DOI:
10.1039/C9MD00291J
(Research Article)
Med. Chem. Commun., 2019,
10, 1900-1906
Structural modification of azolylacryloyl derivatives yields a novel class of covalent modifiers of hemoglobin as potential antisickling agents
Received
23rd May 2019
, Accepted 15th August 2019
First published on 23rd August 2019
Abstract
The intracellular polymerization and the concomitant sickling processes, central to the pathology of sickle cell disease, can be mitigated by increasing the oxygen affinity of sickle hemoglobin (HbS). Attempts to develop azolylacryloyl derivatives to covalently interact with βCys93 and destabilize the low-O2-affinity T-state (deoxygenated) HbS to the polymer resistant high-O2-affinity R-state (liganded) HbS were only partially successful. This was likely due to the azolylacryloyls carboxylate moiety directing the compounds to also bind in the central water cavity of deoxygenated Hb and stabilizing the T-state. We now report a second generation of KAUS compounds (KAUS-28, KAUS-33, KAUS-38, and KAUS-39) without the carboxylate moiety designed to bind exclusively to βCys93. As expected, the compounds showed reactivity with both free amino acid L-Cys and the Hb βCys93. At 2 mM concentrations, the compounds demonstrated increased Hb affinity for oxygen (6% to 15%) in vitro, while the previously reported imidazolylacryloyl carboxylate derivative, KAUS-15 only showed 4.5% increase. The increased O2 affinity effects were sustained through the experimental period of 12 h for KAUS-28, KAUS-33, and KAUS-38, suggesting conserved pharmacokinetic profiles. When incubated at 2 mM with red blood cells from patients with homozygous SS, the compounds inhibited erythrocyte sickling by 5% to 9%, respectively in correlation with the increase Hb-O2 affinity. These values compare to 2% for KAUS-15. When tested with healthy mice, KAUS-38 showed very low toxicity.
Introduction
Sickle cell disease (SCD) is the most common inherited hematologic disorder, affecting many areas in the world. The intracellular sickling process, central to the pathology of sickle cell disease can be mitigated by increasing the oxygen affinity of sickle hemoglobin (HbS).1–3 Aromatic aldehydes exhibit these pharmacologic properties by stabilizing the relaxed state of Hb (R2-state) and increase Hb affinity for oxygen via a Schiff-base interaction with the N-terminal αVal1 nitrogen, and through several inter-subunit mediated interactions.1–4 Several aromatic aldehydes have been studied in the clinic for the treatment of SCD, with one such compound, GBT440 (Voxelotor) currently undergoing phase III studies.4 With very few exceptions, e.g. GBT440, a major drawback of aromatic aldehydes as therapeutic agents is poor pharmacokinetic properties due to significant and rapid metabolic oxidation of the aldehyde moiety into the corresponding inactive functional metabolites.5–8 Hence, efforts are being made to replace the aldehyde group with different metabolically stable electrophiles.9–12 We recently studied several such covalent modifiers for their antisickling activity.11 The compounds were expected to increase oxygen affinity of Hb via a Michael addition reaction between their β-unsaturated carbon and the sulfur atom of βCys93 (Scheme 1), disrupting a T-state stabilization salt-bridge interaction, and shifting the allosteric equilibrium to the R-state.11 Unfortunately, the compounds, as exemplified by KAUS-15 (Fig. 1), only showed weak or no effect on Hb oxygen affinity, explaining their weak antisickling activities. Solution studies suggest that the compounds bind predominantly to free L-Cys and Hb βCys93. Crystallographic studies also showed a second binding to the N-terminal αVal1 at the central water cavity of deoxygenated Hb, with their carboxylate moieties involved in protein interactions that stabilize the T-state Hb, explaining the observed biological effect.11 This study reports a second generation of several KAUS molecules without the carboxylate moiety, and expected to bind exclusively to βCys93. The compounds were studied for their reactivity with βCys93 of Hb and free L-Cys, in vitro effects on Hb-O2 affinity and sickle RBC morphology, as well as acute toxicity with mice.
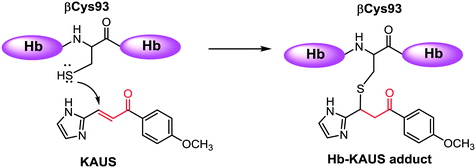 |
| Scheme 1 Schematic representation of the Michael addition reaction between the β-unsaturated carbon of Michael addition compound and the βCys93 sulfur of hemoglobin. | |
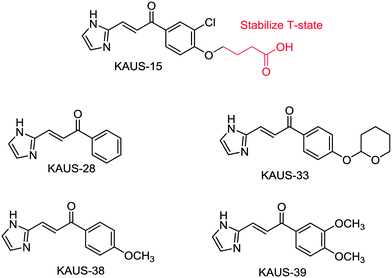 |
| Fig. 1 Azolylacryloyl derivatives without carboxylate functional group. | |
Materials and methods
General
All melting points were uncorrected and measured using the capillary melting point instrument BI 9100 (Barnstead Electrothermal, UK). 1H NMR spectra were determined on an AVANCE-III 600 MHz and AVANCE-III HD 850 MHz spectrometers (Bruker, Germany), and chemical shifts are expressed as ppm against TMS as an internal standard (King Fahd Center for Medical Research and Faculty of Science, King Abdulaziz University, Jeddah, Saudi Arabia). LC/MS analyses were performed on an Agilent 6320 Ion Trap HPLC-ESI-MS/DAD (Santa Clara, CA, USA) with the following settings: the analytes were separated using a Macherey-Nagel Nucleodur-C18 column (150 mm length × 4.6 mm i.d., 5 μm) (Macherey-Nagel GMBH & Co. KG, Duren, Germany). Mobile phase; isocratic elution using a mixture of acetonitrile and 0.01 formic acid in water (80
:
20, v/v). The flow rate was 0.4 mL min−1; total run time = 20 min. Purities are reported according to percentage of Peak Areas at wavelength 280 nm. According to LC/MS analyses, all compounds in this study were confirmed to have 95% purity or higher. High-resolution mass spectrometry (HRMS) was performed in the Faculty of Science, King Abdulaziz University on Impact II™ Q-TOF spectrometer (Bruker, Germany).
De-identified, leftover EDTA venous sickle whole blood samples from patients with SCD who visited The Children's Hospital of Philadelphia for routine clinic visits, were obtained after informed consent. Normal whole blood were obtained from adult donors at the Virginia Commonwealth University after informed consent.
Synthesis of (E)-3-(1H-imidazol-2-yl)-1-phenylprop-2-en-1-one (KAUS-28)
1H-Imidazole-2-carbaldehyde (2.88 g, 30 mmol) and acetophenone (4.32 g, 4.2 mL, 36 mmol) were dissolved in 40 ml of ethanol, and a 1 N aqueous solution (40 ml) of sodium hydroxide was added to the solution. The mixture was stirred at room temperature for 8 h. 1 N hydrochloric acid (40 ml) was added to the resultant reaction mixture to conduct the extraction with chloroform. An organic layer was washed with saturated brine, dried and then concentrated under reduced pressure. The resultant residue was subjected to column chromatography on silica gel. Crystals obtained from a fraction eluted with 1% (v/v) methanol–chloroform were recrystallized from ethyl acetate to obtain the titled compound as off-white powder (2.1 g, 60%). MP: 182–184 °C. 1H NMR (850 MHz, DMSO-d6) δ ppm 7.25–7.38 (m, 1H) 7.51 (d, J = 15.57 Hz, 1H) 7.60 (t, J = 7.79 Hz, 2H) 7.69 (t, J = 7.27 Hz, 1H) 7.87 (d, J = 15.57 Hz, 1H) 8.05 (d, J = 7.27 Hz, 2H). 13C NMR (151 MHz, DMSO-d6) δ 188.8, 143.4, 137.4, 133.2, 132.0, 128.9, 128.2, 121.1. HRMS (ESI) calculated m/z 199.0866; found m/z 199.0850 (M+).
Synthesis of (E)-3-(1H-imidazol-2-yl)-1-(4-((tetrahydro-2H-pyran-2-yl)oxy)phenyl)prop-2-en-1-one (KAUS-33)
1H-Imidazole-2-carbaldehyde and 1-(4-((tetrahydro-2H-pyran-2-yl)oxy)phenyl)ethan-1-one were reacted to afford a white solid (4.9 g, 51%) from (E)-3-(1H-imidazol-2-yl)-1-(4-((tetrahydro-2H-pyran-2-yl)oxy)phenyl)prop-2-en-1-one (KAUS-33) by the same procedure described above for the synthesis of KAUS-28. MP: 186–187 °C. 1H NMR (850 MHz, DMSO-d6) δ ppm 1.52–1.57 (m, 1H) 1.58–1.68 (m, 2H) 1.76–1.80 (m, 1H) 1.83–1.92 (m, 2H) 3.60 (dt, J = 11.29, 4.22 Hz, 1H) 3.72–3.76 (m, 1H) 5.66 (t, J = 3.37 Hz, 1H) 7.19–7.22 (m, 2H) 7.43 (br. s., 1H) 7.47 (d, J = 15.57 Hz, 1H) 7.87 (d, J = 15.57 Hz, 1H) 8.03–8.06 (m, 1H) 12.82 (br. s., 1H). 13C NMR (151 MHz, DMSO-d6) δ 187.0, 160.5, 143.5, 131.3, 131.1, 130.9, 130.4, 130.0, 121.1, 119.9, 116.2, 115.5, 95.4, 61.7, 61.6, 29.5, 24.5, 18.3. HRMS (ESI) calculated m/z 299.1390; found m/z 299.1337 (M+).
Synthesis of (E)-3-(1H-imidazol-2-yl)-1-(4-methoxyphenyl)prop-2-en-1-one (KAUS-38)
This compound was prepared following the procedure described above for the synthesis of the similar analogue KAUS-28 and was obtained as a white solid (3.6 g, 53%). MP: 193–194 °C. 1H NMR (850 MHz, DMSO-d6) δ ppm 3.88 (s, 3 H) 7.11–7.14 (m, 1H) 7.18 (br. s., 1H) 7.43 (br. s., 1H) 7.47 (d, J = 15.57 Hz, 1H) 7.89 (d, J = 15.57 Hz, 1H) 8.04–8.08 (m, 1H) 12.82 (br. s., 1H).
Synthesis of (E)-1-(3,4-dimethoxyphenyl)-3-(1H-imidazol-2-yl)prop-2-en-1-one (KAUS-39)
This compound was prepared following the procedure described above for the synthesis of the similar analogue KAUS-28 and was obtained as an off-white solid (2.7 g, 37%). MP: 222–223 °C. 1H NMR (400 MHz, DMSO-d6) δ ppm 3.88 (s, 3H) 3.90 (s, 3H) 7.16 (d, J = 8.53 Hz, 1H) 7.20–7.34 (m, 1H) 7.37 (br. s., 1H) 7.50 (d, J = 15.56 Hz, 1H) 7.58 (d, J = 2.01 Hz, 1H) 7.78 (dd, J = 8.53, 2.01 Hz, 1H) 7.90 (d, J = 15.56 Hz, 1H).
Oxygen equilibrium curve (OEC) studies.
The ability of the compounds, KAUS-28, KAUS-33, KAUS-38, and KAUS-39 (1 mM and 2 mM final concentration) to increase hemoglobin affinity for oxygen was tested with normal whole blood (hematocrit of 20%) with 2 h incubation at 37 °C as previously reported.11,13,14 The studies were performed at least in triplicates. Time dependent studies were also conducted with whole blood at 2 mM concentration, with incubation for up to 24 h at 37 °C. Aliquot samples were tested at 1, 2, 4, 8, and 24 h, and DMSO was used as control. KAUS-15 was used as a control.
Antisickling studies.
All compounds, KAUS-28, KAUS-33, KAUS-38, KAUS-39, and the control KAUS-15 were tested for their abilities to prevent RBC sickling under hypoxic condition following previously reported assay.11,13,14 Briefly SS cells were suspended in Hemox buffer (TCS Scientific Corp, Southampton, PA), pH 7.4, supplemented with 10 mM glucose and 0.2% bovine serum albumin. The solution was incubated under air in the absence (control) or presence of 2 mM concentration of test compound at 37 °C for 1 h. Following, the suspension was incubated under hypoxic condition (4% oxygen/96% nitrogen) at 37 °C for 2 h. The suspension was fixed with 2% glutaraldehyde solution without exposure to air and then subjected to microscopic morphological analysis as previously reported.13
Reactivity of KAUS compounds toward free amino acid, L-cysteine
The KAUS compounds were expected to covalently bind to Hb through a Michael addition reaction with the βCys93 to affect their allosteric and antisickling activities. We therefore investigated L-Cys conjugates with KAUS-28, KAUS-33, KAUS-38 and KAUS-39 using UPLC-MS analysis as previously described11 with an Acquity H-Class UPLC, which is connected to a PDA detector and an Acquity TQD detector. The column used was an Acquity UPLC BEH C18 1.7 μm, 2.1 × 50 mm, with a Vanguard pre-column attached. Solvent A consisted of water with 0.02% formic acid, while solvent B consisted of 90
:
10 acetonitrile
:
water with 0.02% formic acid. A gradient run was performed such that solvent B was increased from 0% B to 100% B from time 0–5 min, followed by 3 minute wash at 100% B and then a return and re-equilibration at 100% A in the next 4 min. The flow rate was maintained at 0.5 ml min−1 throughout the run. 2 μL of sample was injected per run. The eluent of the column was connected to a PDA UV detector, which scanned from 220–350 nm and showed a 2D channel of 280 nm. The eluent was then introduced into the TQD detector. The TQD detector was set at positive ionization mode with a capillary voltage of 3.20 kV, cone voltage of 20 V, extractor voltage of 1 V, and RF lens voltage of 0.1 V. The source temperature was set at 150 °C, while the desolvation temperature was set at 350 °C and the desolvation and cone gas flows were set at 650 and 50 L h−1 respectively. Scans were made from 100–700 m/z with scan duration of 0.5 seconds to obtain mass spectra at different time points.
Reactivity of KAUS compounds toward βCys93 of Hb
The accessible sulfhydryl groups in Hb, and their reactivity with the KAUS compounds, were quantified by observing the results of the disulfide exchange reaction of the thiols of βCys93 and DTNB at 412 nm (ε = 14
150 M−1 cm−1).11 An aqueous solution of Hb (50 μM in PBS) was mixed with KAUS-28, KAUS-38, KAUS-39 and the positive control KAUS-15 (2 mM final concentration) in a final volume of 500 μL. The mixture was incubated at room temperature for 2 h or 16 h. The mixture was initially centrifuged at 10
000 rpm for 10 min at 4 °C to settle any precipitate that may have formed during the reaction. The supernatant was transferred to a microfiltration centrifugal tube (MWCO 10 kDa) and was centrifuged to separate Hb from excess reagents at 7000 rpm for 30 min at 4 °C. Hb was washed with PBS and centrifuged again to a final volume of 100 μL. The washed Hb was stored at 4 °C. 25 μL of each Hb solution was added to 0.1 M potassium phosphate buffer (475 μL) at pH 8.0 and incubated at 25 °C for 1 h (non-DTNB control samples). Another 25 μL of each Hb solution was added to the phosphate buffer (465 μL) with 10 μL of DTNB (10 mM in buffer) and incubated at 25 °C for 1 h. Before centrifuging the non-DTNB control samples, the absorbance of each sample was taken at 576 nm to determine the concentration of Hb and quantify the βCys93 concentration in each sample. Both sets of tubes were centrifuged using different centrifugal filters (7000 rpm, 20 min, 4 °C) to collect the yellow filtrate (2-nitrobenzoate), which was quantified by measuring absorbance at 412 nm.
Determination of acute oral toxicity (LD50)
The experiment was conducted on 9 healthy Swiss albino mice (males and females) weighing 22–27 g and aged 8 to 10 weeks obtained from the Animal Station, Pharmacology Dept., Faculty of Pharmacy, King Abdulaziz University, Jeddah. All animals were kept at the regulated temperature (average 23 °C), air quality (central air conditioning) and light (12 h light/dark cycles). Animals were provided free access to food pellets ad libitum and water. All animal procedures were performed in accordance with the Guidelines for Care and Use of Laboratory Animals of King Abdulaziz University and approved by the Animal Ethics Committee of Research on living Creatures in the Kingdom of Saudi Arabia. Animals were humanely treated according to international and scientific principles. KAUS-38 of purity 95% or more (LC/MS) was prepared as 10% suspension in water containing 0.5% tween 80. Mice (n = 3) were treated with an oral starting dose of KAUS-38 of 2000 mg per kg body weight using a stainless-steel gouge gavage needle. On day 2, same steps, also using an oral dose of KAUS-38 of 2000 mg per kg body weight were repeated on a second group of mice (n = 3). On day 3, an oral dose of KAUS-38 of 5000 mg per kg body weight was administrated on a third group of mice (n = 3). Following each dose, the animals were observed for 24 h for any signs of toxicity including mortality.
Result and discussion
Scheme 2 was followed for the synthesis of KAUS-28, KAUS-33, KAUS-38 and KAUS-39 (Fig. 1). The control, KAUS-15 was synthesized as previously published.11 For the synthesis, 2-imidazole carbaldehyde (2.88 g, 30 mmol) and substituted acetophenone (36 mmol) were dissolved in 40 ml of ethanol, and a 1 N aqueous solution (40 ml) of sodium hydroxide was added to the solution. The mixture was stirred at room temperature for 8 h. 1 N hydrochloric acid (40 ml) was added to the resultant reaction mixture to conduct the extraction with chloroform. The organic layer was washed with saturated brine, dried and then concentrated under reduced pressure. The resultant residue was subjected to column chromatography on silica gel. Product obtained from a fraction eluted with 1% (v/v) methanol–chloroform was recrystallized from ethyl acetate.
 |
| Scheme 2 | |
Compounds react with both free L-Cys and βCys93 of Hb
UPLC-MS analysis of the reaction between the compounds and free L-Cys (that was incubated for 3 h) is shown in Table 1. KAUS-38 was the most reactive (77%), followed by KAUS-33 (55%), KAUS-39 (54%), while KAUS-28 showed the least reactivity (20%). As previously reported, solution-based sulfhydryl assay identified only two accessible thiols, out of the six present in Hb, consistent with the two βCys93 as the only solvent accessible and reactive cysteine.11 The reactivity of the KAUS compounds (KAUS-28, KAUS-38 and KAUS-39) with these accessible sulfhydryl groups in Hb was quantified by the disulfide exchange reaction of the thiols of βCys93 in Hb and DTNB at 412 nm. All three compounds showed 10% reactivity with βCys93 at 2 h incubation, which increased to 37% for KAUS-38 and 20% for both KAUS-28 and KAUS-39 after 16 h (Table 2). The positive control KAUS-15 shows comparative values of 10% and 30% at 2 h and 16 h, respectively. It is also apparent that the KAUS compounds are more reactive toward free Cys than βCys93 of Hb, which could be due to steric constraint to reacting with the partially buried βCys93.
Table 1 UPLC-MS measurements of relative ratio of azolylacryloyl compounds and their adduct products with L-cysteine
Amino acid |
Compound |
Peak |
RTa (min) |
Max. m/z |
Area |
% compoundb or adduct |
RT = retention time.
% compound or adduct = (area of compound or adduct/total area) × 100.
Loss of THP is due to phosphonic acid treatment.
|
Cysteine |
KAUS-28 |
Compound |
2.09 |
199.1 |
3997 |
80.4 |
Adduct |
1.61 |
320.2 |
972 |
19.6 |
KAUS-33c |
Compound |
1.77 |
215.1c |
5238 |
45.1 |
Adduct |
1.27 |
336.2c |
6387 |
54.9 |
KAUS-38 |
Compound |
2.21 |
229.1 |
2528 |
23.0 |
Adduct |
1.81 |
350.2 |
8450 |
77.0 |
KAUS-39 |
Compound |
2.12 |
259.1 |
3921 |
46.3 |
Adduct |
1.74 |
380.2 |
4555 |
53.7 |
Table 2 Relative ratios of free thiol from βCys93 in Hb and their adduct products with azolylacryloyl compounds
Compound |
Incubation time (h) |
% available – SH from two βCys93 |
% adduct formed |
Values are mean of quadruplet measurements ± SD. % adduct formed = 100 – (available – SH from two βCys93).
|
KAUS-15 |
2 |
89 ± 4 |
11 |
16 |
70 ± 5 |
30 |
KAUS-28 |
2 |
90 ± 9 |
10 |
16 |
79 ± 1 |
21 |
KAUS-38 |
2 |
89 ± 4 |
11 |
16 |
63 ± 5 |
37 |
KAUS-39 |
2 |
91 ± 5 |
9 |
16 |
80 ± 3 |
20 |
Compounds increased Hb affinity for oxygen with sustained effect
The compounds were subjected to oxygen equilibrium studies using 3-point tonometry to assess P50 shifts or increase in Hb oxygen affinity. P50 is the oxygen pressure at which hemoglobin is 50% saturated with oxygen. All compounds increased Hb affinity for oxygen, showing a left shift in the OEC curve, when tested with normal whole blood (Fig. 2). The compounds also showed concentration-dependent OEC effect. In whole blood KAUS-33 showed the most potent effect (9.9 and 15.2% at 1 mM and 2 mM, respectively), followed by KAUS-38 (8.2 and 11.8%), then KAUS-28 (7.0 and 11.3%), and KAUS-39 (∼6.5%) (Fig. 3). These values compare with the positive control, KAUS-15 at 1.9 and 4.5%, respectively, clearly indicating improvement in the allosteric properties of these new compounds. Unlike KAUS-15, these compounds do not contain carboxylate function, and therefore we attribute the increased potency to the removal of the carboxylate moiety, which is expected to exclude binding to the central water cavity of deoxygenated Hb to stabilize the T-state.
 |
| Fig. 2 Representative OEC (KAUS-38) showing a dose-dependent increase in Hb oxygen affinity. | |
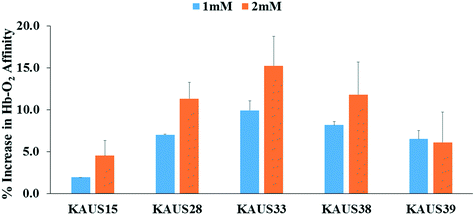 |
| Fig. 3 Degree of shift in oxygen equilibria (ΔP50) by test compounds expressed in percentage. Data reported are mean values and standard deviations; from at least three replicate experiments. | |
Time-dependent OEC studies showed that at 2 mM concentration, KAUS-28, KAUS-33, and KAUS-38 sustained their activity throughout the 24 h experiment, while KAUS-39 started to decline after 8 h (Fig. 4). A similar activity decline was also observed with KAUS-15. It is also interesting to point out that KAUS-39, among the five compounds studied (including KAUS-15) showed the slowest onset. The apparent metabolic stability of these compounds' contrasts with most aromatic aldehyde antisickling agents, which suffer from rapid metabolism and thus have short pharmacologic activities. Thus, as proposed, the current azolylacryloyl derivatives appear to have superior in vitro allosteric effect than the carboxylate-containing azolylacryloyl, as well as are metabolically stable than some of the previously studied antisickling aromatic aldehydes.5,7,13,15 The four KAUS compounds differ by having varying, or no substitutions on the phenyl ring. However, it does not appear that the size of the substitution correlates with the allosteric activities of these compounds, suggesting that the substituted phenyl ring may not be important in binding.
 |
| Fig. 4 Time-dependent P50 shift of Hb in normal blood incubated with test compounds. | |
Compounds demonstrated mild in vitro antisickling effect on blood samples from subjects with homozygous sickle cell disease
Compounds that increase the oxygen affinity of Hb are expected to inhibit RBC sickling as high-O2-affinity sickle Hb does not polymerize.2,14,16 As expected from their ability to increase the oxygen affinity of Hb, all the KAUS compounds inhibited erythrocyte sickling, with KAUS-38 showing the most potent effect of 9%, followed by KAUS-33 and KAUS 28 (7%), then KAUS-39 (5%), and lastly the control KAUS-15 (2%) (Fig. 5).
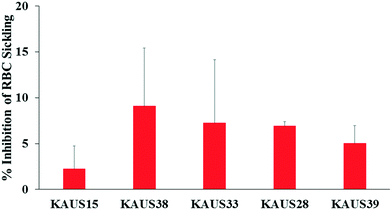 |
| Fig. 5 Inhibition of SS cell sickling by KAUS compounds. Data reported are mean values and standard deviations; from at least three replicate experiments. | |
Compounds demonstrated low toxicity in healthy mice
Since compounds with electrophilic properties, e.g. Michael addition are usually involved in a variety of toxic effects on mammalians, we decided to investigate the general acute toxicity of KAUS-38 by determining the in vivo lethal dose (LD50)17 according to the Globally Harmonized Classification System (GHS) and following the OECD guideline 423 (modified, adopted March 23, 2006). Healthy Swiss albino mice (males and females) weighing 22–27 g and aged 8 to 10 weeks were divided into 3 groups (3 mice in each group). Group 1 and 2 were given an oral dose of 2000 mg per kg body weight while group 3 was given an oral dose of 5000 mg per kg body weight. Following dosing, the mice was observed for 24 h for any signs of toxicity, including mortality. It was observed that all mice tolerated an oral dose of 2000 mg kg−1 of KAUS-38, since no mice from groups 1 and 2 died after 24 h of drug administration. However, 2 out of the 3 mice in group 3 (given 5000 mg kg−1 doses) were found to be dead. This preliminary toxicity study suggests KAUS-38 to have potentially low toxicity on mammals. We note that the excipient used for the in vivo delivery were much better in solubilizing the compounds, but not appropriate for in vitro studies. The toxicity result is consistent with our published cellular study with similar Michael addition class of compounds that are show more solubility, which also showed no toxicity effect.11 Further toxicity studies are warranted for sub-chronic and chronic ranges to confirm safety of the compounds.
Conclusion
We previously developed azolylacryloyl-carboxylate compounds that we hypothesize would bind covalently to βCys93 of Hb and destabilize the T-state to increase the protein affinity for oxygen that would translate to antisickling effects.11 These compounds, with the representative example of KAUS-15 showed only marginal effect in increasing Hb oxygen affinity or preventing RBC sickling.11 Crystallographic studies suggested that their low functional/biological activities are in part due to the compounds binding to the central water cavity of deoxygenated Hb to stabilize the T-state.11 In the present study, we modified some of these compounds by removing their carboxylate groups to allow the compounds to bind preferentially at βCys93 and exhibit more potent OEC and/or antisickling activities. Four compounds were synthesized, and as expected exhibited improvement in vitro Hb-O2 affinity and RBC sickling inhibition over KAUS-15, although not as potent as expected. Nonetheless, our findings suggest that our structural modifications are in the right direction, and establish the current non-carboxylate azolylacryloyl derivatives as a novel and potentially non-toxic promising group of anti-sickling agents, and provide proof-of-concept justifications for further structural modifications to develop candidate therapeutics for SCD.
All human experiments were performed in accordance with the Guidelines of the Institutional Review Boards (IRB) of The Children's Hospital of Philadelphia and Virginia Commonwealth University, and experiments were approved by the ethics committee at The Children's Hospital of Philadelphia and Virginia Commonwealth University, respectively. Informed consents were obtained from human participants of this study.
Conflicts of interest
The Azolylacryloyl derivatives are under consideration for patent protection.
Acknowledgements
The authors acknowledge with thanks technical and financial support by Deanship of Scientific Research (DSR) at King Abdulaziz University, Jeddah, under grant no. (RG-1-166-39). Other financial support by NIH/NIMHD grant MD009124/MD009124S1 (MKS). Special thanks to Professor Ashraf B. Abdel-Naim, Pharmacology and Toxicology Dept., King Abdulaziz University for valuable guidance in conducting the LD50 study.
References
- M. K. Safo and G. J. Kato, Therapeutic strategies to alter the oxygen affinity of sickle hemoglobin, Hematol. Oncol. Clin. North Am., 2014, 28(2), 217–231 CrossRef PubMed.
- M. K. Safo, M. H. Ahmed, M. S. Ghatge and T. Boyiri, Hemoglobin-ligand binding: understanding Hb function and allostery on atomic level, Biochim. Biophys. Acta, 2011, 1814(6), 797–809 CrossRef CAS PubMed.
-
M. K. Safo and S. Bruno, in Allosteric Effectors of Hemoglobin: Past, Present and Future, Chemistry and Biochemistry of Oxygen Therapeutics, ed. A. Mozzarelli and S. Bettati, John Wiley & Sons, Ltd, 2011, pp. 285–300, Available from: http://onlinelibrary.wiley.com/doi/10.1002/9781119975427.ch21/summary Search PubMed.
- Study to Evaluate the Effect of GBT440 Administered Orally to Patients With Sickle Cell Disease (GBT_HOPE) - Full Text View - ClinicalTrials.gov [Internet], [Cited 2018 Mar 21], Available from: https://clinicaltrials.gov/ct2/show/NCT03036813.
- G. G. Xu, P. P. Pagare, M. S. Ghatge, R. P. Safo, A. Gazi and Q. Chen,
et al., Design, Synthesis, and Biological Evaluation of Ester and Ether Derivatives of Antisickling Agent 5-HMF for the Treatment of Sickle Cell Disease, Mol. Pharmaceutics, 2017, 14(10), 3499–3511 CrossRef CAS PubMed.
- A. Parikh and J. Venitz, Novel In Vitro Target-site Drug Disposition (TSDD)/Pharmacodynamic (PD) Model for 5-Hydroxymethylfurfural (5-HMF) in Human Whole Blood, Clin. Pharmacol. Ther., 2014, 95(1), S83–S84 Search PubMed.
- V. B. Godfrey, L. J. Chen, R. J. Griffin, E. H. Lebetkin and L. T. Burka, Distribution and metabolism of (5-hydroxymethyl)furfural in male F344 rats and B6C3F1 mice after oral administration, J. Toxicol. Environ. Health, Part A, 1999, 57(3), 199–210 CrossRef CAS.
- D. J. Abraham, A. S. Mehanna, F. C. Wireko, J. Whitney, R. P. Thomas and E. P. Orringer, Vanillin, a potential agent for the treatment of sickle cell anemia, Blood, 1991, 77(6), 1334–1341 CAS.
- S. Park, B. L. Hayes, F. Marankan, D. C. Mulhearn, L. Wanna and A. D. Mesecar,
et al., Regioselective Covalent Modification of Hemoglobin in Search of Antisickling Agents, J. Med. Chem., 2003, 46(6), 936–953 CrossRef CAS PubMed.
- A. Nakagawa, F. E. Lui, D. Wassaf, R. Yefidoff-Freedman, D. Casalena and M. A. Palmer,
et al., Identification of a Small Molecule that Increases Hemoglobin Oxygen Affinity and Reduces SS Erythrocyte Sickling, ACS Chem. Biol., 2014, 9(10), 2318–2325 CrossRef CAS PubMed.
- A. M. Omar, M. A. Mahran, M. S. Ghatge, N. Chowdhury, F. H. Bamane and M. E. El-Araby,
et al., Identification of a novel class of covalent modifiers of hemoglobin as potential antisickling agents, Org. Biomol. Chem., 2015, 13(22), 6353–6370 RSC.
- A. M. Omar, M. A. Mahran, M. S. Ghatge, F. H. A. Bamane, M. H. Ahmed and M. E. El-Araby,
et al., Aryloxyalkanoic Acids as Non-Covalent Modifiers of the Allosteric Properties of Hemoglobin, Molecules, 2016, 21(8), 1057 CrossRef PubMed.
- O. Abdulmalik, M. K. Safo, Q. Chen, J. Yang, C. Brugnara and K. Ohene-Frempong,
et al., 5-hydroxymethyl-2-furfural modifies intracellular sickle haemoglobin and inhibits sickling of red blood cells, Br. J. Haematol., 2005, 128(4), 552–561 CrossRef CAS PubMed.
- O. Abdulmalik, M. S. Ghatge, F. N. Musayev, A. Parikh, Q. Chen and J. Yang,
et al., Crystallographic analysis of human hemoglobin elucidates the structural basis of the potent and dual antisickling activity of pyridyl derivatives of vanillin, Acta Crystallogr., Sect. D: Biol. Crystallogr., 2011, 67(Pt 11), 920–928 CrossRef CAS PubMed.
- A. Parikh, Poster Session II (PII-001–120) Displayed 7:30 am – 3:30 pm Attended 11:45 am – 1:15 pm, Clin. Pharmacol. Ther., 2014, 95, S83–S84 Search PubMed.
- M. K. Safo, O. Abdulmalik, R. Danso-Danquah, J. C. Burnett, S. Nokuri and G. S. Joshi,
et al., Structural basis for the potent antisickling effect of a novel class of five-membered heterocyclic aldehydic compounds, J. Med. Chem., 2004, 47(19), 4665–4676 CrossRef CAS PubMed.
- S. M. Shoieb, A. Esmat, A. E. Khalifa and A. B. Abdel-Naim, Chrysin attenuates testosterone-induced benign prostate hyperplasia in rats, Food Chem. Toxicol., 2018, 111, 650–659 CrossRef CAS PubMed.
|
This journal is © The Royal Society of Chemistry 2019 |
Click here to see how this site uses Cookies. View our privacy policy here.