DOI:
10.1039/C8FO02099J
(Paper)
Food Funct., 2019,
10, 997-1006
Enriching novel dark chocolate with Bacillus coagulans as a way to provide beneficial nutrients
Received
25th October 2018
, Accepted 3rd January 2019
First published on 1st February 2019
Abstract
The aim of the present study was to develop a novel type of probiotic chocolate with the additive Bacillus coagulans bacteria and determine the concentration of polyphenols and their bioaccessibility. The manufactured chocolate possessed significantly higher concentrations of polyphenols than the control sample. The sensory profiles of the tested probiotic chocolate were similar to those of the control sample. In future, the probiotic chocolate could be regarded as a functional food product by chocolate producers.
Introduction
Chocolate is manufactured from cacao powder, cacao butter (cacao fat), and a portion of sugar or another sweetening agent; the production process of chocolate comprises many stages. This process includes fermentation of cacao beans; the beans are then dried, cleaned, roasted, ground, squeezed, rolled, conched, and tempered.1 Chocolate is criticized for its saturated fatty acid and high calorific content. Nevertheless, it is recognized for the concentration of biologically active compounds in it.2,3 It is a source of polyphenols (mainly flavonoids), biogenic amines (tyramine and phenylethylamine), purine alkaloids (caffeine and theobromine), and mineral salts (i.e., magnesium).3,4 In light of growing interest in functional food rich in nutrients and health-promoting compounds as well as considering the demand from the consumers, primarily from children, probiotic chocolate is an interesting product meeting all these criteria. At present, only a few products containing probiotic bacteria, primarily of the genus Lactobacillus, are known.5–7 An alternative and completely new product of this group is milk chocolate with the addition of Bacillus bacteria, namely Bacillus coagulans GBI-30, 6086. This bacterial strain with the commercial name GanedenBC30® has unique technical and functional characteristics, because of which it has been used in the food industry. B. coagulans GBI-30, 6086 is a bacterial strain producing lactic acid in the form of the +L isomer, with the GRAS (Generally Recognized As Safe) status; it exhibits resistance to unfavorable conditions of the gastrointestinal tract (high and low pH) as well as to harsh production conditions (high temperature and elevated pressure).8,9 The capacity of the genus Bacillus to form endospores determines its ability to resist stress conditions. This is a highly significant trait, which is atypical of the majority of microorganisms with probiotic potential.10,11
The aim of the study was to assess the influence of the storage time of probiotic chocolate with B. coagulans on the content of bioactive compounds and the quality using physicochemical and sensory parameters. In addition, the maintenance of bacterial counts during the storage period and in the course of digestion in an in vitro model was investigated.
Materials and methods
Materials
The samples of chocolates were purchased from the semi-industrial scale factory of confectionery products “BARS” Halina Kalemba (Wloszakowice, Poland). The examined dark chocolate masses and bars of these chocolates were enriched in a lyophilizate of live cells of probiotic Bacillus coagulans GBI-30, 6086 strains. Table 1 presents the nutritional value of the used chocolate mass.
Table 1 Nutritional value of prepared chocolate mass
Energy (kcal kJ−1) |
457/1888 |
Fat (g per 100 g) |
35.1 |
Saturated fatty acids (g per 100 g) |
21.4 |
Monosaturated fatty acids (g per 100 g) |
12.3 |
Polysaturated fatty acids (g per 100 g) |
1.4 |
Cholesterol (mg per 100 g) |
0.4 |
Carbohydrates (g per 100 g) |
47.1 |
Starch (g per 100 g) |
3.6 |
Sugars (g per 100 g) |
0.3 |
Lactose (g per 100 g) |
0 |
Sucrose (g per 100 g) |
0.3 |
Polyols (g per 100 g) |
43.3 |
Proteins (g per 100 g) |
5.5 |
Digestive fiber (g per 100 g) |
7.8 |
Iron (mg per 100 g) |
13.1 |
Calcium (mg per 100 g) |
37.1 |
Preparation of prototypes of probiotic chocolate with the addition of Bacillus coagulans bacteria
The chocolate was dissolved in a stainless steel heater with a water jacket equipped with a mixer set at 100 rpm at 70 °C. A B. coagulans lyophilizate was prepared in three variants corresponding to the counts of 106 (Ch1), 107 (Ch2), and 108 (Ch3) CFU g−1 by dissolving the lyophilizate. The contents were heated and mixed for 5 min and subsequently poured into forms measuring 1 cm × 1 cm × 0.4 cm that were left to cool at 21 °C. The cooled samples were stored to the time of analysis (T1—after production; T2—after 1 month of storage; T3—after 3 months of storage; T4—after 6 months of storage; T5—after 12 months of storage and T6—after 24 of storage which was only for bacterial count determined) at room temperature in tightly closed, dark containers.
Methods
Ph and water activity (aw) measurement.
The pH changes in three types of probiotic chocolates were determined using a pH meter (Mettler-Toledo, USA).5 Water activity (aw) was determined using the aw-meter Thermoconstanter Novasina RTD 33 TH-1. Analyses of the listed parameters were performed at the beginning of the experiment and during the storage period at measurement points (T2–T5).
Extraction.
Extraction from chocolate samples was based on Belščak-Cvitanović et al.'s procedure.12 First, the samples were defattened three times in the ratio of 2.0 g of chocolate material per 10 mL of n-hexane. After that point, the defattened cocoa solids were air-dried for 24 h to remove the residual organic solvent.13 The cleaned and defattened 2.0 g samples were extracted two times with 10 mL of aqueous methanol (40
:
60) (700 g L−1) for 30 min in an ultrasonic bath in order to obtain the extract.12 After each extraction, the mixture was centrifuged for 10 min at 3000g and the supernatant was decanted. The supernatants were combined and filtered to remove the residual particles.
Catechin content.
Catechins were identified by the HPLC method by using the column RP-C18 (150 × 4.6 mm; 5 μm) and a photo-diode detector (λ = 279 nm) and performing the analysis in a gradient elution system. As an eluent, a mixture of 0.01 M phosphate buffer and methyl alcohol was used with a flow rate of 1 mL min−1.14 The used references were as follows: (−)-epicatechin (min. 90%, Sigma-Aldrich), (+)-catechin (min. 98%, Sigma-Aldrich), and (−)-epigallocatechin gallate (min. 95%, Sigma-Aldrich). The relationship between the resultant peak surfaces and the concentrations of the three studied compounds was observed. Calibration curves of the studied compounds were determined with 7 concentration levels and 5 independent measurements for each of them. The concentrations of (+)-catechin and (−)-epicatechin in the analyzed products were presented in mg per 100 g d.w. and the concentration of (−)epigallocatechin gallate in g per 100 g d.w.
Composition of phenolic acids.
The procedure was based on the methodology described previously by Kulczyński et al.15 Phenolic acids were analysed as a percentage composition using an Agilent UPLC equipped with a Bin Pump Infinity DAD 1290 detector (λ = 260 nm and 310 nm). A non-linear concentration gradient was used to analyse the dry extract. The gradient consisted of H3PO4 buffer (solvent A), pH = 2.7, which was adjusted using 1
:
1 v/v acetonitrile–water (solvent B). The gradient profile decreased from 95% of solvent A at 1 min to 50% of B at 52 min and the run time was 58 min. The injected volume of samples after filtration (PTFE filter; 0.45 μm) was 10 μL. Phenolic acids were identified using the standards dissolved in methanol (caffeic, chlorogenic, ferulic, p-coumaric and sinapic acids).
Composition of flavonols.
The percentage composition of flavonols was determined according to the method described by Kulczyński et al.15 Extracted flavonols were separated and identified by Agilent UPLC using a Nova-Pak C18 reversed-phase column (3.9 × 150 mm, 5 μm particle size; both from Waters, Milford, MA, USA). Solvent A was 0.3% (v/v) HCOOH in H2O, while solvent B was 100% CH3CN. The flow rate was maintained at 1 mL min−1. The gradient profile was as follows: 85% of A at 0 min and 25% of A at 40 min. Chromatograms were obtained using a UV-Vis detector at λ = 370 nm. Separated compounds of individual flavonols were determined as isoquercetin, quercetin, hyperoside and kaempferol and their percentage composition was calculated.
Sensory profiling.
Profile assessment of the tested dark probiotic chocolate samples was performed in the sensory laboratory. To determine a broad range of sensory characteristics, that is, the sensory profiling, a quantitative method was used. The sensory profiling was performed by a 16-person team who had been trained for this purpose, and their sensory sensitivity was confirmed. As a part of the analysis, the following qualitative determinants were marked: color (brown, gray, crème, and general preference), odor (yeast, off, seasoning, metallic, and general preference), taste (salty, spicy, yeast, off, metallic, chemical, bitter, and general preference), and consistency (moisture and crispness). The intensity of each qualitative mark was determined using a structured 10 cm-long line scale with adequate edge captions (intense/not intense). The obtained results were converted into numerical values and noted in points. Trials were encoded and given to the examiners, guaranteeing the anonymity of the first, by placing them in white, odorless, lockable vessels. All trials were performed at the same temperature (ambient temperature), and the trial quantity was suited for the test so as to guarantee the possibility of testing the product several times. Selected results were based on three individual marks of examiners, done during three sessions.
In vitro digesting model.
To determine the kinetics of bacterial count changes in the probiotic chocolate containing Bacillus genus bacteria, the in vitro digestive system model was applied. The experimental set was built up with a 1-liter volume fermenter (Sartorius Poland). The set temperature was maintained at 37 °C. Two general experimental trials were conducted in this study. The first was the control run, in which the bacterial lyophilizate was dissolved in PBS buffer (pH 7.4) (Merck-Sigma). The latter trial involved the milk chocolate with B. coagulans at concentrations of 1.0 × 106, 1.0 × 107, and 1.0 × 108 CFU g−1. In the initial step, a mixture of chocolate samples in PBS buffer or a selected nutrient matrix was prepared. This prepared sample was subjected to the first step of in vitro digesting, which was designed for simulating the conditions occurring in the stomach. A modeling mixture of the gastric juice was prepared by adding 300 U mL−1 pepsin and lowering the pH to 4.0 (1 M HCl). This step was performed for 4 h at 37 °C (P2). Peristaltic movements were simulated by stirring with a magnetic stirrer. In the next step, digestion in the small intestine was imitated by changing the pH to 6.0 by using 1 M NaHCO3 and adding 10 mL of pancreatico-intestinal extract (P3). The next step was to increase the pH to 7.4 by adding 1 M NaHCO3 and introduce a standardized inoculum of intestinal microflora (106 CFU mL−1 of the food content) isolated from human stool. The process was carried out for 2 h at 37 °C (P4). To simulate the passage of the product through the large intestine, the pH was increased to 8.0 with 2 M NaHCO3, and further digestion was carried out under anaerobic conditions for 18 h (P5).
Bacterial count determination.
One gram of the sample was dissolved in 199 mL of peptone water and mixed for 5 min in a Stomacher. The suspension pH was adjusted to 8.5. Then, 20 mL of the suspension was incubated at 75 °C for 30 min. Subsequently, the suspension was immediately cooled to a temperature below 40 °C. In the next step, 1.0 mL of the cooled suspension was transferred to a sterile tube containing 9 mL of peptone water. Thus, in the prepared suspension, the microbial count was 0.5 × 103 CFU g−1 of product. Then, further decimal dilutions were performed. A volume of 1.0 mL of the sample prepared was spread on the surface of the media, and at least 2 Petri dishes for each medium were used for each dilution of the sample. To determine the total Bacillus count, a Bacillus selective agar base without supplement liquefied with medium was used. The dishes were incubated for a minimum of 48 h at 40 °C.
Storage test.
All chocolate variants, as well as the bacterial lyophilizate, were stored for 24 months both at room temperature (20 °C) and under refrigeration conditions (4 °C). Analysis of bacterial viability was performed in accordance with the description of bacterial count determination at the following time intervals: 1-week post-production and 1, 3, 6, 12, and 24 months post-production.
Statistical analysis.
The experiment was based on threefold repetition, and all measurements were duplicated. The STATISTICA PL 13.1 (StatSoft, Inc., Krakow, Poland) software was used for the calculation of the principal component analysis (PCA), values of means, standard deviations, and the significance of differences between means (p < 0.05, analysis of variance, Tukey's multiple range test). The analysis of variance (ANOVA) was conducted for a completely randomized design (CRD) experiment. Then, Tukey's test was used for comparing the mean values at a significance level of p ≤ 0.05. The slope of the regression curve (coefficient A/24 h) was determined in the Excel 2007 program to present the dynamics of measured factors, that is, lipid oxidation and physical and microbial changes, and to show the changes in the activity of AChE and BChE during storage. The data were expressed as mean values and standard deviations of independent measurements for four specimens (n = 4). The relationship between the factors noted in Table 1 and storage periods for each factor is described using the equation: Y = A × X + B, where y is the dependent variable, x is the independent variable, A is the independent variable coefficient per slope of the line, and B is the intercept.
Results
Ph measurements, water activity, and catechin and polyphenol content changes
In the tested chocolates, during the entire storage period, the course of pH and water activity (aw) changes was monitored, together with the monitoring of the polyphenol content (Table 2). During storage, the pH of the chocolates increased and remained in the range from 5.16–5.23 at the beginning of the experiment to 6.04–6.22 at the last stage of the study (after 24 months of storage). The R2 coefficient confirmed the linear dynamics changes of the pH value during the chocolate sample storage. The R2 coefficient remained in the range of 0.480–0.868, thus indicating a disproportionate increase in pH relative to the storage time. It has been demonstrated that the addition of probiotic bacteria does not influence the pH of the tested samples. The water activity index was monitored during the storage of chocolates. There was an increase in the aw from 0.231 to 2.255 directly after production and it decreased to 0.121–0.134 after 12 months of storage; thus, the water activity was reduced almost twofold. During storage, the smallest changes in water activity were observed in the sample with the lowest addition of probiotic bacteria (106 CFU g−1). The R2 coefficient remained in the range of 0.826–0.969, indicating a proportionate decrease in the aw during storage. A decrease in the total polyphenol content was demonstrated in the probiotic chocolates within the experimental duration. However, the addition of probiotic bacteria did not influence these changes. In all chocolate samples, the changes in the level of the polyphenol content were statistically insignificant throughout the 12-month storage period. Only after 24 months of storage, a significant reduction in the polyphenol content was observed, which amounted to 15.9% in the sample with the highest addition of bacteria and up to 16.5% in the control sample. In the case of epicatechin and catechin, the most dynamic decrease in the content was observed at the second measurement point, that is, after 3 months. A further decrease in the contents of these components was minor and comparable in all samples. At the final stage of the study, the decrease in these components was found to have reached 45%, considering the high dynamics of decrease in the first storage period. In contrast to the above finding, epigallocatechin showed the highest stability during storage, and only at the final measurement point, its content decreased significantly by 15% relative to the initial value. The dynamics of the decrease in the epigallocatechin content compared to that of catechins and gallocatechins showed the lowest rate in all tested samples. The results show that the addition of probiotic bacteria to chocolate does not influence changes in the stability of the tested catechins.
Table 2 Changes in the pH and aw values observed at different storage times
Sample |
Storage time |
Dynamics of changes* |
T1 |
T2 |
T3 |
T4 |
T5 |
Coefficient A × 10−2/24h (R2) |
The mean values in the entries marked with different small letters indicate the significance of differences (p < 0.05). *Linear regression equation, coefficient A/time of storage − change of coefficient A during storage, R2 – coefficient of determination, p < 0.05. |
pH |
Control |
5.16ab ± 0.09 |
5.29b ± 0.12 |
5.21b ± 0.11 |
5.12a ± 0.13 |
6.19c ± 0.13 |
0.4806 |
Ch1 |
5.17a ± 0.03 |
5.23b ± 0.09 |
5.21b ± 0.09 |
5.24c ± 0.13 |
6.22b ± 0.16 |
0.5839 |
Ch2 |
5.20a ± 0.07 |
5.21a ± 0.15 |
5.39b ± 0.06 |
5.60c ± 0.08 |
6.14d ± 0.11 |
0.8684 |
Ch3 |
5.23ab ± 0.08 |
5.26b ± 0.17 |
5.28bc ± 0.09 |
5.30c ± 0.11 |
6.04d ± 0.17 |
0.6090 |
a
w
|
Control |
0.234c ± 0.01 |
0.211b ± 0.01 |
0.201b ± 0.01 |
0.122a ± 0.03 |
0.126a ± 0.04 |
0.8835 |
Ch1 |
0.243d ± 0.04 |
0.233c ± 0.01 |
0.211b ± 0.03 |
0.132a ± 0.02 |
0.134a ± 0.03 |
0.8757 |
Ch2 |
0.255e ± 0.02 |
0.221d ± 0.03 |
0.199c ± 0.03 |
0.143b ± 0.04 |
0.131a ± 0.02 |
0.9691 |
Ch3 |
0.231d ± 0.04 |
0.229d ± 0.04 |
0.201c ± 0.05 |
0.155b ± 0.06 |
0.121a ± 0.02 |
0.9351 |
Polyphenolic compound content analysis
In the examined probiotic chocolates (Fig. 1), the selected flavonols and phenolic acids were analysed qualitatively and quantitatively. In the examined chocolate, the percentage share of flavonols was demonstrated in the following order: quercetin > isoquercetin > kaempferol > hyperoside. The quercetin content constituted over 50% of the share of the analyzed flavonols. In terms of phenolic acids, the percentage share was as follows: chlorogenic acid > ferulic acid > p-coumaric acid > sinapic acid. Chlorogenic acid constituted over 50% of all analyzed acids. It was found that the addition of probiotic bacteria to chocolate did not have a statistically significant effect on the percentage composition of the tested polyphenols.
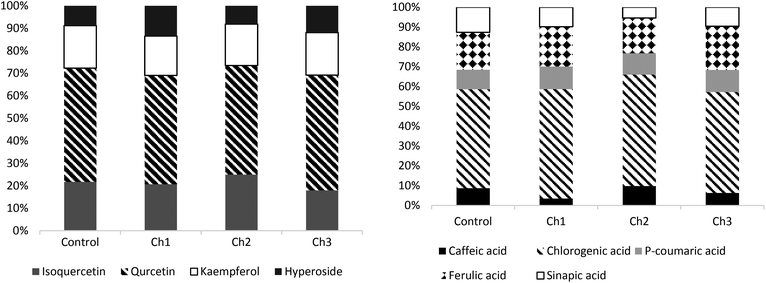 |
| Fig. 1 The percentage composition of flavonols and phenolic acids in the tested probiotic chocolate samples. | |
Sensory profile
Fig. 2 presents the sensory profiles of taste, odor, color, and consistency. The following odor and taste descriptors were selected on the basis of the preliminary assessment of experimental samples: odor: bitter, off aroma, chocolate aroma, and sourness; taste: sweetness, chocolate flavor, sourness, spicy, bitter, and off taste. The influence of probiotic bacteria on the color and consistency of chocolates was also assessed. Among the tested odor descriptors in the analyzed samples, the dominant descriptors were chocolate aroma and sourness. Off aroma was almost indiscernible. Minor changes were observed in the odor profiles of the tested samples and the control sample without Bacillus bacteria. In terms of the taste of samples with probiotic bacteria, significant differences were found in the sensation of chocolate and spicy taste. The addition of probiotics at a level of 106 CFU g−1 to chocolate showed a significant effect on the reduction of sensation of these tastes. There was no influence of probiotic bacteria on the color and consistency of the examined chocolates.
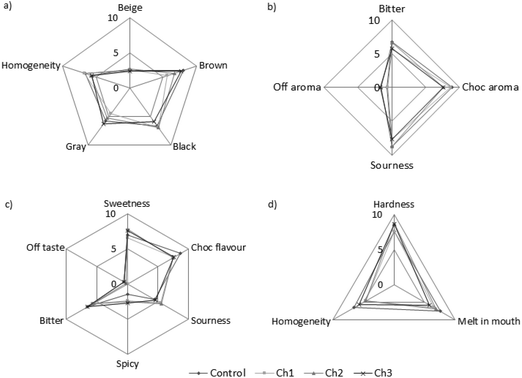 |
| Fig. 2 Sensory profiles of the tested chocolate samples: (a) color; (b) aroma; (c) taste, and (d) consistency. | |
In vitro digestion model
In the subsequent stage of the study, the influence of conditions simulating the digestion process on the viability of B. coagulans bacteria added to the chocolate was analyzed. Table 4 presents the study results. In the variant, which tested the influence of in vitro digestion on the lyophilized bacteria suspended in PBS buffer, the highest decrease in bacterial counts was observed in the consecutive stages of the digestion process. Initially (P1), the B. coagulans count was 2.2 × 107 CFU g−1. After 4 h of incubation, the bacterial count reduced to 2.1 × 104 CFU g−1 (P2). In the next stage, in which the aim was to simulate the environment of the small intestine, the count of B. coagulans was stable in the presence of pancreatic and intestinal juice and amounted to 4.5 × 104 CFU g−1 (P3). The next stage involved increasing the pH to 7.4 (P4); after completion of this stage, the count of B. coagulans increased to 5.2 × 104 CFU g−1. After the completion of the passage of the product through the large intestine (P5), the count of B. coagulans was 2.1 × 104 CFU g−1. The next experimental variant analyzed the variability of the count of B. coagulans contained in milk chocolate at a varying initial dose of the lyophilizate used at the production stage. The best results were obtained for the variant in which the used dose of the lyophilizate enabled obtaining the count of B. coagulans bacteria in the ready product at 1.9 × 108 CFU g−1. The count of bacteria decreased to 9.2 × 106 CFU g−1 (P2) after 4 h of incubation. The subsequent phase simulated the environment of the small intestine in the presence of pancreatic and intestinal juice, and the count of B. coagulans was again reduced to 2.8 × 106 CFU g−1 (P3). The next stage involved increasing the pH to 7.4 (P4). After this stage was completed, the count of B. coagulans was slightly changed and increased to 3.2 × 108 CFU g−1. After the product passed through the conditions that imitated the large intestine (P5), the count of B. coagulans was again increased to 2.2 × 1010 CFU g−1. The present results thus indicate that chocolate constitutes a good matrix for the growth of Bacillus bacteria. This matrix enables to maintain a high count of living cells even after the transfer through stages simulating conditions prevailing in the gastrointestinal tract.
The last stage of the study aimed at assessing the stability of B. coagulans bacteria in the finished product, that is, milk chocolate. Three variants of chocolate containing the bacterial lyophilizate were examined. The products were stored both at room temperature and under refrigeration conditions. The obtained refrigeration test results are presented in Fig. 3. The analysis was performed at defined time intervals, thereby enabling the determination of the possible product storage time. The data presented in the chart demonstrate that the highest stability in terms of the bacterial survival rate was shown by the lyophilizate of B. coagulans bacteria stored at room temperature. In this case, the decrease in cell survival was from 3.1 × 109 to 2.8 × 105 CFU g−1 after 3 months of storage. After 24 months of storage, the bacterial count in the lyophilizate was 1.7 × 103 CFU g−1. In the variants, where chocolate constitutes the matrix, to which lyophilized bacteria were added, a similar tendency was observed both at room temperature and under refrigeration conditions. However, under refrigeration conditions, the decrease in live bacterial cells was lower. Thus, in the variant where the initial bacterial count in the product was equal to 108 CFU g−1 and the chocolate was stored under refrigeration conditions, the initial bacterial count was maintained until the period of 6 months and changed only slightly from 2.1 × 108 to 1.9 × 108 CFU g−1. In 12 months of storage, a reduction in the number of live cells to 5.8 × 107 CFU g−1 was observed. This value was maintained until the end of the storage period, that is, up to 24 months. In the same product variant that was stored at room temperature, a reduction in the viability of cells was already observed in 3 months of storage. From the value of 2.1 × 108 CFU, the count of live cells reduced to 1.8 × 107 CFU; these values, however, did not reduce further.
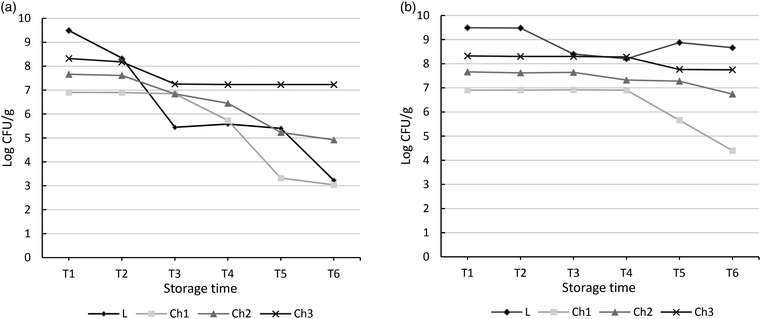 |
| Fig. 3 Survivability of B. coagulans stored at (a) 4 °C and (b) 18 °C: (L) lyophilizate of probiotic B. coagulans; (Ch1) chocolates with the lyophilizate of probiotic B. coagulans – 106 CFU g−1; (Ch2) chocolates with the lyophilizate of probiotic B. coagulans – 107 CFU g−1; (Ch3) chocolates with the lyophilizate of probiotic B. coagulans – 108 CFU g−1. | |
Discussion
In the present study, the physicochemical characteristics, sensory properties, and functional properties were analyzed for chocolate with the addition of B. coagulans GBI-30, 6086 (GanedenBC30) bacteria. Supplementation of sugar confectioneries with probiotics is problematic because certain manufacturing processes are conducted at high temperatures, and the products are normally stored at room temperature. Moreover, chocolates and chocolate products should be stored in rooms with a relative air humidity of 75% and a temperature of 18 °C. The storage time varies and depends on the properties of products. During storage, various physicochemical processes occur in chocolate products, that is, hydrolysis, oxidation, lipid migration, water migration, and ethanol migration as well as transformation in sugars—sugar bloom and sucrose inversion.16 Water migration typically occurs in the products with a difference in the water activity of the filling and that of the external coating.17 The consequences of migration may involve product damage, expressed as cracking of the external portion; drying out of the internal portion; or appearance of mold.18,19 Considering the fact that water availability is one of the major factors limiting the growth of microorganisms, the monitoring of the water activity of stored products constitutes one of the methods to determine their stability.20 In the examined chocolates, water activity decreased clearly only after 6 months of storage, and despite this fact, the microbial quality indicated that the product was safe and of high quality (Table 2). Acidity is another important quality indicator.2 It depends on the composition of raw materials used to produce chocolate. Chocolate may be subject to rancidity because of its high fat content. Thus, changes in acidity may constitute the first indicator of coca fat rancidity in chocolate. In the present study, the results of chocolate samples indicated that the addition of probiotic bacteria did not contribute towards causing acidity changes (Table 2). Similar results were already obtained and described in the study of ref. 20.
The high quality of probiotic chocolates determined from the results of physicochemical indices was confirmed by the sensory assessment (Fig. 2). Sensory properties such as taste, consistency, and color reflect a product's quality and are significant in the selection of the product by the consumer.21 In the present study, the addition of probiotic bacteria did not have any influence on the odor and color of chocolate. Among the known taste descriptors, probiotic bacteria influenced solely the reduction in the chocolate and spicy taste (Fig. 2). The presence of metabolites such as lactic acid influences the change in taste sensation.21 Similar relationships were noted in studies conducted by other researchers, where the use of probiotics in ice cream did not reduce the desirability and sensation of the chocolate flavor.22
Another significant aspect covered in this study is the assessment of the biofunctionality of the developed probiotic chocolate, the composition of which differed in the dose of B. coagulans bacteria. One of the significant characteristics that determine the biofunctionality of a food product is the assessment of the amount of compounds with antioxidative properties, including polyphenols.3 Thus, the concentration of these compounds in chocolate has been investigated by numerous studies.21,23 Both the content and the percentage share of the tested compounds classified as polyphenols differed from the contents in the described experiments (Table 3), similar to those observed in other studies.21,23 As an example, catechins constitute a group of compounds with the highest quantitative share in cocoa and products containing cocoa and constitute approximately 37% of the total amount of polyphenols contained in cocoa seed. These include (−)-epicatechin, (+)-catechin, (+)-gallocatechin, and (−)-epigallocatechin, among which (−)-epicatechin constitutes 35% flavan-3-ols.24 Martini et al. demonstrated that quercetin-3-O-pentoside was the dominant flavanol in chocolate, the content of which was 3.31 mg per 100 g of dark chocolate, and the most concentrated phenolic acid was ferulic acid (61 mg per 100 g).23 Apart from ferulic acid, the present study indicated a considerable share of chlorogenic acid (Fig. 1b). Previous studies have shown many health-promoting properties of polyphenolic compounds, such as bioavailability, antioxidative potential, prevention of atherosclerosis, anti-inflammatory and anti-neurogenerative properties as well as the capacity to react with cellular receptors, stem directly from the chemical structure of these compounds.25–27 According to Tresserra-Rimbau et al., the polyphenol retention may depend on the interaction of these compounds with other nutrients.25 When the nutritional matrix is rich in fatty acids, increased bioavailability may be observed.28 Secondly, polyphenols are capable of binding to the proteins present in food, thus reducing their bioretention.28 Proteins present in chocolate are typically of milk origin. They might constitute a part of the chocolate mix (e.g., whey) or constitute a contaminant, which found its way into a food product due to either inaccurate meeting of GMP principles or technical issues. In recent years, numerous studies have been conducted on the bioavailability of epicatechin from dark chocolate and its antioxidative properties.29,30 The results have demonstrated that the presence of proteins in chocolate reduces the antioxidative potential and bioavailability of flavonoids. The process of releasing flavonoids from the nutritional matrix and their absorption, distribution, metabolism, and egestion are of key importance from the viewpoint of bioavailability assessment. Serafini et al. revealed that the matrix shows its specific influence on the bioactivity of flavonoids, and it may not be explained alone through the flavonoid–milk protein interaction mechanism.30 Gallo et al. have also confirmed this fact in their study.31
Table 3 Changes in the total count of polyphenols and content values of epicatechin, catechin and (−)epigallocatechin observed at different storage times
Sample |
Storage time |
Dynamics of changes* |
T1 |
T2 |
T3 |
T4 |
T5 |
Coefficient A × 10−2/24h (R2) |
The mean values in the entries marked with different small letters indicate the significance of differences (p < 0.05). *Linear regression equation, coefficient A/time of storage − change of coefficient A during storage, R2 – coefficient of determination, p < 0.05. |
Total polyphenols [g per 100 g d.w.]
|
Control |
33.79c ± 0.05 |
33.21b ± 0.03 |
32.26b ± 0.02 |
32.40b ± 0.03 |
28.22a ± 0.05 |
0.8171 |
Ch1 |
33.78b ± 0.06 |
32.09b ± 0.03 |
31.29b ± 0.03 |
29.37a ± 0.02 |
28.66a ± 0.03 |
0.9582 |
Ch2 |
33.88b ± 0.02 |
32.14b ± 0.02 |
32.29b ± 0.02 |
29.39a ± 0.02 |
28.49a ± 0.01 |
0.9165 |
Ch3 |
33.88c ± 0.02 |
32.14b ± 0.02 |
31.29b ± 0.02 |
29.39a ± 0.02 |
28.49a ± 0.01 |
0.9677 |
Epicatechin content [g per 100 g d.w.]
|
Control |
4.70b ± 0.03 |
3.79c ± 0.07 |
3.20b ± 0.06 |
3.32b ± 0.02 |
3.29a ± 0.01 |
0.6334 |
Ch1 |
4.76e ± 0.07 |
3.78d ± 0.03 |
3.65c ± 0.03 |
3.53b ± 0.02 |
3.45a ± 0.03 |
0.6776 |
Ch2 |
4.60a ± 0.02 |
3.88d ± 0.05 |
3.13e ± 0.02 |
3.24f ± 0.05 |
3.45h ± 0.04 |
0.5302 |
Ch3 |
4.88c ± 0.03 |
3.74b ± 0.06 |
3.29a ± 0.08 |
3.39a ± 0.03 |
3.49a ± 0.04 |
0.5181 |
Catechin content [g per 100 g d.w.]
|
Control |
3.22d ± 0.01 |
2.29c ± 0.04 |
2.11b ± 0.07 |
2.08b ± 0.02 |
1.69a ± 0.06 |
0.8005 |
Ch1 |
3.33d ± 0.06 |
2.12c ± 0.06 |
2.07b ± 0.03 |
2.01b ± 0.05 |
1.79a ± 0.03 |
0.6487 |
Ch2 |
3.35d ± 0.02 |
2.55c ± 0.02 |
2.56c ± 0.06 |
2.21b ± 0.08 |
1.98a ± 0.02 |
0.8554 |
Ch3 |
3.36b ± 0.01 |
2.65c ± 0.08 |
2.41c ± 0.06 |
2.16b ± 0.02 |
1.09a ± 0.01 |
0.9570 |
(−)-Epigallocatechin gallate content [g per 100 g d.w.]
|
Control |
2.31c ± 0.02 |
2.18b ± 0.01 |
2.13b ± 0.08 |
2.02b ± 0.03 |
1.42a ± 0.03 |
0.8511 |
Ch1 |
2.21c ± 0.02 |
2.21c ± 0.01 |
2.16b ± 0.08 |
2.11b ± 0.03 |
1.66a ± 0.03 |
0.7456 |
Ch2 |
2.27c ± 0.02 |
2.23c ± 0.01 |
2.18b ± 0.08 |
2.17b ± 0.03 |
1.55a ± 0.03 |
0.7141 |
Ch3 |
2.17b ± 0.02 |
2.14b ± 0.04 |
2.10b ± 0.08 |
1.94b ± 0.03 |
1.53a ± 0.03 |
0.8456 |
In 2011, B. coagulans GBI-30, 6086 was classified by the Food and Drug Administration (FDA) as GRAS, that is, Generally Recognized As Safe; thus, it can be used as an ingredient of food products. The literature contains reports describing the addition of probiotic bacteria to the food matrix of chocolate.7,32–34 However, all data are related to the addition of lactic acid bacteria. There have been no reports on the addition of Bacillus bacteria to chocolate. For a bacterial strain to be classified as probiotic, it must satisfy the strictly determined characteristics.20,35 One of the major arguments for the classification of the strain as a health-promoting strain is its resistance to the harsh conditions prevailing in the gastrointestinal tract and its qualitative stability (including maintaining the counts and cell survival) during storage under various temperature conditions.36–39 For the new strain of B. coagulans GBI-30, 6086, with probiotic potential, the assessment of sensitivity to simulated digestion conditions was reported by Hyronimus et al.10 These authors tested the influence of low pH and various concentrations of bile salts on 13 different bacterial strains, of which B. laevolacticus DSM 6475 and all strains from the genus Sporolactobacillus (with the exception of S. racemicus IAM 12395) were resistant to pH 3.0. In contrast, only B. racemilacticus and B. coagulans tolerated the bile concentration in an excess of 0.3% (v/v). Other authors tested the tolerance of probiotic L. brevis subsp. coagulans to in vitro digestive juice.7,40 The efficiency of bacterial survivability subject to digestion, which is found in chocolate, was higher than that of the lyophilizate of the bacteria subject to digestion. The authors proved that chocolate constitutes a good matrix for probiotic bacteria, because of which they are delivered to the large intestine in good condition, thus fulfilling their health-promoting function that results in probiotics being delivered to the intestine in good condition and are efficient for the host's health. Similar results were obtained in the present study. In the case of the lyophilizate, the bacterial count was reduced from 107 (count prior to digestion) to 104 CFU g−1 (count after the last stage of digestion) (Table 4). Another study reported the results of in vivo tests, where patients consumed chocolate with L. brevis NTT001 and suffered from constipation.7,40 Results of experiments conducted enabled the determination of the higher count of Lactobacillus bacteria in the feces and the higher concentration of acetic acid in the test group patients who consumed probiotic chocolate, compared to the control group who consumed chocolate without the addition of bacteria.
Table 4 Number of bacteria of the Bacillus genus in probiotic preparation, in a simulated gastrointestinal tract
Measurement point |
Incubation time (h) |
pH |
Bacillus genus bacteria total count |
LB* |
Ch1 |
Ch2 |
Ch3 |
CFU g−1 |
(LB) lyophilizate of probiotic B. coagulans in PBS buffer; (Ch1) chocolates with the lyophilizate of probiotic B. coagulans – 106 CFU g−1; (Ch2) chocolates with the lyophilizate of probiotic B. coagulans – 107 CFU g−1; and (Ch3) chocolates with the lyophilizate of probiotic B. coagulans – 108 CFU g−1. |
P1 |
0 |
4.0 |
2.2 × 107 |
5.8 × 106 |
5.6 × 107 |
1.9 × 108 |
P2 |
4 |
4.0 |
2.1 × 104 |
4.2 × 105 |
2.6 × 106 |
9.2 × 106 |
P3 |
1.4 |
6.0 |
4.5 × 104 |
2.7 × 105 |
1.2 × 106 |
2.8 × 106 |
P4 |
2 |
7.4 |
5.2 × 104 |
3.1 × 107 |
5.2 × 108 |
3.2 × 108 |
P5 |
18 |
8.0 |
2.1 × 104 |
5.8 × 107 |
6.3 × 109 |
2.2 × 1010 |
Another criterion in screening tests aiming at proving the probiotic potential of new strains is demonstration of their high survivability rate during storage.5 The authors developed a prototype of milk chocolate with the addition of L. casei and L. paracasei species. They demonstrated that such chocolates possessed identical sensory properties to chocolates without the addition of probiotics. Moreover, the count of live bacterial cells remained at the level of 106–108 CFU g−1 for a period of 12 months. Similarly, in the present study (Fig. 3), the highest count of live probiotic bacteria was found in chocolate stored at 4 °C. Undoubtedly, the low water content of chocolate, as well as the presence of protective substances such as milk proteins and sugars, contributed to the high stability of chocolate products containing probiotic bacteria. The above characteristics of B. coagulans GBI-30, 6086 are closely associated with the capacity to form endospores by representatives of this bacterial genus.10,41 The formation of spores may be both a desirable and an undesirable characteristic in the technical context. Resistance to the influence of high or low temperature, intensive mixing, and variable pH during the course to obtain the final product, as well as maintaining viability during storage (extended shelf life) and transport are indisputable factors for the capacity to create a dormant form. However, from the viewpoint of hygiene processes or a change in the direction of technology (including the use of a specific or different species), the elimination of the microflora of the genus Bacillus may constitute an impediment.
Conclusions
We managed to produce a novel type of probiotic chocolate with comparative concentrations of active compounds. The addition of probiotic strains to chocolate does not affect its quality as measured from the water activity, acidity, and polyphenol content. The study results indicate that dark chocolate constitutes a suitable matrix for B. coagulans probiotic bacteria, and the sensory characteristics of probiotic chocolate are similar to those of the traditional product. Moreover, the high survival rate of bacteria contained in chocolate after passing through the simulated digestion conditions of the gastrointestinal tract and during storage of a ready product allows us to confirm that probiotic chocolate with B. coagulans bacteria may constitute a new alternative as a probiotic supplement in diet, especially for children.
Conflicts of interest
There are no conflicts to declare.
Funding source
This work was financed and developed as a project: POIR.04.01.02-00-0059/17.
Notes and references
-
I. McFarlane, in Industrial Chocolate Manufacture and use, ed. S. T. Beckett, Chapman and Hall, New York, 2nd edn, 1994, p. 314 Search PubMed.
- K. A. Cooper, J. L. Donovan, A. L. Waterhouse and G. Williamson, Cocoa and health: a decade of research, Br. J. Nutr., 2008, 99, 1–11 CrossRef CAS PubMed.
- D. Ackar, K. Valek Lendić, M. Valek, D. Šubarić, B. Miličević, J. Babić and I. Nedić, Cocoa Polyphenols: Can We Consider Cocoa and Chocolate as Potential Functional Food?, J. Chem., 2013, 2013, 1–7 CrossRef.
- L. Cinquanta, C. Di Cesare, R. Manoni, A. Piano, P. Roberti and G. Salvatori, Mineral essential elements for nutrition in different chocolate products, Int. J. Food Sci. Nutr., 2016, 67, 773–778 CrossRef CAS PubMed.
- D. Żyżelewicz, E. Nebesny, I. Motyl and Z. Libudzisz, Effect of milk chocolate supplementation with lyophilised Lactobacillus cells on its attributes, Czech J. Food Sci., 2010, 28, 392–406 CrossRef.
- Y. Yonejima, K. Hisa, M. Kawaguchi, H. Ashitani, T. Koyama, Y. Usamikrank, N. Kishida, S. Kishino and J. Ogawa, Lactic acid bacteria-containing chocolate as a practical probiotic product with increased acid tolerance, Biocatal. Agric. Biotechnol., 2015, 4, 773–777 CrossRef.
- H. Higuchi, K. Yoshida, K. Osawa, Y. Yonejima, K. Hisa and Y. Iwama, Effect of chocolate containing Lactobacillus brevis, NTT001 on intestinal environment - A randomized, double-blind, placebo-controlled, crossover trial, Jpn. Pharmacol. Ther., 2018, 46, 549–560 Search PubMed.
- W. B. Sarles and B. W. Hammer, Observations on Bacillus coagulans, J. Bacteriol., 1932, 23, 301–314 CAS.
- H. Honda, L. Hoyles, G. R. Gibson, S. Farmer, D. Keller and A. Mccartney, Impact of GanedenBC30 (Bacillus coagulans GBI-30, 6086) on population dynamics of the human gut microbiota in a continuous culture fermentation system., Int. J. Probiotics Prebiotics, 2011, 6, 65–72 Search PubMed.
- B. Hyronimus, C. Le Marrec, A. Hadj Sassi and A. Deschamps, Acid and bile tolerance of spore-forming lactic acid bacteria, Int. J. Food Microbiol., 2000, 61, 193–197 CrossRef CAS PubMed.
- R. Jäger, K. A. Shields, R. P. Lowery, E. O. De Souza, J. M. Partl, C. Hollmer, M. Purpura and J. M. Wilson, Probiotic Bacillus coagulans GBI-30, 6086 reduces exercise-induced muscle damage and increases recovery, PeerJ, 2016, 4, 2276 CrossRef PubMed.
- A. Belščak-Cvitanović, D. Komes, K. Durgo, A. Vojvodić and A. Bušić, Nettle (Urtica dioica L.) extracts as functional ingredients for production of chocolates with improved bioactive composition and sensory properties, J. Food Sci. Technol., 2015, 52, 7723–7734 CrossRef PubMed.
- G. E. Adamson, S. A. Lazarus, A. E. Mitchell, R. L. Prior, G. Cao, P. H. Jacobs, B. G. Kremers, J. F. Hammerstone, R. B. Rucker, K. A. Ritter and H. H. Schmitz, HPLC Method for the Quantification of Procyanidins in Cocoa and Chocolate Samples and Correlation to Total Antioxidant Capacity, J. Agric. Food Chem., 1999, 47, 4184–4188 CrossRef CAS PubMed.
- H. Wang, Determination of rosmarinic acid and caffeic acid in aromatic herbs by HPLC, Food Chem., 2004, 87, 307–311 CrossRef CAS.
- B. Kulczyński, J. Kobus-Cisowska, D. Kmiecik, A. Gramza-Michałowska, D. Golczak and J. Korczak, Antiradical capacity and polyphenol composition of asparagus spears varieties cultivated under different sunlight conditions, Acta Sci. Pol., Technol. Aliment., 2016, 15, 267–279 CrossRef PubMed.
- L. M. Nightingale, S.-Y. Lee and N. J. Engeseth, Impact of Storage on Dark Chocolate: Texture and Polymorphic Changes, J. Food Sci., 2011, 76, 142–153 CrossRef PubMed.
- L. Svanberg, N. Lorén and L. Ahrné, Chocolate Swelling during Storage Caused by Fat or Moisture Migration, J. Food Sci., 2012, 77, 328–334 CrossRef PubMed.
- V. Ghosh, G. R. Ziegler and R. C. Anantheswaran, Fat, Moisture, and Ethanol Migration through Chocolates and Confectionary Coatings, Crit. Rev. Food Sci. Nutr., 2002, 42, 583–626 CrossRef CAS PubMed.
- S. S. Kim, S. Y. Kim, D. W. Kim, S. G. Shin and K. S. Chang, Moisture Sorption Characteristics of Composite Foods Filled with Chocolate, J. Food Sci., 1999, 64, 300–302 CrossRef CAS.
- J. R. Endres, A. Clewell, K. A. Jade, T. Farber, J. Hauswirth and A. G. Schauss, Safety assessment of a proprietary preparation of a novel Probiotic, Bacillus coagulans, as a food ingredient, Food Chem. Toxicol., 2009, 47, 1231–1238 CrossRef CAS PubMed.
- D. B. Zarić, M. L. Bulatović, M. B. Rakin, T.Ž. Krunić, I. S. Lončarević and B. S. Pajin, Functional, rheological and sensory properties of probiotic milk chocolate produced in a ball mill, RSC Adv., 2016, 6, 13934–13941 RSC.
- J. Peres, E. Esmerino, A. L. da Silva, I. Racowski and H. Bolini, Sensory Profile, Drivers of Liking, and Influence of Information on the Acceptance of Low-Calorie Synbiotic and Probiotic Chocolate Ice Cream, J. Food Sci., 2018, 83, 1350–1359 CrossRef CAS PubMed.
- S. Martini, A. Conte and D. Tagliazucchi, Comprehensive evaluation of phenolic profile in dark chocolate and dark chocolate enriched with Sakura green tea leaves or turmeric powder, Food Res. Int., 2018, 112, 1–16 CrossRef CAS PubMed.
- J. Wollgast and E. Anklam, Review on polyphenols in Theobroma cacao: changes in composition during the manufacture of chocolate and methodology for identification and quantification, Food Res. Int., 2000, 33, 423–447 CrossRef CAS.
- A. Tresserra-Rimbau, R. M. Lamuela-Raventos and J. J. Moreno, Polyphenols, food and pharma. Current knowledge and directions for future research, Biochem. Pharmacol., 2018, 156, 186–195 CrossRef CAS PubMed.
- S. Kamiloglu and E. Capanoglu, Investigating the in vitro bioaccessibility of polyphenols in fresh and sun-dried figs (Ficus carica L.), Int. J. Food Sci. Technol., 2013, 48, 2621–2629 CrossRef CAS.
- J. Kobus-Cisowska, D. Szymanowska, P. Maciejewska, D. Kmiecik, A. Gramza-Michałowska, B. Kulczyński and J. Cielecka-Piontek,
In vitro, screening for acetylcholinesterase and butyrylcholinesterase inhibition and antimicrobial activity of chia seeds (Salvia hispanica), Electron. J. Biotechnol., 2019, 37, 1–10 CrossRef.
- T. Bohn, G. J. McDougall, A. Alegría, M. Alminger, E. Arrigoni, A.-M. Aura, C. Brito, A. Cilla, S. N. El, S. Karakaya, M. C. Martínez-Cuesta and C. N. Santos, Mind the gap-deficits in our knowledge of aspects impacting the bioavailability of phytochemicals and their metabolites - position paper focusing on carotenoids and polyphenols, Mol. Nutr. Food Res., 2015, 59, 1307–1323 CrossRef CAS PubMed.
- M. Serafini and A. Crozier, Milk and absorption of dietary flavanols, Nature, 2003, 426, 788–788 CrossRef CAS.
- M. Serafini, R. Bugianesi, G. Maiani, S. Valtuena, S. De Santis and A. Crozier, Plasma antioxidants from chocolate, Nature, 2003, 424, 1013–1013 CrossRef CAS PubMed.
- M. Gallo, G. Vinci, G. Graziani, C. De Simone and P. Ferranti, The interaction of cocoa polyphenols with milk proteins studied by proteomic techniques, Food Res. Int., 2013, 54, 406–415 CrossRef CAS.
- Ö. Erdem, M. Gültekin-Özgüven, I. Berktaş, S. Erşan, H. E. Tuna, A. Karadağ, B. Özçelik, G. Güneş and S. M. Cutting, Development of a novel synbiotic dark chocolate enriched with Bacillus indicus, HU36,
maltodextrin and lemon fiber: Optimization by response surface methodology, LWT – Food Sci. Technol., 2014, 56, 187–193 CrossRef.
- M. Maillard and A. Landuyt, Chocolate: An ideal carrier for probiotics, Agro Food Ind. Hi-Tech, 2008, 19, 13–15 CAS.
- J. Laličić-Petronijević, J. Popov-Raljić, D. Obradović, Z. Radulović, D. Paunović, M. Petrušić and L. Pezo, Viability of probiotic strains Lactobacillus acidophilus NCFM® and Bifidobacterium lactis, HN019 and their impact on sensory and rheological properties of milk and dark chocolates during storage for 180 days, J. Funct. Foods, 2015, 15, 541–550 CrossRef.
- E. Tuomola, E. Isolauri, S. Salminen, R. Crittenden and M. Playne, Quality assurance criteria for probiotic bacteria., Am. J. Clin. Nutr., 2001, 73, 393s–398s CrossRef CAS PubMed.
- S. J. Hur, B. O. Lim, E. A. Decker and D. J. McClements,
In vitro human digestion models for food applications, Food Chem., 2011, 125, 1–12 CrossRef CAS.
- D. Charalampopoulos, S. S. Pandiella and C. Webb, Evaluation of the effect of malt, wheat and barley extracts on the viability of potentially probiotic lactic acid bacteria under acidic conditions, Int. J. Food Microbiol., 2003, 82, 133–141 CrossRef CAS PubMed.
- H. Kimoto-Nira, C. Suzuki, K. Sasaki, M. Kobayashi and K. Mizumachi, Survival of a Lactococcus lactis strain varies with its carbohydrate preference under in vitro conditions simulated gastrointestinal tract, Int. J. Food Microbiol., 2010, 143, 226–229 CrossRef CAS PubMed.
- X. Pan, F. Chen, T. Wu, H. Tang and Z. Zhao, The acid, bile tolerance and antimicrobial property of Lactobacillus acidophilus, NIT, Food Control, 2009, 20, 598–602 CrossRef CAS.
- H. Higuchi, K. Yoshida, K. Osawa, Y. Yonejima, K. Hisa and Y. Iwama, Effect of chocolate containing Lactobacillus brevis NTT001 on defecation - A randomized, double-blind, placebo-controlled, parallel-group trial, Jpn. Pharmacol. Ther., 2017, 45, 463–472 Search PubMed.
- M. E. Sanders, L. Morelli and T. A. Tompkins, Sporeformers as Human Probiotics: Bacillus, Sporolactobacillus, and Brevibacillus, Compr. Rev. Food Sci. Food Saf., 2003, 2, 101–110 CrossRef.
|
This journal is © The Royal Society of Chemistry 2019 |