DOI:
10.1039/C9FO00758J
(Paper)
Food Funct., 2019,
10, 6690-6698
The impact of reduced red and processed meat consumption on cardiovascular risk factors; an intervention trial in healthy volunteers
Received
12th April 2019
, Accepted 30th August 2019
First published on 27th September 2019
Abstract
Meat represents an important part of the diet for many adults, supplying essential amino acids and micronutrients. However, high red and processed meat (RPM) intake is implicated in the development of cardiovascular disease and dyslipidemia. This study aimed to reduce RPM consumption in healthy, non-obese omnivores (21–48 years), who ate RPM ≥4 times per week, and to investigate its effect on cardiovascular risk factors using a single-group longitudinal study design (comprising an initial 4-week baseline period, followed by a 12-week intervention). Participants (16M
:
21F) were assessed before (BL) and after (T0) the pre-intervention period and before (T0), at week 6 (T6) and at the end of intervention (T12). In a subset (8M
:
15F), haematological parameters were measured at BL, T6 and T12. Compared with BL, protein intake from RPM reduced by 67% at T6 and 47% at T12 (4-day dietary records). BMI, body fat mass, and blood pressure did not change over the intervention in the whole cohort, but mean total, LDL and HDL cholesterol were reduced in males at T12 (effect sizes −0.52, −0.41 and −0.15 mmol l−1, respectively; each P < 0.01), with no change in total
:
HDL ratio observed. In the study sub-set, haemoglobin concentration, plus red and white cell count fell during the intervention (estimated effect size ηp2 0.300, 0.301 and 0.354, respectively; each P < 0.001). It was possible for omnivores to approximately halve their RPM intake, and in males this dietary change appeared to reduce blood lipid concentrations. However, acute dietary changes to RPM intake may have had an unfavourable impact on haematological parameters.
Introduction
Meat represents an important part of a healthy balanced diet for many adults across the globe, supplying high quality protein, essential amino acids and micronutrients such as iron, vitamins A and B12, zinc, selenium and folic acid.1 The bioavailability and concentration of these micronutrients in plant-derived foods are generally lower,1,2 and plant protein is often of poorer quality (lower quantities of indispensable amino acids and/or poorer bioavailability), requiring higher intakes, or consumption of a combination of different plant sources. Indeed, the prevalence of some micronutrient deficiencies seems to be greater in vegetarian and vegan populations.3,4
The high protein and low carbohydrate properties of meat results in a low glycaemic index (GI) for this food group, and high protein-low GI diets are being recommended to improve weight management following weight-loss.5,6 However, diets high in red (pork, beef, lamb and veal) and processed meat (meats that have been smoked, cured or preserved in some way), have been implicated in the development of cardiovascular disease (CVD), and colon cancer.7,8 Indeed, data from the Health Professionals Follow-up, and Nurses’ Health Study indicate that those in the highest quintile of total red meat consumption had a 40% higher risk of cardiovascular mortality than those in the lowest.9
While the specific reason for the impact of red meat on CVD risk remains to be established, such products can contribute significantly to saturated fatty acid (SFA) intake, which is directly associated with elevated serum total (TC) and LDL cholesterol; risk factors for CVD.10,11 Atherosclerosis, the underlying cause of CVD, develops over decades and it may be important to reduce risk factors before the onset of clinical manifestation.
In addition to the potential impact of meat consumption on human health, there is increasing concern over the sustainability of livestock production and its impact on the environment in the face of global population growth and climate change.12 As such, both the health issues associated with high red meat consumption, together with the detrimental environmental impact of livestock production, have culminated in developed societies being encouraged to reduce meat intake.13,14 This has resulted in a marked increase in the adoption of vegetarian and vegan diets in numerous countries. However, many omnivores still face significant barriers to reducing meat intake,15,16 including a lack of knowledge and understanding of the availability, preparation and nutritional value of meat alternatives or meat substitutes.
The current study aimed to see whether a 50% reduction in red and processed meat (RPM) intake could be achieved in healthy, normal weight omnivores by encouraging the consumption of reduced meat and meat-free meals, and to monitor changes to blood lipid profile and other cardiovascular risk factors that might result from this behavioural change.
Results
Participants
Initial interest was expressed by 55 individuals, 46 of whom fulfilled inclusion criteria, and undertook the medical screening. In total, 43 of these individuals (22F
:
21M; BMI 18–28 kg m−2; aged 21–48 years) were suitable to participate. Three individuals withdrew from the study before the intervention, with 3 individuals withdrawing between assessment visits T6 (6 weeks into the meat-reduction intervention) and T12 (end of the intervention), citing other commitments and lack of time as the reason for drop-out. Thirty-seven participants (16M
:
21F) completed the protocol.
Although a matched group design was not specifically employed in the current protocol, at baseline (BL; on recruitment), male and female participants were similar with respect to age, fasting blood glucose, HOMA-IR, serum total cholesterol, LDL and triacylglycerides (TAG), whilst females demonstrated a higher serum HDL concentration when compared to males (Table 1).
Table 1 Parameters at baseline (BL; n = 37). M, males (n = 16). F, females (n = 21). BMI, body mass index. TC, total cholesterol. LDL, low-density lipoprotein. HDL, high-density lipoprotein. TAG, triacylglycerides. Mean values are expressed with standard deviation in parentheses; median values1 are shown with the 25th and 75th percentile in square brackets. P values for between-sex comparisons are shown in the final column
|
All |
Male |
Female |
M vs. F |
Age (y)1 |
27 [24–32] |
27 [24–30] |
27 [24–32] |
0.964 |
BMI (kg m−2) |
23.1 (2.29) |
23.4 (1.67) |
22.9 (2.70) |
0.503 |
Fasting glucose (mmol l−1) |
4.07 (0.34) |
4.16 (0.35) |
4.00 (0.33) |
0.173 |
HOMA-IR1 |
1.73 [1.40–2.14] |
1.68 [1.21–1.96] |
1.87 [1.54–2.34] |
0.138 |
TC (mmol l−1) |
3.87 (0.74) |
3.75 (0.86) |
3.96 (0.65) |
0.408 |
LDL (mmol l−1) |
2.11 (0.63) |
2.24 (0.75) |
2.01 (0.52) |
0.303 |
HDL (mmol l−1) |
1.37 (0.35) |
1.14 (0.23) |
1.54 (0.32) |
<0.001 |
TAG (mmol l−1) |
0.67 (0.23) |
0.68 (0.16) |
0.67 (0.28) |
0.945 |
Dietary intake
Complete sets of diet diary data (4 time points) were collected in 34 participants (16M
:
18F). The median [25th–75th percentile] relative reported frequency of eating RPM was 9 [7–11] times per week at BL, 11 [5–12] at T0, 4 [2–7] at T6 and 5 [1–8] times per week at T12, with 8 out of 34 participants (24%) recording no RPM intake in their T12 diet diary. There were no sex differences in the reduction in frequency of RPM consumption, and 96% of the whole cohort reported reducing the number of times they consumed these products per week. Participants could also reduce their meat intake by changing portion size, and during the intervention average protein intake from RPM was significantly lower at both T6 and T12 when compared with BL (P < 0.005 in each case). However, 9 (3F
:
6M) participants did not have a protein intake from RPM that was ≥50% below BL intake at T6, with 5 (1F
:
4M) of these individuals also reporting a protein intake from RPM that was not ≥50% below BL intake at T12. Protein intake derived from RPM showed a strong trend (P = 0.052) to be higher at T0 (end of the pre-intervention period) than at BL, with over half (19 out of 32) reporting eating more at T0. Daily protein intake provided by seafood, chicken, and dairy did not change over the pre-intervention or intervention periods, and intake from pulses and nuts (P < 0.001) and mycoprotein (P < 0.001) during the intervention was greater than at T0 (Fig. 1).
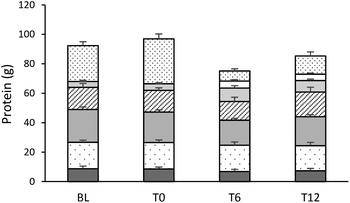 |
| Fig. 1 Mean protein intake contributed by 7 food groups. seafood, dairy, ‘starchy’ foods, chicken, pulses and nuts, mycoprotein and red and processed meats. Error bars display the standard error of the mean for each food grouping. | |
Over the pre-intervention period, no change in macronutrient intake was seen in the whole cohort, and the macronutrient composition of the diet (expressed as a percentage of total energy intake) did not change over the pre-intervention or intervention period (Table 2). However, in the whole cohort, EI, total and saturated (SFA) dietary fat intake were lower at both T6 and T12 compared with T0 (P < 0.05 in each case; Table 2). When separated by sex, total fat intake in women was reduced during the intervention (P < 0.05), with a trend for EI, total fat and SFA intake to be lower in men (P = 0.087, P = 0.068 and P = 0.087, respectively). Carbohydrate intake during the intervention period did not change in the whole cohort or within sex, whereas a significant alteration in AOAC fibre intake over the intervention was noted in the women (P < 0.05). Similarly, in women, a trend for a difference in protein intake over the intervention was seen (P = 0.066), although no such change in protein intake was observed in males (Table 2).
Table 2 Macronutrient dietary intake during the pre-intervention (BL and T0) and intervention (T6 and T12) periods (n = 34). SFA, saturated fatty acids. PUFA, polyunsaturated fatty acids. CHO, carbohydrate. Fibre, AOAC fibre. % Fat, percentage of total daily energy intake provided by fat. % Protein, percentage provided by protein. % CHO, percentage provided by carbohydrate. Mean values are expressed with standard deviation in parentheses, median values are shown with the 25th and 75th percentile in square brackets. *P < 0.05 compared to pre-intervention (T0)
|
|
BL |
T0 |
T6 |
T12 |
Energy (kJ) |
Men |
9862 [9203–11 641] |
10 635 [9003–10 971] |
9081 [7350–9922] |
9153 [6678–11 544] |
Women |
8684 [8066–10 091] |
8713 [7712–11 058] |
*7974 [6650–8917] |
7974 [6721–9730] |
All |
9395 [8295–10 953] |
10 295 [7988–10 952] |
*8472 [7018–9414] |
*8328 [6782–10 132] |
Total fat (g) |
Men |
96.8 [79.3–112.6] |
106.2 [89.3–136.3] |
83.1 [65.8–92.1] |
89.1 [73.0–110.0] |
Women |
86.7 [72.2–103.7] |
80.9 [67.3–104.9] |
76.9 [56.8–91.5] |
70.3 [58.5–87.2] |
All |
89.7 [77.7–109.9] |
91.0 [74.4–125.3] |
*79.7 [61.1–91.8] |
*79.0 [64.9–102.0] |
SFA (g) |
Men |
34.3 [28.6–43.1] |
37.2 [26.8–48.9] |
25.4 [22.4–35.9] |
32.2 [24.0–38.8] |
Women |
30.3 [25.5–38.2] |
28.2 [25.4–34.8] |
23.3 [17.3–31.1] |
22.1 [18.0–30.2] |
All |
30.9 [26.3–41.6] |
32.4 [25.6–44.4] |
*25.0 [18.6–32.3] |
*26.9 [21.4–35.2] |
PUFA (g) |
Men |
9.8 [12.8–14.7] |
8.5 [11.8–16.3] |
9.9 [12.8–16.2] |
11.3 [14.5–17.1] |
Women |
9.2 [11.8–16.1] |
9.2 [11.6–17.4] |
7.9 [11.8–15.6] |
8.1 [10.5–14.5] |
All |
9.6 [12.1–15.5] |
8.7 [11.6–16.6] |
8.5 [12.7–15.6] |
8.93 [12.7–16.5] |
Protein (g) |
Men |
122.2 [98.7–143.9] |
114.0 [91.5–142.4] |
102.5 [84.7–124.8] |
112.2 [97.3–126.7] |
Women |
85.0 [74.0–112.4] |
95.6 [78.5–117.5] |
76.9 [60.5–98.8] |
89.8 [73.5–104.7] |
All |
101.1 [82.2–125.3] |
100.2 [79.1–125.0] |
92.1 [68.2–105.7] |
98.3 [84.7–115.1] |
CHO (g) |
Men |
269.4 (81.1) |
271.5 (73.0) |
262.6 (82.1) |
254.4 (93.5) |
Women |
258.7 (75.7) |
248.6 (68.0) |
219.6 (59.2) |
235.6 (56.1) |
All |
263.8 (77.3) |
259.4 (70.3) |
240.0 (73.1) |
244.5 (75.4) |
Fibre (g) |
Men |
21.7 (5.3) |
23.1 (6.4) |
25.8 (4.9) |
26.2 (8.0) |
Women |
24.0 (10.7) |
24.0 (10.3) |
22.3 (8.4) |
26.5 (9.2) |
All |
22.9 (8.6) |
23.6 (8.6) |
24.0 (7.1) |
26.3 (8.5) |
% Fat |
Men |
35.5 [32.0–38.5] |
39.0 [34.0–43.3] |
34.5 [28.0–41.5] |
35.5 [32.3–38.0] |
Women |
37.0 [33.5–39.0] |
37.5 [32.0–41.3] |
35.0 [32.3–41.0] |
34.0 [30.0–38.0] |
All |
36.0 [32.0–39.0] |
38.0 [32.8–41.3] |
35.0 [30.0–41.0] |
35.0 [31.0–38.0] |
% Protein |
Men |
20.0 (3.6) |
18.8 (4.5) |
19.2 (4.3) |
20.2 (4.6) |
Women |
16.7 (2.7) |
17.7 (3.9) |
17.2 (3.9) |
18.9 (4.5) |
All |
18.3 (3.5) |
18.2 (4.1) |
18.2 (4.2) |
19.5 (4.5) |
% CHO |
Men |
41.3 (10.9) |
41.9 (12.2) |
45.6 (9.6) |
43.1 (7.0) |
Women |
45.6 (7.0) |
43.3 (7.7) |
45.0 (9.2) |
46.1 (7.0) |
All |
43.5 (9.2) |
42.7 (9.9) |
45.3 (9.3) |
44.7 (7.1) |
Anthropometry and blood pressure
At BL, all participants were normal weight, with mean BMI being similar between men and women (Table 1). BMI did not change between BL and T0, or over the intervention. Females demonstrated higher percentage body fat than men (P < 0.001), but within sex, no changes to percentage body fat were observed over the pre-intervention or the intervention period (Table 3).
Table 3 Anthropometric and blood pressure measurements during the pre-intervention period (BL and T0) and intervention (T6 and T12) periods. Where sex differences were noted at BL, data are presented for males (M; n = 16) and females (F; n = 21) separately. Where no such differences were observed, data have been combined and are displayed for the whole cohort (n = 37). SBP, systolic blood pressure. DBP, systolic blood pressure. Mean values are expressed with the standard deviation in parentheses; median values1 are shown with the 25th and 75th percentile in square brackets. Between sex comparisons at BL, *P < 0.05, **P < 0.01
|
|
BL |
T0 |
T6 |
T12 |
BMI (kg m−2) |
All |
23.1 (2.29) |
23.3 (2.41) |
23.3 (2.43) |
23.3 (2.42) |
Body fat (%) |
M |
**13.8 (3.6) |
14.9 (4.5) |
13.6 (4.3) |
14.3 (4.5) |
F |
27.2 (6.1) |
26.7 (6.6) |
28.9 (5.9) |
26.4 (6.2) |
All |
21.7 (8.4) |
21.8 (8.2) |
20.8 (8.0) |
21.4 (8.2) |
SBP (mmHg) |
M |
*116 (8.7) |
115 (10.7) |
118 (9.3) |
112 (9.1) |
F |
109 (10.8) |
108 (10.1) |
108 (10.8) |
108 (11.8) |
All |
112 (10.6) |
111 (10.8) |
112 (11.1) |
110 (10.8) |
DBP1 (mmHg) |
All |
68 [66–69] |
67 [62–71] |
67 [64–73] |
67 [62–72] |
Males had higher systolic blood pressure (SBP) than females throughout the study (P < 0.05). No change in SBP was seen between BL and T0 in either sex, and SBP was the same in females across the intervention. However, there was a weak trend for a change in this variable to occur across the intervention in men (P = 0.096), with a significant fall in SBP noted between T6 and T12 (P < 0.05). Diastolic blood pressure was similar between sexes, and this variable did not change in the whole cohort across the pre-, or intervention period.
Lipids
Participants in the current study were not recruited on the basis of specified circulating lipid concentrations, but the mean concentration of lipid parameters reflect the ‘healthy’10 nature of the cohort (Table 1). However, two participants (1M
:
1F) demonstrated fasting TC values above the healthy range at the start of the study, with ten (7M
:
3F) having LDL values above 2.6 mmol l−1, although all were below a concentration which would require therapeutic intervention, in the UK, in otherwise healthy individuals.17 The response of serum LDL to the intervention was different in men and women (P < 0.05), with LDL falling significantly over the 12 weeks in the male participants (P < 0.01), but remaining unchanged in the females (Fig. 2a). In females, there was no change in LDL during the pre-intervention period, whereas in males this variable increased at T0 when compared with BL. In men, serum LDL was significantly lower at the end of the intervention compared to T0 (P < 0.01). However, serum LDL concentration was not significantly lower at T12 when compared to values observed at BL. In males, there was a significant correlation between the concentration of serum LDL at the start of the intervention (T0) and the change in this variable at the end of the intervention (R = 0.771, P < 0.001), with those starting with higher serum LDL demonstrating greater reduction over the 12 weeks intervention. Furthermore, the concentration of serum LDL at BL remained significantly associated (R = 0.735, P < 0.001) with a change in this variable at the end of the intervention (T12), with no such correlation observed in females irrespective of whether LDL concentration at T0 or BL was used as the comparator to T12.
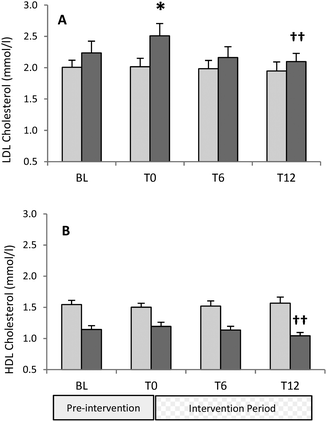 |
| Fig. 2 Mean serum LDL (A) and HDL cholesterol (B) across the pre-intervention and intervention period in women ( ) and men ( ). *P < 0.05 compared with BL, ††P < 0.01 compared with start of intervention (T0). Error bars indicate the standard error of the mean. | |
In males, LDL concentration was significantly, albeit weakly, correlated with dietary total fat intake (R = 0.251; P < 0.05) and negatively correlated with AOAC fibre intake (R = −0.274; P < 0.05), with a trend for a positive correlation with dietary cholesterol consumption (R = 0.231; P = 0.067). There was no co-linearity detected between total fat and AOAC fibre intake, and a combined regression model accounted for 32% of the variance in serum LDL concentration in men (P < 0.001). In women, a significant association between LDL and reported dietary fat and cholesterol intake was not observed, although a correlation between this variable and AOAC fibre intake, of a similar magnitude to that seen in men, was noted (R = −0.249; P < 0.05).
Fasting serum HDL concentration did not change between BL and T0 in either sex (Fig. 2b). Similarly, no changes in HDL were noted over the intervention period in the female participants. However, there was a significant reduction in HDL concentration over the intervention in men (P < 0.05) with this variable being lower at T12 when compared with T0 (P < 0.01), but also significantly lower when compared to values measured at BL (P < 0.05). It is worth noting that total cholesterol
:
HDL ratio did not change over the intervention in males (ratio at BL 3.49 vs. 3.52 at T12).
Serum total cholesterol (TC) data largely reflect the changes observed in LDL and HDL, with the response of TC to the intervention being different in men and women (P < 0.05), and serum TC falling significantly over the 12 weeks in the male participants (effect size ηp2 0.270; P < 0.01), but remaining unchanged in females. Similarly, in females there was no change in mean TC from BL to T0 (−0.03 (0.65) mmol l−1), whereas in males this variable increased over this time frame (0.34 (0.55) mmol l−1; P < 0.05). In men, serum TC was significantly lower at T12 than at T0 (−0.52 (0.58) mmol l−1; P < 0.005), but not significantly lower when compared to BL values (−0.18 (0.50) mmol l−1). As with LDL, the concentration of serum TC at T0 and BL were both significantly correlated with the change in this variable at T12 (R = 0.804, P < 0.001 and R = 0.722, P < 0.005, respectively), with the greatest reduction being observed in those with higher total cholesterol concentrations at T0 or BL.
However, there was no such association in the women, irrespective of whether values at BL or T0 were used (R = 0.145, P = 0.530 and R = 0.087, P = 0.710 respectively).
Circulating concentration of TAG was not different between sexes at BL and did not change over the pre-intervention or intervention period in the whole cohort or within sex.
Insulin resistance
As with other normal weight cohorts reported in the literature, HOMA-IR values did not indicate the presence of fasting insulin resistance at the start of the study (Table 1). Men and women were matched for HOMA-IR at BL and this measure did not alter in the whole cohort, as a result of the pre-intervention period. However, HOMA-IR changed over the intervention period (P < 0.005), with median HOMA-IR increasing from 1.71 [1.34–2.23] at T0 to 2.05 [1.67–2.46] at T6 (P < 0.025), but returning to pre-intervention values at T12 (1.65 [1.25–2.25]).
Haematological measures
Erythrocyte parameters.
Haematological measures were collected in 23 individuals (8M
:
15F) and in these participants, males had significantly greater circulating haemoglobin (Hb) than females (Table 4; P < 0.01). During the intervention, a reduction in Hb concentration was observed in men and women (P < 0.05 for each sex), with the response being similar between sexes. Hb concentration in males was significantly lower at T12 (P < 0.025) when compared to the start of the study, and in females this variable was significantly lower at T6 (P < 0.025; Table 4).
Table 4 Haematological parameters at BL and during the intervention (T6 and T12) in a sub-set of the study cohort (15F
:
8M). Where differences between sex were identified at BL, data for men and women are displayed separately. Where values at BL were the same between sex, data have been combined and values for all participants (n = 23) are reported. RCC, red cell count. Hb, haemoglobin. MCH, mean corpuscular haemoglobin. MCV, mean corpuscular volume. WCC, white cell count. Mean values are expressed with the standard deviation in parentheses; median values1 are shown with the 25th and 75th percentile in square brackets. Comparisons to BL *P < 0.025, **P < 0.01
|
|
BL |
T6 |
T12 |
RCC (x1012 l−1) |
Men |
4.95 (0.28) |
*4.73 (0.23) |
*4.83 (0.25) |
Women |
4.49 (0.40) |
*4.32 (0.32) |
4.40 (0.34) |
All |
4.65 (0.42) |
**4.46 (0.35) |
**4.54 (0.37) |
Hb1 (g l−1) |
Men |
146 [144–150] |
141 [134–144] |
*139 [137–147] |
Women |
134 [127–141] |
*131 [125–134] |
133 [126–136] |
All |
141 [132–145] |
**133 [129–138] |
*136 [128–139] |
MCH (pg) |
All |
29.8 (1.13) |
29.8 (1.02) |
29.6 (1.05) |
MCV (fl) |
Men |
86.0 (1.77) |
*84.6 (1.85) |
84.6 (1.60) |
Women |
89.1 (3.35) |
88.9 (2.95) |
**88.0 (3.07) |
All |
88.0 (3.22) |
87.4 (3.30) |
**86.8 (3.08) |
WCC1 (x109 l−1) |
All |
6.9 [5.8–7.5] |
**5.0 [4.1–6.0] |
**5.2 [4.8–5.8] |
Neutrophils1 (x109 l−1) |
All |
3.7 [3.1–4.3] |
**2.6 [2.1–3.8] |
**2.7 [2.4–3.2] |
Platelets1 (x109 l−1) |
All |
230 [221–242] |
**207 [189–236] |
*217 [198–246] |
Results for Hb appeared to be related to red cell count (RCC), as Mean Corpuscular Haemoglobin (MCH) was similar between sexes at the start of the study and this variable did not change in men or women over the study period, whereas the pattern of change in RCC mirrored those already described for Hb (Table 4). Mean Corpuscular Volume (MCV) did reduce over the intervention in both men and women (P < 0.05 in each case), but a MCV below normal values was only detected in one female at T6, and three at T12. Pencil cells and the presence of other poikilocytes were not reported.
One female participant developed an Hb concentration and RCC below the normal range during the meat reduction period (Hb 112 g l−1 at T6 and 114 g l−1 at T12; RCC 3.6 and 3.7 × 1012 cells per l at T6 and T12 respectively). On clinical advice this individual was not withdrawn from the intervention at week 6 (T6) when reduced Hb and RCC was first noted, and at a follow-up (2 months after the intervention had been completed), Hb and RCC had reverted back to being within the normal range.
Leukocyte parameters.
There were no statistical differences, between sex, for white cell, neutrophil and platelet count at BL and response to the intervention was similar in men and women. During the RPM reduction phase of the study, a change in all three variables was detected (P < 0.005 in each case), with cell counts being significantly lower at T6 and T12 when compared to starting values. Indeed, 47% of females and 50% of males had a fall in neutrophil count of >1 × 109 l−1, with four women and one man demonstrating a neutrophil count just below the normal range at T6. At T12, only one woman still demonstrated a neutrophil count below normal levels, although 40% of the females and 25% of males still had a neutrophil count which was >1 × 109 l−1 below starting values. All white cell parameters returned to being within the normal range at the 2-month follow-up.
Discussion
Omnivorous diets high in RPM have been implicated in the development of a number of chronic diseases, including CVD.7,8 Analysis of the combined Health Professionals Study (37
698 men) and Nurses Health Study (83
644 women) suggested that reducing red meat intake by 1 portion (equivalent of 85 g fresh red meat) per day could reduce mortality risk by 7–18%,9 whilst in a modelling study, Aston et al. reported a 2.5-fold difference in RPM intake between the highest and lowest quintiles of consumers, and predicted that by moving from the highest to the lowest quintile of processed (but not fresh) meat, men could potentially reduce coronary heart disease risk by 20.6% and women by 11.1%.18 Although processed meat is generally associated with high intakes of salt and other preservatives (such as nitrites and nitrates) which may influence hypertension, the mechanisms whereby RPM consumption is associated with CVD remain to be fully elucidated, with the impact of associated SFA on plasma LDL cholesterol often suggested to be the primary effect.19 However, in the combined Health Professionals Study and Nurses Health Study, residual effects of meat consumption were apparent even after accounting for differences in SFA (and dietary cholesterol) intake.9
In the present study, median RPM consumption decreased from 1.3 portions a day to 0.7 portions, and this dietary change appeared to be a strong enough stimulus to bring about a reduction in LDL in the men but not women. Any potential benefit of this was, at least partly, offset by a decrease in HDL. This obviously leads to questions concerning compliance of the female participants. It was possible that dietary records for females in the current study did not reflect actual behaviour and they did not reduce their RPM intake, or any changes made did not impact dietary fat intake to the same degree as the men. However, subjectively authors did not note a difference in engagement with the study protocol between sexes, and several couples were included in the cohort, such that meals were being shared by both individuals within the couple. Indeed, although results should be viewed with caution (due to limitations associated with dietary records), numerical changes in absolute intake and percentage contribution of SFA, PUFA, trans-fats and cholesterol to total EI, during the intervention were not different in men and women. Moreover, similar responses in haematological variables were observed, suggesting that whatever aspect of the intervention was driving these changes was similar in both sexes. Average TC concentrations in women have been shown to vary during the menstrual cycle by approximately 0.2 mmol l−1
20 and this variance is of a similar magnitude to the diet-induced reduction in TC seen in males (compared with BL values). In the current protocol, blood sampling time points were not standardised for point in the menstrual cycle, and it is possible that normal fluctuations in TC were masking diet-induced changes in women. However, we propose that a more likely explanation for this lack of response in women is that the effect of RPM reduction on total fat, SFA and cholesterol intake, despite being of the same magnitude as achieved in men, was not a sufficient stimulus to induce blood lipid changes in women. Diets designed to reduce LDL in those at risk of CVD aim to lower SFA to approximately 5% of total EI,21 whereas reduction of SFA in the current study was much smaller (control period 12.9% of EI; intervention period 11.5% of EI). In humans there does not appear to be differences in intestinal fat absorption between the sexes22 but differences in lipid metabolism, such as postprandial LDL clearance rates23 may be sufficient to influence the effect that small dietary changes have on circulating lipids.
Despite the current study participants demonstrating lipoprotein cholesterol concentrations within the ‘normal’ range, and as a result the range to assess a change to these parameters was narrow, a significant reduction in LDL and HDL was induced over the intervention period in men. Although these findings may have been confounded by changes in behaviour during the pre-intervention period, which tended to elevate TC and LDL from BL concentrations, those with total and LDL cholesterol concentrations in the upper ranges of normal at BL still demonstrated greater reduction in this variable over the intervention period compared with those starting with lower TC and LDL levels. This suggests that in males those who have the highest level of these CVD risk factors may benefit most from reducing their RPM intake.
Although meat-based diets are rich in the essential micronutrients required for haemopoiesis, the extent to which the intervention appeared to impact RCC, WCC and platelet count was surprising. In Western societies, reduced RPM intake is now being advocated.13,14 However, veganism is associated with a risk of vitamin B12 deficiency,24 and established vegetarians have been shown to have reduced RCC and WCC compared to age matched omnivores.25 Unfortunately, these parameters are rarely reported in dietary interventions that acutely reduce meat intake, but reductions in blood cell counts were not observed when older omnivores changed to a vegetarian diet for 12 weeks.26 No detectable changes in dietary intake of copper, iron, zinc, or the B vitamins were noted from the diet diaries over the study. However, 4-day recording is not sufficient to reliably investigate changes in micronutrient intake.27
A strength of the current study was that the longitudinal study design allowed changes over time, within individuals, to be assessed, a feature that is not possible with cross-sectional studies used to infer links between dietary variables and blood lipid profile. However, a notable weakness was that it is likely that small changes to the habitual diet (in relation to RPM intake) occurred during the pre-intervention period, which may have led to an overestimation of the dietary change and (in males) the serum lipid reduction that could be achieved by the intervention. Indeed, anecdotally, several participants reported using up their meat stores prior to starting the intervention, and a strong trend for increased intake of protein from RPM was noted at T0. Despite the limitations, correlation findings illustrate the impact that RPM consumption and dietary fat content could have on LDL parameters in healthy men and identified that males and premenopausal women may respond differently to small dietary changes.
Experimental
Trial design
A single group, longitudinal study design was adopted, comprising an initial 4-week baseline (‘pre-intervention’) period followed by a 12-week intervention. To maximise the statistical power of the study, a within subject design was favoured, but behavioural interventions do not lend themselves to a randomised cross-over design, as any behaviour change message delivered during a first phase could affect behaviour at the second. Assessment visits to the laboratory were scheduled before and after each period; BL pre-baseline period (week-4), T0 post baseline period/start of intervention (week 0) and T12 at end of the intervention period (week 12), with an interim assessment (T6) made at the intervention midpoint (week 6).
This study was conducted in accordance with the guidelines laid down in the Declaration of Helsinki of 1975 (as revised in 1983) and all procedures involving human participants were approved by the University of Nottingham Medical School Ethics Committee (reference A18062015). Written informed consent was obtained from all subjects. The study was registered at http://www.ClinicalTrials.gov (reference NCT02907112).
Participants
Forty-six (23 males (M)
:
23 females (F)) volunteers from the general population of Nottinghamshire, UK attended the David Greenfield Human Physiology Unit (Queen's Medical Centre, Nottingham, UK) for an initial medical screening visit to confirm health status and frequency of eating RPM; a threshold for the weight of meat consumed was not used as a recruitment criteria. Blood pressure (BP) was measured at the left upper arm (after lying semi-supine for 5 min) using automated oscillometry (Dynamap Pro 1000; GE Medical, Milwaukee, USA), and body mass index (BMI) was calculated from measured height and weight. If participants were suitable to take part with regards to their health questionnaire, reported habitual meat intake in a food frequency questionnaire (across 11 meat and 1 poultry categories) and BMI measures (BMI < 28 kg m−2), a blood sample was then taken from a forearm vein to assess full blood count, urea, and electrolytes (Dept of Pathology Queen's Medical Centre, Nottingham, UK). Those taking lipid-lowering medication, or who reported using nutritional strategies to lower their cholesterol, were excluded. Participants were accepted onto the trial if they reported habitual consumption of 4 or more portions of red and/or processed meat per week (with 3 or more of these being consumed as main meals), they did not have food allergies related to soya or mycoprotein, and did not demonstrate any clinically significant abnormalities on screening. Eligibility criteria were not altered during the recruitment period.
Study protocol
After recruitment, participants were asked to record all food intake (including snacks and drinks), in a diet diary, for 4 days (3× week or work days, and 1× weekend or rest day) prior to BL visit. This provided a baseline assessment of dietary macronutrient, energy and meat intake. Individuals were asked to complete further 4-day diet diaries in the week before assessment visits T0, T6 and T12 (weeks-1, 5, and 11), to assess any changes in macronutrient or energy intake, and meat consumption, which may have occurred as a consequence of participating in the study. Household measures were used to estimate portion size, and diaries were subsequently analysed using a food composition database (Nutritics, Dublin, Eire). To calculate habitual diet composition, a mean daily intake was obtained from all 4 days of each recording period, and macronutrient composition was expressed as a percentage of total energy intake. This individual information was combined to produce grouped data for the whole cohort and for males and females. To identify changes to habitual dietary behaviour, all foods recorded in the individuals’ diet diaries were allocated to 12 food groups, 7 of which represented major dietary protein contributors; red and processed meat, seafood (fish and shell fish), chicken, dairy (milk, cheese, yogurt and eggs), pulses and nuts, mycoprotein and ‘starchy’ foods (breads, cereals, rice and potato). Prior to food group allocation, components of composite foods were calculated from recipes provided by the participants, or where relevant, from ingredient information provided by manufacturers. The macronutrient composition of each food item within categories was calculated from weight consumed, and within each of these 7 food group categories, an average value for each macronutrient (protein, fat, carbohydrate) was produced for every individual. These were combined to form group means as before. Mean protein intake contributed by the RPM category was used to identify any changes to intake over the control and intervention period. Where RPM content of foods, such as sausages, could not be identified, an assumption was made that the protein content of that food was provided by the meat component.
Assessment visits took place in the morning after the individual had fasted from midnight the night before. Initially, measurement of body mass was made to the nearest 0.1 kg using a Seca 882 Digital Scale (Birmingham, UK). Participants were then asked to rest, semi-supine, on a couch and estimation of body composition was made using bioelectrical impedance (BodyStat Ltd, Douglas, Isle of Man), with resting blood pressure measured as described above. A 5 ml fasting blood sample was taken for determination of serum insulin, total cholesterol (TC), HDL, LDL, TAG and whole blood glucose. Four millilitres of blood were placed into a Vacutainer SST™ tube (Becton, Dickinson and Co, USA) and left at room temperature for 20 min to clot. The sample was then centrifuged for 10 min (3000 rcf, 4 °C), the serum was transferred to labelled micro-tubes, and stored at −80 °C until analysis for lipids and insulin concentration. The remaining blood collection was transferred into a fluoride heparin microtube (Sarstedt, Germany), mixed well and whole blood glucose then assessed within 10 min using the glucose oxidase method (Yellowsprings Inc., Yellowsprings, CO, USA; within assay precision being 1%). Homeostatic assessment of insulin resistance (HOMA-IR), an index of fasting insulin resistance, was subsequently calculated from insulin and glucose concentration according to standard methods.28
In a convenience sample of 23 individuals, who represent all those recruited in the period between January and April 2016 (8M
:
15F), an additional 1 ml of blood was taken at T6 and T12, placed into a Vaccutainer EDTA tube (Becton, Dickinson and Co, USA), and sent to the Clinical Pathology Laboratories at Queens Medical Centre (Nottingham University Hospitals NHS Trust, UK) for determination of full blood count using an XN-9000 automated cell counter (Sysmex UK Ltd, Milton Keynes, UK). Reference values provided by these laboratories were used to define the normal range of haematological parameters; Hb 130–180 g l−1, MCH 28–33 pg, RCC 4.2–6.5 × 1012 l−1, MCV 84–102 fl, WCC 4–11 × 109 l−1, neutrophils 2.0–7.5 × 109 l−1, platelets 150–450 × 109 l−1.
Intervention
After initial measurements (BL; week-4), participants were asked not to make any changes to their lifestyle and to continue their habitual diet until the second visit (T0; week 0) when a second assessment was made (‘pre-intervention’ period). The 12 weeks intervention period began on the day after the second study visit. At this point, participants were given information regarding how meat intake could safely be reduced in their diet, including a recipe book containing ideas for meals using meat analogues (meat-free or reduced-meat products that resemble commonly consumed meat foodstuffs) and plant foods which are rich sources of protein. However, beyond the request to reduce RPM intake by 50%, participants were not given set menus to follow, or any stipulation on which strategy/strategies they were to use to achieve their meat-reduction target. To assist with this reduction in RPM intake, a range of frozen and chilled meat-free, and frozen reduced-meat products were available for the participants to use if they wished. These included soya (Tescos PLC, Welwyn Garden City, UK) or mycoprotein (Quorn™) mince, Quorn™ sausages, Quorn™ ‘meatballs’ and Quorn™ ‘steak strips’ (all Quorn™ products were provided by Marlow Foods, Stokesley, UK). The frozen reduced-meat products included pork sausages, beef meatballs, beef mince and beef burgers manufactured specifically for the project by DuPont (Aarhus, DK), and had 50% of the meat content replaced with soya or mycoprotein. In addition, 2 chilled mycoprotein products were offered as an alternative to delicatessen meats (Quorn™ ‘ham’, Quorn™ ‘Turkey’ slices), and a range of dried beans (red kidney, haricot, black turtle), pulses (chick peas, green and red lentils) and grains (quinoa) were also available. Pulses, lentils and grains were supplied by A Poortman Ltd (London, UK). Monthly newsletters and weekly email contact with the study participants was maintained over the intervention period to improve compliance, to identify any problems and to arrange further food deliveries if required.
In those who had demonstrated blood cell counts or Hb levels below the normal range, a follow-up assessment of haematological parameters was arranged 8 weeks after the end of the study.
Analytical methods
Serum insulin was assessed using a human-specific radioimmunoassay (Merck Millipore, Billerica, MA, USA), with an intra-assay coefficient of variation (CV) of 4.41%, and an inter-assay CV of 10.53%. TAG, TC, LDL and HDL were measured using enzymic photometric methods (Horiba Medical, Montpellier, France), and the intra-assay CVs were 2.16%, 2.63%, 2.21% and 0.77% respectively, with inter-assay CVs being 3.27%, 2.6%, 4.77% and 4.72%, respectively.
Statistical methods
All data were coded and analysed using SPSS version 22.0 (Statistical Package for the Social Sciences 2013). Data were initially checked for normality of distribution (using criteria of skewness and kurtosis z-score between −1.96 and 1.96). Data with a normal distribution are described in the text and tables as the mean with the standard deviation in parentheses, and displayed in figures as the mean with error bars indicating the standard error of the mean (SEM). Data which did not show a normal distribution are displayed in the text and tables as the median with the 25% and 75% percentile values shown in square brackets.
Between sex comparisons at a single time point were made using unpaired Student's t-test or Mann–Whitney U as appropriate, with assessment of responses between sexes within study periods (pre-intervention and intervention), carried out using 2-way repeated measures ANOVA. Violation of the assumption of sphericity was assessed using Mauchly's test, and where appropriate the Greenhouse-Geisser statistic used to determine the significance level. Where differences in BL values or responses were noted between sexes, data for men and women were analysed and are reported separately. Where no differences were observed, data were analysed and are reported for the whole cohort to improve statistical power.
One-way repeated measures ANOVA (or Friedman's test) was used to investigate changes across the intervention (in the whole cohort, or within sex) and across the pre-intervention period paired Student's t-test, or Wilcoxon signed ranks was used. All analysis used a two-tailed assessment and statistical significance was assumed where P < 0.05. Post-hoc analysis, with Bonferroni correction was used to probe differences in repeated measures data, and multiple regression analysis utilised to investigate relationships between dietary intake of macronutrients and serum lipid concentration.
Sample size
Pilot data generated within the research group was used to inform the variability of serum TC and LDL concentration in a young, healthy male and female population (n = 25), and the magnitude of change which could be expected with a dietary intervention. In this pilot cohort, average TC was 4.45 (0.54) mmol l−1 and a reduction of 0.41 mmol l−1 was observed after a 4 weeks period of red and processed meat reduction. From these data, a sample size of sixteen participants was predicted to be able to detect a difference in mean TC concentration with a statistical power at the level of 80% in the current study. Average LDL concentration for this group was 2.40 (0.37) mmol l−1 and a reduction of 0.25 mmol l−1 was observed. Sample size expected to power this variable would be 20 individuals. To allow investigation of any effects of the intervention within sex, it was proposed that 20 men and 20 women were recruited.
Conclusions
It was possible for omnivores to approximately halve their RPM intake over a 12 weeks period, but this dietary change was accompanied by an unfavourable effect on blood cell counts. There was a suggestion that reducing red meat intake was of greatest benefit to men with higher LDL and total cholesterol concentration at the start of the study, whilst blood lipid profiles in women were unaffected by reported dietary changes. In future dietary interventions modifying meat intake, close monitoring of haematological parameters and detailed dietary assessment would be recommended.
Conflicts of interest
No conflicts of interest to declare
Acknowledgements
Authors would like to thank Mrs S Cordon for carrying out the biochemical analysis, Miss M. Ali for entering the diet diary data, Marlow Foods for providing the frozen and chilled meat alternatives, and A. Poortman Ltd for supplying the dried beans and pulses. This study was funded by the BBSRC and MRC through Innovate UK (project reference 101732).
Notes and references
- H. K. Biesalski, Meat as a component of a healthy diet - are there any risks or benefits if meat is avoided in the diet?, Meat Sci., 2005, 70, 509–524 CrossRef PubMed.
- R. S. Gibson, Content and bioavailability of trace elements in vegetarian diets, Am. J. Clin. Nutr., 1994, 59, 1223S–1232S CrossRef CAS PubMed.
- L. M. Haider, L. Schwingshackl and G. Hoffmann,
et al., The effect of vegetarian diets on iron status in adults: A systematic review and meta-analysis, Crit. Rev. Food Sci. Nutr., 2018, 58, 1359–1374 CrossRef CAS PubMed.
- D. Alexander, M. J. Ball and J. Mann, Nutrient intake and haematological status of vegetarians and age-sex matched omnivores, Eur. J. Clin. Nutr., 1994, 48, 538–546 CAS.
- T. M. Larsen, S. M. Dalskov and M. van Baak,
et al., Diets with high or low protein content and glycemic index for weight-loss maintenance, N. Engl. J. Med., 2010, 363, 2102–2113 CrossRef CAS PubMed.
- D. Paddon-Jones, E. Westman and R. D. Mattes,
et al., Protein, weight management, and satiety, Am. J. Clin. Nutr., 2008, 87, 1558S–1561S CrossRef CAS PubMed.
- S. Rohrmann, K. Overvad and H. B. Bueno-de-Mesquita,
et al., Meat consumption and mortality–results from the European Prospective Investigation into Cancer and Nutrition, BMC Med., 2013, 11, 63 CrossRef PubMed.
- G. C. Chen, D. B. Lv and Z. Pang,
et al., Red and processed meat consumption and risk of stroke: a meta-analysis of prospective cohort studies, Eur. J. Clin. Nutr., 2013, 67, 91–95 CrossRef PubMed.
- A. Pan, Q. Sun and A. M. Bernstein,
et al., Red meat consumption and mortality: results from 2 prospective cohort studies, Arch. Intern. Med., 2012, 172, 555–563 CrossRef PubMed.
- Third Report of the National Cholesterol Education Program (NCEP) Expert Panel on detection, evaluation, and treatment of high blood cholesterol in adults (adult treatment panel III) final report. Circulation, 2002, 106, 3143–3421.
- A. Keys, J. T. Anderson and F. Grande, Prediction of serum-cholesterol responses of man to changes in fats in the diet, Lancet, 1957, 273, 959–966 CrossRef CAS.
-
The Lancet Commission, Food in the Anthropocene, The EAT- Lancet Commission on healthy diets from sustainable food systems, in The Lancet, London, 2019, https://www.thelancet.com/journals/lancet/article/PIIS0140-6736(18)31788-4/fulltext?utm_campaign=tleat19&utm_source=HPfeature Search PubMed.
- M. Springmann, M. Clark and D. Mason-D'Croz,
et al., Options for keeping the food system within environmental limits, Nature, 2018, 562, 519–525 CrossRef CAS PubMed.
- R. H. Eckel, J. M. Jakicic and J. D. Ard,
et al., 2013 AHA/ACC Guideline on Lifestyle Management to Reduce Cardiovascular Risk, J. Am. Coll. Cardiol., 2014, 63, 2960 CrossRef PubMed.
- L. Holm and M. Mohl, The role of meat in everyday food culture: an analysis of an interview study in Copenhagen, Appetite, 2000, 34, 277–283 CrossRef CAS PubMed.
- E. Lea and A. Worsley, Benefits and barriers to the consumption of a vegetarian diet in Australia, Public Health Nutr., 2003, 6, 505–511 CrossRef PubMed.
-
R. M. Santos and S. S. Martin, Hypercholesterolaemia, BMJ Best Practice, 2018, https://bestpractice.bmj.com/topics/en-gb/170 Search PubMed.
- L. M. Aston, J. N. Smith and J. W. Powles, Impact of a reduced red and processed meat dietary pattern on disease risks and greenhouse gas emissions in the UK: a modelling study, BMJ Open, 2012, 2, e001072 CrossRef PubMed.
-
R. Mensink, Effects of saturated fatty acids on serum lipids and lipoproteins: a systematic review and regression analysis, World Health Organization, Geneva, 2016 Search PubMed.
- S. L. Mumford, S. Dasharathy and A. Z. Pollack,
et al., Variations in lipid levels according to menstrual cycle phase: clinical implications, Clin. Lipidol., 2011, 6, 225–234 CrossRef CAS PubMed.
- M. A. Briggs, K. S. Petersen and P. M. Kris-Etherton, Saturated Fatty Acids and Cardiovascular Disease: Replacements for Saturated Fat to Reduce Cardiovascular Risk, Healthcare, 2017, 5(2), 29 CrossRef PubMed.
- M. S. Bosner, L. G. Lange and W. F. Stenson,
et al., Percent cholesterol absorption in normal women and men quantified with dual stable isotopic tracers and negative ion mass spectrometry, J. Lipid Res., 1999, 40, 302–308 CAS.
- X. Wang, F. Magkos and B. Mittendorfer, Sex Differences in Lipid and Lipoprotein Metabolism: It's Not Just about Sex Hormones, J. Clin. Endocrinol. Metab., 2011, 96, 885–893 CrossRef CAS PubMed.
- R. Obeid, J. Geisel and H. Schorr,
et al., The impact of vegetarianism on some haematological parameters, Eur. J. Haematol., 2002, 69, 275–279 CrossRef CAS PubMed.
- E. Neubauerova, J. Tulinska and M. Kuricova,
et al., The Effect of Vegetarian Diet on Immune Response, Epidemiology, 2007, 18, S196 CrossRef.
- A. M. Wells, M. D. Haub and J. Fluckey,
et al., Comparisons of vegetarian and beef-containing diets on hematological indexes and iron stores during a period of resistive training in older men, J. Am. Diet. Assoc., 2003, 103, 594–601 CrossRef PubMed.
- P. P. Basiotis, S. O. Welsh and F. J. Cronin,
et al., Number of days of food intake records required to estimate individual and group nutrient intakes with defined confidence, J. Nutr., 1987, 117, 1638–1641 CrossRef CAS PubMed.
- D. R. Matthews, J. P. Hosker and A. S. Rudenski,
et al., Homeostasis model assessment: insulin resistance and β-cell function from fasting plasma glucose and insulin concentrations in man, Diabetologia, 1985, 28, 412–419 CrossRef CAS PubMed.
|
This journal is © The Royal Society of Chemistry 2019 |