DOI:
10.1039/C8FO02328J
(Paper)
Food Funct., 2019,
10, 3822-3827
Vitis vinifera ‘Pinot noir’ leaves as a source of bioactive nutraceutical compounds†
Received
26th November 2018
, Accepted 12th April 2019
First published on 6th June 2019
Abstract
Agricultural by-products are often hidden sources of healthy plant ingredients. The investigation of the nutritional values of these by-products is essential towards sustainable agriculture and improved food systems. In the vine industry, grape leaves are a bulky side product which is strategically removed and treated as waste in the process of wine production. In this work we performed an untargeted metabolomic profiling of the methanol extract of the leaves of Vitis vinifera cultivar ‘Pinot noir’, analysed their fatty acid content, and estimated their antioxidative capacity, with the purpose of investigating its nutritional and medicinal value. Fourier transform ion cyclotron resonance mass spectrometry (FT-ICR-MS) analysis identified the presence of numerous compounds which are known to possess diverse nutritional and pharmacological properties, particularly polyphenols and phenolic compounds (e.g. caffeic acid, catechin, kaempferol and quercetin), several phytosterols and fatty acids. Fatty acids were the most represented lipids’ secondary class, with the essential alpha-linolenic acid being the most abundant in ‘Pinot noir’ leaves, with a relative content of 42%. Also, we have found that ‘Pinot noir’ leaves present a high antioxidant capacity, putting grapevine leaves at the top of the list of foods with the highest antioxidative activity. Our findings scientifically confirmed that ‘Pinot noir’ leaves have a high content and diversity of biologically active phytochemical compounds which make it of exceptional interest for pharmaceutical and food industries.
Introduction
Used since ancient times in traditional medicine, plants are a major source of natural bioactive compounds with a wide range of applications in the pharmaceutical, food and cosmetic industries.1 However, the use of plants as a source of natural products faces quite a few challenges, mainly the availability of the compounds of interest, which can be very low, and the amount of the starting material, not always adequate for industrial extraction purposes.2 One strategy to overcome these issues is the selection of an abundant plant material with a high phytochemical content and nutritional value that is normally seen as agricultural waste and turn it into a valuable resource.
Considering its importance in the wine industry, grapevine (Vitis vinifera L.) is considered one of the most important fruit crops in the world, occupying over 7.5 Mha of the global area.3 Currently, there are about 2000 different cultivars of V. vinifera used for grape production and grown all over the world.4 About 50% of the world's produced grape is destined to wine production, a highly strategic industry for the economy of several countries, with 30% being targeted for the commercialization of fresh grapes and the rest is dried or consumed as grape juice or musts.3 Grapes contain a great diversity of secondary bioactive metabolites, namely vitamins, anthocyanins, flavonoids, polyphenols and stilbenoids, and are already being used for the development of food supplements5–7 and in cosmetics.8 Grapevine's bioactive compounds have been mostly studied at the grape level, whereas the vegetative parts, particularly leaves, are still a disregarded by-product of this industry. Grapevine leaves are a very abundant plant material, containing a wide range of phenolic compounds and antioxidants, being already used in traditional medicine for the treatment of bleeding, inflammation, diarrhoea and diabetes-induced hepatic complications.7,9 Additionally, vine leaves have already been included in human diet in several countries of the Mediterranean Basin, both fresh and brined, and therefore are getting the attention from top European wine producers.10
We performed an untargeted metabolomics approach to unfold the global view of the metabolic composition of leaves from V. vinifera ‘Pinot noir’. This cultivar is one of the world's most planted grapevines, occupying the 12th position worldwide (the 10th position among the wine making cultivars) and representing around 112 kha of the planted area.11 Fourier transform ion cyclotron resonance mass spectrometry (FT-ICR-MS) was used for metabolite profiling, already successfully used in plant metabolomics due to its ultra-high-resolution and ultra-high-mass accuracy.12–16 These performance characteristics allow the assignment of putative metabolite formulae based solely on accurate mass, by an exhaustive search and match with computed masses for a given chemical series of compounds, in combination with the metabolomic database search by accurate mass.14,17 In our analysis, lipids, mostly fatty acids, and phytochemical compounds were the most prevalent metabolites. Hence, fatty acids were subsequently quantified and the antioxidant capacity of ‘Pinot noir’ leaves was determined to assess their potential as a source of nutra-pharmaceutical compounds.
Results and discussion
V. vinifera ‘Pinot noir’ metabolic profile
A total of 6348 ions (peaks) were identified in ESI+, while in ESI− 1105 peaks were detected in the metabolic profile of V. vinifera ‘Pinot noir’ leaves. Through the analysis of the four metabolite fractions, nearly 1000 metabolic entities (unique masses) were identified: 785 in the positive ionization mode and 151 in the negative ionization mode. To provide insight into the metabolite diversity and elemental ratios, metabolite formulas were assigned to the detected masses (combining data from positive and negative ion modes), obtaining a total of 857 different formulas. Compound formulas were used to plot H/C ratios against O/C ratios in a Van Krevelen diagram and build a frequency histogram of CHO, CHON, CHOS and CHONS elemental compositions (Fig. 1).
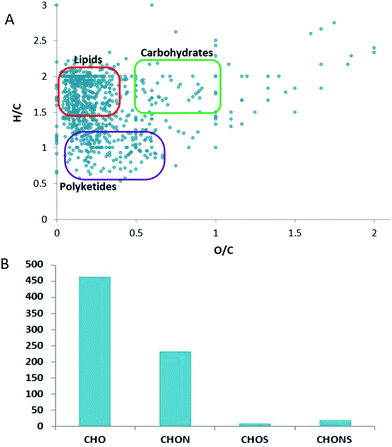 |
| Fig. 1 (A) Van Krevelen diagram of ‘Pinot noir’ leaves’ metabolic composition, built from 857 different formulas. Representative elemental ratio areas for selected groups of compounds are highlighted: lipids in red, carbohydrates in green, and polyketides in purple. (B) Frequency histogram of CHO, CHON, CHOS and CHONS elemental compositions in the metabolite formulas displayed in (A). | |
The highest density of formulas contains H/C ratios between 1 and 2 and O/C ratios between 0 and 0.5. This area corresponds mainly to lipids. Indeed, the compound database match and compound taxonomical annotation revealed that within the major metabolic classes, the lipids’ class was the most prevalent, accounting for 67% of the total detected metabolome.
Fatty acids (FAs) were the most represented lipids’ secondary class (48% of the total lipids, according to LipidMapsDB classification), followed by prenol lipids, polyketides and sterols (Fig. 2).
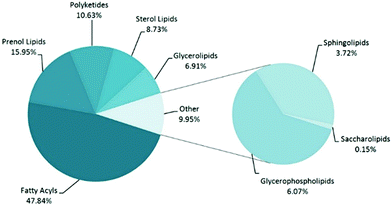 |
| Fig. 2 Lipids secondary classes’ annotation of grapevine lipids detected by FT-ICR-MS, according to the LipidMaps classification. | |
Fatty acids
Fatty acid quantification in ‘Pinot noir’ showed that alpha-linolenic acid (C18:3) was the most abundant FA in leaves (42% of the total quantified FAs). The second most abundant FAs were linoleic acid (C18:2) and palmitic acid (16:0), with 25% and 22% of the total FAs. The essential polyunsaturated fatty acids (PUFAs) C18:2 (omega-6) and C18:3 (omega-3) are not synthesized by human cells18,19 but are required to produce long chain PUFAs such as arachidonic and eicosapentaenoic acids, respectively. Therefore, some PUFAs have already been included in nutraceutical products (reviewed in ref. 20). These two PUFAs, which conserved signalling molecules involved in immune responses (reviewed in ref. 21), were putatively identified by MS in ‘Pinot noir’ leaves (ESI 1†), most likely from a microbiological source. Less abundant, but also present in significant amounts and also identified by MS, were oleic acid (C18:1), trans-hexadecanoic acid (C16:1t) and stearic acid (C18:0), (ESI 1†). Other FAs identified by MS included docosanedioic acid, dodecanoic acid, eicosanedioic acid and octacosanoic acid (ESI 1†), in agreement with the FA composition recently described for other grapevine cultivars.22
Grapevine leaves’ fatty acids profile was used to assess lipid nutritional quality, through the determination of atherogenicity (IA) and thrombogenicity (IT) health lipid indexes. These indexes are indicators of the effects of fatty acids in human health, mainly their contribution to the prevention of atheroma and thrombus formation. ‘Pinot noir’ leaves have low IA and IT levels, 0.32 and 0.17 respectively, meaning that they could be part of a healthy diet.
Sterols
Plant sterols (also named phytosterols) are another secondary class of lipids already incorporated in several foods23 for their ability to interfere with cholesterol absorption. In ‘Pinot noir’ leaves, we detected several putative sterols, including 22S-hydroxysitosterol, stylisterol B, 16α,17β-estriol 17-(β-D-glucuronide), 1-hydroxyvitamin D3 3-D-glucopyranoside and several other vitamin D3 derivatives (ESI 1†).
Polyketides
Within the polyketide subclass, flavonoids and phenolic lipids are the most relevant (reviewed in ref. 24). A highly important antioxidative phenolic acid putatively found in V. vinifera ‘Pinot noir’ was caffeic acid, together with its 3-glucoside and phenethyl ester forms (ESI 1†). Within the phenolic diterpenes, carnosol was putatively identified in ‘Pinot noir’ leaves (ESI 1†). The extraction of several polyphenols was already optimized from grape seeds to be used in food, cosmetics and pharmaceutical industries (reviewed in ref. 25). Flavonoids were already found in all V. vinifera tissues and organs.26–29 Putatively identified flavonoids in ‘Pinot noir’ leaves included the most common glycosylated forms and isoforms of kaempferol and quercetin, particularly glucoside, galactoside and glucuronide conjugate forms, rutin, catechin (and/or epicatechin) and gallocatechin (and/or epigallocatechin), (ESI 1†). Plant catechins (flavan-3-ols) are particularly interesting and have been widely used by the pharmaceutical industry as nutraceutical products.30 Together with caffeic acid, catechin and epicatechin were extracted from Uncaria sinensis to be used in the treatment of fevers and nervous disorders (reviewed in ref. 25). The dihydroflavonol astilbin, also present in grapes and wine (reviewed in ref. 29), was detected in ‘Pinot noir’ leaves. Resveratrol-derived flavonoids were also detected in ‘Pinot noir’ leaves, namely dihydroresveratrol, 4′-prenyloxyresveratrol and the resveratrol dimer ampelopsin (also named dihydromyrcetin), (ESI 1†). Anthocyanins and anthocyanidins also have antioxidant activity and potential biologically therapeutic effects (reviewed in ref. 24). Glucoside forms of the anthocyanins peonidin and cyanidin, delphinidin 3-O-rutinoside, leucocyanidin and 3-deoxyleucocyanidin were detected in ‘Pinot noir’ leaves (ESI 1†). An acylated anthocyanin, peonidin 3-(6′′-acetylglucoside), was putatively identified for the first time in ‘Pinot noir’. These acylated pigments combine their potent antioxidant properties with an increased stability, being therefore more attractive for use as supplements in commercial food products.31 Other natural antioxidants putatively detected in ‘Pinot noir’ leaves included ascorbic and dehydroascorbic acid and alpha-tocopherol (ESI 1†).
Antioxidant capacity of ‘Pinot noir’ leaves
The presence of polyphenols and phenolic compounds in grapevine leaves suggests that they can be a potential source of antioxidants (reviewed in ref. 9). Indeed, the metabolic profile of ‘Pinot noir’ leaves revealed several antioxidant compounds, hinting for a quite relevant antioxidant capacity. We measured the antioxidant activity in ‘Pinot noir’ leaves using the TEAC (Trolox Equivalents Antioxidant Capacity) method. ‘Pinot noir’ leaves have a high antioxidant capacity (2402 ± 198 μmol TE per 100 g fresh weight (Fw)), comparable to red cabbage (2591 μmol TE per 100 g Fw), and higher than strawberries (1846 μmol TE per 100 g Fw) or raspberries (1825 μmol TE per 100 g Fw), usually considered at the top of the list of foods with significantly high antioxidant activity.32 Like grape seeds, skin and pomace, already recognized as a good source of antioxidants33 grapevine leaves have thus an enormous potential as a source for the extraction of natural antioxidant compounds.
Conclusions
The grapevine associated industry is one of the biggest worldwide. Some premium cultivars as ‘Pinot noir’ are highly cultivated reaching plantation areas of 112 kha.11 Within this industry, leaves are strategically removed every season, to improve sunlight penetration, air circulation into the vine and grape quality and are considered a waste product. Here, we have shown that ‘Pinot noir’ leaves have high nutritional potential for human or animal consumption or to be used as a source of bioactive compounds. Grapevine leaves present a high content in fatty acids, with alpha-linolenic acid at the top of the most abundant FA. ‘Pinot noir’ leaves also contain several antioxidant compounds, particularly phenolic compounds and polyphenols, like caffeic acid, catechin, kaempferol, quercetin and resveratrol-derived flavonoids, with a very interesting antioxidant activity, among other several metabolites with interesting properties in the context of human health.
Grapevine leaves’ potential as a source of nutraceuticals has been overlooked. It is time to exploit this abundant natural product as a source of bioactive compounds.
Experimental
Plant material
V. vinifera ‘Pinot noir’ leaves were collected at the Portuguese Ampelographic Grapevine Collection (CAN, international code PRT051, established in 1988), at INIAV-Dois Portos, located at Quinta da Almoinha, 60 km north of Lisbon. This vineyard is trained on a double-cordon system, selecting only four canes in each arm. Plants were grafted on SO4 rootstock (Selection Oppenheim 4), in alluvial soil (pH 8.1 at 0–21 cm deep), in temperate oceanic climate (according to the Koppen climate system). Sanitary status is controlled for viral diseases and maintained with preventive phytochemical application every 12 to 15 days in the growing season. Since the beginning of the season (March 2015) until sampling time (May 2015), the mean temperature was 18 °C (Tmax: 24 °C; Tmin: 10 °C) and the precipitation had a mean value of 50 mm (reaching 100 mm in April), (data from the Instituto Português do Mar e da Atmosfera). The third to fifth leaves (from the shoot apex) were collected from 5 fully developed plants and were combined in 1 biological replicate, and 3 biological replicates were collected for the analysis. Leaves were immediately frozen in liquid nitrogen and stored at −80 °C until analysis.
Metabolite profile analysis by FT-ICR-MS
Metabolite extraction from V. vinifera ‘Pinot noir’ leaves was performed following a previously developed protocol compatible with direct infusion-FT-ICR-MS.15 After extraction, the different obtained fractions (chloroform, water, methanol and acetonitrile) were analysed by direct infusion on a 7T-FT-ICR mass spectrometer (Brüker Daltonics), using positive (ESI+) and negative (ESI−) electrospray ionization modes, as previously reported.15 Spectra analysis, compound identification and annotation were also performed as previously described.15 Compound formulas, obtained from MassTRIX analysis, were used to plot H/C ratios against O/C ratios in a Van Krevelen diagram and build a frequency histogram of CHO, CHON, CHOS and CHONS elemental compositions.
Fatty acid quantification
Fatty acids’ methyl esters (FAME) were prepared by direct trans-methylation of fatty acids using ground leaves with a mixture of methanol
:
sulfuric acid (97.5
:
2.5, v/v), with incubation at 70 °C for 1 h. Methyl esters were recovered in the organic phase after the addition of petroleum ether
:
ultrapure water (3
:
2, v/v). Quantitative analysis of fatty acids was achieved by gas chromatography (3900 Gas Chromatograph, Varian, Palo Alto, CA, USA) equipped with a flame ionization detector, using a fused silica capillary column (0.25 mm i.d. × 50 m, WCOT Fused Silica, CP-Sil 88 for FAME, Varian) at 210 °C. Heptadecanoic acid (C17:0) was used as the internal standard.
Indexes of lipid quality determination
Lipid nutritional quality can be assessed through the determination of the indexes of atherogenicity (IA) and thrombogenicity (IT). IA is defined as the relationship between the sum of the main saturated fatty acids (proatherogenic) and the sum of the main classes of unsaturated fatty acids (antiatherogenic), while IT represents the relationship between C16:0 and C18:0, saturated fatty acids (prothrombogenetic) with monounsaturated fatty acids (MUFAs), polyunsaturated fatty acids -n6 (PUFA-n6) and -n3 (PUFA-n3) (antithrombogenetic).34 The following equations were used for IA and IT calculation:
Antioxidant assay
Total antioxidant capacity was determined in ‘Pinot noir’ leaves. Total antioxidant capacity was analysed by measuring the Trolox equivalent antioxidant capacity (TEAC), following a previously described protocol.35 Briefly, 100 mg of frozen leaves were homogenized in 3 mL 50 mM potassium phosphate buffer pH 7.2, supplemented with 1% (w/v) of insoluble polyvinylpolypyrrolidone (PVPP 40000), at 4 °C. After centrifugation at 16
000g for 1 min, the supernatant was collected and used for the assay. Total antioxidant capacity was determined spectrophotometrically at 405 nm using the antioxidant assay kit and Trolox as the standard (Sigma-Aldrich, ref. CS0790). The assay was performed in 3 biological replicates, each analysed twice.
Conflicts of interest
There are no conflicts to declare.
Acknowledgements
Work supported by projects UID/MULTI/00612/2013, PEst-OE/QUI/UI0612/2013, PEst-OE/BIA/UI4046/2014, PTDC/BAA-MOL/28675/2017, IF 00819/2015 and grants SFRH/BPD/99712/2014 and SFRH/BD/116900/2016 from Fundação para a Ciência e Tecnologia (Portugal). We also acknowledge the support from the Portuguese Mass Spectrometry Network (LISBOA-01-0145-FEDER-022125) and the Project EU_FT-ICR_MS, funded by the Europe and Union's Horizon 2020 research and innovation programme under grant agreement nr. 731077.
References
- M. M. Cowan, Plant products as antimicrobial agents, Clin. Microbiol. Rev., 1999, 12, 564–582 CrossRef CAS PubMed
.
- A. G. Atanasov, B. Waltenberger, E. M. Pferschy-Wenzig, T. Linder, C. Wawrosch, P. Uhrin, V. Temml, L. Wang, S. Schwaiger, E. H. Heiss, J. M. Rollinger, D. Schuster, J. M. Breuss, V. Bochkov, M. D. Mihovilovic, B. Kopp, R. Bauer, V. M. Dirsch and H. Stuppner, Discovery and resupply of pharmacologically active plant-derived natural products: A review, Biotechnol. Adv., 2015, 33, 1582–1614 CrossRef CAS PubMed
.
- FAO and OIV, FAO-OIV FOCUS 2016 Table and dried grapes, Paris, 2016, ISBN 978-92-5-109708-3 (FAO), ISBN 979-10-91799-74-4 (OIV), 62 pp.
- T. Lacombe, L. Audeguin, M. Boselli, B. Bucchetti, F. Cabello, P. Chatelet, M. Crespan, C. D'Onofrio, J. Eiras Dias, S. Ercisli, M. Gardiman, M. S. Girando, S. Imazio, O. Jandurova, A. Jung, E. Kiss, P. Kozma, E. Maul, D. Maghradze, M. C. Martinez, G. Munoz, J. K. Patkova, I. Pejic, E. Peterlunger, D. Pitsoli, D. Preiner, S. Raimondi, F. Regner, G. Savin, S. Savvides, A. Schneider, J. L. Spring, A. Szoke, A. Veres, J.-M. Boursiquot, R. Bacilieri and P. This, Grapevine european catalogue: towards a comprehensive list, Vitis, 2011, 50, 65–68 Search PubMed
.
- M. Monagas, B. Hernandez-Ledesma, C. Gomez-Cordoves and B. Bartolome, Commercial dietary ingredients from Vitis vinifera L. leaves and grape skins: antioxidant and chemical characterization, J. Agric. Food Chem., 2006, 54, 319–327 CrossRef CAS PubMed
.
- A. Davalos, C. Gomez-Cordoves and B. Bartolome, Commercial dietary antioxidant supplements assayed for their antioxidant activity by different methodologies, J. Agric. Food Chem., 2003, 51, 2512–2519 CrossRef CAS PubMed
.
- M. Nassiri-Asl and H. Hosseinzadeh, Review of the pharmacological effects of Vitis vinifera (Grape) and its bioactive compounds, Phytother. Res., 2009, 23, 1197–1204 CrossRef CAS PubMed
.
- A. Barbulova, G. Colucci and F. Apone, New Trends in Cosmetics: By-Products of Plant Origin and Their Potential Use as Cosmetic Active Ingredients, Cosmetics, 2015, 2, 82–92 CrossRef CAS
.
-
D. S. Lacerda, P. C. Costa, C. Funchal, C. Dani and R. Gomez, Benefits of Vine Leaf on Different Biological Systems, in Grape and Wine Biotechnology, InTechOpen, 2016, ch. 6, pp. 125–143 Search PubMed
.
- A. Rizzuti, R. Caliandro, V. Gallo, P. Mastrorilli, G. Chita and M. Latronico, A combined approach for characterisation of fresh and brined vine leaves by X-ray powder diffraction, NMR spectroscopy and direct infusion high resolution mass spectrometry, Food Chem., 2013, 141, 1908–1915 CrossRef CAS PubMed
.
- OIV, OIV FOCUS 2017 Distribution of the world's grapevine varieties, Paris, 2017, ISBN 979-10-91799-89-8, 54 pp.
- M. Adrian, M. Lucio, C. Roullier-Gall, M. C. Heloir, S. Trouvelot, X. Daire, B. Kanawati, C. Lemaitre-Guillier, B. Poinssot, R. Gougeon and P. Schmitt-Kopplin, Metabolic Fingerprint of PS3-Induced Resistance of Grapevine Leaves against Plasmopara viticola Revealed Differences in Elicitor-Triggered Defenses, Front. Plant Sci., 2017, 8, 101 Search PubMed
.
- M. Ghaste, R. Mistrik and V. Shulaev, Applications of Fourier Transform Ion Cyclotron Resonance (FT-ICR) and Orbitrap Based High Resolution Mass Spectrometry in Metabolomics and Lipidomics, Int. J. Mol. Sci., 2016, 17, 816 CrossRef PubMed
.
- J. Han, R. M. Danell, J. R. Patel, D. R. Gumerov, C. O. Scarlett, J. P. Speir, C. E. Parker, I. Rusyn, S. Zeisel and C. H. Borchers, Towards high-throughput metabolomics using ultrahigh-field Fourier transform ion cyclotron resonance mass spectrometry, Metabolomics, 2008, 4, 128–140 CrossRef CAS PubMed
.
- M. Maia, F. Monteiro, M. Sebastiana, A. P. Marques, A. E. N. Ferreira, A. P. Freire, C. Cordeiro, A. Figueiredo and M. Sousa Silva, Metabolite extraction for high-throughput FTICR-MS-based metabolomics of grapevine leaves, EuPa Open Proteomics, 2016, 12, 4–9 CrossRef CAS PubMed
.
- Z. Lei, D. V. Huhman and L. W. Sumner, Mass spectrometry strategies in metabolomics, J. Biol. Chem., 2011, 286, 25435–25442 CrossRef CAS PubMed
.
- A. Aharoni, C. H. Ric de Vos, H. A. Verhoeven, C. A. Maliepaard, G. Kruppa, R. Bino and D. B. Goodenowe, Nontargeted metabolome analysis by use of Fourier Transform Ion Cyclotron Mass Spectrometry, OMICS, 2002, 6, 217–234 CrossRef CAS PubMed
.
- E. C. Borsonelo and J. C. Galduroz, The role of polyunsaturated fatty acids (PUFAs) in development, aging and substance abuse disorders: review and propositions, Prostaglandins, Leukotrienes Essent. Fatty Acids, 2008, 78, 237–245 CrossRef CAS PubMed
.
- V. M. Ursin, Modification of plant lipids for human health: development of functional land-based omega-3 fatty acids, J. Nutr., 2003, 133, 4271–4274 CrossRef CAS PubMed
.
- L. Das, E. Bhaumik, U. Raychaudhuri and R. Chakraborty, Role of nutraceuticals in human health, J. Food Sci. Technol., 2012, 49, 173–183 CrossRef CAS PubMed
.
- J. W. Walley, D. J. Kliebenstein, R. M. Bostock and K. Dehesh, Fatty acids and early detection of pathogens, Curr. Opin. Plant Biol., 2013, 16, 520–526 CrossRef CAS PubMed
.
- G. Laureano, J. Figueiredo, A. R. Cavaco, B. Duarte, I. Caçador, R. Malhó, M. Sousa Silva, A. R. Matos and A. Figueiredo, The interplay between membrane lipids and phospholipase A family members in grapevine resistance against, Plasmopara viticola, Sci. Rep., 2018, 8, 14538 CrossRef PubMed
.
- R. Korpela, J. Tuomilehto, P. Hogstrom, L. Seppo, V. Piironen, P. Salo-Vaananen, J. Toivo, C. Lamberg-Allardt, M. Karkkainen, T. Outila, J. Sundvall, S. Vilkkila and M. J. Tikkanen, Safety aspects and cholesterol-lowering efficacy of low fat dairy products containing plant sterols, Eur. J. Clin. Nutr., 2006, 60, 633–642 CrossRef CAS PubMed
.
- M. S. Brewer, Natural Antioxidants: Sources, Compounds, Mechanisms of Action, and Potential Applications, Compr. Rev. Food Sci. Food Saf., 2011, 10, 221–247 CrossRef CAS
.
- G. J. Gil-Chavez, J. A. Villa, J. F. Ayala-Zavala, J. B. Heredia, D. Sepulveda, E. M. Yahia and G. A. Gonzalez-Aguilar, Technologies for Extraction and Production of Bioactive Compounds to be Used as Nutraceuticals and Food Ingredients: An Overview, Compr. Rev. Food Sci. Food Saf., 2013, 12, 5–23 CrossRef CAS
.
- E. Braidot, M. Zancani, E. Petrussa, C. Peresson, A. Bertolini, S. Patui, F. Macri and A. Vianello, Transport and accumulation of flavonoids in grapevine (Vitis vinifera L.), Plant Signaling Behav., 2008, 3, 626–632 CrossRef PubMed
.
-
I. Hermosin-Gutierrez, N. Castillo-Munoz, S. Gomez-Alonso and E. Garcia-Romero, in Progress in Authentication of Food and Wine, ACS Publications, 2011, vol. 1081, ch. 8, pp. 113–129 Search PubMed
.
- K. Schoedl, R. Schuhmacher and A. Forneck, Studying the polyphenols of grapevine leaves according to age and insertion level under controlled conditions, Sci. Hortic., 2012, 141, 37–41 CrossRef CAS
.
- K. Ali, F. Maltese, Y. H. Choi and R. Verpoorte, Metabolic constituents of grapevine and grape-derived products, Phytochem. Rev., 2010, 9, 357–378 CrossRef CAS PubMed
.
- Q. V. Vuong, J. B. Golding, M. Nguyen and P. D. Roach, Extraction and isolation of catechins from tea, J. Sep. Sci., 2010, 33, 3415–3428 CrossRef CAS PubMed
.
- M. M. Giusti and R. E. Wrolstad, Acylated anthocyanins from edible sources and their applications in food systems, Biochem. Eng. J., 2003, 14, 217–225 CrossRef CAS
.
- A. R. Proteggente, A. S. Pannala, G. Paganga, L. Van Buren, E. Wagner, S. Wiseman, F. Van De Put, C. Dacombe and C. A. Rice-Evans, The antioxidant activity of regularly consumed fruit and vegetables reflects their phenolic and vitamin C composition, Free Radical Res., 2002, 36, 217–233 CrossRef CAS PubMed
.
- T. Varzakas, G. Zakynthinos and F. Verpoort, Plant Food Residues as a Source of Nutraceuticals and Functional Foods, Foods, 2016, 5, 88 CrossRef PubMed
.
- M. Garaffo, R. Vassallo-Agius, Y. Nengas, E. Lembo, R. Rando, R. Maisano, G. Dugo and D. Giuffrida, Fatty Acids Profile, Atherogenic (IA) and Thrombogenic (IT) Health Lipid Indices, of Raw Roe of Blue Fin Tuna (Thunnus thynnus L.) and Their Salted Product “Bottarga”, Food Nutr. Sci., 2011, 2, 736–743 CAS
.
- A. Figueiredo, J. Martins, M. Sebastiana, A. Guerreiro, A. Silva, A. R. Matos, F. Monteiro, M. S. Pais, P. Roepstorff and A. V. Coelho, Specific adjustments in grapevine leaf proteome discriminating resistant and susceptible grapevine genotypes to Plasmopara viticola, J. Proteomics, 2017, 152, 48–57 CrossRef CAS PubMed
.
Footnotes |
† Electronic supplementary information (ESI) available: Tables with the putatively identified compounds by MS. See DOI: 10.1039/c8fo02328j |
‡ These authors are both corresponding and co-senior authors in this paper. |
|
This journal is © The Royal Society of Chemistry 2019 |
Click here to see how this site uses Cookies. View our privacy policy here.