K., C. N.-O., S. B., Å. G., A. G. C., R. S., K. V. and F. A. analyzed data and wrote the manuscript.
Abbreviations
AUC | Area under curve |
C. trachomatis
|
Chlamydia trachomatis
|
CL | Clearance |
DIPEA |
N,N-Diisopropylethylamine |
DMEM | Dulbecco's modified eagle medium |
EB | Elementary body |
FBS | Fetal bovine serum |
HATU | 1-[Bis(dimethylamino)methylene]-1H-1,2,3-triazolo[4,5-b]pyridinium 3-oxide hexafluorophosphate |
HBSS | Hanks balanced salt solution |
IFU | Inclusion forming unit |
MOI | Multiplicity of infection |
MWI | Microwave irradiation |
RB | Reticulate body |
rt | Room temperature |
TBTU |
O-(Benzotriazol-1-yl)-N,N,N′,N′-tetramethyluronium tetrafluoroborate |
IV | Intravenously |
PO | Per oss (oral) |
Conflicts of interest
Sven Bergström and Fredrik Almqvist are cofounders of Quretech Bio AB.
Acknowledgements
We acknowledge funding from the Swedish Research Council (F. A. and S. B.), the Knut and Alice Wallenberg Foundation (F. A. and S. B.), the Göran Gustafsson Foundation (F. A.), the Kempe Foundation (F. A.), and the Swedish Foundation for Strategic Research (F. A.). We thank Ingela Nilsson and Berit Sondén for technical assistance and Fritiof Pontén for valuable comments on the manuscript.
References
-
World Health Organization, Global incidence and prevalence of selected curable sexually transmitted infections – 2008, 978 92 4 150383 9, World Health Organization, Geneva, Switzerland, 2012.
- J. A. Whittum-Hudson and A. P. Hudson, Human chlamydial infections: persistence, prevalence, and outlook for the future, Nat. Sci. Soc., 2005, 13, 371–382 CrossRef.
- A. Dautry-Varsat, A. Subtil and T. Hackstadt, Recent insights into the mechanisms of Chlamydia entry, Cell. Microbiol., 2005, 7, 1714–1722 CAS.
- A. Omsland, B. S. Sixt, M. Horn and T. Hackstadt, Chlamydial metabolism revisited: interspecies metabolic variability and developmental stage-specific physiologic activities, FEMS Microbiol. Rev., 2014, 38, 779–801 CrossRef CAS PubMed.
- K. Hybiske and R. S. Stephens, Mechanisms of host cell exit by the intracellular bacterium Chlamydia, Proc. Natl. Acad. Sci. U. S. A., 2007, 104, 11430–11435 CrossRef CAS PubMed.
- W. M. Geisler, Diagnosis and Management of Uncomplicated Chlamydia trachomatis Infections in Adolescents and Adults: Summary of Evidence Reviewed for the 2015 Centers for Disease Control and Prevention Sexually Transmitted Diseases Treatment Guidelines, Clin. Infect. Dis., 2015, 61, S774–S784 CrossRef PubMed.
- M. R. Hammerschlag and S. A. Kohlhoff, Treatment of chlamydial infections, Expert Opin. Pharmacother., 2012, 13, 545–552 CrossRef CAS PubMed.
- C. Jernberg, S. Löfmark, C. Edlund and J. K. Jansson, Long-term impacts of antibiotic exposure on the human intestinal microbiota, Microbiology, 2010, 156, 3216–3223 CrossRef CAS PubMed.
- P. Engström, K. S. Krishnan, B. D. Ngyuen, E. Chorell, J. Normark, J. Silver, R. J. Bastidas, M. D. Welch, S. J. Hultgren, H. Wolf-Watz, R. H. Valdivia, F. Almqvist and S. Bergström, A 2-pyridone-amide inhibitor targets the glucose metabolism pathway of Chlamydia trachomatis, mBio, 2014, 6, e02304–e02314 CrossRef PubMed.
- J. A. Good, J. Silver, C. Nunez-Otero, W. Bahnan, K. S. Krishnan, O. Salin, P. Engstrom, R. Svensson, P. Artursson, A. Gylfe, S. Bergstrom and F. Almqvist, Thiazolino 2-pyridone amide inhibitors of Chlamydia trachomatis infectivity, J. Med. Chem., 2016, 59, 2094–2108 CrossRef CAS PubMed.
- J. A. D. Good, M. Kulén, J. Silver, K. S. Krishnan, W. Bahnan, C. Núñez-Otero, I. Nilsson, E. Wede, E. de Groot, Å. Gylfe, S. Bergström and F. Almqvist, Thiazolino 2-pyridone amide isosteres as inhibitors of Chlamydia trachomatis infectivity, J. Med. Chem., 2017, 60, 9393–9399 CrossRef CAS PubMed.
- B. N. Ames, J. McCann and E. Yamasaki, Methods for detecting carcinogens and mutagens with the Salmonella/mammalian-microsome mutagenicity test, Mutat. Res., 1975, 31, 347–364 CAS.
- M. Kulén, M. Lindgren, S. Hansen, A. G. Cairns, C. Grundström, A. Begum, I. van der Lingen, K. Brännström, M. Hall, U. H. Sauer, J. Johansson, A. E. Sauer-Eriksson and F. Almqvist, Structure-based design of inhibitors targeting PrfA, the master virulence regulator of Listeria monocytogenes, J. Med. Chem., 2018, 61, 4165–4175 CrossRef PubMed.
- J. R. Dunetz, Y. Xiang, A. Baldwin and J. Ringling, General and Scalable Amide Bond Formation with Epimerization-Prone Substrates Using T3P and Pyridine, Org. Lett., 2011, 13, 5048–5051 CrossRef CAS PubMed.
- V. Åberg, M. Sellstedt, M. Hedenström, J. S. Pinkner, S. J. Hultgren and F. Almqvist, Design, synthesis and evaluation of peptidomimetics based on substituted bicyclic 2-pyridones-targeting virulence of uropathogenic E. coli, Bioorg. Med. Chem., 2006, 14, 7563–7581 CrossRef PubMed.
- M. D. Evans, J. Ring, A. Schoen, A. Bell, P. Edwards, D. Berthelot, R. Nicewonger and C. M. Baldino, The accelerated development of an optimized synthesis of 1,2,4-oxadiazoles: application of microwave irradiation and statistical design of experiments, Tetrahedron Lett., 2003, 44, 9337–9341 CrossRef CAS.
- Code:logP(o/w). Molecular Operating Environment (MOE), version 2016.08, Chemical Computing Group, Quebec, Canada.
- G. M. Castanedo, P. S. Seng, N. Blaquiere, S. Trapp and S. T. Staben, Rapid synthesis of 1,3,5-substituted 1,2,4-triazoles from carboxylic acids, amidines, and hydrazines, J. Org. Chem., 2011, 76, 1177–1179 CrossRef CAS PubMed.
- J. Yang, M. Jamei, K. R. Yeo, A. Rostami-Hodjegan and G. T. Tucker, Misuse of the well-stirred model of hepatic drug clearance, Drug Metab. Dispos., 2007, 35, 501–502 CrossRef CAS PubMed.
- H. D. Caldwell, J. Kromhout and J. Schachter, Purification and partial characterization of the major outer membrane protein of Chlamydia trachomatis, Infect. Immun., 1981, 31, 1161–1176 CAS.
- S. Marwaha, H. Uvell, O. Salin, A. E. Lindgren, J. Silver, M. Elofsson and Å. Gylfe, N-acylated derivatives of sulfamethoxazole and sulfafurazole inhibit intracellular growth of Chlamydia trachomatis, Antimicrob. Agents Chemother., 2014, 58, 2968–2971 CrossRef PubMed.
- I. Hubatsch, E. G. E. Ragnarsson and P. Artursson, Determination of drug permeability and prediction of drug absorption in Caco-2 monolayers, Nat. Protoc., 2007, 2, 2111 CrossRef CAS PubMed.
- K. Riccardi, S. Cawley, P. D. Yates, C. Chang, C. Funk, M. Niosi, J. Lin and L. Di, Plasma protein binding of challenging compounds, J. Pharm. Sci., 2015, 104, 2627–2636 CrossRef CAS PubMed.
- H. Emtenäs, L. Alderin and F. Almqvist, An enantioselective ketene−imine cycloaddition method for synthesis of substituted ring-fused 2-pyridinones, J. Org. Chem., 2001, 66, 6756–6761 CrossRef PubMed.
- P. Baranczewski, A. Stanczak, K. Sundberg, R. Svensson, Å. Wallin, J. Jansson, P. Garberg and H. Postlind, Introduction to in vitro estimation of metabolic stability and drug interactions of new chemical entities in drug discovery and development, Pharmacol. Rep., 2006, 58(4), 453–472 CAS.
Footnotes |
† These authors contributed equally. |
‡ Division of Infection Medicine, BMC, Lund University, Sweden. |
§ ReViral Ltd, NETpark Plexus, Thomas Wright Way, Sedgefield, TS21 3FD, United Kingdom. |
|
This journal is © The Royal Society of Chemistry 2019 |