DOI:
10.1039/C8FO02438C
(Paper)
Food Funct., 2019,
10, 479-489
Lentinan modulates intestinal microbiota and enhances barrier integrity in a piglet model challenged with lipopolysaccharide†
Received
10th December 2018
, Accepted 7th January 2019
First published on 10th January 2019
Abstract
The intestinal microbiota plays a vital role in metabolism, pathogen resistance, and immune development in host cells, and is modifiable by dietary change. Lentinan (LNT), a type of mushroom polysaccharide, is known to ameliorate intestinal inflammation with the potential of therapeutic effect on digestive diseases. We hypothesized that LNT could alleviate Escherichia coli lipopolysaccharide (LPS)-induced intestinal injury via regulating the composition and metabolites of intestinal microbiota in a piglet model. Twenty-four weaned piglets were used in a 2 × 2 factorial design, and the main factors included a dietary treatment (basal or LNT diet) and immunological challenge (LPS or saline). After feeding basal or LNT diet for 21 days, pigs were injected with LPS or saline. At 4 h post-injection, pigs were killed and jejunum, ileum and cecal digesta were collected. LNT improved intestinal morphology and barrier function. LNT also inhibited inflammatory signaling pathways (toll-like receptor 4 and nucleotide binding oligomerization domain protein) and pro-inflammatory cytokines (tumor necrosis factor-α, interleukin-1β and interleukin-6) expression, as well as up-regulated the heat shock protein 70 expression in small intestine. In addition, LNT enhanced the concentrations of propionate, butyrate, isobutyrate and isovalerate in cecal digesta, resulting in a significant increase in histone acetylation without affecting the protein level of G protein-coupled receptor 41 (GPR41), a short chain fatty acid receptor. Bacterial 16S rRNA gene pyrosequencing showed that LNT had a great impact on gut microbiota composition at different taxonomic levels. Moreover, the correlation analysis revealed some potential relationships between cecal metabolites and certain intestinal microbiota. These results indicate that LNT promotes intestinal health, in part, through altering intestinal microbiota composition and increasing the short chain fatty acid synthesis, which subsequently lead to a reduction in inflammation and hyper-acetylation of histones.
Introduction
The mammalian gastrointestinal tract harbors a dynamic and complex community of bacteria, which constitutes the intestinal microbiota. The mutualistic symbiotic relationship between microbiota and the host is essential for the maintenance of intestinal homeostasis.1 These commensal bacteria participate in various physiological functions, e.g., digesting cellulose, strengthening gut integrity, shaping the intestinal epithelium, and improving the development and maturation of the intestinal and systemic immune system.2,3 Intestinal dysbiosis has been implicated in the pathogenesis of certain inflammatory diseases and infections.3
It has been confirmed that the effects of intestinal microbiota on host tissues are at least partly mediated by bacterial metabolites, especially short chain fatty acids (SCFAs),4 which serve a wide range of functions from immune regulation to metabolism.5 Numerous studies have demonstrated that SCFAs elicit their effects through either inhibition of histone deacetylases (HDACs) or activation of G protein-coupled receptors (GPRs).6,7
Diet has emerged as an important factor that regulates intestinal microbiota, immunity, and homeostasis. Polysaccharides not only can be utilized by intestinal microbiota but also contribute to improve the abundance and diversity of microbiota.8 Lentinan (LNT), a type of polysaccharide extracted from mushroom Lentinus edodes, is a β-(1,3)-glucan with β-(1,6) branches.9 This plant polysaccharide has no harm and places few side effects on the human body.10 LNT is currently being used as an immunomodulatory drug treating various diseases including digestive cancer11–13 and infectious diseases.14 In addition, LNT can ameliorate intestinal inflammation by reducing pro-inflammatory cytokines, inflammatory cell infiltration and the pathology of intestinal epithelia in colitis.15 However, the exact mechanism(s) underlying the protective role of LNT on the intestine remain to be explored.
We hypothesized that LNT would improve intestinal health by altering intestinal bacterial community and metabolites. In the present study, the pig was used as an animal model to study human gastrointestinal pathophysiology because of their similarities in intestinal anatomy, physiology, and microbial ecosystem.16 We also made use of a model of intestinal injury induced by Escherichia coli lipopolysaccharide (LPS),16 to evaluate whether LNT could ameliorate LPS-induced intestinal injury through modulation of intestinal microbiota and SCFAs, and subsequent regulation of HDACs and GPR signaling.
Materials and methods
Piglets, diets and experimental protocol
The experimental proposals and procedures were approved by the Animal Care and Use Committee of Wuhan Polytechnic University. All animal treatments and experiments were performed according to the recommendations of Laboratory animals-Guideline for ethical review of animal welfare in the national standards of the People's Republic of China (GB/T 35892-2018). The piglets were provided by Aodeng Agriculture and Animal Husbandry Technology Co., Ltd (Hubei, China). It was confirmed that the piglets had no enteric diseases and had not been inoculated with any Escherichia coli vaccines. A total of 24 weaned castrated barrows (Duroc × Large White × Landrace, weaned at 21 ± 1 d of age) were individually housed in 1.80 × 1.10 m pen in an environmentally controlled room and allowed ad libitum access to feed and water. Pigs were subjected to a 2 × 2 factorial design, with the main factors being diet [basal diet (control) or 0.02% commercial LNT product] and immunological challenge [LPS (Escherichia coli serotype O55:B5; purity >99%; #L2880; Sigma-Aldrich, St Louis, MO, USA) or sterile saline]. The basal diet (Table 1) was formulated to meet the nutrient requirements for pigs as recommended by the NRC standards.17 The commercial LNT product [containing LNT (50%), polyphenol, protein, amino acid, fat, fiber, etc.] used in this study was provided by Shaanxi Sciphar Natural Products (Shaanxi, China). The dose of LNT was chosen in accordance with Liu et al.18 All pigs were randomly allocated to either of the two diets till d 21, when half of the piglets in each dietary treatment were injected intraperitoneally with either Escherichia coli LPS at 100 mg per kg body weight16 or the same volume of saline.
Table 1 Ingredient composition of experimental diets (%, as-fed basis)
Ingredients |
|
Nutrients levelb |
|
Premix supplied per kg diet: retinyl acetate, 5512 IU; cholecalciferol, 2200 IU; DL-α-tocopheryl acetate, 30 IU; menadione sodium bisulfite complex, 4 mg; riboflavin, 5.22 mg; D-calcium–pantothenate, 20 mg; niacin, 26 mg; vitamin B12, 0.01 mg; Mn (MnSO4·H2O), 40 mg; Fe (FeSO4·H2O), 75 mg; Zn (ZnSO4·7H2O), 75 mg; Cu (CuSO4·5H2O), 100 mg; I (CaI2), 0.3 mg; Se (Na2SeO3), 0.3 mg.
The nutrients level was analyzed value except digestible energy, apparent total tract digestible phosphorus, standardized ileal digestible lysine, methionine, methionine + cystine, threonine and tryptophan which are calculated values.
|
Corn |
53.38 |
Digestible energy (MJ kg−1) |
14.82 |
Soybean meal, 44% crude protein |
14.55 |
Crude protein |
23.71 |
Fermented soybean meal |
15.00 |
Calcium |
0.80 |
Fish meal |
6.00 |
Total phosphorus |
0.63 |
Whey powder |
5.00 |
Apparent total tract digestible phosphorus |
0.36 |
Glucose |
2.00 |
Total lysine |
1.59 |
Soybean oil |
1.01 |
Standardized ileal digestible lysine |
1.35 |
Dicalcium phosphate |
0.37 |
Total methionine |
0.50 |
Limestone |
0.88 |
Standardized ileal digestible methionine |
0.44 |
Salt |
0.30 |
Total methionine + cystine |
0.86 |
L-Lysine HCl, 78% |
0.34 |
Standardized ileal digestible methionine + cystine |
0.72 |
L-Methionine, 98% |
0.09 |
Total threonine |
0.99 |
L-Threonine, 98% |
0.08 |
Standardized ileal digestible threonine |
0.79 |
Vitamin and mineral premixa |
1.00 |
Total tryptophan |
0.27 |
|
|
Standardized ileal digestible tryptophan |
0.22 |
Sample collection
At 4 h after administration with saline or LPS,16 the piglets were humanely euthanized with pentobarbital (80 mg per kg body weight). A 10 cm segment of the mid-jejunum and mid-ileum was collected to scrape mucosal epithelial layer.16 Another 3 cm segment of the mid-jejunum and mid-ileum was collected in 4% paraformaldehyde in PBS for subsequent histologic analysis. Approximately 0.5 ml of cecal digesta was also collected for 16S rRNA gene pyrosequencing and SCFA analysis. The mucosa and digesta samples were stored at −80 °C until analysis.
Assessment of intestinal morphology
After fixation for 24 h, the intestinal samples were dehydrated, embedded in paraffin, sectioned, and stained with haematoxylin and eosin.19 The method to measure the villus height, crypt depth and villus height/crypt depth ratio (VCR) in the jejunum and ileum was performed as previously described.19
Immunoblot
Protein immunoblot analysis was measured according to the procedures of Liu et al.16 Specific primary antibodies included rabbit anti-claudin-1 (1
:
1000, 23 kDa, #51-9000; Invitrogen, Carlsbad, CA, USA), mouse anti-heat shock protein 70 (HSP70; 1
:
1000, 70 kDa, #ADI-SPA-810; Enzo Life Sciences, Raamsdonksveer, The Netherlands), mouse anti-β-actin (1
:
1000, 43 kDa, #A2228; Sigma-Aldrich, St Louis, MO, USA), rabbit anti-acetyl-histone H3 (1
:
1000, 17 kDa, #27683; Cell Signaling Technology, Danvers, MA, USA), rabbit anti-total histone H3 (1
:
1000, 17 kDa, #9717; Cell Signaling Technology, Danvers, MA, USA), rabbit anti-GPR41 (1
:
1000, 39 kDa, #ab103718; Abcam, Cambridge, MA, USA), and rabbit anti-α-tubulin (1
:
1000, 52 kDa, #2125; Cell Signaling Technology, Danvers, MA, USA). The relative abundance of claudin-1, HSP70, acetyl-histone H3, and GPR41 were expressed as the ratio of claudin-1/β-actin, HSP70/β-actin, acetyl–histone H3/total histone H3 and GPR41/α-tubulin, respectively.
RT-qPCR analyses of target genes
Total RNA from the jejunal and ileal mucosa was prepared using the total RNA extraction reagent [#9108; TaKaRa Biotechnology (Dalian) Co., Ltd, Dalian, China] as recommended by the manufacturer. The first-strand cDNA was synthesized using PrimeScript® RT reagent kit with gDNA Eraser [#RR047A; TaKaRa Biotechnology (Dalian) Co., Ltd, Dalian, China] from 1 μg of total RNA. Quantitative analysis of PCR was carried out on a ABI 7500 Real-Time PCR System (Applied Biosystems, Life Technologies, Gaithersburg, MD, USA) using a SYBR® Premix Ex Taq™ (Tli RNaseH Plus) qPCR kit [#RR420A; TaKaRa Biotechnology (Dalian) Co., Ltd, Dalian, China]. Primer sequences for the target pig genes are shown in Table S1.† The relative fold difference in the mRNA expression levels was calculated using the 2−ΔΔCT method20 with GAPDH as the housekeeping gene. Our results showed that there was no difference in GAPDH expression among tissues and treatments. Relative mRNA expression of each target gene was normalized to the basal diet group treated with saline.
Concentrations of pro-inflammatory cytokines
The frozen intestinal mucosal samples were weighed, homogenized in ice-cold 0.9% NaCl solution with a 1
:
10 (wt/vol) ratio, and then centrifuged at 2500 rpm for 10 min at 4 °C to collect the supernatants. The protein concentration of the supernatant was measured by Bradford protein assay method.21 The tumor necrosis factor-α (TNF-α), interleukin (IL)-1β and IL-6 concentrations in intestinal mucosa supernatant were assayed using a commercially available porcine ELISA kit (#PTA00, #PLB00B, #P6000B; R&D Systems, Minneapolis, MN, USA) as recommended by the manufacturer. The results were expressed as pg mg protein−1.
SCFA analysis
The concentrations of SCFAs in cecal digesta were analyzed as previously described22 by using a HP 6890 Series Gas Chromatography (Hewlett Packard, Palo Alto, CA, USA) equipped with a HP 19091N-213 column with 30.0 m × 0.32 mm i.d. (Agilent, Santa Clara, CA, USA). The injector and detector temperatures were 185 and 210 °C, respectively.
Microbial diversity analysis
DNA extraction, PCR amplification, DNA quantification, and Illumina MiSeq sequencing in accordance with the standardized protocol of Shanghai Majorbio Bio-pharm Technology (Shanghai, China). In brief, microbial DNA was extracted from cecal digesta samples with FastDNA spin kit (#116560200; MP Biomedicals, Irvine, CA, USA). The V3–V4 regions of the bacteria 16S rRNA genes were amplified by PCR using 338F/806R barcoded primers. PCR amplification products were quantified with a QuantiFluor™-ST Handheld Fluorometer with UV/Blue Channels (Promega Corporation, Madison, WI, USA), normalized, and then sequenced on Miseq (Illumina Inc., San Diego, CA, USA). Operational taxonomic units (OTUs) were clustered with 97% similarity cutoff using Usearch (version 7.1; http://drive5.com/uparse/). The taxonomy of each 16S rRNA gene sequence was analyzed by RDP Classifier (version 2.2; http://sourceforge.net/projects/rdp-classifier/) against the Silva (Release128; http://www.arb-silva.de) 16S rRNA database using confidence threshold of 70%. Analyses for rarefaction curves, and calculation of the Good's coverage, ACE, Chao, Shannon and Simpson diversity indices were based on MOTHUR (version v.1.30.1; http://www.mothur.org/wiki/Schloss_SOP#Alpha_diversity).
Statistical analysis
Data were analyzed using the general linear model procedures of ANOVA (SAS Inst. Inc., Cary, NC, USA) with diet (control or LNT), challenge (saline or LPS) and their interactions as sources of variation. When a significant interaction or a trend for interaction was detected, data were further analyzed using one-way ANOVA with Duncan's multiple range tests. Differences at P < 0.05 were considered statistically significant, and trends were declared with 0.05 < P < 0.10. In addition, partial least squares discriminant analysis (PLS-DA) was performed using the R package “mixOmics” to compare bacterial composition between four groups. The associations between cecal SCFAs concentrations and microbiota (at the genera level) were assessed by Pearson's correlation test using the R package “Pheatmap”.
Results
Growth performance
Throughout the 21 d feeding trial (pre-challenge), there were no differences in initial and final body weight, average daily gain, average daily feed intake, and feed/gain ratio among the two groups (Table S2†).
Small intestinal morphology
Challenge with LPS caused fever, diarrhoea, anorexia, shivering and inactivity within 1 h in all piglets (data not shown). Compared with pigs injected with saline, pigs challenged with LPS had lower jejunal villus height (P < 0.05) and VCR (P < 0.001) (Fig. 1). There was a diet × LPS interaction observed for jejunal VCR, ileal villus height, and crypt depth (P < 0.05). In the absence of LPS challenge, LNT led to higher jejunal VCR, ileal villus height, and crypt depth.
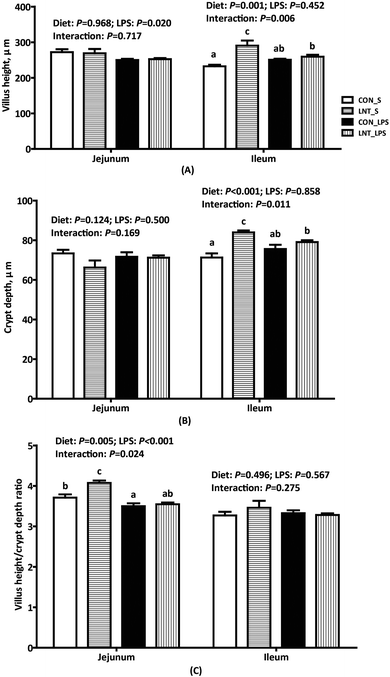 |
| Fig. 1 Effects of lentinan (LNT) on intestinal morphology of weaned pigs after 4 h lipopolysaccharide (LPS) challenge. Values are mean and SE, n = 6 (1 pig per pen). Means with different letters differ significantly (P < 0.05). CON_S, piglets fed the control diet and injected with saline; LNT_S, piglets fed the LNT diet and injected with saline; CON_LPS, piglets fed the control diet and challenged with LPS; LNT_LPS, piglets fed the LNT diet and challenged with LPS. | |
Claudin-1 protein expression in small intestine
There was no diet × LPS interaction observed for claudin-1 protein expression in jejunum and ileum (Fig. 2). Pigs challenged with LPS had lower ileal claudin-1 protein expression than that injected with saline (P < 0.05). Relative to pigs fed with control diet, pigs fed with LNT had higher claudin-1 protein expression in ileum (P < 0.05).
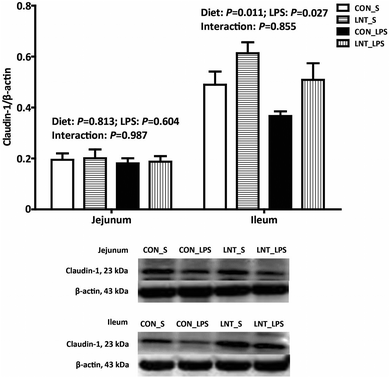 |
| Fig. 2 Effects of lentinan (LNT) supplementation on claudin-1 protein expression of weaned pigs after 4 h lipopolysaccharide (LPS) challenge. The bands were the representative western blot images. Values are mean and SE, n = 6 (1 pig per pen). CON_S, piglets fed the control diet and injected with saline; LNT_S, piglets fed the LNT diet and injected with saline; CON_LPS, piglets fed the control diet and challenged with LPS; LNT_LPS, piglets fed the LNT diet and challenged with LPS. | |
mRNA expression of toll-like receptor 4 (TLR4) and nucleotide binding oligomerization domain proteins (NODs) and their downstream signals in small intestine
There was no diet × LPS interaction observed for the mRNA expression of TLR4 and NODs and their downstream signals in jejunum and ileum (Table 2). LPS up-regulated the mRNA expression of jejunal cluster of differentiation 14 (CD14), NOD2, and ileal TLR4, lipolysaccharide binding protein (LBP), myeloid differential protein 2 (MD2), NOD2, receptor-interacting serine/threonine–protein kinase 2 (RIP2) (P < 0.05), and tended to increase jejunal RIP2 mRNA expression (P = 0.054) relative to saline. LNT down-regulated the mRNA expression of jejunal TLR4, LBP, MD2, myeloid differentiation factor 88 (MyD88), IL-1 receptor-associated kinase 1 (IRAK1), TNF receptor associated factor 6 (TRAF6), NOD1, RIP2, NF-κB, and ileal TRAF6 and NOD1 (P < 0.05), and tended to decrease ileal NOD2 mRNA expression (P = 0.057).
Table 2 Effects of lentinan (LNT) on mRNA expression of toll-like receptor 4 (TLR4) and nucleotide binding oligomerization domain protein (NOD) signaling, pro-inflammatory cytokines and heat shock protein 70 (HSP70) in small intestine of weaned pigs after 4 h lipopolysaccharide (LPS) challenge
Item |
Saline |
LPS |
SEM |
P value |
CON |
LNT |
CON |
LNT |
Diet |
LPS |
Interaction |
Values are mean and pooled SEM, n = 6 (1 pig per pen). Labeled means in a row without a common letter differ, P < 0.05. CD14, cluster of differentiation 14; IL, interleukin; IRAK1, IL-1 receptor-associated kinase 1; LBP, lipolysaccharide binding protein; MD2, myeloid differential protein 2; MyD88, myeloid differentiation factor 88; RIP2, receptor-interacting serine/threonine–protein kinase 2; TNF-α, tumor necrosis factor-α; TRAF6, TNF receptor associated factor 6. |
Jejunum
|
TLR4
|
1.00 |
0.60 |
1.15 |
0.71 |
0.13 |
0.004 |
0.320 |
0.883 |
LBP
|
1.00 |
0.77 |
1.03 |
0.45 |
0.17 |
0.030 |
0.406 |
0.322 |
CD14
|
1.00 |
0.79 |
1.53 |
1.40 |
0.17 |
0.331 |
0.003 |
0.810 |
MD2
|
1.00 |
0.84 |
1.07 |
0.88 |
0.08 |
0.032 |
0.496 |
0.785 |
MyD88
|
1.00 |
0.68 |
0.87 |
0.57 |
0.06 |
<0.001 |
0.071 |
0.861 |
IRAK1
|
1.00 |
0.85 |
1.02 |
0.65 |
0.09 |
0.009 |
0.304 |
0.225 |
TRAF6
|
1.00 |
0.79 |
0.99 |
0.71 |
0.08 |
0.007 |
0.547 |
0.670 |
NOD1
|
1.00 |
0.68 |
1.19 |
0.65 |
0.11 |
0.001 |
0.509 |
0.336 |
NOD2
|
1.00 |
0.74 |
3.33 |
1.66 |
0.76 |
0.219 |
0.045 |
0.367 |
RIP2
|
1.00 |
0.68 |
1.25 |
0.93 |
0.12 |
0.018 |
0.054 |
1.000 |
NF-κB
|
1.00 |
0.93 |
1.10 |
0.76 |
0.09 |
0.035 |
0.719 |
0.165 |
TNF-α
|
1.00 |
0.81 |
1.07 |
0.43 |
0.17 |
0.022 |
0.366 |
0.187 |
IL-1β
|
1.00 |
0.67 |
1.24 |
0.81 |
0.14 |
0.015 |
0.192 |
0.737 |
IL-6
|
1.00 |
0.61 |
1.18 |
1.21 |
0.10 |
0.032 |
0.002 |
0.144 |
HSP70
|
1.00ab |
0.52a |
4.20b |
13.88c |
1.17 |
0.001 |
<0.001 |
<0.001 |
Ileum
|
TLR4
|
1.00 |
0.86 |
1.51 |
1.45 |
0.13 |
0.463 |
0.001 |
0.757 |
LBP
|
1.00 |
0.89 |
1.98 |
1.61 |
0.40 |
0.564 |
0.048 |
0.749 |
CD14
|
1.00 |
1.01 |
1.00 |
1.27 |
0.15 |
0.368 |
0.417 |
0.401 |
MD2
|
1.00 |
0.99 |
1.16 |
1.28 |
0.11 |
0.603 |
0.047 |
0.550 |
MyD88
|
1.00 |
0.83 |
0.96 |
0.94 |
0.08 |
0.238 |
0.645 |
0.310 |
IRAK1
|
1.00 |
0.79 |
0.75 |
0.74 |
0.12 |
0.386 |
0.221 |
0.435 |
TRAF6
|
1.00 |
0.68 |
1.01 |
0.88 |
0.07 |
0.006 |
0.161 |
0.197 |
NOD1
|
1.00 |
0.62 |
0.90 |
0.69 |
0.14 |
0.042 |
0.928 |
0.544 |
NOD2
|
1.00 |
0.68 |
1.24 |
1.15 |
0.10 |
0.057 |
0.002 |
0.262 |
RIP2
|
1.00 |
0.70 |
1.37 |
1.34 |
0.11 |
0.139 |
<0.001 |
0.224 |
NF-κB
|
1.00 |
0.90 |
0.96 |
0.93 |
0.08 |
0.416 |
0.960 |
0.671 |
TNF-α
|
1.00 |
0.72 |
0.85 |
0.63 |
0.09 |
0.012 |
0.199 |
0.709 |
IL-1β
|
1.00 |
0.55 |
0.98 |
0.73 |
0.17 |
0.010 |
0.532 |
0.419 |
IL-6
|
1.00b |
0.64a |
0.58a |
0.79ab |
0.12 |
0.506 |
0.243 |
0.020 |
HSP70
|
1.00a |
0.80a |
14.28b |
30.52c |
3.44 |
0.030 |
<0.001 |
0.027 |
Pro-inflammatory cytokines mRNA expression and concentrations in small intestine
LPS significantly increased jejunal IL-6 mRNA expression and concentration, ileal TNF-α, IL-1β, and IL-6 concentrations (Tables 2 and 3, P < 0.05). There was no diet × LPS interaction observed for mRNA expression and concentrations of TNF-α and IL-1β in the jejunum and ileum, and jejunal IL-6 mRNA expression and concentration. LNT suppressed jejunal IL-1β and IL-6 mRNA expression and the mRNA expression and concentration of TNF-α, and ileal TNF-α and IL-1β mRNA expression and concentrations (P < 0.05). There was a diet × LPS interaction observed for IL-6 mRNA expression (P < 0.05), and a trend for a diet × LPS interaction was observed for IL-6 concentration (P = 0.061) in ileum. Pigs fed with LNT had lower IL-6 mRNA expression in ileum relative to pigs fed with control diet among saline-treated pigs, and had lower ileal IL-6 concentration relative to pigs fed with control diet among LPS-challenged pigs.
Table 3 Effects of lentinan (LNT) on concentrations of pro-inflammatory cytokines in small intestine of weaned pigs after 4 h lipopolysaccharide (LPS) challenge
Item |
Saline |
LPS |
SEM |
P value |
CON |
LNT |
CON |
LNT |
Diet |
LPS |
Interaction |
Values are mean and pooled SEM, n = 6 (1 pig per pen). Labeled means in a row without a common letter differ, P < 0.05. ND, not detectable, low than the minimum detectable dose of TNF-α. IL, interleukin; TNF-α, tumor necrosis factor-α. |
Jejunum
|
TNF-α (pg mg protein−1) |
12 |
5 |
46 |
ND |
14 |
0.047 |
0.374 |
0.125 |
IL-1β (pg mg protein−1) |
71 |
77 |
75 |
88 |
8 |
0.271 |
0.376 |
0.644 |
IL-6 (pg mg protein−1) |
64 |
105 |
129 |
123 |
17 |
0.315 |
0.025 |
0.181 |
Ileum
|
TNF-α (pg mg protein−1) |
22 |
17 |
30 |
18 |
2 |
0.002 |
0.049 |
0.221 |
IL-1β (pg mg protein−1) |
92 |
69 |
113 |
105 |
7 |
0.043 |
0.001 |
0.321 |
IL-6 (pg mg protein−1) |
35a |
32a |
69b |
42a |
6 |
0.023 |
0.002 |
0.061 |
HSP70 mRNA and protein expression in small intestine
The pigs challenged with LPS had higher mRNA and protein expression of HSP70 in the jejunum and ileum compared with pigs injected with saline (Fig. 3 and Table 2, P < 0.001). No diet × LPS interaction was observed for protein expression of HSP70 in jejunum and ileum. The pigs fed with LNT had higher protein expression of HSP70 in ileum (P < 0.05). There was a diet × LPS interaction observed for mRNA expression of HSP70 in the jejunum and ileum (P < 0.05). Pigs fed with LNT had higher HSP70 mRNA expression in the jejunum and ileum relative to pigs fed with the control diet among LPS-challenged pigs, whereas there was no difference in saline injected pigs.
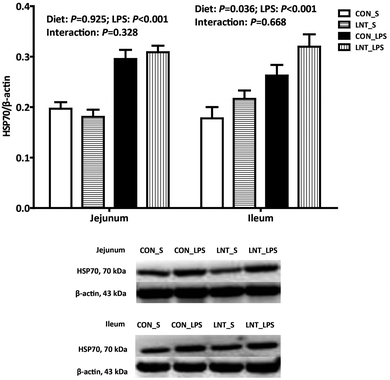 |
| Fig. 3 Effects of lentinan (LNT) supplementation on heat shock protein 70 (HSP70) protein expression of weaned pigs after 4 h lipopolysaccharide (LPS) challenge. The bands were the representative western blot images. Values are mean and SE, n = 6 (1 pig per pen). CON_S, piglets fed the control diet and injected with saline; LNT_S, piglets fed the LNT diet and injected with saline; CON_LPS, piglets fed the control diet and challenged with LPS; LNT_LPS, piglets fed the LNT diet and challenged with LPS. | |
SCFAs concentrations in cecal digesta
There was no diet × LPS interaction observed for SCFAs in cecal digesta (Table 4). The pigs fed with LNT had higher concentrations of propionate, butyrate, isobutyrate, and isovalerate in cecal digesta (P < 0.05).
Table 4 Effects of lentinan (LNT) on concentrations of short chain fatty acids in cecal digesta of weaned pigs after 4 h lipopolysaccharide (LPS) challenge
Item |
Saline |
LPS |
SEM |
P value |
CON |
LNT |
CON |
LNT |
Diet |
LPS |
Interaction |
Values are mean and pooled SEM, n = 6 (1 pig per pen). |
Acetate, mg g−1 |
2.08 |
2.25 |
2.01 |
2.04 |
0.31 |
0.750 |
0.651 |
0.804 |
Propionate, mg g−1 |
1.07 |
1.24 |
1.01 |
1.36 |
0.11 |
0.030 |
0.757 |
0.428 |
Butyrate, mg g−1 |
0.407 |
0.501 |
0.381 |
0.586 |
0.061 |
0.024 |
0.640 |
0.376 |
Isobutyrate, mg g−1 |
0.135 |
0.153 |
0.125 |
0.141 |
0.008 |
0.043 |
0.179 |
0.887 |
Valerate, mg g−1 |
0.120 |
0.112 |
0.113 |
0.113 |
0.024 |
0.860 |
0.887 |
0.876 |
Isovalerate, mg g−1 |
0.219 |
0.270 |
0.226 |
0.260 |
0.017 |
0.019 |
0.942 |
0.587 |
Protein expression of acetyl–histone H3 and GPR41 in small intestine
No diet × LPS interaction was observed for protein expression of acetyl–histone H3 and GPR41 in the jejunum and ileum (Fig. 4). LPS reduced protein expression of ileal acetyl–histone H3 relative to saline, while LNT significantly increased acetyl–histone H3 in the jejunum and ileum (P < 0.05). Neither LPS nor diet had an effect on the protein expression of GPR41.
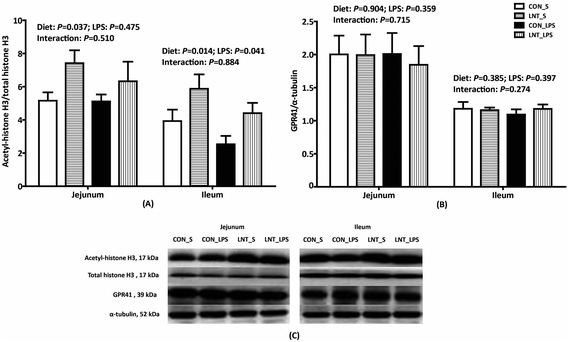 |
| Fig. 4 Effects of lentinan (LNT) supplementation on protein expression of acetyl-histone H3 (A) and G protein-coupled receptor 41 (GPR41) (B) of weaned pigs after 4 h lipopolysaccharide (LPS) challenge. The bands were the representative western blot images (C). Values are mean and SE, n = 6 (1 pig per pen). CON_S, piglets fed the control diet and injected with saline; LNT_S, piglets fed the LNT diet and injected with saline; CON_LPS, piglets fed the control diet and challenged with LPS; LNT_LPS, piglets fed the LNT diet and challenged with LPS. | |
Bacterial composition in cecal digesta
In total, 907969 high-quality sequence reads were obtained from the 24 samples. The Good's coverage of each group was over 99%, and the rarefaction curves showed clear asympyoyes (Fig. S1†), which together indicate a near-complete sampling of the community. The community richness estimators (ACE and Chao) and the diversity estimators (Shannon and Simpson) were shown in the Table S3.† There were no significant differences in any of these estimators among four groups. PLS-DA revealed a distinct clustering of microbiota composition for each treatment (Fig. 5).
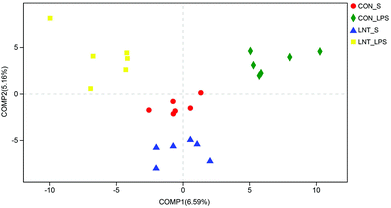 |
| Fig. 5 Partial least squares discriminant analysis (PLS-DA) at the OTU level. CON_S, piglets fed the control diet and injected with saline; LNT_S, piglets fed the lentinan diet and injected with saline; CON_LPS, piglets fed the control diet and challenged with lipopolysaccharide; LNT_LPS, piglets fed the lentinan diet and challenged with lipopolysaccharide. | |
Bacterial sequences from the four groups were analyzed at the phylum and genus levels (Fig. 6 and Table S4†). At the phylum level, no diet × LPS interaction was observed for the abundance of Bacteroidetes and Firmicutes. LPS reduced the Bacteroidetes, but increased Firmicutes (P < 0.05). Pigs receiving the LNT diet had higher Bacteroidetes abundance and lower Firmicutes abundance compared to the pigs receiving the control diet (P < 0.05). Furthermore, top 50 genus of relative abundance were selected for comparison. The pigs challenged with LPS had lower Faecalibacterium and higher Intestinibacter, Oscillospira and Phascolarctobacterium (P < 0.05), and tended to have lower Lachnospiraceae_UCG-007 (P = 0.073) and Lactobacillus (P = 0.067). No diet × LPS interaction was observed for the abundance of Actinobacillus, Coprococcus_3, Faecalibacterium, Intestinibacter, Lachnospiraceae_UCG-007, Lactobacillus, Oscillospira, Phascolarctobacterium, Prevotella_9, Solobacterium, and norank_f__Ruminococcaceae. The pigs fed with LNT had lower Actinobacillus, Coprococcus_3, Oscillospira and Phascolarctobacterium, and higher Faecalibacterium, Prevotella_9 and norank_f__Ruminococcaceae (P ≤ 0.05), and tended to have higher Lachnospiraceae_UCG-007 (P = 0.064) and lower Solobacterium (P = 0.059). There was a diet × LPS interaction observed for Sutterella and Prevotella_1 (P < 0.05). Pigs fed with LNT had lower Sutterella abundance relative to pigs fed with the control diet among saline injected pigs.
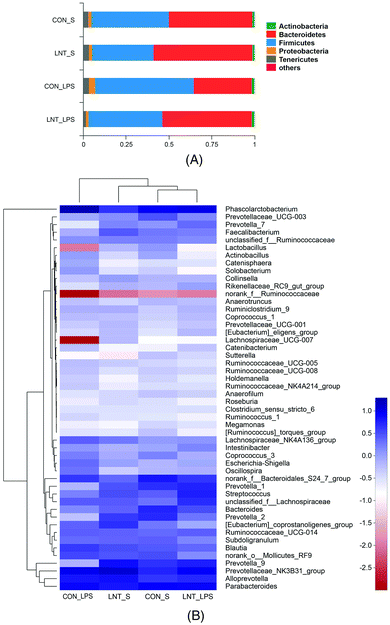 |
| Fig. 6 Relative abundance of bacterial composition at the phylum (A) and genus (B) level. CON_S, piglets fed the control diet and injected with saline; LNT_S, piglets fed the lentinan diet and injected with saline; CON_LPS, piglets fed the control diet and challenged with lipopolysaccharide; LNT_LPS, piglets fed the lentinan diet and challenged with lipopolysaccharide. | |
Association of microbial metabolites concentration and microbiota in cecal digesta
The relationship between SCFAs concentrations and microbiota (at the genera level) was shown in Fig. 7. Acetate concentration was positively correlated with Lachnospiraceae_NK4A136_group (P < 0.05), Prevotella_1 (P < 0.05), Prevotella_7 (P < 0.05), Prevotella_9 (P < 0.05), and Prevotellaceae_UCG-003 (P < 0.01). Propionate concentration was positively correlated with Prevotella_9 (P < 0.05). Butyrate concentration was positively correlated with Prevotella_9 (P < 0.01), Rikenellaceae_RC9_gut_group (P < 0.05), and unclassified_f__Lachnospiraceae (P < 0.05). Isobutyrate concentration was positively correlated with Faecalibacterium (P < 0.05) and Prevotella_9 (P < 0.05). Valerate concentration was negatively correlated with Escherichia-Shigella, Holdemanella, Ruminococcaceae_UCG-008, and [Ruminococcus]_torques_group (P < 0.05). Isovalerate concentration was positively correlated with Prevotella_9 and unclassified_f__Ruminococcaceae (P < 0.05).
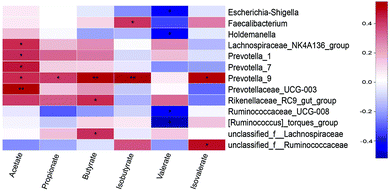 |
| Fig. 7 Heatmap of correlation between microbial metabolites concentration and microbiota (at the genera level) in the cecal digesta of pigs. Only taxa nominally associated with either of the six traits are depicted. The red represents a positive correlation, while the blue represents a negative correlation. *P < 0.05, **P < 0.01. | |
Discussion
The intraperitoneal injection of LPS is widely used as an experimental model in the study of endotoxin-induced acute intestinal injury.16,23 LPS irritates the intestine, causing mucosal injury, metabolic disorder, and bacterial translocation, as well as strong intestinal inflammaotry response.16,23 The effects of LPS are associated with the binding of TLR4/MD2/CD14 receptor complex, resulting in the over-production of pro-inflammatory cytokines.16 Thus, nutritional interventions targeting the regulation of inflammation may exert protective effects that attenuates intestinal injury. Polysaccharide, a kind of natural macromolecule polymer, is difficult to be directly absorbed through the intestine, but can be utilized by gut microbiota.24 Studies have demonstrated that polysaccharides intake can enrich intestinal health-promoting bacteria and metabolite.25 Diverse biological activities of polysaccharides such as anti-tumor, anti-virus, anti-oxidation, immune regulation and wound-healing have also been reported.25–27 To our best knowledge, this is the first study investigating the role of LNT (a type of polysaccharide) in promoting intestinal health in piglets challenged with LPS at the cellular and molecular levels.
Intestinal morphology is one of essential indicators to reflect the health status of gut.16 The present study showed that LPS challenge decreased villus height and VCR, indicating that LPS caused acute intestinal injury in piglets. These adverse effects of LPS were attenuated by LNT supplementation, which is in agreement with an earlier finding that LNT increased jejunal VCR in piglets.28 We further confirmed that claudin-1, a major tight junction protein, is enhanced by LNT, which demonstrates that dietary LNT helps improve intestinal integrity and barrier function, and alleviate tissue injury.
We hypothesized that LNT improve intestinal integrity and epithelial function via attenuating inflammatory response. TLR4, NODs and their downstream signaling molecules are key members of inflammatory signaling pathways.16 TLR4 is best known for recognizing LPS and lead NF-κB nuclear translocation via the signaling pathway containing LBP, CD14, MD2, MyD88, IRAK1, and TRAF6.16 NOD1 and NOD2 could self-oligomerize to recruit and activate the adaptor molecule RIP2, which is critical for NF-κB activation.16 The up-regulated activity of NF-κB may increase the expression of pro-inflammatory cytokines.16 Overproduction of the cytokines can exacerbate the inflammatory response and disturb intestinal barrier, ultimately result in diseases.16 We did show that higher mRNA expressions of TLR4 and NOD1/2 signaling and pro-inflammatory cytokines in small intestine challenged with LPS in weaned piglets, which were associated with the breakdown of intestinal integrity and epithelial function. Adding LNT in the diet alleviated the impact of LPS. Consistently, LNT was found to suppress IL-6 production in macrophages,11 reduce IL-8 mRNA expression in intestinal epithelial cells,15 and decrease serum IL-1β and TNF-α levels in a progressive inflammatory disorder.29
Similarly, HSP70, an anti-inflammatory factor, is basally expressed under normal state in intestine, but highly induced in inflammation state.16 In the current study, pigs challenged with LPS had higher expression of HSP70. Interestingly, after LPS challenge, the high mRNA and protein expression of HSP70 were found in pigs given the LNT diet, which is in consistent with a recent study, which suggests that high level of intracellular HSP70 can reduce the inflammatory response.30 It is possible that, LNT may exhibit anti-inflammatory activity through regulation of both pro-inflammatory cytokines and HSP70 expression.
SCFAs, the saturated aliphatic organic acids that are comprised of one to six carbons, are produced by fermentation of polysaccharides by anaerobic intestinal microbiota.8 SCFAs are transported from the intestinal lumen into the blood compartment of the host and are utilized by organs where they play roles as substrates or signal molecules.31 Growing evidence has demonstrated that SCFAs suppress the generation of pro-inflammatory cytokines and could potentially function as therapeutic agents to prevent various inflammatory diseases.7 SCFAs also play a crucial role as energy sources for intestinal epithelial cells and certain bacterial species, and thus improve gut function.32 In this study, dietary LNT supplementation increased the concentrations of propionate, butyrate, isobutyrate, and isovalerate in the cecal digesta, which may help contribute to the improvement of intestinal integrity and the decrease of pro-inflammatory cytokines expression. Consistently, Tedelind et al. reported that acetate, propionate, and butyrate reduced LPS-induced TNF-α release from human blood-derived neutrophils, and also inhibited TNF-α-mediated activation of the NF-κB pathway in a human colon adenocarcinoma cell line.33
SCFAs are well-known to exert anti-inflammatory effects and suppress the expression of pro-inflammatory cytokines through inhibiting HDACs or activating GPRs.6,7 Our data showed that LNT increased histone H3 acetylation (reflecting inhibition of HDAC), which is likely due to increased SCFA production. Another study indicated that propionate and butyrate could inhibit the expression of pro-inflammatory mediators and HDAC activity.34 Covalent modification of histones by acetylation is important in gene expression under physiological and pathological conditions, that influences inflammatory and host defence responses.35 Histone acetylation is controlled by histone acetyltransferases and HDACs.35 It was demonstrated that HDAC3 activation promotes inflammatory gene expression in macrophages36 and cardiomyocytes37 when cells were treated with LPS. Therefore, inhibition of HDACs activities exerts anti-inflammatory effects. On the other hand, contrary to our hypothesis, LNT had no effect on the protein expression of GPR41. Andrade-Oliveira et al. reported that SCFAs suppressed inflammatory process via inhibiting HDAC activity in a GPR-independent manner.6 However, the exact mechanism with regard to how GPR41 functions in LNT related pathway needs more investigation.
Intestinal microbiota have a recognized role in immunity, and is critical for host's intestinal function and health.38 Studies in both humans and animals have found differences in microbiota composition between disease and healthy subjects.39 These differences are mainly detected at the phylum level, especially Firmicutes and Bacteroidetes.39 Thus, we speculated that LNT might affect the microbiota composition in cecum. We performed 16S rRNA gene pyrosequencing of the cecal digesta of various diet treatments and found that more Firmicutes and fewer Bacteroidetes in cecal digesta of LPS-induced pigs, whereas LNT had the reverse effects. In agreement, Ren et al. reported that oral supplementations of LNT decreased the relative abundance of Firmicutes and increased that of Bacteroidetes.29 As a main contributor of SCFAs, Bacteroidetes promote the balance of intestinal microbiota, and play an important role in many major metabolic activities involving fermentation of carbohydrates, utilization of nitrogenous substances and prevention of pathogen colonization.40,41 Higher abundance of Bacteroidetes in the cecum suggested that LNT diet might change bacterial fermentation.
LPS or LNT also alter bacterial composition at genus level. We found that LPS decreased Faecalibacterium abundance, while LNT increased it. Faecalibacterium is a butyrate producing bacterial group,42 which is in consistent with the result of correlation analysis. The major representative of the Faecalibacterium, Faecalibacterium prausnitzii, is an important component of a healthy human gut microbiome.43 Faecalibacterium prausnitzii is also an anti-inflammatory commensal bacterium that blocks NF-κB activation and pro-inflammatory mediator secretion.44 In addition, LNT up-regulated Prevotella abundance, which is in agreement with the findings by Ren et al.29 Prevotella belongs to the family Prevotellaceae and phylum Bacteroidetes. Prevotellaceae represents an important bacterial group for polysaccharide degradation and the formation of SCFAs.45 The correlation analysis showed that Prevotella_9 revealed a significant positive correlation with acetate, propionate, butyrate, isobutyrate, and isovalerate concentration. Prevotella is one of the predominant dietary fiber fermenters in gut of pigs.46 Thus, LNT supplementation may play an important role in maintaining gut health by modulating gut microbiota composition at different taxonomic levels.
In summary, LNT plays beneficial roles in the improvement of intestinal integrity and intestinal injury. The protective effects of LNT may be associated with inhibition of inflammation, production of SCFAs, and alterations of microbiota composition.
Conflicts of interest
There are no conflicts to declare.
Acknowledgements
This study was supported by the projects of the National Natural Science Foundation of China (31772615), Wuhan Science and Technology Bureau (2018020401011304) and Hubei Provincial Department of Education (T201508).
References
- X. Song, D. Dai, X. He, S. Zhu, Y. Yao, H. Gao, J. Wang, F. Qu, J. Qiu, H. Wang, X. Li, N. Shen and Y. Qian, Growth factor FGF2 cooperates with interleukin-17 to repair intestinal epithelial damage, Immunity, 2015, 43, 488–501 CrossRef CAS PubMed.
- M. J. Saad, A. Santos and P. O. Prada, Linking gut microbiota and inflammation to obesity and insulin resistance, Physiology, 2016, 31, 283–293 CrossRef CAS PubMed.
- E. Thursby and N. Juge, Introduction to the human gut microbiota, Biochem. J., 2017, 474, 1823–1836 CrossRef CAS PubMed.
- Z. Ang and J. L. Ding, GPR41 and GPR43 in obesity and inflammation - protective or causative?, Front. Immunol., 2016, 7, 28 Search PubMed.
- H. Ohira, W. Tsutsui and Y. Fujioka, Are short chain fatty acids in gut microbiota defensive players for inflammation and atherosclerosis?, J. Atheroscler. Thromb., 2017, 24, 660–672 CrossRef CAS PubMed.
- V. Andrade-Oliveira, M. T. Amano, M. Correa-Costa, A. Castoldi, R. J. Felizardo, D. C. de Almeida, E. J. Bassi, P. M. Moraes-Vieira, M. I. Hiyane, A. C. Rodas, J. P. Peron, C. F. Aguiar, M. A. Reis, W. R. Ribeiro, C. J. Valduga, R. Curi, M. A. Vinolo, C. M. Ferreira and N. O. Câmara, Gut bacteria products prevent AKI induced by ischemia-reperfusion, J. Am. Soc. Nephrol., 2015, 26, 1877–1888 CrossRef CAS PubMed.
- M. Kobayashi, D. Mikami, H. Kimura, K. Kamiyama, Y. Morikawa, S. Yokoi, K. Kasuno, N. Takahashi, T. Taniguchi and M. Iwano, Short-chain fatty acids, GPR41 and GPR43 ligands, inhibit TNF-α-induced MCP-1 expression by modulating p38 and JNK signaling pathways in human renal cortical epithelial cells, Biochem. Biophys. Res. Commun., 2017, 486, 499–505 CrossRef CAS PubMed.
- X. Xu, P. Xu, C. Ma, J. Tang and X. Zhang, Gut microbiota, host health, and polysaccharides, Biotechnol. Adv., 2013, 31, 318–337 CrossRef CAS PubMed.
- Y. Zhang, H. Mei, W. Shan, L. Shi, X. Chang, Y. Zhu, F. Chen and X. Han, Lentinan protects pancreatic β cells from STZ-induced damage, J. Cell. Mol. Med., 2016, 20, 1803–1812 CrossRef CAS PubMed.
- Y. Wang, X. Han, Y. D. Li, S. Y. Zhao, D. J. Zhang, Z. H. Zhao and Y. B. Wang, Effects of tumor-specific antigen induced by lentinan on murine H22 hepatocellular carcinoma immunoprophylaxis, Eur. Rev. Med. Pharmacol. Sci., 2015, 19, 4516–4524 CAS.
- S. Hazama, S. Watanabe, M. Ohashi, M. Yagi, M. Suzuki, K. Matsuda, T. Yamamoto, Y. Suga, T. Suga, S. Nakazawa and M. Oka, Efficacy of orally administered superfine dispersed lentinan (beta-1,3-glucan) for the treatment of advanced colorectal cancer, Anticancer Res., 2009, 29, 2611–2617 CAS.
- J. L. Wang, Z. Bi, J. W. Zou and X. M. Gu, Combination therapy with lentinan improves outcomes in patients with esophageal carcinoma, Mol. Med. Rep., 2012, 5, 745–748 CAS.
- K. Ina, T. Kataoka and T. Ando, The use of lentinan for treating gastric cancer, Anticancer Agents Med. Chem., 2013, 13, 681–688 CrossRef CAS PubMed.
- S. X. Wang, Q. Y. Liu and Y. Li, Lentinan ameliorates burn sepsis by attenuating CD4+ CD25+ Tregs, Burns, 2016, 42, 1513–1521 CrossRef PubMed.
- Y. Nishitani, L. Zhang, M. Yoshida, T. Azuma, K. Kanazawa, T. Hashimoto and M. Mizuno, Intestinal anti-inflammatory activity of lentinan: influence on IL-8 and TNFR1 expression in intestinal epithelial cells, PLoS One, 2013, 8, e62441 CrossRef CAS PubMed.
- Y. Liu, F. Chen, J. Odle, X. Lin, S. K. Jacobi, H. Zhu, Z. Wu and Y. Hou, Fish oil enhances intestinal integrity and inhibits TLR4 and NOD2 signaling pathways in weaned pigs after LPS challenge, J. Nutr., 2012, 142, 2017–2024 CrossRef CAS PubMed.
-
NRC, Nutrient requirements of swine, National Academies Press, Washington, DC, 11th revised edn, 2012 Search PubMed.
- D. K. Liu, H. J. Zhu, H. F. Dong, L. H. Wang, Y. Y. Zhao, J. J. Zhao and G. L. Shi, Effect of lentinus edodes polysaccharide on the growth of weaned piglets, Tianjin Sci. Technol., 2014, 41, 92–93 Search PubMed.
- H. L. Zhu, Y. L. Liu, X. L. Xie, J. J. Huang and Y. Q. Hou, Effect of L-arginine on intestinal mucosal immune barrier function in weaned pigs after Escherichia coli LPS challenge, Innate Immun., 2013, 19, 242–252 CrossRef CAS PubMed.
- K. J. Livak and T. D. Schmittgen, Analysis of relative gene expression data using real-time quantitative PCR and 2−ΔΔCT method, Methods, 2001, 25, 402–408 CrossRef CAS PubMed.
- M. M. Bradford, A rapid and sensitive method for the quantitation of microgram quantities of protein utilizing the principle of protein-dye binding, Anal. Biochem., 1976, 72, 248–254 CrossRef CAS PubMed.
- M. Han, P. Song, C. Huang, A. Rezaei, S. Farrar, M. A. Brown and X. Ma, Dietary grape seed proanthocyanidins (GSPs) improve weaned intestinal microbiota and mucosal barrier using a piglet model, Oncotarget, 2016, 7, 80313–80326 Search PubMed.
- X. Wang, Y. Liu, S. Li, D. Pi, H. Zhu, Y. Hou, H. Shi and W. Leng, Asparagine attenuates intestinal injury, improves energy status, and inhibits AMPK signaling pathways in weaned piglets challenged with Escherichia coli lipopolysaccharide, Br. J. Nutr., 2015, 114, 553–565 CrossRef CAS PubMed.
- J. Zhang, G. Yang, Y. Wen, S. Liu, C. Li, R. Yang and W. Li, Intestinal microbiota are involved in the immunomodulatory activities of longan polysaccharide, Mol. Nutr. Food Res., 2017, 61, 1700466 CrossRef PubMed.
- L. Liu, M. Li, M. Yu, M. Shen, Q. Wang, Y. Yu and J. Xie, Natural polysaccharides exhibit anti-tumor activity by targeting gut microbiota, Int. J. Biol. Macromol., 2019, 121, 743–751 CrossRef CAS PubMed.
- F. Chen and G. Huang, Preparation and immunological activity of polysaccharides and their derivatives, Int. J. Biol. Macromol., 2018, 112, 211–216 CrossRef CAS PubMed.
- I. A. Schepetkin and M. T. Quinn, Botanical polysaccharides: macrophage immunomodulation and therapeutic potential, Int. Immunopharmacol., 2006, 6, 317–333 CrossRef CAS PubMed.
- C. J. van Nevel, J. A. Decuypere, N. Dierick and K. Molly, The influence of Lentinus edodes (Shiitake mushroom) preparations on bacteriological and morphological aspects of the small intestine in piglets, Arch. Tierernahr., 2003, 57, 399–412 CAS.
- G. Ren, M. Yu, K. Li, Y. Hu, Y. Wang, X. Xu and J. Qu, Seleno-lentinan prevents chronic pancreatitis development and modulates gut microbiota in mice, J. Funct. Foods, 2016, 22, 177–188 CrossRef CAS.
- H. Wu, Y. Liu, D. Pi, W. Leng, H. Zhu, Y. Hou, S. Li, H. Shi and X. Wang, Asparagine attenuates hepatic injury caused by lipopolysaccharide in weaned piglets associated with modulation of toll-like receptor 4 and nucleotide-binding oligomerisation domain protein signalling and their negative regulators, Br. J. Nutr., 2015, 114, 189–201 CrossRef CAS PubMed.
- G. den Besten, K. van Eunen, A. K. Groen, K. Venema, D. J. Reijngoud and B. M. Bakker, The role of short-chain fatty acids in the interplay between diet, gut microbiota, and host energy metabolism, J. Lipid Res., 2013, 54, 2325–2340 CrossRef CAS PubMed.
- A. Trompette, E. S. Gollwitzer, K. Yadava, A. K. Sichelstiel, N. Sprenger, C. Ngom-Bru, C. Blanchard, T. Junt, L. P. Nicod, N. L. Harris and B. J. Marsland, Gut microbiota metabolism of dietary fiber influences allergic airway disease and hematopoiesis, Nat. Med., 2014, 20, 159–166 CrossRef CAS PubMed.
- S. Tedelind, F. Westberg, M. Kjerrulf and A. Vidal, Anti-inflammatory properties of the short-chain fatty acids acetate and propionate: a study with relevance to inflammatory bowel disease, World J. Gastroenterol., 2007, 13, 2826–2832 CrossRef CAS PubMed.
- M. A. Vinolo, H. G. Rodrigues, R. T. Nachbar and R. Curi, Regulation of inflammation by short chain fatty acids, Nutrients, 2011, 3, 858–876 CrossRef CAS PubMed.
- E. Ciarlo, A. Savva and T. Roger, Epigenetics in sepsis: targeting histone deacetylases, Int. J. Antimicrob. Agents, 2013, 42(Suppl), S8–S12 CrossRef CAS PubMed.
- X. Chen, I. Barozzi, A. Termanini, E. Prosperini, A. Recchiuti, J. Dalli, F. Mietton, G. Matteoli, S. Hiebert and G. Natoli, Requirement for the histone deacetylase Hdac3 for the inflammatory gene expression program in macrophages, Proc. Natl. Acad. Sci. U. S. A., 2012, 109, E2865–E2874 CrossRef CAS PubMed.
- H. Zhu, L. Shan, P. W. Schiller, A. Mai and T. Peng, Histone deacetylase-3 activation promotes tumor necrosis factor-alpha (TNF-alpha) expression in cardiomyocytes during lipopolysaccharide stimulation, J. Biol. Chem., 2010, 285, 9429–9436 CrossRef CAS PubMed.
- W. Ren, S. Chen, J. Yin, J. Duan, T. Li, G. Liu, Z. Feng, B. Tan, Y. Yin and G. Wu, Dietary arginine supplementation of mice alters the microbial population and activates intestinal innate immunity, J. Nutr., 2014, 144, 988–995 CrossRef CAS PubMed.
- L. Bervoets, K. Van Hoorenbeeck, I. Kortleven, C. Van Noten, N. Hens, C. Vael, H. Goossens, K. N. Desager and V. Vankerckhoven, Differences in gut microbiota composition between obese and lean children: a cross-sectional study, Gut Pathog., 2013, 5, 10 CrossRef PubMed.
- P. Xu, M. Li, J. Zhang and T. Zhang, Correlation of intestinal microbiota with overweight and obesity in Kazakh school children, BMC Microbiol., 2012, 12, 283 CrossRef PubMed.
- C. Zhang, M. Yu, Y. Yang, C. Mu, Y. Su and W. Zhu, Effect of early antibiotic administration on cecal bacterial communities and their metabolic profiles in pigs fed diets with different protein levels, Anaerobe, 2016, 42, 188–196 CrossRef CAS PubMed.
- S. H. Duncan, G. L. Hold, H. J. Harmsen, C. S. Stewart and H. J. Flint, Growth requirements and fermentation products of Fusobacterium prausnitzii, and a proposal to reclassify it as Faecalibacterium prausnitzii gen. nov., comb. nov., Int. J. Syst. Evol. Microbiol., 2002, 52, 2141–2146 CAS.
- R. Tang, Y. Wei, Y. Li, W. Chen, H. Chen, Q. Wang, F. Yang, Q. Miao, X. Xiao, H. Zhang, M. Lian, X. Jiang, J. Zhang, Q. Cao, Z. Fan, M. Wu, D. Qiu, J. Y. Fang, A. Ansari, M. E. Gershwin and X. Ma, Gut microbial profile is altered in primary biliary cholangitis and partially restored after UDCA therapy, Gut, 2018, 67, 534–541 CrossRef CAS PubMed.
- H. Sokol, B. Pigneur, L. Watterlot, O. Lakhdari, L. G. Bermúdez-Humarán, J. J. Gratadoux, S. Blugeon, C. Bridonneau, J. P. Furet, G. Corthier, C. Grangette, N. Vasquez, P. Pochart, G. Trugnan, G. Thomas, H. M. Blottière, J. Doré, P. Marteau, P. Seksik and P. Langella, Faecalibacterium prausnitzii is an anti-inflammatory commensal bacterium identified by gut microbiota analysis of Crohn disease patients, Proc. Natl. Acad. Sci. U. S. A., 2008, 105, 16731–16736 CrossRef CAS PubMed.
- S. N. Heinritz, E. Weiss, M. Eklund, T. Aumiller, S. Louis, A. Rings, S. Messner, A. Camarinha-Silva, J. Seifert, S. C. Bischoff and R. Mosenthin, Intestinal microbiota and microbial metabolites are changed in a pig model fed a high-fat/low-fiber or a low-fat/high-fiber diet, PLoS One, 2016, 11, e0154329 CrossRef PubMed.
- L. Zhou, L. Fang, Y. Sun, Y. Su and W. Zhu, Effects of the dietary protein level on the microbial composition and metabolomic profile in the hindgut of the pig, Anaerobe, 2016, 38, 61–69 CrossRef CAS PubMed.
Footnotes |
† Electronic supplementary information (ESI) available. See DOI: 10.1039/c8fo02438c |
‡ These authors equally contributed to the work. |
|
This journal is © The Royal Society of Chemistry 2019 |