DOI:
10.1039/C8FO00173A
(Paper)
Food Funct., 2019,
10, 4854-4860
Chia seed mucilage – a vegan thickener: isolation, tailoring viscoelasticity and rehydration
Received
26th January 2018
, Accepted 13th July 2019
First published on 15th July 2019
Abstract
Chia seeds and their mucilage gels provide a nutritionally and functionally promising ingredient for the food and pharmaceutical industry. Application and utilization of the gel remain limited due to the tightly adhesion of the mucilage to the seeds, which affects the organoleptic properties, control of concentration and structuring possibilities. To exploit the full potential of chia mucilage gels as a functional ingredient calls for separation and purification of the gel. Herein, the gel was extracted by centrifugation and characterized rheologically and microscopically to link the viscoelastic properties to the structural properties. Subsequently, the gel was dried employing three different methods for facilitated storage and prolonged shelf life. The dried gels were readily soluble and its viscoelastic properties were fully regenerated upon rehydration demonstrating its potential to envisage industrial applications. The viscoelastic chia mucilage demonstrated shear-thinning behavior with complete relaxation upon stress removal. The gel's elasticity was enhanced with increasing mucilage concentration resulting in a highly tunable system. The extractable and rehydratable functional chia gel is a viable candidate as additive for the development of products requiring specific viscoelastic properties. Addition of the gel enhances the nutritional profile without interfering with the organoleptic properties.
Introduction
Chia (Salvia hispanica L. ) is experiencing an enormous revival after it was consumed centuries ago as staple food by the Mayas and Aztecs in Central and North America falling into oblivion after the Spanish conquest.1,2 This “superfood” is encountered in manifold food products ranging from baked goods over cereal bars, yoghurt-based breakfast formulations and smoothies but can also be obtained in its pure form of seeds. The recently gained recognition derives from their outstanding nutritional profile. Chia seeds provide a great source of ω-3 and ω-6 fatty acids, proteins of high biological value, soluble and insoluble fibers, antioxidants, vitamins and minerals.3–9 Moreover, it has been reported that the seeds are capable of preventing inflammatory disorders, heart and cardiovascular diseases, diabetes and protect the central nervous system.3,10–14 However, chia seeds are not only striking due to their nutritional value but also due to their ability to exudate a mucilage layer when in contact with water. This mucilage bears promising high water absorption capacity for food and pharmaceutical applications.15
Mucilages are water-soluble polysaccharides and are produced by several plants, algae and microorganism species.6,16 Upon hydration a hydrogel network is formed, which is governed by hydrophilic functional groups attached to the polymeric backbone of the polysaccharide.17 Such networks are capable of absorbing large quantities of water due to swelling and can be used in emulsifying and foaming processes.
Chia mucilage gels have been previously described by several authors.16,18–20 The polysaccharides that make up the mucilage network are located in the outer three seed coat layers forming the testa. They are among others composed of β-D-xylopyranosyl, α-D-glucopyranosyl and 4-O-methyl-α-D-glucopyranosyluronic acid.16,21 Upon addition of water the polysaccharides exudate, absorb water and unravel to full extension. The mucilage in contrast to other seeds including flax remains attached to the seed with remarkable tenacity. Application and utilization of the gel therefore remain limited owing to the adhering seed, which requires extensive extraction procedures. Nevertheless chia mucilage gels provide a promising material for the food industry. In contrasts to common vegan thickeners such as alginate, polyvinyl-alcohol and carrageenan, chia mucilage is biodegradable and digestible. To exploit the full potential of chia mucilage as functional ingredient the gel needs to separated by e.g. hydration, freeze-drying, rubbing of the gel and purification.21–23 All methods are laborious and extraction, purification, drying and other modification processes can significantly affect the molecular structure of the natural biopolymers. Moreover, most methods were focusing on the soluble fraction of the chia mucilage. To overcome these shortcomings a simplified extraction procedure was applied envisaging industrial applicability. The proposed extraction procedure based on centrifugation and allows to extract both the soluble and non-soluble gel fraction. The freshly extracted gel was characterized both microscopically and rheologically aiming at linking its viscoelastic properties to the underlying structure. Since the drying process can have a significant influence on the rehydration ability, three different drying approaches were investigated: rotary evaporation, oven- and freeze-drying. The rehydration potential was evaluated based the gels’ viscoelastic properties. Furthermore, different concentrations and resulting viscoelastic gel properties were elucidated to define tenability envisaging industrial application.
Materials and methods
Chia seed characterization
Chia seeds were purchased from MeaVita (Bio Chia Seeds from Mexico, DE-ÖKO-037) and used for all trials. A general characterization of the seed was carried out determining their moisture content, water hydration capacity and swelling volume. All measurements were conducted in triplicates and the average was reported.
Moisture content.
The determination of the moisture content was conducted with a Halogen Moisture Analyzer (HR73 Mettler Toledo, Switzerland). 5 g seeds where weighed and placed into the aluminum dish of the moisture analyzer. The seeds were dried for 50 min at 110 °C allowing to reach a constant weight. The moisture content on dry bases MCdb was calculated according to the following equation:
Water holding capacity.
To determine the general water holding capacity of the seeds, the standardized AACC International protocol 56-30.01 was applied in an adapted version.24 The seeds were placed in 50 mL centrifugal tubes and water (25 °C) was added to saturate the sample. The tubes were slightly centrifuged followed by discarding of the supernatant and calculation of the water holding capacity WHC:
Gel swelling volume.
The swelling was determined as an additional possibility to characterize the water holding capacity of the seeds, by regarding the volume of swelling. The determination was conducted by the AACC International Method 56-21-01.25 It was performed as described in the method by adding water, applying a standardized heat treatment, subsequent centrifugation and measurement of the resulting gel height. The swelling volume SSV was calculated using the following equation:
where the constant values derive from calibration of the employed 15 mL falcon tubes.
Mucilage formation and gel extraction procedure
For mucilage gel extraction chia seeds were hydrated in water at a 1
:
20 ratio at 25 °C and mixed on a magnetic stirrer for 2 h ensuring complete hydration (see Fig. 1). The gel extraction was carried out by centrifugation of the viscous solution obtained during hydration. The tubes were centrifuged at 6600g for 50 minutes and after centrifugation three different layers were distinguishable in the test tubes. The top layer of seeds and excess water including some of the soluble polysaccharide fraction was removed. The mucilage gel was collected with a spatula and either used fresh or stabilized by the different drying methods. The remaining chia seeds at the bottom of the test tubes with some remaining mucilage were discarded.
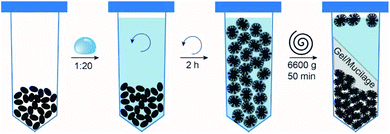 |
| Fig. 1 Chia mucilage formation and extraction procedure. Chia seeds were hydrated at 1 : 20 ratio and stirred for two hours to allow gel formation. Extraction was performed by centrifugation at 6600g for 50 minutes. Three different layers were obtained after centrifugation. The different layers were separated and only the gel layer was collected. | |
Gel stabilization by drying
As the extracted chia gels are of short shelf life due to microbiological stresses, drying improves both shelf life as well as handling. Herein, three different drying methods were investigated as well as the respective rehydration potential of the gels.
Oven drying.
By drying the gel in an oven, the effect of heating by convection and conduction were studied. Extracted gel was weighed and spread on a baking sheet covered tray. The gels were dried at 50 °C, with a 10% ventilation and 10% latch opening for 8 h (UF 110 Plus Dryer, Memmert GmbH+Co. KG, Schwabach, Germany). The dried gel film was removed from the baking sheet for storage and later use.
Rotary evaporation.
For rotary evaporation the gels were weighed into round-bottom flasks attached to the rotary evaporator (Hei-VAP Value, Heidolph Instruments GmbH+Co. KG, Schwabach, Germany). Vacuum was first lowered to 100 mbar, subsequently to 72 mbar and finally to 22 mbar, allowing the sample to stabilize at applied pressure. The sample was rotated at 100 rpm in a water bath of 45 °C until the gel was completely dry. The glass flask with dried gel film was closed and stored at 21 °C until further utilization.
Freeze-drying.
During freeze-drying, direct sublimation of water from the gel was achieved by shock freezing the gel samples in 125 mL plastic containers with liquid nitrogen and subsequently placing them in the lyophilisator (BenchTop Pro with Omnitronis, SP Scientific, Gardiner, NY, USA). A vacuum pressure of 512 μPa was applied and samples were left for 48 h until completely dried. The dried foams were stored at 21 °C in airtight plastic containers.
Gel rehydration
To elucidate the effect of the different drying methods on the rehydration potential, the dried samples were rehydrated to concentrations of 0.3% (w/w) corresponding to the initial concentration and 2 or 4% (w/w) to investigate higher concentrated solutions. The water (25 °C) was added to the dried gel and stirred with a magnetic stirrer for 1 h ensuring complete dissolution.
Rheological gel characterization
The rheological properties of chia gels were studied by shear, frequency and amplitude sweeps using a MCR 300 Rheometer (Anton Paar GmbH, Graz, Austria). The rheometer was equipped with a TEK 150-P measuring cell and Couette geometry CC27. The temperature was kept constant at 25 °C through a Peltier Element. Shear rate measurements were conducted at shear rates from 0.1 to 100 1 s−1. Frequency sweeps were carried out at frequencies of 0.1 to 100 rad s−1 at an amplitude of 1%. For the amplitude sweeps deformations of 0.1 to 100% were applied at a frequency of 1 rad s−1. Rheological measurements were performed for the freshly extracted gel and the hydrated gel. All experiments were conducted in triplicates.
Microscopic gel characterisation
The viscoelastic behavior and functional properties are closely linked to the underlying gel structure. To investigate the structure and orientation of mucilage strands samples were analyzed with an inverse light microscope (NIKON Diaphot-TMD). Pictures were taken and analyzed with NIS Elements Software. The structure of the gel was investigated after the individual processing steps, i.e. after hydration, extraction, drying and rehydration. The gel was stained with crystal violet (Sigma Aldrich) or Congo red (Alfa Aesar, Thermo Fischer) to dye the polysaccharide strand.
Results and discussion
Seed properties
Chia seed properties were characterized serving as basic classification of the raw material. The dried chia seeds came at a moisture content of 8% db going in line with the values reported by Ixtaina et al.6,7 Mucilage formation was initiated with addition of water demonstrating a fast unraveling in the first 5 min and further expansion endured up to 30 min from the water addition.15 The hydrated seeds reached water hydration capacities and swelling volumes of 11.7 and 16.0 mL g−1 seed. Higher water holding capacities were observed by other research groups focusing solely on the fiber rich part of the seed.26,27 The fiber rich part is considered as being responsible for the water absorption capacity explaining the differences in values determined.
Microscopic gel characterization
Chia gel structure was investigated microscopically after the individual processing steps unraveling the optical properties and network structure of the mucilage strands. Initially hydrated, the gel formed a transparent layer surrounding the seed. The mucilage strands of the gel were highly oriented showing no entanglement or overlap and were tightly attached to the seed surface (Fig. 2A). Extraction yielded a clear gel of concentrated mucilage strands. Due to the centrifugal extraction the highly ordered structure of the initial gel was lost resulting in a randomly oriented polymer network (Fig. 2B).
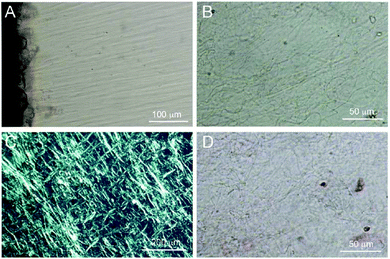 |
| Fig. 2 Structure of the freshly extracted and rehydrated chia gels. (A) Highly ordered chia mucilage strands attached to the seed, which can be seen at the left edge of the image. (B) Freshly extracted gels show overlapping and interacting strands. (C) Freeze-dried gels (under polarized light) demonstrate the highly concentrated system. Multiple layers of mucilage strands came to lie on top of each other. (D) Rehydrated samples show similar structural features as the freshly extracted sample. | |
In the dried state the gel is at its most concentrated state. Appearance of the gel was dictated by the drying method applied. Transparent and shiny films were obtained after oven drying or rotary evaporation, whereas freeze-drying generated voluminous, white foam-like structures. Despite their differing optical properties, the dry mucilage structure revealed a highly concentrated system with overlapping layers of mucilage strands (Fig. 2C). Examination of the rehydrated gel at initial concentration of 0.3% (w/w) resulted in comparable gel properties and structure (Fig. 2D). Consequently, the extraction resulted in a loss of mucilage strand order. The initial order might derive from volume depletion, repulsive forces amongst the negatively charged strands, or forced order due to the tight attachment to the seed. However, the applied drying and rehydration steps do not to interfere with the mucilage strands indicating macroscopic structure preservation.
Shear rheological properties of freshly extracted and rehydrated gels
The flow behavior of the extracted gel was characterized by shear rate tests as well as frequency and amplitude sweeps. The freshly extracted gel served as reference for the dried and rehydrated gels. After extraction the fresh gel yield a solid concentration of around 0.3% (w/w). The gel demonstrated a shear thinning behavior at shear rates between 0.1 and 100 1 s−1 (Fig. 3, red curve). There results are in line with the findings of Capitani et al. determining slightly higher viscosity values for gel at a concentration of 0.25% (w/w) whereas comparable behavior was observed by Timilsena et al. for 0.02% (w/w) chia polysaccharide solution.22,23 The shear thinning behavior is caused by shear induced ordering of the polymer chains parallel to the flow direction causing a viscosity decrease due to lesser interactions amongst the chains.21,28–30
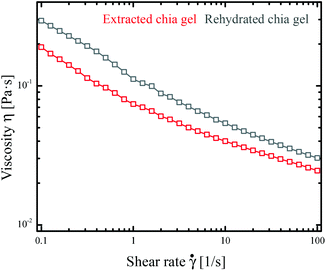 |
| Fig. 3 Shear rheological characterization of the extracted and rehydrated chia mucilage gels. The shear viscosity η of the gel is plotted as a function of the shear rate γ. The freshly extracted and rotary evaporated and rehydrated sample demonstrated shear-thinning behavior (mucilage concentration of 0.3% (w/w)). | |
The freshly extracted gel was dried by rotary evaporation, oven and freeze-drying aiming towards the most suitable method for a rehydratable gel while keeping the structure and functionality of the fresh gel. The rehydrated gel shows comparable rheological behavior to the fresh gel as depicted in Fig. 3 (grey curve). Upon shearing the gel demonstrated the same shear thinning behavior over all shear rates. Slightly higher viscosity values were observed for samples dried by rotary evaporation, which is attributable to a slightly larger solid concentration after rehydration and denser network structure restricting the intermolecular motion.27,31
Viscoelastic properties of rehydrated gels
The viscoelastic properties of chia mucilage gels were investigated by frequency and amplitude sweeps. For freshly extracted and rehydrated gels at the natural mucilage concentration of about 0.3% (w/w) both torque sensitivity as well as inertia effects compromise the rheological data. However, extracted or rehydrated mucilage gels show weak-gel properties, which is in line with the shear thinning behavior reported in the shear rate experiments. Similar behavior was reported for the soluble gel fraction and for the entire gel.21–23
Owing to the detection limit of the rheometer at the natural concentration, frequency sweeps were performed at a concentration of 2% (w/w) for rehydrated mucilage samples (Fig. 4). All rehydrated samples, regardless of the drying process showed viscoelastic behavior. Highest G′ and G′′ values were obtained for the freeze-dried followed by the rotary evaporated and the oven dried sample. At low frequencies, the storage modulus G′ was larger than the loss modulus G′′ indicating the weak-gel character of the samples. Both values increased with increasing frequency with G′′ becoming dominate at higher frequencies, i.e. after the crossing point. The respective crossover points of G′ and G′′ are 1 rad s−1 for rotary evaporation, 5 rad s−1 for freeze-dried, and 11 rad s−1 for oven dried samples. The complex viscosity exhibited a shear thinning behavior for all samples. In conclusion, the drying methods influence the mucilage functionality, entanglement properties, average molar mass and thus the gel properties. Freeze-drying enabled preservation of the initial gel structure throughout drying. However, water crystals forming upon freezing could have affected network structure decreasing its stability. Rotary evaporation is regarded as very gentle process compacting the gel under rotational movement and might hence have had relatively little impact in the structure and mucilage functionality. Oven treatment is by far the most severe treatment. At temperatures of 50 °C denaturation of protein might have occurred affecting the molar mass of the mucilage strands. Higher angular frequencies at the crossover point can be attributed to a lower average molar mass, which could indicate an induced alteration of the molecules.32 The mucilage strands appeared to be relatively resistant to the applied moderate heat treatments. Simple extraction with subsequent drying hence allows for improved storage and handling of the gel without compromising its functional properties and quality. This makes dried and rehydratable chia gel a viable candidate as additive for food production. Additionally, selecting different drying principles or further processing steps offer the possibility to tailor the viscoelastic behavior widening the frame of probable applications.
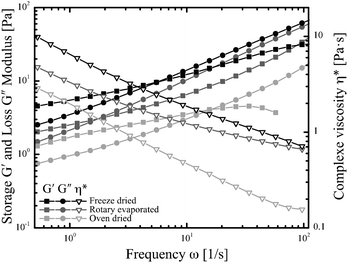 |
| Fig. 4 Viscoelastic behavior of the differently dried and rehydrated chia mucilage gels at concentrations of 2% (w/w). The complex viscosity η*, storage G′ and loss G′′ modulus are illustrated as a function of the frequency ω for the rehydrated gels. The highest complex viscosity, storage and loss moduli were observed for freeze-dried followed by rotary evaporated and oven dried samples. | |
Influence of mucilage concentration on gel properties
To elucidate the potential of the extracted chia gel for future applications, higher mucilage concentrations of 4% (w/w) were examined in frequency sweep tests (Fig. 5). In comparison the sample with mucilage concentrations of 2% (w/w) similar shear thinning behavior but higher values of the complex viscosity η* were observed, which agrees with the findings of Capitani et al.22 More distinct differences in the viscoelastic behavior were observed for the storage G′ and loss modulus G′′ with increasing mucilage concentration. First, larger storage and loss moduli were recorded, which has been also documented for several other biopolymers including xanthan gum, welan, and rhamsan.33 Second, the storage modulus G′ surpassed the loss modulus G′′ for sample with a mucilage concentration of 4% (w/w) indicating the dominate elasticity of the gel. And finally, the slopes of both moduli in double logarithmic representation indicate the transition from a weak-gel system (concentration of 2% (w/w)) to a fully cross-linked gel (concentration of 4% (w/w)). For weak-gel systems the slopes of the moduli are relative similar and in the order of ω0.5. Also, a dominant storage modulus at low frequencies can be observed for all samples as depicted in Fig. 4. In same case, the slope of G′ may level out to zero. Such low frequency plateau in G′ reflects the weak network structure of the sample, which will be damaged at higher frequencies and thus leading to dominant G′′ values as also seen in Fig. 4. With increasing concentration as shown in Fig. 5, more and more network points are established in the gel and, as a consequence, G′ becomes the dominant rheological parameter. The slope of about zero as seen in Fig. 5 for the 4% (w/w) sample indicates a gel- or rubber-like behavior. In summary, chia mucilage gels can be adjusted in their rheological behavior from liquid-like fluids to weak-gel structures to fully gelled samples by increasing the mucilage concentration. The adjustability of the viscoelastic behavior as a function of the concentration offers a promising tool for tailoring the properties of the resulting gel and food product.
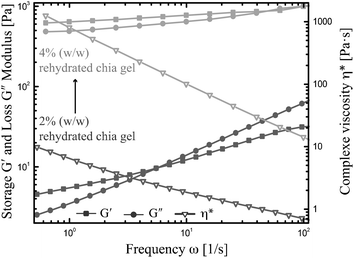 |
| Fig. 5 Viscoelastic behavior of chia mucilage gel at different concentrations. Complex viscosity η*, storage G′ and loss G′′ modulus as a function of frequency ω for rotary evaporated and rehydrated gels at concentrations of 2 and 4% (w/w). With increasing concentrations, G′ and G′′ are increased and a transition from weak-gel structures to gel- or rubber-like behavior is seen. | |
Conclusions
Chia seed mucilage bears a huge potential for application as vegan thickener in the food and pharmaceutical industry owing to its excellent nutritional profile and functional properties. Nonetheless, its application in bulk remains restricted due to the tight adhesion of the mucilage layer to the seed. Herein, chia seed mucilage was extracted employing a simple extraction approach envisaging industrial applications. The obtain freshly extracted and rehydrated gels were characterized rheologically and microscopically indicating a gel with randomly oriented mucilage strands giving the gel its unique viscoelastic properties. Upon rehydration, its properties were fully regenerated. The viscoelastic mucilage demonstrated shear-thinning behavior, which was enhanced with increasing mucilage concentration resulting in highly tunable systems. At >higher concentration, the drying procedure becomes important, i.e. is influencing the gel properties. Freeze-drying yielded the strongest gel probably due to structure preservation while harsher drying treatments in the oven reduced gel strength. Freshly extracted and rehydrated functional chia gel is a viable candidate as additive for the development of products requiring specific viscoelastic properties. Structure and functionality of the gel are tunable by the mucilage concentration and pre-treatment or drying procedure. Owing to its non-toxicity, biodegradability and digestibility chia gel provides a promising alternative to commercially available vegan thickeners such as polyvinyl alcohol, carrageenan and alginate. In the next steps, both incorporation of chia gels in existing or novel food matrices as well as their sensory properties will be exanimated.
Conflicts of interest
There are no conflicts to declare.
Acknowledgements
The authors acknowledge ETH Zurich for funding this project.
References
- A. G. Chicco, M. E. D'Alessandro, G. J. Hein, M. E. Oliva and Y. B. Lombardo, Dietary chia seed (Salvia hispanica L-) rich in α-linolenic acid improves adiposity and normalizes hypertriacylglycerolaemia and insulin resistance in dyslipaemic rats, Br. J. Nutr., 2009, 101, 41–50 CrossRef CAS PubMed.
- P. Peiretti and F. Gai, Fatty acid and nutritive quality of chia (salvia hispanica L.) seeds and plant during growth, Anim. Feed Sci. Technol., 2009, 148, 267–275 CrossRef CAS.
-
D. Armstrong, Application for approval of whole chia (Salvia hispanica L.) seed and ground whole seed as novel food ingredient, R. Craig & Sons Ltd, Antrim, Northern Ireland, 2004 Search PubMed.
- A. A. Bushway, P. R. Belyea and R. J. Bushaway, Chia seed as a source of oil, polysaccharide, and protein, J. Food Sci., 1981, 46, 1349–1350 CrossRef CAS.
- R. Ayerza, Oil content and fatty acid composition of chia (Salvia hispanica L.) from five northwestern locations in Argentina, J. Am. Oil Chem. Soc., 1995, 72, 1079–1081 CrossRef CAS.
- V. Y. Ixtaina, S. M. Nolasco and M. C. Tomas, Physical properties of chia (Salvia hispanica L.) seeds, Ind. Crops Prod., 2008, 28, 286–293 CrossRef.
- V. Y. Ixtaina, M. L. Martinez, V. Spotorno, C. M. Mateo, D. M. Maestri, B. W. K. Diehl, S. M. Nolasco and M. C. Tomas, Characterization of chia seed oils obtained by pressing and solvent extraction, J. Food Compos. Anal., 2011, 24, 166–174 CrossRef CAS.
- R. Ayerza and W. Coates, Some quality components of flour chia (Salvia hispanica L.) genotypes grown under tropical coastal desert ecosystem conditions, Asian J. Plant Sci., 2009, 8, 301–307 CrossRef CAS.
- M. I. Capitani, V. Spotorno, S. M. Nolasco and M. C. Tomas, Physicochemical and functional characterization of by-products from chia (Salvia hispanica L.) seeds of Argentina, LWT–Food Sci. Technol., 2012, 45, 94–102 CrossRef CAS.
- R. Ayerza and W. Coates, Ground chia seed and chia oil effects on plasma lipids and fatty acids in the rat, Nutr. Res., 2005, 25, 995–1003 CrossRef CAS.
- I. Fernández, R. Ayerza, W. Coates, S. M. Vidueiros, N. Slobodianik and A. N. Pallaro, Nutritional characteristics of chia, Actualización en Nutrición, 2006, 7, 23–25 Search PubMed.
- A. Tamargo, C. Cueva, L. Laguna, M. W. Moreno-Arribas and L. A. Munoz, Understanding the impact of chia seed mucilage on human gut microbiota by using the dynamic gastrointestinal model simgi (R), J. Funct. Foods, 2008, 50, 104–111 CrossRef.
- V. Vuksan, A. L. Jenkins, A. G. Dias, A. S. Lee, E. Jovanovski, A. L. Rogovik and A. Hanna, Reduction in postprandial glucose excursion and prolongation of satiety: possible explanation of the long-term effects of whole grain Salba (Salvia Hispanica L.), Eur. J. Clin. Nutr., 2010, 64, 436–438 CrossRef CAS PubMed.
- V. Vuksan, D. Whitman, J. Sievenpiper, A. Jenkins, A. Rogovik, R. Bazinet, E. Vidgen and A. Hanna, Supplementation of conventional therapy with the novel grain Salba (Salvia hispanica L.) improves major and emerging cardiovascular risk factors in type 2 diabetes, Diabetes Care, 2007, 30, 2804–2810 CrossRef CAS PubMed.
- L. A. Muñoz, A. Cobos, O. Diaz and J. M. Aguilera, Chia seed (Salvia hispanica): an ancient grain and new functional food, Food Rev. Int., 2013, 29, 394–408 CrossRef.
- K.-Y. Lin, J. R. Daniel and R. L. Whistler, Structure of chia seed polysaccharide exudate, Carbohydr. Polym., 1994, 23, 13–18 CrossRef CAS.
- B. Singh, G. S. Chauhan, S. Kumar and N. Chauhan, Synthesis, characterization and swelling responses of pH sensitive psyllium and polyacrylamide based hydrogels for the use in drug delivery (I), Carbohydr. Polym., 2007, 67, 190–200 CrossRef CAS.
- L. A. Muñoz, A. Cobos, O. Diaz and J. M. Aguilera, Chia seeds: Microstructure, mucilage extraction and hydration, J. Food Eng., 2012, 108, 216–224 CrossRef.
- R. Whistler, Industrial gums from plants: Guar and chia, Econ. Bot., 1982, 36, 195–202 CrossRef CAS.
- M. I. Capitani, V. Y. Ixtaina, S. M. Nolasco and M. C. Tomas, Microstructure, chemical composition and mucilage exudation of chia (Salvia hispanica L.) nutlets from Argentina, J. Sci. Food Agric., 2013, 93, 3856–3862 CrossRef CAS PubMed.
- K. K. T. Goh, L. Matia-Merino, J. H. Chiang, R. Quek, S. J. Bing Soh and R. G. Lentle, The physic-chemical properties of chia seed polysaccharide and its microgel dispersion rheology, Carbohydr. Polym., 2016, 149, 297–307 CrossRef CAS PubMed.
- M. I. Capitani, L. J. Corzo-Rios, L. A. Chel-Guerrero, D. A. Betancur-Ancona, S. M. Nolasco and M. C. Tomas, Rheological properties of aqueous dispersions of chia (Salvia hispanica L.) mucilage, J. Food Eng., 2015, 149, 70–77 CrossRef.
- Y. P. Timilsena, R. Adhikari, S. Kasapis and B. Adhikari, Rheology and microstructural properties of the chia seed polysaccharide, Int. J. Biol. Macromol., 2015, 81, 991–999 CrossRef CAS PubMed.
-
AACC International, Approved Methods of Analysis, 11th Ed. Method 56-30.01. Water Hydration Capacity of Protein Materials. AACC International, St. Paul, MN, U.S.A. Approved November 3, 1999 Search PubMed.
-
AACC International, Approved Methods of Analysis, 11th Ed. Method 56-21.01. Flour Swelling Volume. AACC International, St. Paul, MN, U.S.A. Approved October 17, 2001 Search PubMed.
- A. Vázquez-Ovando, J. G. Rosado Rubio, J. Gabriel, L. Chel-Guerrero and D. Betancur, Physiochemical properties of a fibrous fraction from chia (Salvia hispanica L.), LWT–Food Sci. Technol., 2009, 42, 168–173 CrossRef.
- Y. P. Timilsena, R. Adhikari, S. Kasapis and B. Adhikari, Molecular and functional characteristics of purified gum from Australian chia seeds, Carbohydr. Polym., 2016, 136, 128–136 CrossRef CAS PubMed.
- A. Koocheki, A. R. Taherian and A. Bostan, Studies on the steady shear flow behavior and functional properties of Lepidium parfoliatum seed gum, Food Res. Int., 2013, 50, 446–456 CrossRef CAS.
- A. Xiu, M. Zhou, B. Zhu, S. Wnag and J. Zhang, Rheological properties of Salecan as new source of thickening agent, Food Hydrocolloids, 2011, 25, 1719–1725 CrossRef CAS.
-
A. Nussinovitch and M. Hirashima, Cooking Innovations: Using Hydrocolloids for Thickening, Gelling and Emulsification, CRC Press, 2013 Search PubMed.
- M. Maskan and F. Gogus, Effect of sugar on the rheological properties of sunflower oil-water emulsions, J. Food Eng., 2000, 43, 173–177 CrossRef.
-
T. G. Mezger, Applied Rheology: With Joe Flow on Rheology Road, Anton Paar, Graz, 2nd edn, 2014 Search PubMed.
- E. R. Morris, M. G. E. Gothard, M. W. N. Hember, C. E. Manning and G. Robinson, Conformational and rheological transitions of welan, rhamsan and acylated gellan, Cabohydr. Polym., 1996, 27, 23–36 Search PubMed.
|
This journal is © The Royal Society of Chemistry 2019 |
Click here to see how this site uses Cookies. View our privacy policy here.