DOI:
10.1039/C8EN01089G
(Communication)
Environ. Sci.: Nano, 2019,
6, 60-67
Plant species-dependent transformation and translocation of ceria nanoparticles†
Received
27th September 2018
, Accepted 9th November 2018
First published on 13th November 2018
Abstract
Uptake of nanoparticles (NPs) by plants is species-dependent; however, its underlying mechanisms are rarely investigated. This study compared the transformation, uptake and translocation of CeO2 NPs (nCeO2) in four different plant species. Corn showed the lowest translocation factor (TF) of Ce, while the differences among the plant species reduced or diminished when the phosphates were removed from the nutrient solution (−P treatment). Using transmission electron microscopy (TEM) and synchrotron-based X-ray absorption near edge spectroscopy (XANES), we found that the removal of phosphate from the nutrient solutions reduces or eliminates the immobilization of Ce3+ on the root surface thus promoting the upward translocation of Ce from roots to shoots, which accounts for the enhanced total Ce detected in all the four plants. Compared with the +P treatment, the Ce(III) contents in the shoots of corn and wheat were enhanced by 27.4 an 4.0 times in the −P treatment, which may account for the reduced biomass of shoots in these two plant species. Our results indicate that different plant species have different abilities of transforming and translocating CeO2 NPs and that the mechanisms differ depending on the chemistry of the surrounding solution (+P/−P). Plant xylems and root exudate compositions are both important factors in determining the transformation of nCeO2 and subsequent translocation of Ce species in plants. Further studies are required to determine the composition of root exudates and to identify the components driving the nCeO2 transformation in different plant species under different culturing conditions.
Environmental significance
Transformation of nanomaterials (NMs) in plants is a critical process that may determine the final fate of NMs in the environment. We previously found that root surface is the major site for nCeO2 transformation. In this study, we compared the uptake and phytotoxicity of nCeO2 to two dicots and two monocots. Different plant species show different capacities to transform nCeO2 into Ce(III) species, which results in different translocation of Ce in plants. Removal of phosphate from the nutrient solution reduces or eliminates the immobilization of Ce3+ on the root surface thus promoting the upward translocation of Ce, which leads to the phytotoxicity found in the monocots. Plant xylems and root exudate compositions are both important factors in determining the transformation of nCeO2 and the subsequent translocation of Ce species in plants. This study reveals the underlying mechanism behind the plant species-dependent uptake and phytotoxicity of NMs in plants. Further studies are required to determine the composition of root exudates in different plant species and identify the determining components for nCeO2 transformation under different culturing conditions.
|
1. Introduction
CeO2 minerals were previously recognized as being highly stable in the environment; however, an increasing body of studies now suggest that CeO2 in its nano form is prone to transform in various environmental surroundings including aquatic mesocosms,1 wastewater treatment plants,2 activated sludge,3 synthetic river water,4 bacteria,5 plants,6etc., and various transformation products were reported depending on the ambient environment chemistry and biochemistry. For example, in an activated sludge where the sulphur concentration is high, Ce3+ ions might be released from nCeO2 upon bacterial reduction and complexation, forming Ce2(SO4)3 in oxidizing environments or forming Ce2S3 in reducing environments.3 In plants, low molecular organic acids and reducing substances excreted by plant roots can facilitate the release of Ce3+ ions from nCeO2 in the rhizosphere vicinity, which then can be translocated from roots to shoots and form either CePO4 or Ce carboyxlates.6–8 Retransformation of Ce3+ ions released from nCeO2 back to CeO2 is also possible.3,9 Transformation processes such as carboxylation/complexation facilitate the release and mobility of Ce(III) thus increasing the toxicity, while sulfidation or phosphorylation reduce the mobility and toxicity of NPs.10 However, re-dissolution of CePO4 is also possible in the presence of strong Ce3+ ion-binding ligands in the root exudates.11
nCeO2 transformation is highly associated with the physicochemical characteristics of nCeO2 such as surface coating,3 size,12 and shape.13 For example, surface coating with citric acid may act as a shield reducing the direct contact of the particle surface with the ambient environment and subsequent dissolution.3 Compared with larger size nCeO2, smaller size nCeO2 released more Ce(III) ions and thus induced higher toxicity to Lactuca plants.12 Rod-shaped nCeO2 with highly reactive crystal facets transformed to a greater extent in cucumber plants than other shapes of nCeO2 such as octahedra and truncated octahedra.13
Another important factor, i.e. the plant species, has been largely ignored in the study of the uptake and transformation of nCeO2 in plants. Previous studies have suggested that the uptake and translocation of NPs are highly dependent on plant species.9,14,15 The discrepancy might not be attributed only to the structural differences but also to the composition difference of the root exudates, considering that the root surface is the major site for the transformation of nCeO2 in plants.16
In this study, we exposed two monocots (corn and wheat) and two dicots (cabbage and soybean) to nCeO2 (2000 mg L−1) under two different hydroponic conditions (with and without phosphates in the nutrient solution, called “+P” and “−P” treatment, respectively), with the aim to understand how plant species and culturing conditions impact the transformation, uptake and translocation of nCeO2 by plants. To supply P for plant growth in the “−P” treatment, 1 mM KH2PO4 was sprayed onto the foliage of the plants every two days. Translocation and transformation of nCeO2 in plants were then examined by ICP-MS, TEM and X-ray absorption near edge spectroscopy (XANES).
2. Materials and methods
2.1. Materials, plant cultivation and exposure
nCeO2 with an average size of 25 nm (Fig. S1†) were synthesized by a precipitation method13 and the physicochemical properties of nCeO2 are described in Table S1.† Seeds of corn, wheat, cabbage and soybean purchased from the Chinese Academy of Agricultural Sciences were sterilized in a 5% NaClO solution and rinsed with deionized water. Then the seeds were placed on filter paper which was soaked with deionized water in a plastic Petri dish and allowed for germination in the dark for 5 days. The seedlings were then transferred into 200 mL beakers containing 100 mL ¼ strength Hoagland solution. In the −P treatment, nutrient solution without phosphate was used. To supply P for normal growth of plant, KH2PO4 (1 mM) solution was sprayed on the foliage of the plants every two days. The seedlings were allowed to grow for 10 days in an artificial climate chamber, and then exposed to 2000 mg L−1 nCeO2 suspension in ¼ strength Hoagland solution; 2000 mg L−1 was the maximum exposure concentration used in the phytotoxicity test established by the U.S. Environmental Protection Agency guideline.17 After 2 weeks of exposure, the seedlings were harvested, rinsed with deionized water and lyophilized for further analysis.
2.2. Phytotoxicity, Ce localization, quantification and chemical species analysis
The dry weights of the shoots and roots of the plants were measured for assessing the phytotoxicity. The root apexes were collected, fixed, dehydrated, embedded, and sectioned following the procedures for TEM observation as described previously.6 A JEM-1230 (JEOL) transmission electron microscope was used for observing the sections. The shoots and roots were ground into fine powders using a pestle and mortar. The powders were then digested with a mixture of nitric acids and hydrogen peroxide (v/v: 4/1), and the total Ce contents were analysed by ICP-MS (Thermo).
For XANES analyses, the fine powders of the shoots and roots were compressed into thin slices (∼2 mm). The Ce L-III edge spectra were collected using a 19-element high purity Ge solid state detector array at beamline 1W1B of the Beijing Synchrotron Radiation Facility. The XANES spectra of nCeO2 and Ce-containing compounds including CePO4, Ce acetate and Ce oxalate were collected for reference. Linear combination fitting (LCF) of the XANES data was performed on Athena software. LCF is justified because the X-ray adsorption of different species in the sample is additive.18 The total absorption coefficient calculated by LCF can be written as
where
M is the least squares fit to the sample spectrum, (STD
i) represents the absorption coefficient of the standard reference spectra, and
fi is the fraction of each reference spectrum in the sample spectrum, summed over the number of references, i. Thus, with appropriate standard references, LCF can identify and quantify the fraction of chemical species in an unknown sample.
3. Results and discussion
3.1. Plant species-dependent Ce uptake – influence of phosphate
Fig. S2† shows that the four plant species respond differently to nCeO2 exposure. In the +P treatment, nCeO2 increased the root biomass of soybean by 36%, but had no effect on the other plant species tested. In the −P treatment, the positive effects of nCeO2 on the soybean roots were remarkably enhanced, with the root biomass increased by 60% compared with that of the control, while nCeO2 still had no effect on the other plant species tested. nCeO2 had no significant effects on the shoot biomass of soybean and cabbage in the −P treatment; however, nCeO2 reduced the shoot biomass of corn and wheat by 24% and 63%, respectively, in the −P treatment. These results suggest that the phytotoxicity of nCeO2 is plant species-dependent and is influenced by the composition of the nutrient solution used in the hydroponic growth system.
Table 1 shows that the Ce uptake and translocation in the plants were also highly species-dependent. In the +P treatment, the Ce content in the plants and the TFs of the monocots (corn and wheat) were lower than those of the dicots (cabbage and soybean) except for wheat where the Ce content in the root is higher than those in the other plant species. This might be due to the fact that cabbage and soybean have larger leaf surface areas resulting in higher transpiration rates, because the driving force for long distance translocation of nutrients mainly depends on the transpiration rate.19
Table 1 Ce content (mg kg−1 dry weight) in the roots and shoots of the plants treated with nCeO2, and translocation factors (TFs, defined by dividing the Ce contents in the shoots by that in the roots) of Ce in the different plant species. Data are expressed as mean ± SD (n = 8). * indicates a significant difference between +P and −P treatment for each plant species (p < 0.05). Significant differences between different species in each treatment are compared separately and expressed as lowercase letters. Different superscript letters indicate a significant difference (p < 0.05)
|
Root |
Shoot |
TFs (×10−3) |
−P |
+P |
−P |
+P |
−P |
+P |
Corn |
68 093 ± 20 530a* |
12 499 ± 2600b |
158 ± 56a* |
5.6 ± 1.6c |
2.3 ± 0.8b* |
0.5 ± 0.1c |
Wheat |
39 269 ± 9278b |
34 322 ± 10 207a |
140 ± 45a* |
28 ± 11b |
3.6 ± 1.0b* |
0.8 ± 0.3b |
Cabbage |
58 315 ± 22 799ab |
31 418 ± 14 821a |
175 ± 44a* |
76 ± 15a |
3.0 ± 0.7b |
2.4 ± 0.4a |
Soybean |
35 003 ± 6615b |
31 527 ± 5669a |
181 ± 37a* |
77 ± 9a |
5.2 ± 0.5a* |
2.4 ± 0.2a |
The removal of phosphate (P) from the nutrient solution remarkably promoted the uptake of Ce into the roots of corn and cabbage and the upward translocation of Ce in all the four species. The Ce contents in the roots of corn and cabbage exposed under the −P treatment condition were 5.5 and 1.9 times higher, respectively, than those under the +P treatment. The Ce contents in the shoots of all the plant species were higher under the −P treatment than those under the +P treatment. In particular, the Ce contents in the shoots of corn, wheat, cabbage and soybean under the −P treatment were 28.4, 5.0, 2.3, and 2.4 times higher, respectively, than those under the +P treatment. Obviously, P has greater effects on the translocation of Ce in the monocots (corn and wheat) than in the dicots (cabbage and soybean). This indicates that the accumulations of Ce in the shoots of all the plant species under the −P treatment are equivalent.
3.2. Ce uptake and transformation by TEM
To further understand the above results, we observed the root sections by TEM (Fig. S3–S6†). In all the plant species, we found needle or rod-like particles on the root surfaces in the +P treatment, which are completely different from the morphology of the pristine octahedral nCeO2; however, in the −P treatment, we found large amounts of flocculated particles in the vacuole of root cells while no needle or rod-like particles were found on the root surface. The needle, rod, or the flocculated particles were CePO4 which have been identified in previous studies.6,20 Given that the transformation of CeO2 mainly occurs on the root surface rather than inside of the plant,16 the Ce3+ ions released from CeO2 can be easily immobilized on the root surface in the presence of P in the nutrient solution, and the upward translocation of Ce will be then inhibited. The removal of P from the medium reduces or eliminates the immobilization of Ce on the root surface, and thus the free translocation of Ce3+ from the roots to shoots can occur. The formation of CePO4 in the vacuoles in the −P treatment was because foliar applied P was adsorbed by plants and stored in the vacuole as a P “pool” which then reacts with the free Ce3+ ions as they translocate into the plant.21 The above results suggest that the P-containing ions have a significant impact on the uptake of nCeO2 in all the plant species tested.
It should be noted that the particulate CeO2 NPs were also observed inside the root of soybean but were not observed in the other plants (Fig. 1 and S5B†). As shown in Fig. 1A and C, nCeO2 were found in the intercellular regions. Interestingly, the morphology of some particles in the large aggregates (Fig. 1B) was different from that of the original nCeO2, suggesting the transformation of nCeO2. We previously found that nCeO2, which have been translocated into the cucumber plant, cannot transform;16 however, a recent study shows that nCeO2 may also be dissolved inside the leaves of sunflower resulting from pH variation and interaction with antioxidant substances in apoplast.22 The nCeO2 that we found here may be transformed either before or after their entering into the plants.
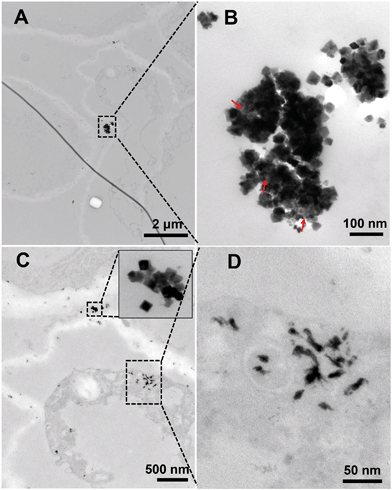 |
| Fig. 1 TEM images of the root sections of soybean treated with nCeO2 under the −P condition. (A) and (C) show the intercellular regions. (B) and (D) are the magnified images of the highlighted rectangle areas in A and C. Red arrows in B indicate the nCeO2 that were morphologically “transformed”. | |
3.3. Transformation of nCeO2 in plants analyzed by XANES
To further understand the translocation of Ce in the four plant species, we quantified the chemical species of Ce in the plant tissues by XANES and linear combination fitting (LCF) analyses (complete LCF results are given in the ESI,† Tables S2 and S3). The XANES spectra show how X-rays are absorbed by a specific atom, and how the absorption is modified by the chemical and physical environment of the atom in different compounds. With appropriate reference compounds, quantification of different chemical species can be achieved by doing the linear combination fitting (LCF) analysis on the XANES spectra. The results show that Ce was mainly presented as CeO2 (>74.1%) in the roots of the four plant species in both +P and −P treatments (Fig. 2A and 3A and B); the Ce(III) species are mainly CePO4 in the +P treatment but are mainly Ce carboxylates in the −P treatment. This is in accordance with the TEM results that the Ce3+ ions were mainly immobilized on the root surface with a minimum amount being translocated into the roots and shoots in the +P treatment; however, in the −P treatment, the Ce3+ ions moved easily into the roots, bound to PO43− in the vacuoles of root cells, and bound to carboxyl groups in the xylem during the upward translocation.
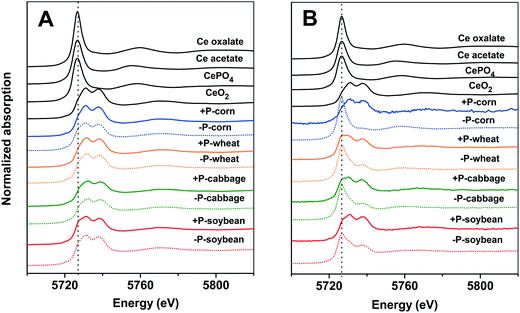 |
| Fig. 2 Ce L-III edge XANES spectra of the root (A) and shoot (B) of the plants with treatment of 2000 mg L−1 nCeO2 for 2 weeks under the +P (solid lines) and −P (dotted lines) conditions. Vertically dotted line indicates the peak of the Ce(III) species centered at 5726.7 eV. | |
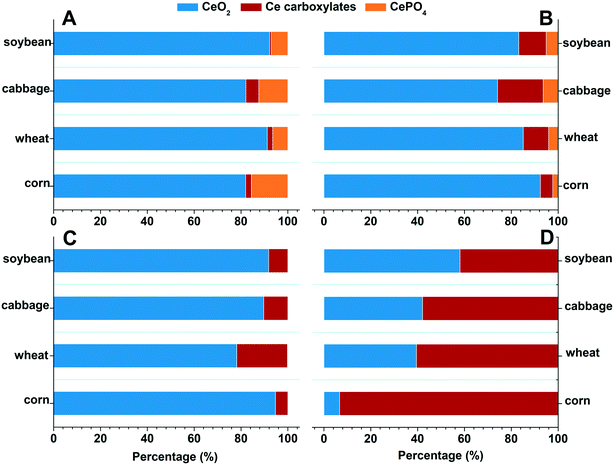 |
| Fig. 3 Fraction of the Ce species in the four types of plants treated with 2000 mg L−1 nCeO2 for 2 weeks. (A) Root, +P, (B) root, −P, (C) shoot, +P, and (D) shoot, −P. | |
The Ce species in the shoots were different from that in the roots (Fig. 2B and 3C and D). In the +P treatment, CeO2 was the predominant species in the shoots, with the highest proportion of 94.7% in corn and the lowest of 78.2% in wheat; only a small amount of Ce presented as Ce carboxylates. However, in the −P treatment, the proportion of Ce carboxylates remarkably increased, with a proportion of 93.3% in corn and the lowest proportion of 43.3% in the wheat shoots. Phosphate deficiency can induce a high abundance of metabolites with chelating ability and increase the active exudation of organic acids out from the roots.23 This process will enhance the dissolution of nCeO2. We further calculated the absolute contents of Ce(IV) and Ce(III) in the roots and shoots by multiplying their proportion by the total Ce contents (Table S4†). The Ce (IV) and Ce(III) contents in the roots and shoots in the −P treatment were both higher than those in the +P treatment (consistent with the data in Table 1). Notably, the Ce(III) contents in the shoots of corn in the −P treatment were 500 times higher than those in the +P treatment. Ce(III) is more toxic to plants than Ce in its nCeO2 form,24 therefore, the enhanced Ce(III) accumulation in the shoots might be the reason leading to the decrease of shoot biomasses of the corn and wheat plants under the −P treatment.
The mechanism of the transformation and translocation of nCeO2 in the cucumber plant has been well discussed in previous studies.6,16 However, discrepancies of the transformation and translocation of nCeO2 in different plant species are not fully explored. We deduced that two factors may account for the species-dependent translocation of the CeO2 NPs found in this study (Fig. 4). Firstly, structural differences between monocots and dicots result in different translocation abilities of substances. The xylem walls of dicots usually have higher affinity for nutrient ions than those of monocots because there are more negatively charged groups (e.g. carboxyl groups) on the xylem walls of dicots than those of monocots.25 Therefore, the translocation of cations in dicots is usually more difficult than in monocots, as the cations become immobilised on the xylem walls. However, this is not consistent with the higher Ce contents found in the dicots in the +P treatment. This can be explained by the different transformation under the two culturing conditions. In the +P treatment, Ce is mainly translocated in its particulate form (Table S4†), which is mainly driven by transpiration and is not affected by xylem walls/ion charges. However, in the −P treatment, more free Ce3+ ions entered into the roots (Table S4†) and can easily bind to the negatively charged groups (carboxyl) on the xylem wall; structural differences will then have significant effects on the translocation of Ce. The xylems of dicots are more negatively charged than the xylems of monocots, thus can bind more Ce3+ ions and restricted the upward translocation of the Ce3+ ions. Different translocation patterns of nCeO2 and Ce3+ in plant tissues are also reported in a previous study which compared the two-dimensional distribution of Ce in cucumber leaves with nCeO2 and Ce3+ ion treatments.26 This suggests that the particulate CeO2 mostly move to the leaf margins along with the water flow within the vascular bundle which is driven by transpiration; however, the Ce3+ ions mostly accumulate along the leaf veins, suggesting the binding of Ce3+ to the negatively charged groups on the xylem wall. The xylem structure-dependent translocation is further evidenced by looking at the translocation factor of the Ce(IV) and Ce(III) species in the plants (Fig. S7†). The TFs of Ce(IV) in the dicots are higher than those in the monocots, and remain largely the same between the +P and −P treatments for each plant species, suggesting a transpiration-driven translocation process of particulate Ce. However, the TFs of Ce(III) change drastically between the +P and −P treatments especially for the dicots. In the corn plants, the TFs of Ce(III) in the −P treatment are 221 times higher than that in the +P treatment, which leads to the accumulation of enough Ce3+ inducing the toxicity. These results indicate that the translocation of positively charged Ce3+ depends on the xylem structures.
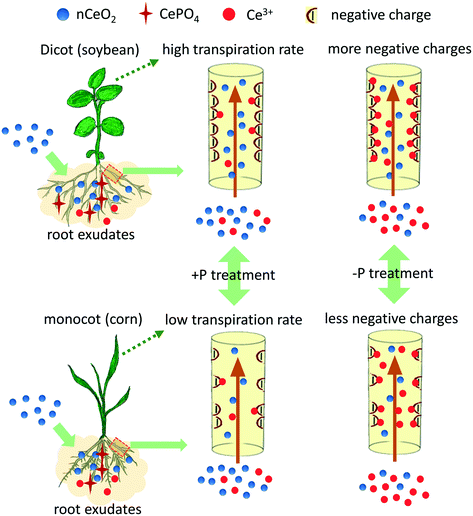 |
| Fig. 4 A schematic illustration of the transformation of nCeO2 on the root surface and the translocation of nCeO2 and Ce3+ ions in the root xylem of the dicot and monocot using soybean and corn as examples. | |
Secondly, root exudates play a critical role in the transformation of nCeO2 in plants. We extracted the root exudates from the plants according to a previously described method,27 and found that the hydrodynamic sizes of nCeO2 in root exudate solutions varied among the four plant species (Fig. S8 and S9†). The largest size (199 nm) was found for corn, which might be one possible reason for the low uptake of Ce in corn. The zeta potential of nCeO2 in the exudates under the +P and −P conditions and among the different species are not significantly different (Table S5†), suggesting that surface charges are not associated with the Ce translocation. Since the transformation of nCeO2 mainly occurs on the root surface with the assistance of the root exudates,16 the compositional difference in the root exudates may lead to different extent of transformation of nCeO2 on the root surface and subsequently different translocation of Ce. Root exudates are composed of low molecular compounds such as saccharides, organic acids, amino acids, phenols, high molecular polysaccharides and proteins.27 The low molecular organic acids (e.g., succinic acid and malic acid)8 and reducing substances such as phenols,28 which are critical for the transformation of nCeO2, varied among different species. Moreover, different acids and reducing substances have different capacities to dissolve the nCeO2.8,29 For example, mugineic acids, which have been found to promote the transformation of CuO NPs,30 are specifically excreted by gramineous plants (e.g., corn and wheat).31 Turnip cabbage excretes two more kinds of organic acids (i.e. malic and glycolic acids) than cucumber.32 Except for the compositional differences, amounts of exudates vary among different species and at different growth stages for the same plant species.33 Plant roots at the seedling stage generally excrete a greater amount of reducing substances than that at the germination stage, which may explain why no transformation of CeO2 in the roots of the four plant species was found in a previous study.14 We also noticed that in the +P treatment, the TFs of Ce(III) in the dicots are actually higher than those in the monocots (Table S4 and Fig. S7†), which may not be explained by either the xylem structures or the exudate compositions. This indicates an additional mechanism influencing the translocation of Ce on top of the xylem structures and the composition of exudates, e.g., dissolution of nCeO2 inside the leaves.
4. Conclusions
In this study, the plant species-dependent transformation and translocation of nCeO2 were comprehensively studied for the first time. Although the exposure concentration used in this study is much higher than the predicted environmental concentration of nCeO2 (e.g., 0.25–1 mg kg−1)34 and natural background concentration of Ce (48 mg kg−1 dry soil),35 the mechanistic understanding of nCeO2 behaviour in plants in this study is of vital importance. Our results suggest that different plant species have different abilities of transforming and translocating the CeO2 NPs and that the mechanisms differ depending on the chemistry of the surrounding solution (+P/−P). Plant xylems and root exudate compositions are both important factors in determining the transformation of nCeO2 and subsequent translocation of Ce species in plants. Further studies are required to identify the root exudate components and the determining components for nCeO2 transformation. In addition, the components of root exudates may vary (e.g., increase of organic acid levels)36 due to ambient stress (e.g., NP exposure) for the same plant species, and therefore, extracting and determining the exudate composition after NP exposure are important. Furthermore, different plant species respond differently to nCeO2 under different culturing conditions. For some plant species (e.g., corn and wheat in this study), deficiency of P can increase the potential phytotoxicity of nCeO2,37 and enhance the accumulation of Ce (mainly in the form of Ce3+) in plants. Besides, deficiency of P is only one of the conditions that might occur for plants, and more factors (e.g., pH values, redox state, and the presence of other ions) that may affect the conditions around the root should be considered in order to comprehensively understand the transformation of NPs in plants.
Conflicts of interest
There is no conflict of interest to declare.
Acknowledgements
This work was financially supported by the National Natural Science Foundation of China (Grants 11405183, 11575208, 11675190, 11875267, and 21507153) and the Ministry of Science and Technology of China (Grant 2016YFA0201600). Financial support from a Marie Skłodowska-Curie Individual Fellowship (NanoLabels 750455) is appreciated.
References
- T. Marie, A. Mélanie, B. Lenka, I. Julien, K. Isabelle, P. Christine, M. Elise, S. Catherine, A. Bernard and A. Ester, Transfer, transformation, and impacts of ceria nanomaterials in aquatic mesocosms simulating a pond ecosystem, Environ. Sci. Technol., 2014, 48, 9004–9013 CrossRef CAS PubMed.
- L. E. Barton, M. Auffan, L. Olivi, J.-Y. Bottero and M. R. Wiesner, Heteroaggregation, transformation and fate of CeO2 nanoparticles in wastewater treatment, Environ. Pollut., 2015, 203, 122–129 CrossRef CAS PubMed.
- L. E. Barton, M. Auffan, M. Bertrand, M. Barakat, C. Santaella, A. Masion, D. Borschneck, L. Olivi, N. Roche and M. R. Wiesner, Transformation of pristine and citrate-functionalized CeO2 nanoparticles in a laboratory-scale activated sludge reactor, Environ. Sci. Technol., 2014, 48, 7289–7296 CrossRef CAS PubMed.
- R. C. Merrifield, K. P. Arkill, R. E. Palmer and J. R. Lead, A high resolution study of dynamic changes of Ce2O3 and CeO2 nanoparticles in complex environmental media, Environ. Sci. Technol., 2017, 51, 8010–8016 CrossRef CAS PubMed.
- X. He, Y. Pan, J. Zhang, Y. Li, Y. Ma, P. Zhang, Y. Ding, J. Zhang, Z. Wu and Y. Zhao, Quantifying the total ionic release from nanoparticles after particle-cell contact, Environ. Pollut., 2015, 196, 194–200 CrossRef CAS PubMed.
- P. Zhang, Y. Ma, Z. Zhang, X. He, J. Zhang, Z. Guo, R. Tai, Y. Zhao and Z. Chai, Biotransformation of ceria nanoparticles in cucumber plants, ACS Nano, 2012, 6, 9943–9950 CrossRef CAS PubMed.
- Y. Ma, P. Zhang, Z. Zhang, X. He, Y. Li, J. Zhang, L. Zheng, S. Chu, K. Yang and Y. Zhao, Origin of the different phytotoxicity and biotransformation of cerium and lanthanum oxide nanoparticles in cucumber, Nanotoxicology, 2015, 9, 262–270 CrossRef CAS PubMed.
- W. Zhang, Y. Dan, H. Shi and X. Ma, Elucidating the mechanisms for plant uptake and in-planta speciation of cerium in radish (Raphanus sativus L.) treated with cerium oxide nanoparticles, J. Environ. Chem. Eng., 2017, 5, 572–577 CrossRef CAS.
- F. Schwabe, S. Tanner, R. Schulin, A. Rotzetter, W. Stark, A. Von Quadt and B. Nowack, Dissolved cerium contributes to uptake of Ce in the presence of differently sized CeO2 nanoparticles by three crop plants, Metallomics, 2015, 7, 466–477 RSC.
- C. Levard, E. M. Hotze, B. P. Colman, A. L. Dale, L. Truong, X. Yang, A. J. Bone, G. E. Brown Jr, R. L. Tanguay and R. T. Di Giulio, Sulfidation of silver nanoparticles: natural antidote to their toxicity, Environ. Sci. Technol., 2013, 47, 13440–13448 CrossRef CAS PubMed.
- J. Cervini-Silva, D. A. Fowle and J. Banfield, Biogenic dissolution of a soil cerium-phosphate mineral, Am. J. Sci., 2005, 305, 711–726 CrossRef CAS.
- P. Zhang, Y. Ma, Z. Zhang, X. He, Y. Li, J. Zhang, L. Zheng and Y. Zhao, Species-specific toxicity of ceria nanoparticles to Lactuca plants, Nanotoxicology, 2015, 9, 1–8 CrossRef CAS PubMed.
- P. Zhang, C. Xie, Y. Ma, X. He, Z. Zhang, Y. Ding, L. Zheng and J. Zhang, Shape-Dependent Transformation and Translocation of Ceria Nanoparticles in Cucumber Plants, Environ. Sci. Technol. Lett., 2017, 4, 380–385 CrossRef CAS.
- M. L. López-Moreno, G. de la Rosa, J. A. Hernández-Viezcas, J. R. Peralta-Videa and J. L. Gardea-Torresdey, XAS corroboration of the uptake and storage of CeO2 nanoparticles and assessment of their differential toxicity in four edible plant species, J. Agric. Food Chem., 2010, 58, 3689 CrossRef PubMed.
- Z. Zhu, H. Wang, B. Yan, H. Zheng, Y. Jiang, O. R. Miranda, V. M. Rotello, B. Xing and R. W. Vachet, Effect of surface charge on the uptake and distribution of gold nanoparticles in four plant species, Environ. Sci. Technol., 2012, 46, 12391–12398 CrossRef CAS PubMed.
- Y. Ma, P. Zhang, Z. Zhang, X. He, J. Zhang, Y. Ding, J. Zhang, L. Zheng, Z. Guo and L. Zhang, Where does the transformation of precipitated ceria nanoparticles in hydroponic plants take place?, Environ. Sci. Technol., 2015, 49, 10667–10674 CrossRef CAS PubMed.
- EPA Ecological effects test guidelines, OPPTS 850.4150 Terrestrial Plant Toxicity, Tier I (vegetative Vigor), EPA 712-C-96-163 (Public Draft); Office of Prevention, Pesticides and Toxic Substances, U.S. Environmental Protection Agency, Washington, DC, 1996.
- A. Gaur, B. Shrivastava and S. Joshi, Copper K-edge XANES of Cu(I) and Cu(II) oxide mixtures, J. Phys.: Conf. Ser., 2009, 190, 012084 CrossRef.
- R. Russell and V. Shorrocks, The relationship between transpiration and the absorption of inorganic ions by intact plants, J. Exp. Bot., 1959, 10, 301–316 CrossRef.
- P. Zhang, Y. Ma, S. Liu, G. Wang, J. Zhang, X. He, J. Zhang, Y. Rui and Z. Zhang, Phytotoxicity, uptake and transformation of nano-CeO2 in sand cultured romaine lettuce, Environ. Pollut., 2017, 220, 1400–1408 CrossRef CAS PubMed.
- R. Bieleski, Phosphate pools, phosphate transport, and phosphate availability, Annu. Rev. Plant Biol., 1973, 24, 225–252 CrossRef CAS.
- S. Schymura, T. Fricke, H. Hildebrand and K. Franke, Elucidating the Role of Dissolution in CeO2 Nanoparticle Plant Uptake by Smart Radiolabeling, Angew. Chem., Int. Ed., 2017, 56, 1–5 CrossRef PubMed.
- N. Edayilam, D. A. Montgomery, B. O. Ferguson, A. S. Maroli, N. Martinez, B. A. Powell and N. Tharayil, Phosphorus stress-induced changes in plant root exudation could potentially facilitate uranium mobilization from stable mineral forms, Environ. Sci. Technol., 2018, 52, 7652–7662 CrossRef CAS PubMed.
- W. Zhang, S. D. Ebbs, C. Musante, J. C. White, C. Gao and X. Ma, Uptake and accumulation of bulk and nanosized cerium oxide particles and ionic cerium by radish (Raphanus sativus L.), J. Agric. Food Chem., 2015, 63, 382–390 CrossRef CAS PubMed.
-
P. J. White, in Marschner's Mineral Nutrition of Higher Plants, Elsevier, 3rd edn, 2012, pp. 49–70 Search PubMed.
- Z. Zhang, X. He, H. Zhang, Y. Ma, P. Zhang, Y. Ding and Y. Zhao, Uptake and distribution of ceria nanoparticles in cucumber plants, Metallomics, 2011, 3, 816–822 RSC.
- K. Tawaraya, R. Horie, T. Shinano, T. Wagatsuma, K. Saito and A. Oikawa, Metabolite profiling of soybean root exudates under phosphorus deficiency, Soil Sci. Plant Nutr., 2014, 60, 679–694 CrossRef CAS.
- V. Vančura, Root exudates of plants, Plant Soil, 1964, 21, 231–248 CrossRef.
- Y. Rui, P. Zhang, Y. Zhang, Y. Ma, X. He, X. Gui, Y. Li, J. Zhang, L. Zheng and S. Chu, Transformation of ceria nanoparticles in cucumber plants is influenced by phosphate, Environ. Pollut., 2015, 198, 8–14 CrossRef CAS PubMed.
-
P. McManus, Rhizosphere interactions between copper oxide nanoparticles and wheat root exudates in a sand matrix; influences on bioavailability and uptake, Utah State University, 2016 Search PubMed.
- T. W.-M. Fan, A. N. Lane, M. Shenker, J. P. Bartley, D. Crowley and R. M. Higashi, Comprehensive chemical profiling of gramineous plant root exudates using high-resolution NMR and MS, Phytochemistry, 2001, 57, 209–221 CrossRef CAS PubMed.
- V. Vančura and A. Hovadik, Root exudates of plants: II. Composition of root exudates of some vegetables, Plant Soil, 1965, 21–32 CrossRef.
- V. Vančura and A. Hanzlíková, Root exudates of plants: IV. Differences in chemical composition of seed and seedlings exudates, Plant Soil, 1972, 271–282 CrossRef.
- F. Gottschalk, T. Sun and Bernd Nowack, Environmental concentrations of engineered nanomaterials: Review of modeling and analytical studies, Environ. Pollut., 2013, 181, 287–300 CrossRef CAS PubMed.
- G. Tyler, Rare earth elements in soil and plant systems - A review, Plant Soil, 2004, 267, 191–206 CrossRef CAS.
- P. Wang, S. Bi, S. Wang and Q. Ding, Variation of wheat root exudates under aluminum stress, J. Agric. Food Chem., 2006, 54, 10040–10046 CrossRef CAS PubMed.
- G. Wang, Y. Ma, P. Zhang, X. He, Z. Zhang, M. Qu, Y. Ding, J. Zhang, C. Xie and W. Luo, Influence of phosphate on phytotoxicity of ceria nanoparticles in an agar medium, Environ. Pollut., 2017, 224, 392–399 CrossRef CAS PubMed.
Footnote |
† Electronic supplementary information (ESI) available: Characterization results of nCeO2 (Fig. S1 and Table S1), biomass of plants (Fig. S2), TEM figures of root sections (Fig. S3–S6), fraction of Ce species obtained by LCF analysis (Tables S2 and S3), Ce species contents (Table S4), translocation factors of Ce species in plants (Fig. S7), and DLS results of nCeO2 in root exudates (Fig. S8 and S9 and Table S5). See DOI: 10.1039/c8en01089g |
|
This journal is © The Royal Society of Chemistry 2019 |