DOI:
10.1039/C9FO01806A
(Paper)
Food Funct., 2019,
10, 7926-7939
Cocoa ameliorates renal injury in Zucker diabetic fatty rats by preventing oxidative stress, apoptosis and inactivation of autophagy
Received
7th August 2019
, Accepted 28th October 2019
First published on 29th October 2019
Abstract
Redox balance, autophagy and apoptosis are main processes involved in the development of diabetic nephropathy. Epidemiological and animal studies suggest that cocoa might reduce the risk of diabetic complications. However, the molecular mechanisms responsible for these potential preventive activities and whether cocoa exerts beneficial effects on dysregulated signalling pathways involved in cellular antioxidant defence, autophagy and apoptosis in the diabetic kidney remain largely unknown. Therefore, this work investigated the effect of a cocoa-rich diet on the mentioned processes in the renal cortex of Zucker Diabetic Fatty (ZDF) rats. Male ZDF rats were fed either a control or cocoa-rich diet (10%), and Zucker lean animals received the control diet (10–20 weeks-of-life). ZDF rats fed with cocoa decreased body weight and glucose and insulin levels, and improved renal function. Cocoa intake further prevented the enhanced renal cortical oxidative stress in diabetic rats by regulating the antioxidant defence system and close-related proteins to cytoprotection and cell response; thus, cocoa diminished oxidative markers (reactive oxygen species and carbonyl groups) and NADPH-oxidase-4 levels, and restored key enzymatic antioxidant activities (superoxide dismutase and catalase), nuclear-erythroid-2-related factor-2, and ERK-MAPK levels, as well as sirtuin-1/5′-AMP-activated-protein kinase signalling. Moreover, in ZDF rats cocoa-rich diet contributed to alleviation of the renal cortical injury through autophagy activation (p62 upregulation, and downregulation of beclin-1 and LC3), and inhibition of apoptosis (Bcl-xL stimulation and suppression of Bax and caspases-9 and -3). These findings provide the first in vivo evidence on the molecular mechanisms of cocoa to circumvent renal cortical damage that involve improvement of antioxidant competences, stimulation of autophagy and suppression of apoptosis in ZDF rats.
1. Introduction
Diabetes often leads to other complications, and among them the diabetic kidney disease constitutes one of the main causes of death in diabetic patients.1 In the diabetic kidney disease, at the earliest stage renal hyperfunction and hypertrophy are present, but later a persistent albuminuria and a progressive decline in the glomerular filtration rate appear.2 Interestingly, one third of patients with diabetic kidney disease have marked tubular lesions and minimal glomerular alterations.2 However, in this disease the role of the glomerulus has typically been examined in contrast to the tubular compartment, which has been scarcely studied although it is earlier altered than glomeruli, as recently shown in ref. 2,3.
In diabetes, numerous underlying pathophysiological mechanisms have been described, but it is becoming clear that in this complicated metabolic disorder the increased oxidative stress, which is accompanied by augmented production of free radicals and/or impaired antioxidant defences, plays a central role in the development and progression of this disease.4 Consequently, inadequate responses related to the systemic enzymatic and non-enzymatic antioxidant defences, including glutathione (GSH), and the enzymes glutathione peroxidase (GPx), glutathione reductase (GR), superoxide dismutase (SOD), and catalase (CAT), have been reported in diabetic patients.5,6 In this context, the redox-sensitive transcription nuclear factor erythroid 2-related factor 2 (Nrf2), among others, constitutes a key protein for modulating the antioxidant response.7
Persistent oxidative stress due to the chronic hyperglycaemia also contributes to the early tubular injury and to the progression of the diabetic kidney disease,4 NADPH oxidases (NOX) being major sources of radical oxygen species (ROS) in this disease.8 In addition, oxidative stress results in the dysregulation of numerous cellular signalling pathways involved in cell response to the insult [mitogen-activated protein kinases (MAPKs), sirtuin-1 (SIRT-1), 5′-AMP-activated protein kinase (AMPK), mammalian target of rapamycin (mTOR)], apoptosis (Bcl-2 family proteins, caspases), and autophagy (beclin-1, p62, LC3), among others.7,9,10 Consequently, antioxidant therapies aimed to prevent the oxidative stress and the dysregulation of close-related processes (apoptosis, autophagy) might constitute a promising approach in the diabetic kidney disease.
Cocoa is a rich source of antioxidant compounds, mainly flavanols (epicatechin and procyanidins), and it is accepted as a powerful antioxidant food.11–13 Different studies have also demonstrated that cocoa exerts antidiabetic effects related to the improvement of glucose tolerance and insulin sensitivity in diverse tissues on several animal models of diabetes and in humans.14–17 In addition, it has been reported that main cocoa flavanols, such as procyanindin B2, exert renoprotective effects in diabetes by preserving the organ functionality and morphology.18 In view of all this, it could be suggested that cocoa might modulate in the tubular compartment crucial processes involved in the development of the diabetic kidney disease, such as oxidative stress, autophagy and apoptosis. However to the best of our knowledge, the potential antidiabetic effect of cocoa related to the modulation of the mentioned key processes in the kidney in an in vivo model of type 2 diabetes remains to be evaluated. Therefore, this study was aimed at investigating the potential protective effect of a cocoa-rich diet on the redox status, autophagy and apoptosis, and exploring the underlying molecular mechanisms in the renal cortex of male Zucker diabetic fatty [ZDF; ZDF/crl-lepr (fa/fa)] rats.
2 Materials and methods
2.1. Materials and chemicals
Glutathione reductase (GR), reduced and oxidized glutathione (GSH and GSSG, respectively), nicotine adenine dinucleotide reduced salt (NADH), nicotine adenine dinucleotide phosphate reduced salt (NADPH), tert-butylhydroperoxide (t-BOOH), o-phthaldehyde (OPT), dichlorofluorescein (DCFH), dinitrophenylhidazine (DNPH), 3,3′-diaminobenzidine (DAB), hydrogen peroxide, proteinase K, and a SOD determination kit were purchased from Sigma Chemicals (Madrid, Spain). A rat insulin ELISA kit was obtained from Mercodia (AD Bioinstruments, Barcelona, Spain). An albumin kit was purchased from Spinreact (BioAnalitica, Madrid, Spain), and uric acid and creatinine kits were from Linear Chemicals S.L. (Barcelona, Spain). Anti-ERK1/2 and antiphospho-ERK1/2 recognizing phosphorylated Thr202/Thy204 of ERK1/2, anti-JNK1/2 and antiphospho-JNK1/2 recognizing phosphorylated Thr183/Tyr185 of JNK1/2, anti-phospho-Thr180/Tyr182-p38, anti-active cleaved caspase-3, anti-active cleaved caspase-9, anti-AMPK and anti-phospho-Thr172-AMPK, anti-phospho-Ser2448-mTOR, and anti-β-actin were obtained from Cell Signalling Technology (Izasa, Madrid, Spain). Anti-p38α (sc-535), anti-NOX-4 (sc-30141), anti-Bcl-x (sc-634), anti-Bax (sc-526), anti-p62 (sc-48389), anti-beclin-1 (sc-48341), anti-LC3 (sc-398822), and anti-SIRT-1 (sc-74465) were purchased from Santa Cruz (Qimigen, Madrid, Spain). Anti-phospho-Ser40-Nrf2 was obtained from Signalway antibody (Quimigen, Madrid, Spain), and anti-mTOR was purchased from Upstate (Izasa, Madrid, Spain). Anti-PCNA Ab-1 was from Lab Vision Corporation (Bionova científica SL. Madrid, Spain). Terminal Transferase recombinant was purchased from Roche Applied Science (Roche Diagnostic, GMbH, Spain). Materials and chemicals for electrophoresis and Bradford reagent were from BioRad Laboratories S.A. (Madrid, Spain).
2.2. Cocoa
Natural Forastero cocoa powder (a kind gift from Idilia S.L., Barcelona, Spain) was used for this study. A detailed description of this cocoa is given elsewhere.14,19 Diets were prepared from an AIN-93G formulation (Panlab S.L., Barcelona, Spain) and provide all nutrients required by adult rats. The 10% cocoa diet was produced by adding 100 g kg−1 cocoa to AIN-93G at the expense of starch and cellulose. As a result, the cocoa diet was slightly richer in proteins (2.2%) and lipids (1.1%) but slightly inferior in carbohydrate (4.2%), to make both diets isocaloric (3612 kcal kg−1 diet). The rationale to select this cocoa dose is based on previous studies in which beneficial effects, also against diabetes, have been shown.14,19,20 The composition of the diets is given in Table 1.
Table 1 Composition of the experimental control and cocoa-rich diets
Components (g per kg dry weight) |
Control |
Cocoa |
Casein |
140 |
140 |
Dextrose |
155 |
155 |
Sucrose |
100 |
100 |
Fat |
40 |
40 |
tert-Butylhydroquinone |
0.008 |
0.008 |
Mineral mix. |
35 |
35 |
Vitamin mix. |
10 |
10 |
L-Cys |
1.8 |
1.8 |
Cholin bitartrate |
2.5 |
2.5 |
Cellulose |
100 |
66 |
Starch |
415.7 |
349.7 |
Cocoa powder |
— |
100 |
2.3. Animals and diets
Nine week-old male Zucker lean (ZL, n = 8) and Zucker diabetic ZDF/crl-lepr fa (n = 16) rats were purchased from Charles River Laboratories (L'Arbresle, France). These animals possess a mutation in the leptin receptor and spontaneously develop severe obesity, hyperglycaemia, hyperlipidaemia, and insulin resistance, being widely used as an animal model that mimics human type 2 diabetes.21 The rationale to select the duration of the treatment is based on previous studies focused on renal pathophysiology;22 thus, in 9 week-old rats renal alterations could be slightly noticed (pre-diabetic animals), whereas an intermediate stage of the diabetic kidney disease that can still be reverted is observed in 20 week-old ZDF rats.22 Rats were housed (in pairs) in cages in a controlled environment (19–23 °C, 50–60% humidity and 12 h light–dark cycles). After one week of acclimatization, ZDF rats were randomly assorted into two different experimental groups: one group received a standard diet (ZDF-C) and the other one was fed with the cocoa-supplemented diet (ZDF-Co). ZL rats remained as a unique group and were fed with the standard control diet. All experimental groups were provided with food and water ad libitum. All animal protocols were approved by the Bioethical Committees from Consejo Superior de Investigaciones Científicas (CSIC, Madrid, Spain) and Universidad Complutense de Madrid (UCM, Madrid, Spain), and the regional Committee for Laboratory Animals Welfare (Comunidad de Madrid, Ref. number PROEX-304-15), and all the animals were treated according to the European (2010/63/EU) and Spanish (RD 53/2013) legislation on Care and Use of Experimental Animals.
Food intake was monitored daily and animal weight was weekly measured. At 20 weeks of age, rats were fasted overnight and sacrificed. Blood samples were collected and allowed to clot for 1 h and serum was separated by centrifugation at 1000g, 10 min, 4 °C for further biochemical analysis. Kidneys were collected and divided into two samples: one was fixed by immersion in paraformaldehyde (PFA) for immunohistochemical analysis and the other sample was frozen in liquid N2 and stored at −80 °C.
2.4. Biochemical analysis
Blood glucose was weekly determined using an Accounted Glucose Analyzer (LifeScan, Madrid, Spain). Serum insulin was analysed with a rat insulin ELISA kit with a detection limit lower than 0.15 ng mL−1. Uric acid was evaluated with a colorimetric kit following the manufacturer's instructions.
Urine samples were collected over 24 h by placing the animals in metabolic cages three days before the end of the study. Animals had free access to food and water. Urine samples were collected in ice-cold bottles and centrifuged at 1000g for 10 min at 4 °C. Then, the supernatants were collected and stored at −80 °C until assayed. Urinary albumin and creatinine were measured by latex turbidimetry and using colorimetric kits, respectively, following the manufacturer's instructions. The estimated creatinine clearance per body weight was calculated according to the following formula: urinary creatinine (mg dL−1) × urinary volume (mL d−1)/serum creatinine (mg dL−1) × (1000/body weight (g)) × (1/1440 (min)).
2.5. Determination of ROS and protein carbonyl groups
ROS were quantified as described based on the oxidation of DCFH assay to dichlorofluorescein (DCF) that emits fluorescence.19 Briefly, renal cortex homogenates were diluted 1
:
20 (v/v) with ice-cold Locke′s buffer [154 mM NaCl, 5.6 mM KCl, 3.6 mM NaHCO3, 2 mM CaCl2, 10 mM D-glucose and 5 mM 4-(2-hydroxyethyl)-1-piperazineethanesulfocnic acid, pH 7.4] and incubated with 5 μM DCFH for 30 min at 37 °C. Fluorescence was measured in a microplate reader (Bio-Tek, Winooski, VT) at an excitation wavelength of 485 nm and an emission wavelength of 530 nm.
Protein oxidation of cortical renal homogenates was measured as carbonyl group content, as previously described in ref. 19. Samples of the frozen renal cortex were homogenized (1
:
5, w/v) in 0.25 M Tris (pH 7.4), 0.2 M sucrose and 5 mM 1,4-dithiothreitol (DTT) buffer. Absorbance was measured at 360 nm and carbonyl content was expressed as nmol mg−1 protein using an extinction coefficient of 22
000 nmol L−1 cm−1. Protein was measured by using the Bradford reagent.
2.6. Analysis of GSH content, and GPx, GR, CAT and SOD activities
GSH content was quantitated by Hissin and Hilf fluorimetric assay, as previously described in ref. 19. The renal cortex was homogenized (1
:
20, w/v) in 50 mM phosphate buffer pH 7.0, and proteins were precipitated with 5% trichloroacetic acid and then centrifuged for 30 min at 10
000g. The method is based on the reaction of GSH with OPT at pH 8.0 and fluorescence was measured at an excitation wavelength of 340 nm and an emission wavelength of 460 nm. The results of samples were referred to those of a standard curve of GSH (5 ng–1 μg).
For analysing the activity of antioxidant enzymes (GPx, GR, CAT, and SOD), samples of the frozen renal cortex were homogenized (1
:
5, w/v) in 0.25 M Tris, 0.2 M sucrose and 5 mM DTT buffer pH 7.4 and centrifuged at 3000g for 15 min. Determination of GPx activity is based on the oxidation of GSH by GPx, using t-BOOH as a substrate, coupled to the disappearance of NADPH by GR.19,23 GR activity was assayed by following the decrease in absorbance due to the oxidation of NADPH utilised in the reduction of oxidized glutathione.19,23 CAT activity was determined by the decomposition of H2O2 as a decrease in absorbance at 240 nm.19,23 SOD activity was assayed by using a commercial kit following the manufacturer's instructions.19,23 The assay is based on the capacity of SOD to reduce superoxide anions, coupled with the disappearance of Dojindo's highly water-soluble tetrazolium salt (WST-1) to yield a dye. SOD activity was quantified by measuring the decrease in the absorbance at 440 nm. Protein concentration was measured by using the Bradford reagent.
2.7. Preparation of total cell lysates and western blot analysis
For protein detection (ERK, p-ERK, JNK, p-JNK, p38, p-p38, NOX-4, AMPK, p-AMPK, mTOR, p62, Beclin-1, LC3, cleaved caspase-3, cleaved caspase-9, Bcl-xL and Bax), samples of the frozen renal cortex were lysed (1
:
5, w/v) at 4 °C in a buffer containing 25 mM HEPES (pH 7.5), 0.3 M NaCl, 1.5 mM MgCl2, 0.2 mM EDTA, 0.5 mM dithiothreitol (DTT), 0.1% Triton X-100, 200 mM β-glycerolphosphate, 0.1 mM Na3VO4, 2 μg mL−1 leupeptin and 1 mM phenylmethanesulfonyl fluoride (PMSF), and later centrifuged at 14
000g for 1 h. Supernatants were collected, assayed for protein concentration by using the Bradford reagent, aliquoted and stored at −80 °C until used for western blot analyses.
Equal amounts of proteins were separated by SDS polyacrylamide gel electrophoresis and transferred to polyvinylidene difluoride filters (Merck, Madrid, Spain). Membranes were probed with the corresponding primary antibody followed by incubation with peroxide-conjugated anti-rabbit (GE Healthcare) or anti-mouse (Sigma) immunoglobulin. Blots were developed with the ECL system (GE Healthcare). Normalization of western blot was ensured by β-actin and band quantification was carried out with a scanner and the Scion Image software.
2.8. Immunohistochemical analyses
Left kidneys were fixed in paraformaldehyde (4%), embedded in paraffin, and cut into 4 μm-thick sections. Serial renal cortical sections were incubated with the primary antibodies (PCNA, p-Nrf2, p-mTOR, or SIRT-1) overnight at 4 °C, and then with HRP-conjugated secondary antibody and revealed with the DAB substrate as the chromogen and haematoxylin. Images of sections were acquired with magnification at 200× and/or 400× using a digital camera (Leica DFC 320 camera, L′Hospitalet del Llobregat, Spain) attached to a light microscope (Leica DM LB2). The proliferating cell nuclear antigen labelling index (PCNA-LI) and the percent of p-Nrf2 immunopositive nuclei were calculated as the percentage of positive nuclei related to total nuclei. SIRT1 and p-mTOR expressions were evaluated by calculating the percentage of their staining area with ImageJ v1.52j software (National Institutes of Health: rsb.info.nih.gov/ij) and the colour deconvolution plug-in. At least six sections per group were analysed. All slides were examined by two different researchers in a blinded manner. All areas were calculated from the mean of 10 cortical fields for each kidney.
2.9. Terminal deoxynucleotidyl transferase dUTP nick end labelling (TUNEL) assay
Apoptotic tubular cells were detected using the terminal transferase dUTP nick end labelling (TUNEL) procedure. Tissue sections after deparaffinization and rehydration were permeabilised with proteinase K (20 μg mL−1) for 15 min at 37 °C, and treated with 3% H2O2 for 5 min to quench endogenous peroxidase activity. After thorough washing with phosphate buffer, sections were incubated with equilibration buffer for 10 min, followed by immediate application of TdT-enzyme working for 1 h at 37 °C in a humidity chamber. The reaction was stopped and the incorporated biotinylated nucleotides were detected by peroxidase conjugated streptavidin and subsequent staining with DAB and counterstaining with methyl green. The apoptotic index represents the proportion of cells undergoing apoptosis within a field divided by the number of total cells multiplied by 100 (400×). For each animal, the total number of apoptotic cells and the total number of cells/field were determined in 50 cortical fields for each kidney of each animal.
2.10. Statistics
Prior to statistical analysis, data were tested for homogeneity of variances by the test of Levene. For multiple comparisons, one-way ANOVA was followed by the Tukey test when variances were homogeneous or by the Tamhane test when variances were not homogeneous. P < 0.05 was considered significant. An SPSS version 25.0 program has been used.
3. Results
3.1. Cocoa-enriched diet improves body weight, and metabolic and renal functionality parameters
The final body weight and total food intake were increased in both groups of ZDF rats in comparison with ZL animals (Table 2). Interestingly, initial body weight was similar in both diabetic animal groups, yet higher than that in ZL rats. However, the final body weight of animals fed with cocoa diet (ZDF-Co) was reduced as compared to ZDF-C rats although total food intake and total energy intakes were similar in both ZDF groups. Moreover, ZDF rats showed higher levels of initial and final glycaemia, as well as final insulinaemia than ZL animals. Values for both parameters (glycaemia and insulinaemia) were lower in ZDF-Co rats than in the ZDF-C group at the end of the study although the initial values of glycaemia were similar in both groups of diabetic animals (Table 2). Likewise, cocoa-enriched diet reduced serum uric acid levels, and maintained lower values for this parameter in comparison with ZDF-C rats yet higher than ZL animals. In addition, both creatinine clearance and the urinary albumin/creatinine ratio (ACR), which showed enhanced levels in ZDF rats when compared to ZL animals, were improved in the ZDF-Co group, displaying intermediate values to those of lean and diabetic rats (Table 2). All these results could suggest that the cocoa-rich diet partly alleviates hyperglycaemia and hyperinsulinemia, and might contribute to amelioration of the renal dysfunction in ZDF rats.
Table 2 Body weight, food intake, and biochemical parameters related to the metabolism and renal function of rats fed with standard (ZL and ZDF) and cocoa-rich (ZDF-Co) dietsa
|
ZL |
ZDF-C |
ZDF-Co |
Data represent the means ± SD. Means in a row without a common letter differ, P < 0.05.
|
Initial body weight (g) |
210.00 ± 9.00a |
284.00 ± 10.00b |
285.00 ± 11.00b |
Final body weight (g) |
333.00 ± 9.00a |
464.00 ± 12.00c |
406.00 ± 9.00b |
Total food intake (g in 10 weeks per cage) |
2446.00 ± 136.00a |
3678.00 ± 165.00b |
3745.00 ± 178.00b |
Total energy intake (kcal in 10 weeks per cage) |
8834.00 ± 489.00a |
13 286.00 ± 597.00b |
13 526.00 ± 642.00b |
Initial glycaemia (mg dL−1) |
84.00 ± 6.00a |
105.00 ± 8.00b |
102.00 ± 13.00b |
Final glycaemia (mg dL−1) |
92.40 ± 4.80a |
272.00 ± 20.74c |
132.82 ± 5.15b |
Final insulinaemia (μU mL−1) |
11.43 ± 0.74a |
125.14 ± 16.14c |
35.75 ± 5.53b |
Serum uric acid (mg dL−1) |
1.14 ± 0.01a |
2.36 ± 0.18c |
1.81 ± 0.14b |
Creatinine clearance/body weight (mL per min per Kg) |
5.50 ± 0.26a |
10.57 ± 1.48c |
6.42 ± 0.56b |
Urinary albumin/creatinine ratio (ACR, mg g−1) |
51.25 ± 12.29a |
361.52 ± 25.45c |
297.19 ± 15.12b |
3.2. Cocoa-rich diet averts enhanced ROS levels, protein oxidation and NOX-4 protein values in the renal cortex of ZDF rats
Chronic hyperglycaemia results in oxidative stress and increased ROS production in diabetes, which could lead to an enhanced macromolecule oxidation.4,7,8 In this line, NOX-4 is reported to be the major source of ROS in the kidneys during the diabetic kidney disease.8 Therefore, to study whether cocoa could prevent these processes, ROS and protein carbonyl content, as well as NOX-4 levels, were measured in the renal cortex of all animals.
ROS values and protein carbonyl content were increased in ZDF-C animals when compared to ZL and ZDF-Co rats, whereas both parameters were similar in ZL and ZDF-Co rats (Fig. 1A and B). In concert, NOX-4 levels were enhanced in ZDF-C rats when compared to ZL animals, but this effect was partially repressed in animals receiving the cocoa rich-diet (Fig. 1C and D). Altogether it suggests that cocoa could contribute to improvement of the redox status as it reduced NOX-4 values, ROS generation and protein oxidation in the renal cortex of ZDF rats.
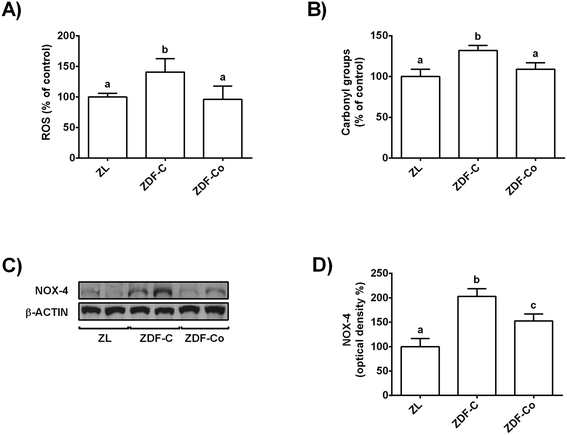 |
| Fig. 1 Effect of the cocoa-enriched diet on (A) ROS production, (B) carbonyl groups and NOX-4 levels in the renal cortex of ZDF rats. (C) NOX-4 bands of representative experiments. (D) Densitometric quantification of total levels NOX-4. All values are expressed as percentage relative to the control condition. Equal loading of Western blots was ensured by β-actin. Means (means ± SD, n = 6–8) without a common letter differ (P < 0.05). | |
3.3. Cocoa-enriched diet modulates antioxidant defences in the renal cortex of ZDF rats
In order to continue studying the redox status of the renal cortex in ZDF diabetic rats, GSH levels, key antioxidant enzymatic activities, and the phosphorylated levels of the transcription factor Nrf2, which is a redox sensor that plays a main role in counteracting the oxidative stress,7 were analysed.
As shown in Fig. 2A, GSH content and GPx and GR activities were not modified in any group of rats. However, SOD and CAT activities diminished in ZDF-C rats when compared to the lean group, although both enzymatic activities were restored to ZL values by feeding the animals with the cocoa-rich diet (Fig. 2B). In concert, ZDF-C rats showed the lowest levels of nuclear p-Nrf2 of the three groups of animals, whereas ZDF-Co exhibited higher nuclear p-Nrf2 values than those of ZL rats (Fig. 2C). Interestingly, p-Nrf2 was localized in the tubules in all groups of animals. All these results might indicate that cocoa-enriched diet modulates relevant antioxidant defences in the renal cortex of ZDF rats, which might contribute to counteracting the oxidative stress in the diabetic animals.
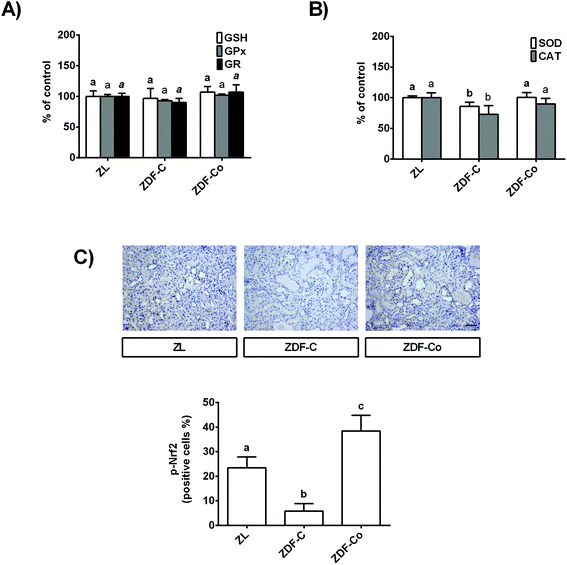 |
| Fig. 2 Effect of the cocoa-rich diet on (A) GSH content and the activity of antioxidant enzymes GPx and GR, and (B) SOD and CAT in the renal cortex of ZDF rats. Values are expressed as a percentage relative to ZL rats (means ± SD, n = 7–9). Different letters over a bar of the same parameter indicate statistically significant differences (P < 0.05). Different styles of letters (bold, plain and italics) have been used for each parameter depicted within the same graph. (C) Representative sections of the renal cortex for immunohistochemical staining of p-Nrf2 (brown-stained). p-Nrf2 is expressed as percentage of positive cells relative to total nuclei in the renal cortex. Scale bar: 20 μm. Data represent means ± SD of 6–8 samples per condition. Means without a common letter differ (P < 0.05). | |
3.4. Cocoa-rich diet regulates MAPK signalling in the renal cortex of ZDF rats
MAPK activation has been related to enhanced oxidative stress in the renal cortex during diabetes and contributes to the promotion of the disease.7,18 Thus, phosphorylated and total levels of ERK, JNK and p38 were evaluated in the three groups of animals by western blot.
Fig. 3A and B illustrate that phosphorylated levels of ERK were similar in ZL and ZDF-Co rats, whereas ZDF-C animals showed enhanced values of p-ERK when compared to other groups. In addition, phosphorylated levels of JNK and p38 were not modified in any group of rats (Fig. 3A, C and D). Likewise there was no difference in the total levels of ERK, JNK and p38 among groups. Therefore, the cocoa-rich diet totally prevented the activation of ERK, which could contribute to attenuation of the redox imbalance in the renal cortex of ZDF-Co animals.
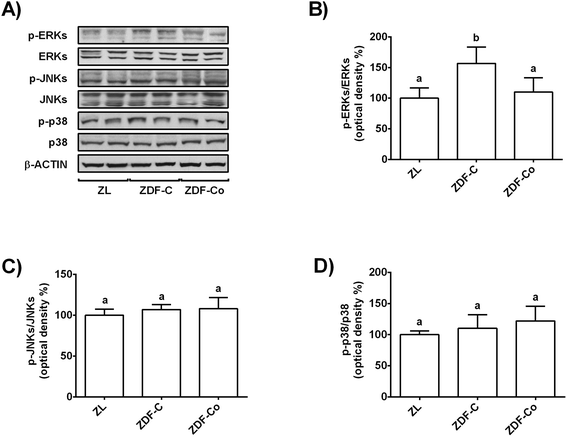 |
| Fig. 3 Effect of the cocoa-rich diet on phosphorylated and total levels of ERK, p-38, JNK in the renal cortex of ZDF rats. (A) Bands of representative experiments. Percentage data of (B) p-ERK/ERK, (C) p-JNK/JNK and (D) p-p38/p38 ratios relative to ZL animals (means ± SD, n = 6–8). Equal loading of western blots was ensured by β-actin. Means without a common letter differ significantly (P < 0.05). | |
3.5. Cocoa-rich diet regulates AMPK, mTOR and SIRT-1 in the renal cortex of ZDF rats
AMPK, SIRT-1 and mTOR have important cytoprotective roles in renal cells against different types of stress, and also during diabetes.3,24 Then, the modulation of these proteins by the cocoa-rich diet was studied, and total and phosphorylated levels of AMPK and mTOR, as well as total levels of SIRT-1, were assayed by western blot or immunohistochemistry.
Phosphorylated levels of AMPK were diminished in ZDF-C animals in comparison with ZL and ZDF-Co groups (Fig. 4A and B). Accordingly, levels of total and phosphorylated mTOR increased in ZDF-C rats when compared to ZL and ZDF-Co animals (Fig. 4A, C and D). Cocoa-rich diet totally restored to ZL values the altered levels of p-AMPK and mTOR; however, the ZDF-Co group showed a partial recovery of p-mTOR values, as these animals displayed levels intermediate to those of lean and diabetic rats. Indeed, the highest levels of p-mTOR were found in the tubulointerstitium area in the ZDF-C group, whereas this protein was clearly diminished in the renal cortex sections of ZL and ZDF-Co rats (Fig. 4D). There was no difference in the total levels of AMPK among groups. Additionally, SIRT-1, which was localized in the mesangial area and in the tubular membrane, showed decreased levels in ZDF-C rats when compared to the ZL group, but this effect was totally repressed in animals receiving the cocoa rich-diet (Fig. 4E). All these results suggest that cocoa might contribute to prevention of the injury in the renal cortex of ZDF rats against the stress insult by modulating key proteins closely related to cellular protection.
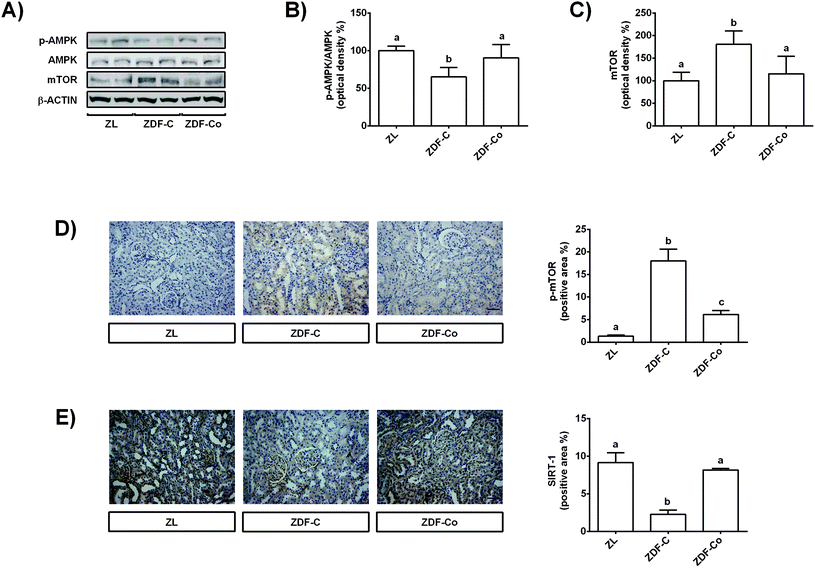 |
| Fig. 4 Effect of the cocoa-rich diet on phosphorylated and total values of AMPK and mTOR, and in total levels of SIRT-1 in the renal cortex of ZDF rats. (A) Bands of representative experiments. Percentage data of densitometric quantification for (B) p-AMPK/AMPK ratio, and (C) mTOR. Values are expressed as a percentage relative to the control condition (means ± SD, n = 6–8). Representative sections of the renal cortex for immunohistochemical staining of (D) p-mTOR and (E) SIRT-1. p-mTOR and SIRT-1 (both brown-stained) are expressed as the percentage of positive area in the renal cortex. Scale bar: 20 μm. Data represent means ± SD of 6–8 samples per condition. Mean values with unlike letters were significantly different (P < 0.05). | |
3.6. Cocoa-enriched diet activates autophagy in the renal cortex of ZDF rats
AMPK, mTOR and SIRT-1 also play a crucial role in the autophagy process, which is considered a general cellular response to stress that can directly induce cell death or act as a cytoprotective mechanism.9,10 Indeed, induction of autophagy has been suggested as a therapeutic target in diabetes kidney disease.9 Then, key autophagy proteins, such as p62, Beclin-1 and LC3, were studied by western blot.
As shown in Fig. 5A and B, p62 levels increased in the renal cortex of ZDF-C rats when compared to ZL animals, but this effect was completely reverted in animals receiving the cocoa rich-diet. In contrast, values of Beclin-1 and LC3-II/LC3-I ratio decreased in ZDF-C animals in comparison with the lean group, whereas rats fed with the cocoa-rich diet showed higher levels than those of the ZL group (Fig. 5A, C and D). All these results suggest that the cocoa-rich diet might also contribute to protection of the kidney through the induction of autophagy in ZDF rats.
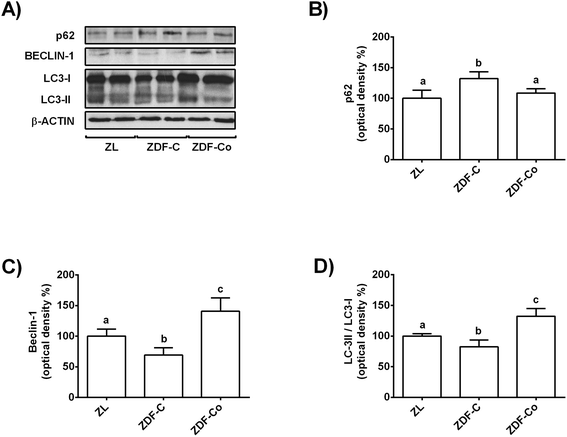 |
| Fig. 5 Effect of the cocoa-rich diet on levels of p62, Beclin-1, and LC3-II/LC3-I ratio in the renal cortex of ZDF rats. (A) Bands of representative experiments. Percentage data of (B) p62, (C) Beclin-1 and (D) LC3-II/LC3-I ratio relative to ZL rats (means ± SD, n = 6–8). Equal loading of western blots was ensured by β-actin. Means without a common letter differ significantly (P < 0.05). | |
3.7 Cocoa-rich diet modulates proliferation and apoptosis in the renal cortex of ZDF rats
During diabetes the situation of stress in renal tubular cells may lead to alterations in growth and apoptosis.2,10,25 In fact oxidative stress and autophagy can contribute to the regulation of apoptosis during diabetes in the kidney.10 Therefore, to get further insight into the mechanisms for the protective effect of cocoa intake in the renal cortex of ZDF rats, cell proliferation and apoptosis were analysed by PCNA and TUNEL assays, respectively, and key proteins related to the apoptotic process were evaluated by western blot.
Cortical renal sections showed an increased level of PCNA positive cells, i.e. higher percentage of tubular proliferating cells in ZDF-C rats when compared to the ZL group, showing ZDF-Co animals’ intermediate values to those of lean and diabetic rats (Fig. 6A). Similarly, the representative photographs for TUNEL-positive cells showed a pro-apoptotic effect in the renal cortical tubular cells of ZDF-C rats, whereas cocoa intake totally prevented all these changes (Fig. 6B). Besides, PCNA-and TUNEL-positive cells were scarcely observed in the glomeruli, the majority of proliferating and apoptotic cells being localized in the tubules (Fig. 6A and B).
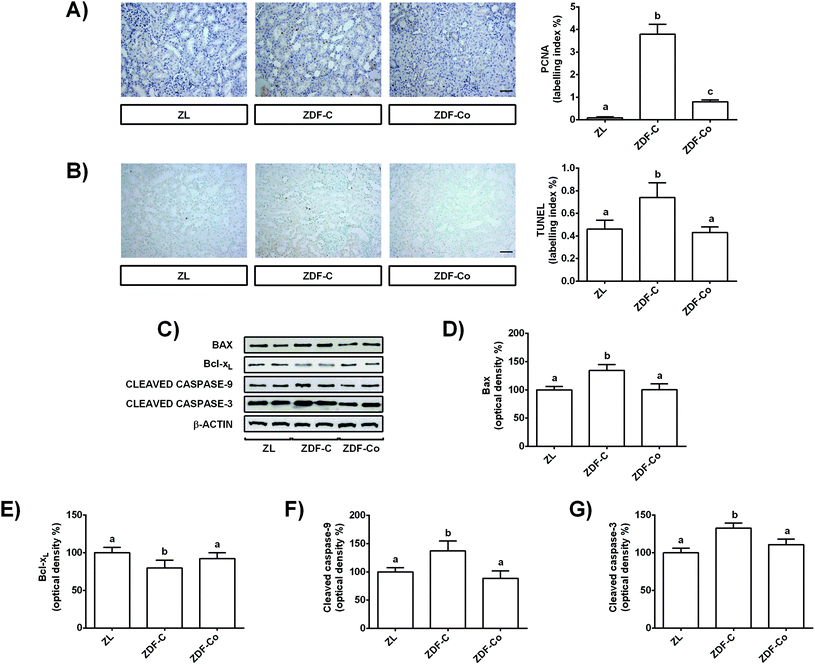 |
| Fig. 6 Effect of the cocoa-rich diet on PCNA, apoptotic index (TUNEL) and total levels of Bax, Bcl-xL, cleaved caspase-9 and cleaved caspase-3 in the renal cortex of ZDF rats. (A) Representative photographs for immunohistochemical staining of PCNA-positive cells (scale bar = 20 μm) and proliferative index expressed as the percentage of labelling index in the renal cortex. (B) Representative sections for immunohistochemical staining of TUNEL positive cells (scale bar = 20 μm) and apoptotic index expressed as the percentage of labelling index in the renal cortex. (C) Representative bands of western blot analyses. Percentage levels of (D) Bax, (E) Bcl-xL, and cleaved (F) caspase-9 and (G) caspase-3 relative to ZL animals (means ± SD, n = 6–8). Equal loading of western blots was ensured by β-actin. Means without a common letter differ significantly (P < 0.05). | |
To better characterize the molecular mechanisms involved in these processes, the expressions of anti-apoptotic (Bcl-xL) and pro-apoptotic (Bax) members of the Bcl-2 protein family, as well as levels of cleaved caspases-9 and -3, were examined. As shown in Fig. 6C–E, ZDF-C rats presented decreased Bcl-xL expression values along with a concomitant increase in Bax levels. However, feeding animals with the cocoa-enriched diet raised Bcl-xL levels and reduced Bax values, displaying both proteins with comparable values to those of the ZL group (Fig. 6C–E). Similarly, cleaved caspase-9 and -3 levels were enhanced in the ZDF-C group, and these values were restored to control levels (ZL) when animals received the cocoa-rich diet (Fig. 6C, F and G). These findings indicate that the cocoa-supplemented diet might also contribute to the renal protective effect by improving the cellular homeostasis in the renal cortex of ZDF rats, as it prevented the enhanced proliferation and apoptosis observed in the diabetic animals (ZDF-C).
4. Discussion
Oxidative stress is a major determinant for the development and progression of the diabetic kidney disease.4 Thus, new candidates aimed to decrease or inhibit sources of ROS, to enhance antioxidant defences and to modulate processes related to the stress, such as apoptosis or autophagy, constitute a promising approach for the development of new strategies against the diabetic kidney disease. In this regard, cocoa has demonstrated antioxidant and antidiabetic activities.11,12,26 However, insufficient investigation in vivo, especially at the molecular level, has been performed to support these observations during the diabetic kidney disease.
The present work provides the first in vivo evidence that a cocoa-rich diet improves the functionality of kidneys by exerting renoprotective effects as it contributes to the maintenance of the redox balance and cellular homeostasis through the activation of autophagy and inhibition of apoptosis in the renal cortex of ZDF rats. The cocoa-rich diet suppresses the increased ROS generation, protein carbonyl content and NOX-4 levels in ZDF animals. Furthermore, cocoa positively modulates renal antioxidant defences (p-Nrf2 levels, and SOD and CAT activities), as well as levels of proteins closely related to oxidative stress (ERK-MAPKs) and to cytoprotection (AMPK, mTOR and SIRT-1). Likewise, cocoa contributes to alleviation of the renal injury through the regulation of the autophagy and apoptosis processes in the renal cortex of ZDF rats.
Diabetes is characterized by chronic hyperglycaemia along with hyperinsulinaemia at the early stage of the disease.12,19,21 However, both parameters were partly alleviated to control values (ZL group) in those animals fed with the cocoa-rich diet. In this line, it has been reported that cocoa improves the glucose metabolism in different diabetic rodent models, as it amended the glucose homeostasis and insulin sensitivity.17,19,26,27 Indeed, although in the present work the glucose metabolism in the liver at the beginning of the study has not been examined, which could be considered as a limitation of the work, the effect of cocoa on this organ could not be ruled out, as well as its contribution to the glucose metabolism.14 In addition, in ZDF rats (ZDF-C and ZDF-Co) food intake increased in comparison with non-diabetic ZL animals, demonstrating the hyperphagic and obese state of ZDF animals.14,17,21 Nevertheless, the body weight was lower in ZDF-Co rats when compared to ZDF-C animals, although total food intake was similar in both groups; this feature has been associated with the ability of cocoa polyphenols to reduce fat adipose tissue.14,16,27
Clinically, the early stage of the diabetic kidney disease is characterized by renal hyperfunction and hypertrophy.2 Indeed, the hyperfunctionality is accompanied by hyperfiltration, which explains the high levels of uric acid and creatinine, as well as the increased values of ACR as a consequence of the albuminuria in ZDF-C rats (Table 2). All these changes were highly reverted by feeding ZDF rats with cocoa. Similarly, foods rich in flavanols (cocoa-enriched in flavanols and green tea) or pure flavanols alone, such as epigallocatechin-3-gallate (EGCG) and procyanidin B2, have demonstrated protective effects against diabetes-induced functional alterations of kidneys in different diabetic rodent models.18,28,29
Hyperfiltration caused by the diabetic milieu activates a number of pathological pathways, such as oxidative stress-related routes, among others.2,7,10 In this line, levels of ROS and the marker of protein oxidation (carbonyl groups) increased in ZDF-C rats, whereas these effects were prevented by the cocoa-rich diet. Accordingly, in ZDF-C rats increased levels of NOX-4, which is considered the main source of ROS during this disease, were highly recovered to ZL values by feeding the animals with cocoa. The exacerbated oxidative stress observed in ZDF-C rats led to a depleted antioxidant defence system (reduced SOD and CAT activities, and decreased nuclear p-Nrf2 levels), although the antioxidant response was restored in animals fed with cocoa. In agreement with our results, diminished activities of SOD and CAT and unaltered levels of GSH and GPx and GR activities in the kidney of diabetic rats have been reported.30–32 In addition, a cocoa-diet enriched in flavanols, a procyanidin extract, epicatechin, EGCG, and procyanidin B2 have shown to suppress the oxidative stress by attenuating ROS generation, protein carbonyl content, and NOX-4 levels, and by increasing the activity of CAT and SOD antioxidant enzymes in different diabetic models.28,31–36 In this regard, epicatechin restored the redox status in cultured renal tubular cells subjected to a high-glucose-challenge, playing NOX-4 a relevant role in this protective effect;23 indeed, inhibition of NOX-4 occurred along with increased SOD activity, reduced oxidative stress markers and improved renal functionality,23,37 in concordance with the present results. Moreover, active Nrf2 modulates antioxidant enzymes and has demonstrated a protective effect against the diabetic kidney disease.7,38 In agreement with this work, the natural compounds betulinic acid and sulfurophane, as well as the flavonoid resveratrol, prevented the diabetic kidney disease by upregulating Nrf2 and downstream genes (SOD and CAT, among others).32,38,39 However, the regulation of the redox status is complex and different pathways are involved in ROS generation (NOX, dysfunctional mitochondrial chain, xanthine oxidase, nitrosative stress, etc.), which leads to the activation of different routes, such as nuclear factor-κB, MAPKs, etc.40 Therefore, the involvement of different signals related to oxidative stress on the cocoa-induced protective effect cannot be ruled out.
Excessive oxidative stress results in the activation of different pathways, including MAPKs.7 In concert, high levels of phosphorylated-ERK-MAPK have been demonstrated during the diabetic kidney disease in rats.18,36,41 Similarly to the current study, feeding diabetic animals with anthocyanins, procyanidin B2 or EGCG abolished the increased p-ERK values, this inhibition being associated with a reduced oxidative stress and with a renoprotective effect.18,36,41
AMPK, SIRT-1 and m-TOR are proteins regulated by oxidative and metabolic stress.3,24 During diabetes kidney disease decreased levels of AMPK and SIRT-1 together with enhanced values of m-TOR have been reported.31,32,34,42 Correspondingly, in diabetes (also in ZDF-C animals) reduced AMPK levels have been associated with the activation of NOX-4, as well as with diminished SIRT-1 values concurrently with decreased CAT and SOD activities, and worsen albuminuria and renal function.23,34,43 However, the cocoa-rich diet (ZDF-Co rats) highly or completely recovered all mentioned parameters to control values (ZL group). Consistent with these findings, a cocoa diet enriched in flavanols, as well as epicatechin and procyanidins, prevented renal SIRT-1 and AMPK diminution, which was associated with a renoprotective effect and improved cellular redox status in in vivo and in vitro diabetic models.23,31–34,42 Moreover, it has been shown that AMPK and SIRT-1 stimulation protected against ROS-mediated damage by suppressing NOX-4 activation in renal cells.23,34 Indeed, SIRT-1 and AMPK activations have also been related to increased levels of Nrf2 and its downstream antioxidant targets in diabetic complications.44 Consequently, the activation of the AMPK-SIRT-1 signalling has been connected to an improved redox status and organ functionality in the kidney of diabetic rodents.32,44
Impaired autophagy is associated with decreased AMPK, which leads to the activation of the mTOR pathway, and diminished levels of SIRT-1,9 as shown in the present work for ZDF-C diabetic rats. Importantly, autophagy plays a crucial role in the pathogenesis of the diabetic kidney disease, as it is an essential mechanism to maintain the homeostasis of tubules and glomeruli in the renal cortex.9 Thus, reduced autophagy and enhanced levels of p62 have been reported in renal cortical cells of diabetic animals and patients.25,45 Accordingly, accumulation of p62, and decreased levels of Beclin-1 and LC3-II/LC3-I ratio were observed in ZDF-C rats. However, the inhibition of autophagy was prevented by feeding the animals with cocoa. These results were associated with recovered levels of AMPK and SIRT-1, and diminished values of mTOR when compared to the ZDF-C group. Correspondingly, the flavonoid resveratrol has been reported to activate renal AMPK and SIRT-1 and to reverse the mTOR stimulation, which in turn activated the autophagy and contributed to amelioration of the renal injury in diabetic animals.46,47
Oxidative stress and autophagy dysregulation are also related to apoptosis, and both processes can contribute to favour this type of programmed cell death in the kidney during diabetes.3,10 Accordingly, ZDF-C rats showed a deteriorated antioxidant system, a reduced autophagy flux, and increased levels of active caspases-3 and -9, as well as enhanced values of Bax concurrently with diminished expression of Bcl-xL, and consequently, augmented the apoptotic index (TUNEL), together with an increased proliferative index (PCNA), as previously shown in renal tubules of diabetic animals.28,48 Indeed, the enhanced proliferation in the renal cortex has been associated with the early tubular hypertrophy and hyperplasia, as a consequence of the renal hyperfunctionality.2 However, the altered cell turnover (proliferative-apoptotic levels) was highly or completely prevented by feeding the animals with cocoa, which was also associated with an improved redox status and with a renoprotective effect. Similarly, the antidiabetic drug pioglitazone and the triterpenoid betulinic acid showed antiapoptotic and antiproliferative effects in the kidney of diabetic rats, which alleviated the injury in the organ.32,48 Quercetin-rich juice, procyanidins, EGCG and anthocyanins also prevented the oxidative stress-induced damage and restored the redox status in diabetic animals and renal cellular models through the inhibition of apoptosis (diminished levels of cleaved caspase-3).28,41,42,49 In this line, catalase overexpression in proximal tubules of transgenic diabetic mice has been shown to attenuate tubular apoptosis.50 Likewise, it could be highlighted that the restored levels of SIRT-1 and AMPK showed after treatments with procyanidins in in vivo and in vitro renal diabetic models have not only been related to an inhibition of oxidative stress, as mentioned, but also to a suppression of apoptosis, which led to an ameliorated damage in renal cells.31,42 Finally, administration of food rich in flavonoids or natural compounds, such as quercetin-rich juice or resveratrol, also contributed to restore the balanced activity of autophagy and apoptosis in the kidney of diabetic animals and to improve the functionality of the organ;46,47,49 therefore, it has been suggested that autophagy induction to an appropriate level could be beneficial for the treatment of this renal disease.51
Interestingly, the health beneficial effects of cocoa have been mainly related to its flavanols, although a role for the fibre should be considered. In this line, it has been recently reported that most of the beneficial effects attributed to cocoa are not only due to its fibre content, and polyphenols and other compounds could also be key factors.52 Thus, epicatechin and procyanidins seem to contribute in the maintenance of renal function and structure during diabetes.17,33,35,42 In addition, theobromine alone can protect against uric acid crystallization,53 and in combination with cocoa flavanols enhance their protective vascular effects,54 which also seem to participate in the preservation of kidneys. It is also worth mentioning that, using the body surface area normalisation method,55 the amount of cocoa employed in the present animal study is equivalent to a daily dose of 64.5 g cocoa, containing 1075 mg polyphenols, for a 60 kg human. Although this total phenolic intake could be considered realistic, the amount of cocoa required is certainly above a recommended individual ingestion. Accordingly, a human intervention trial has been conducted using 20 g d−1 of polyphenol-rich dark chocolate containing 1000 mg polyphenols.56 This dose of 20 g d−1 of enriched dark chocolate represents 5–10% of dietary energy, an amount that most people could readily incorporate into their diet. However, more research needs to be done using lower doses of cocoa with greater physiological relevance to humans.
5. Conclusions
In summary, the present work shows for the first time that in the renal cortex cocoa exerts protective effects by contributing to the balance of the redox status in ZDF diabetic rats, as it prevented ROS generation, protein oxidation, and activation of stress related key proteins (ERK-MAPKs and NOX-4), as well as the diminution of antioxidant defences (CAT, SOD, Nrf2), and modulates cytoprotective-related proteins, namely AMPK, SIRT-1 and mTOR. In addition, cocoa-rich diet modulates the autophagy (p62, Beclin-1 and LC3-II/LC3-I ratio) and apoptosis (caspase-3, -9, Bax, Bcl-xL, TUNEL and PCNA) pathways, contributing to the balance between both processes and to amelioration of the renal injury in the renal cortex of ZDF rats. This study provides in vivo evidence about the beneficial effects derived from the cocoa intake on the protection of the cortical renal function during diabetes, providing the underlying mechanisms related to crucial processes involved in the development of diabetic nephropathy (redox status, autophagy and apoptosis), as well as potential therapeutic targets for the prevention and therapy of this disease.
Conflicts of interest
The authors declare that there are no conflicts of interest.
Acknowledgements
This work was supported by the grant AGL2015-67087-R (MINECO/FEDER, UE) from the Spanish Ministry of Science and Innovation (MINECO). D. Álvarez-Cilleros is a FPI fellow from the predoctoral program of MINECO (BES-2016-076721). We acknowledge support of the publication fee by the CSIC Open Access Publication Support Initiative through its Unit of Information Resources for Research (URICI).
References
- P. H. Winocour, Diabetes and chronic kidney disease: an increasingly common multi-morbid disease in need of a paradigm shift in care, Diabetic Med., 2018, 35, 300–305 CrossRef CAS.
- J. M. Forbes and M. E. Cooper, Mechanisms of diabetic complications, Physiol. Rev., 2013, 93, 137–188 CrossRef CAS PubMed.
- V. Vallon, The proximal tubule in the pathophysiology of the diabetic kidney, Am. J. Physiol.: Regul., Integr. Comp. Physiol., 2011, 300, R1009–R1022 CrossRef CAS PubMed.
- R. C. Stanton, Oxidative stress and diabetic kidney disease, Curr. Diabetes Rep., 2011, 11, 330–336 CrossRef CAS PubMed.
- F. N. Ahmed, F. N. Naqvi and F. Shafiq, Lipid peroxidation and serum antioxidant enzymes in patients with type 2 diabetes mellitus, Ann. N. Y. Acad. Sci., 2006, 1084, 481–489 CrossRef CAS PubMed.
- V. Ramakrishna and R. JailKhani, Oxidative stress in non-insulin-dependent diabetes mellitus (NIDDM) patients, Acta Diabetol., 2010, 45, 41–46 CrossRef PubMed.
- L. Rochette, M. Zeller, Y. Cottin and C. Vergely, Diabetes, oxidative stress and therapeutic strategies, Biochim. Biophys. Acta, 2014, 1840, 2709–2729 CrossRef CAS PubMed.
- M. Sedeek, A. C. Montezano, R. L. Hebert, S. P. Gray, E. Di Marco, J. C. Jha, M. E. Cooper, K. Jandeleit-Dahm, E. L. Schiffrin, J. L. Wilkinson-Berka and R. M. Touyz, Oxidative stress, Nox isoforms and complications of diabetes-potential targets for novel therapies, J. Cardiovasc. Trans. Res., 2012, 5, 590–518 Search PubMed.
- Y. Ding and M. E. Choi, Autophagy in diabetic nephropathy, J. Endocrinol., 2015, 224, R15–R30 CAS.
- K. Turkmen, Inflammation, oxidative stress, apoptosis, and autophagy in diabetes mellitus and diabetic kidney disease: the Four Horsemen of the Apocalypse, Int. Urol. Nephrol., 2017, 49, 837–844 CrossRef CAS PubMed.
- J. Giacometti, D. Muhvic, A. Pavletic and D. Đudaric, Cocoa polyphenols exhibit antioxidant, antiinflammatory, anticancerogenic, and antinecrotic activity in carbon tetrachloride intoxicated mice, J. Funct. Foods, 2016, 23, 177–187 CrossRef CAS.
- M. A. Martín, L. Goya and S. Ramos, Antidiabetic actions of cocoa flavanols, Mol. Nutr. Food Res., 2016, 60, 1756–1769 CrossRef PubMed.
- J. A. Vinson, J. Proch, P. Bose, S. Muchler, P. Taffera, D. Shutta, N. Samman and G. A. C. Agbor, Chocolate is a powerful ex vivo and in vitro antioxidant, antiatherosclerotic agent in an animal model, and a significant contributor to antioxidants in the European and American diets, J. Agric. Food Chem., 2006, 54, 8071–8076 CrossRef CAS PubMed.
- I. Cordero-Herrera, M. A. Martín, F. Escrivá, C. Álvarez, L. Goya and S. Ramos, Cocoa-rich diet ameliorates hepatic insulin resistance by modulating insulin signaling and glucose homeostasis in Zucker diabetic fatty rats, J. Nutr. Biochem., 2015, 26, 704–712 CrossRef CAS PubMed.
- D. Grassi, G. Desideri, S. Necozione, C. Lippi, R. Casale, G. Properzi, J. B. Blumberg and C. Ferri, Blood pressure is reduced and insulin sensitivity increased in glucose-intolerant, hypertensive subjects after 15 days of consuming high-polyphenol dark chocolate, J. Nutr., 2008, 138, 1671–1676 CrossRef CAS PubMed.
- M. Fidaleo, A. Fracassi, A. Zuorro, R. Lavecchia, S. Moreno and C. Sartori, Cocoa protective effects against abnormal fat storage and oxidative stress induced by a high-fat diet involve PPARα signalling activation, Food Funct., 2014, 5, 2931–2939 RSC.
- D. Alvarez-Cilleros, E. López-Oliva, L. Goya, M. A. Martin and S. Ramos, Cocoa intake attenuates renal injury in Zucker Diabetic fatty rats by improving glucose homeostasis, Food Chem. Toxicol., 2019, 127, 101–109 CrossRef CAS PubMed.
- Z. Zhang, B.-Y. Li, X.-L. Li, M. Cheng, F. Yu, W.-D. Lu, Q. Cai, J.-F. Wang, R.-H. Zhou, H.-Q. Gao and L. Shen, Proteomic analysis of kidney and protective effects of grape seed procyanidin B2 in db/db mice indicate MFG-E8 as a key molecule in the development of diabetic nephropathy, Biochim. Biophys. Acta, 2013, 1832, 805–813 CrossRef CAS PubMed.
- I. Cordero-Herrera, M. A. Martín, L. Goya and S. Ramos, Cocoa intake ameliorates hepatic oxidative stress in young Zucker diabetic fatty rats, Food Res. Int., 2015, 69, 194–201 CrossRef CAS.
- E. Ramiro-Puig, M. Urpi-Sarda, F. J. Pérez-Cano, A. Franch, C. Castellote, C. Andres-Lacueva, M. Izquierdo-Pulido and M. Castell, Cocoa-enriched diet enhances antioxidant enzyme activity and modulates lymphocyte composition in thymus from young rats, J. Agric. Food Chem., 2007, 55, 6431–6438 CrossRef CAS PubMed.
- B. L. Leonard, R. N. Watson, K. M. Loomes, A. R. J. Phillips and G. J. Cooper, Insulin resistance in the Zucker diabetic fatty rat: a metabolic characterisation of obese and lean phenotypes, Acta Diabetol., 2005, 42, 162–170 CrossRef CAS PubMed.
- J. Hempe, R. Elvert, H.-L. Schmidts, W. Kramer and A. W. Herling, Appropriateness of the Zucker Diabetic Fatty rat as a model for diabetic microvascular late complications, Lab. Anim., 2012, 46, 32–39 CrossRef CAS PubMed.
- D. Alvarez-Cilleros, M. A. Martín, L. Goya and S. Ramos, (−)-Epicatechin and the colonic metabolite 3,4-dihydroxyphenylacetic acid protect renal proximal tubular cell against high glucose-induced oxidative stress by modulating NOX-4/SIRT-1 signalling, J. Funct. Foods, 2018, 46, 19–28 CrossRef CAS.
- C.-M. Hao and V. H. Haase, Sirtuins and their relevance to the kidney, J. Am. Soc. Nephrol., 2010, 21, 1620–1627 CrossRef CAS PubMed.
- M. Kitada, Y. Ogura, I. Monno and D. Koya, Regulating autophagy as therapeutic target for diabetic nephropathy, Curr. Diab. Res., 2017, 17 DOI:10.1007/s11892-017-0879-y.
- A.-M.-M. Jalil, A. Ismail, C.-P. Pei, M. Hamid and S.-H.-S. Kamaruddin, Effects of cocoa extract on glucometabolism, oxidative stress, and antioxidant enzymes in obese-diabetic (ob-db) rats, J. Agric. Food Chem., 2008, 56, 7877–7884 CrossRef CAS PubMed.
- A. Ruzaidi, I. Amin, A. G. Nawalyah, M. Hamid and H. A. Faizul, The effect of Malaysian cocoa extract on glucose levels and lipid profiles in diabetic rats, J. Ethnopharmacol., 2005, 98, 55–60 CrossRef CAS PubMed.
- T. Mohan, P. Velusamy, L. N. Chakrapani, A. K. Srinivasan, A. Singh, T. Johnson and K. Periandavan, Impact of EGCG supplementation on the progression of diabetic nephropathy in rats: an insight into fibrosis and apoptosis, J. Agric. Food Chem., 2017, 65, 8028–8036 CrossRef CAS PubMed.
- W. M. Renno, S. Abdeen, M. Alkhalaf and S. Asfar, Effect of green tea on kidney tubules of diabetic rats, Br. J. Nutr., 2008, 100, 652–659 CrossRef CAS PubMed.
- S. Mohamad, A. Taha, R. N. K. Bamezai, S. F. Basir and N. Z. Baquer, Lower doses of vanadate in combination with trigonella restore altered carbohydrate metabolism and antioxidant status in alloxan-diabetic rats, Clin. Chim. Acta, 2004, 342, 105–114 CrossRef CAS PubMed.
- L. Bao, X. Cai, X. Dai, Y. Ding, Y. Jiang, Y. Li, Z. Zhang and Y. Li, Grape seed proanthocyanidin extracts ameliorate podocyte injury by activating peroxisome proliferator-activated receptor-g coactivator 1a in low-dose streptozotocin-and high-carbohydrate/high-fat diet-induced diabetic rats, Food Funct., 2014, 5, 1872–1880 RSC.
- R. Xie, H. Zhang, X.-Z. Wang, X.-Z. Yang, S.-N. Wu, H.-G. Wang, P. Shen and T.-H. Ma, The protective effect of betulinic acid (BA) diabetic nephropathy on streptozotocin (STZ)-induced diabetic rats, Food Funct., 2017, 8, 299–306 RSC.
- L. Bao, X. Cai, Z. Zhang and Y. Li, Grape seed procyanidin B2 ameliorates mitochondrial dysfunction and inhibits apoptosis via the AMP-activated protein kinase–silent mating type information regulation 2 homologue 1−PPARg co-activator-1a axis
in rat mesangial cells under high-dose glucosamine, Br. J. Nutr., 2015, 113, 35–44 CrossRef CAS PubMed.
- A. Papadimitriou, E. B. M. I. Peixoto, K. C. Silva, J. M. Lopes de Faria and J. B. Lopes de Faria, Increase in AMPK brought about by cocoa is renoprotective in experimental diabetes mellitus by reducing NOX4/TGFβ-1 signaling, J. Nutr. Biochem., 2014, 25, 773–784 CrossRef CAS PubMed.
- P. D. Prince, C. Rodríguez-Lanzi, C. G. Fraga and M. Galleano, Dietary (−)-epicatechin affects NF-κB activation and NADPH oxidases in the kidney cortex of high-fructose-fed rats, Food Funct., 2019, 10, 26–32 RSC.
- X. H. Yang, Y. Pan, X. L. Zhan, B. L. Zhang, L. L. Guo and H. M. Jin, Epigallocatechin-3-gallate attenuates renal damage by suppressing oxidative stress in diabetic db/db mice, Oxid. Med. Cell. Longevity, 2016, 2016, 2968462 Search PubMed.
- B. Y. Jeong, H. Y. Lee, C. G. Park, J. Kang, S. L. Yu, D. R. Choi, S. Y. Han, M. H. Park, S. Cho, S. Y. Lee, W. M. Hwang, S. R. Yun, H. M. Ryu, E. J. Oh, S. H. Park, Y. L. Kim and S. H. Yoon, Oxidative stress caused by activation of NADPH oxidase 4 promotes contrast-induced acute kidney injury, PLoS One, 2018, 13, e0191034 CrossRef PubMed.
- W. Cui, Y. Bai, X. Miao, P. Luo, Q. Chen, Y. Tan, M. J. Rane, L. Miao and L. Cai, Prevention of diabetic nephropathy by sulforaphane: possible role of Nrf2 upregulation and activation, Oxid. Med. Cell. Longevity, 2012, 821936, DOI:10.1155/2012/821936.
- P. Palsamy and S. Subramanian, Resveratrol protects diabetic kidney by attenuating hyperglycemia-mediated oxidative stress and renal inflammatory cytokines via Nrf2−Keap1 signaling, Biochim. Biophys. Acta, 2011, 1812, 719–731 CrossRef CAS PubMed.
- A. Duni, V. Liakopoulos, S. Roumeliotis, D. Peschos and E. Dounousi, Oxidative stress in the pathogenesis and evolution of chronic kidney disease: untangling Ariadne's thread, Int. J. Mol. Sci., 2019, 20, 3711, DOI:10.3390/ijms20153711.
- J. Wei, H. Wu, H. Zhang, F. Li, S. Chen, B. Hou, Y. Shi, L. Zhao and H. Duan, Anthocyanins inhibit high glucose-induced renal tubular cell apoptosis caused by oxidative stress in db/db mice, Int. J. Mol. Med., 2018, 41, 1608–1618 CAS.
- X. Cai, L. Bao, J. Ren, Y. Li and Z. Zhang, Grape seed procyanidin B2 protects podocytes from high glucose-induced mitochondrial dysfunction and apoptosis via the AMPK-SIRT1-PGC-1α axis in vitro, Food Funct., 2016, 7, 805–815 RSC.
- S. Hou, F. Zheng, Y. Li, L. Gao and J. Zhang, The protective effect of glycyrrhizic acid on renal tubular epithelial cell injury induced by high glucose, Int. J. Mol. Sci., 2014, 15, 15026–15043 CrossRef CAS PubMed.
- S. L. Habib, A. Yadav, D. Kidane, R. H. Weiss and S. Liang, Novel protective mechanism of reducing renal cell damage in diabetes: Activation AMPK by AICAR increased NRF2/OGG1 proteins and reduced oxidative DNA damage, Cell Cycle, 2016, 15, 3048–3059 CrossRef CAS PubMed.
- K. Yamahara, S. Kume, D. Koya, Y. Tanaka, Y. Morita, M. Chin-Kanasaki, H. Araki, K. Isshiki, S.-I. Araki, M. Haneda, T. Matsusaka, A. Kashiwagi, H. Maegawa and T. Uzu, Obesity-mediated autophagy insufficiency exacerbates proteinuria-induced tubulointerstitial lesions, J. Am. Soc. Nephrol., 2013, 24, 1769–1781 CrossRef CAS PubMed.
- L. Ma, R. Fu, Z. Duan, J. Lu, J. Gao, L. Tian, Z. Lv, Z. Chen, J. Han, L. Jia and L. Wang, Sirt1 is essential for resveratrol enhancement of hypoxia-induced autophagy in the type 2 diabetic nephropathy rat, Pathol., Res. Pract., 2016, 212, 310–318 CrossRef CAS PubMed.
- X.-H. Xu, D.-F. Ding, H.-J. Yong, C.-L. Dong, N. You, X.-L. Ye, M.-L. Pan, J.-H. Ma, Q. You and Y.-B. Lu, Resveratrol transcriptionally regulates miRNA-18a-5p expression ameliorating diabetic nephropathy via increasing autophagy, Eur. Rev. Med. Pharmacol. Sci., 2017, 21, 4952–4965 Search PubMed.
- J. E. Toblli, G. Cao, J. F. Giani, M. Angerosa, F. P. Dominici and N. F. Gonzalez-Cadavid, Antifibrotic effects of pioglitazone at low doses on the diabetic rat kidney are associated with the improvement of markers of cell turnover, tubular and endothelial integrity, and angiogenesis, Kidney Blood Pressure Res., 2011, 34, 20–33 CrossRef CAS PubMed.
- C. F. Lin, Y. T. Kuo, T. Y. Chen and C. T. Chien, Quercetin-rich guava (Psidium guajava) juice in combination with trehalose reduces autophagy, apoptosis and pyroptosis formation in the kidney and pancreas of type II diabetic rats, Molecules, 2016, 21, 334, DOI:10.3390/molecules21030334.
- M. L. Brezniceanu, F. Liu, C. C. Wei, I. Chénier, N. Godin, S. L. Zhang, J. G. Filep, J. R. Ingelfinger and J. S. Chan, Attenuation of interstitial fibrosis and tubular apoptosis in db/db transgenic mice overexpressing catalase in renal proximal tubular cells, Diabetes, 2008, 57, 451–459 CrossRef CAS PubMed.
- W.-H. Wu, M.-P. Zhang, F. Zhang, F. Liu, Z.-X. Hu, Q.-D. Hu, X.-Y. Yan and S.-M. Hunag, The role of programmed cell death in streptozotocin-induced early diabetic nephropathy, J. Endocrinol. Invest., 2011, 34, e296–e301 CrossRef CAS PubMed.
- M. Massot-Cladera, A. Franch, M. Castell and F. J. Pérez-Cano, Cocoa polyphenols and fiber modify colonic gene expression in rats, Eur. J. Nutr., 2017, 56, 1871–1885 CrossRef CAS PubMed.
- A. Costa-Bauza, F. Grases, P. Calvó, A. Rodriguez and R. M. Prieto, Effect of consumption of cocoa-derived products on uric acid crystallization in urine of healthy volunteers, Nutrients, 2018, 1516, DOI:10.3390/nu10101516.
- R. Sansone, J. I. Ottaviani, A. Rodriguez-Mateos, Y. Heinen, D. Noske, J. P. Spencer, A. Crozier, M. W. Merx, M. Kelm, H. Schroeter and C. Heiss, Methylxanthines enhance the effects of cocoa flavanols on cardiovascular function: randomized, double-masked controlled studies, Am. J. Clin. Nutr., 2017, 105, 352–360 CrossRef CAS PubMed.
- S. Reagan-Shaw, M. Nihal and N. Ahmad, Dose translation from animal to human studies revisited, FASEB J., 2008, 22, 659–661 CrossRef CAS PubMed.
- S. Almoosawi, L. Fyfe, C. Ho and E. Al-Dujaili, The effect of polyphenol-rich dark chocolate on fasting capillary whole blood glucose, total cholesterol, blood pressure and glucocorticoids in healthy overweight and obese subjects, Br. J. Nutr., 2010, 103, 842–850 CrossRef CAS PubMed.
|
This journal is © The Royal Society of Chemistry 2019 |
Click here to see how this site uses Cookies. View our privacy policy here.