DOI:
10.1039/C9AY90147G
(AMC Technical Brief)
Anal. Methods, 2019,
11, 5833-5836
Laser-induced breakdown spectroscopy (LIBS) in cultural heritage
Received
18th September 2019
First published on 8th November 2019
Abstract
Laser-induced breakdown spectroscopy (LIBS) is a versatile technique that provides nearly instant elemental analysis of materials, both in the laboratory and in the field. This is done by focusing a short laser pulse on the surface of the sample, or object, studied and analysing the resulting spectrum from the laser-induced plasma. LIBS has been employed in the analysis of archaeological and historical objects, monuments and works of art for assessing the qualitative, semi-quantitative and quantitative elemental content of materials such as pigments, pottery, glass, stone, metals, minerals and fossils. It is also used in robotic planetary rovers. This Technical Brief describes the basic concepts of LIBS, presents relevant aspects of instrumentation and discusses how the technique is applied in the context of cultural heritage studies.
Introduction
Laser-induced breakdown spectroscopy (LIBS) was introduced as a potential analytical tool in the context of cultural heritage studies during the mid-1990s. It facilitates the rapid elemental analysis of samples or objects examined, in situ, with no need for any sample or surface preparation. Despite these attractive features, the use of LIBS is still not widespread among conservators or archaeologists. This is primarily because of the lack, to date, of commercial instruments dedicated to heritage applications, but also in some instances, of its micro-destructive character.
How does LIBS work?
The fundamental principle underlying LIBS stems from the brief interaction – just a few nanoseconds – between a focused laser pulse and a target object. This concentration of light both in space and time (leading to irradiance values of the order of a few GW/cm2) is key to the initiation of a process (known as ‘laser ablation’) that triggers the rapid formation of a microplasma plume just above the sample surface. Following the laser pulse, this microplasma persists for a few microseconds, emitting radiation that arises from the relaxation of its constituents (electrons, excited atoms and ions). Recording this emission with a spectrometer produces a typical LIBS spectrum (Fig. 1) with emission peaks at characteristic wavelengths, reflecting the elemental composition of the sample (qualitative analysis). The peak intensities or areas can also be associated with the concentrations of specific elements in the substrate (quantitative analysis). It is noted that up to around 0.5 μs after the interaction of the laser pulse with the sample, the emission of the plasma is strong and mainly in the form of an unstructured spectral continuum. As this continuum decays, sharp atomic emission lines emerge. Therefore, in order to obtain clean spectra, it is essential to start measuring the plume emission after a short delay (typically 0.5–1 μs) with respect to the laser pulse.
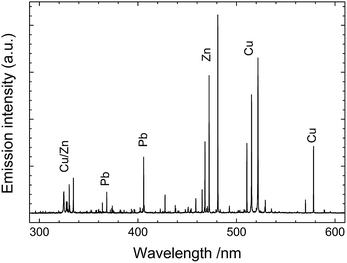 |
| Fig. 1 LIBS spectrum collected from a leaded brass object. Characteristic atomic emission lines from Cu, Zn and Pb are observed. | |
Instrumentation
In recent years, a variety of LIBS instruments, both bench-top and portable, have been introduced by manufacturers for specific applications such as screening of on-site materials or industrial process monitoring. The use of these instruments for the investigation of heritage objects would require several adaptations so as to ensure, for example: fine energy adjustment of the laser pulse; accurate aiming and proper focusing of the beam; single pulse operation; and, the ability to handle samples and objects of varied sizes. The small size of the heritage market has not encouraged manufacturers to perform such instrumental modifications. As an outcome, the relatively small number of research groups using LIBS for heritage applications have been employing custom-designed instruments optimized for use in heritage studies.
As a typical example of LIBS instrumentation used for the analysis of cultural heritage objects, a custom-built mobile LIBS spectrometer (Fig. 2) developed at IESL-FORTH for research purposes, is briefly described. The system consists of an optical probe head (1), a spectrometer (2), and a power supply unit (3). It weighs less than 9 kg and fits in a compact case. It is operated and controlled through a laptop computer. A micrometer translation stage is used for accurate positioning of the probe head and, where necessary, a tripod or appropriate scaffolding is used to position the equipment in front of the object (Fig. 3).
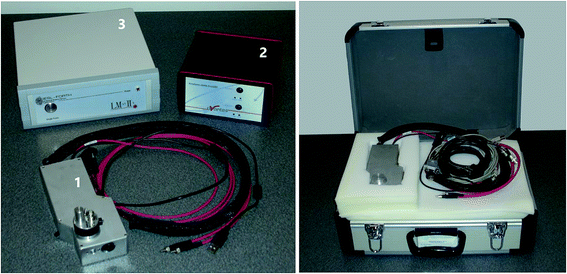 |
| Fig. 2 Main subunits of a custom-built mobile LIBS spectrometer (left) and the instrument in a carrying case (right). | |
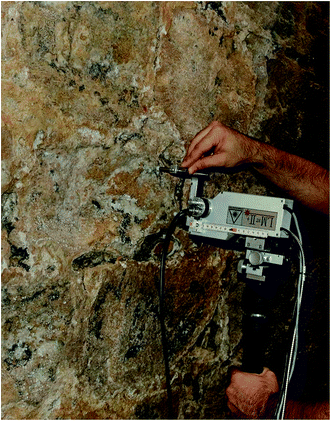 |
| Fig. 3 Analysis of salts in the inner wall of the Venetian castle of Koules at Heraklion, Crete (Greece) with the mobile LIBS spectrometer shown in Fig. 2. | |
The probe head houses a small-sized, pulsed Nd:YAG laser operating at 1064 nm (10 mJ per pulse, 10 ns) and is equipped with a miniature charged-coupled device (CCD) camera. The camera allows the analyst to visualize the sample and accurately aim the instrument, and facilitates photographic documentation during all stages of analysis.
Specific instruments exploit specific features of laser sources or spectrometers and detectors. Picosecond or femtosecond pulses can be used (not available in mobile systems), leading to different mechanisms of laser ablation and plasma formation, while double-pulse LIBS has been found to enhance sensitivity. Employing spectrometers based on echelle gratings offers superb spectral resolution and thus accurate qualitative analysis, although their use increases analysis time. The use of intensified CCD detectors provides the ability to time-resolve the spectra and reject the early continuum background, also offering enhanced sensitivity.
Analysis protocol
In a typical analysis, the object/sample is placed at the focal point of the lens and the probe head (with the laser deactivated) is positioned accurately. The area probed by the laser beam is approximately 0.2 mm in diameter. It should be recognised that there is an act of sampling in LIBS but it is made by the laser under the control of the analyst (see also ref. 7). A single laser pulse is sufficient for obtaining a spectrum with a high signal-to-noise ratio. In routine measurements, a few pulses, typically 1–5, are delivered at each spot investigated on the object/sample surface. Separate spectra are recorded when depth profiling information is required. This can be achieved because of the nature of the laser ablation which results in the removal of thin layers of materials (of the order of a few microns per pulse) during analysis (see also ‘Advantages and limitations’).
Advantages and limitations
LIBS provides multi-elemental analysis data, including low atomic number elements such as, for example, Li, F, Na, Mg, Al, and Si with typical limit of detection (LOD) values in the range of 0.5% in single-pulse spectra (see further discussion in Analysis of Spectra).
Spatial resolution: LIBS is effectively a microprobe technique which provides analysis of areas 0.1–0.2 mm across, which can be further reduced to 0.01 mm if a microscope objective is employed (Fig. 4).
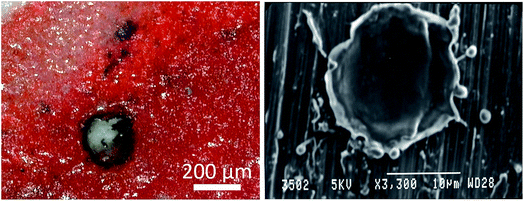 |
| Fig. 4 Optical image of a crater formed on tempera paint following depth profile analysis with multiple (approx. 50) laser pulses (left). Scanning electron microscope (SEM) image of a crater formed on a test metal piece by a single laser pulse focused through a laser objective (right). | |
Speed: the simplicity of the technique and its speed permit the analysis of a large number of objects in a short time. For example, LIBS is ideal for the quick screening and characterization of artefacts from archaeological excavations (Fig. 5).
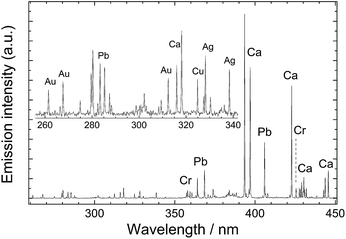 |
| Fig. 5 Single shot spectrum recorded during LIBS analysis of yellow paint remains on a stone sculpture at the Historical Museum of Heraklion, Crete (Greece). Dominant in the spectrum are the lines of Ca emerging from the stone. The detection of Pb and Cr suggests the presence of chrome yellow (PbCrO4), independently verified through Raman analysis. Au is also detected, along with Ag and Cu. indicating the use of gold leaf (traces of which are seen under a microscope). | |
Sampling: the technique can be used in situ, so there is no need for sampling and/or sample preparation, which can be time consuming operations that sometimes damage the object.
Versatility: almost any size of object can be analysed, as long as the spot to be probed can be positioned at the focal point of the laser beam. The use of remote LIBS analysis of monument surfaces (even at distances exceeding 50 m) has been demonstrated.
Depth profiling: it is possible to perform the equivalent of depth profile analysis. For example, if a painting is examined, each laser pulse removes a small amount of material from the surface and therefore the following pulse always probes a new section of the paint, at a layer that is slightly deeper than the previous one. As a result, successive LIBS spectra obtained with a few pulses on the same point reveal the stratigraphy of the paint layers and this essentially provides an in situ cross-sectional analysis.
Environmental applications: LIBS is ideal for detecting environmental contaminants on surfaces.
A significant drawback of LIBS is that by its very principle of operation – relying on laser ablation – the technique is, strictly speaking, invasive. It may be classified as minimally invasive or micro-destructive, given the very small area affected, but the fact remains that the analysis does remove a very small amount of material (typically of the order of a few nanograms) to generate plasma. The material analysed no longer remains in place and if the analyst wishes to repeat the analysis at the same spot, they will obtain data from a fresh layer of material. This is not a problem with homogeneous materials, but it could lead to confusion with materials of which the composition changes abruptly with depth.
Quantitative analysis is possible but complex. The use of external calibration requires the availability of matrix-matched calibrators (reference materials with compositions that match those of the objects under analysis). This is straightforward in the case of archaeological metal alloys (typically binary to quaternary alloys) but it can be demanding with more complex matrices such as pottery or minerals. In some cases, calibration-free methods can be employed. Either way, detecting and quantifying elements at mass fractions below 0.5% remains a challenge, particularly because when performing LIBS on heritage objects or samples the laser pulse energy employed is kept at low levels (typically not over 10 mJ), hence emissions from low concentration elements are very weak.
Analysis of spectra
The interpretation of LIBS spectra, to identify which elements are present, is simple for an experienced analyst. It is becoming more straightforward even for new users thanks to databases (see ref. 8) that provide spectral line data for each element (aiding element identification) or even reference spectra that allow users to compare their LIBS spectra against those of the library and qualitatively identify the elemental compositions of their samples.
Several commercially available LIBS instruments provide some spectral recognition features, while several research groups have developed custom-made software that enables simulation of emission spectra.
Conclusions – outlook
LIBS is a versatile technique that can be used in the characterization of many types of heritage materials, rapidly providing information on their elemental compositions. Being mobile, LIBS facilitates analysis at diverse locations and this can be valuable at several stages of archaeological research and conservation campaigns and studies. Close interactions between members of the heritage (archaeologists, historians and conservators) and analytical communities will be needed for exploiting the potential of LIBS.
Safety
The operation of laser equipment requires proper training and the use of protective eyewear.
Demetrios Anglos (Department of Chemistry, University of Crete and Institute of Electronic Structure and Laser, Foundation for Research and Technology-Hellas)
This Technical Brief was prepared by the Heritage Science Subcommittee and approved by the AMC on 21 May 2019.
Further reading
- Various articles on LIBS analysis and instrumentation in: http://www.spectroscopyonline.com/libs-0.
- The use of LIBS for the analysis of archaeological objects and artefacts: http://www.science4heritage.org/COSTG7/booklet/chapters/libs.htm.
- A. Botto, B. Campanella, S. Legnaioli, M. Lezzerini, G. Lorenzetti, S. Pagnotta, F. Poggialini and V. Palleschi, Applications of laser-induced breakdown spectroscopy in cultural heritage and archaeology: a critical review, J. Anal. At. Spectrom., 2019, 34, 81–103 RSC.
-
D. Anglos and V. Detalle, Cultural Heritage Applications of LIBS, in Laser-Induced Breakdown Spectroscopy – Theory and Applications, Springer Series in Optical Sciences, ed. S. Musazzi and U. Perini, Springer, Berlin, 2014, vol. 182, pp. 531–553 Search PubMed.
- Z. E. Papliaka, A. Philippidis, P. Siozos, M. Vakondiou, K. Melessanaki and D. Anglos, A multi-technique approach, based on mobile/portable laser instruments, for the in situ pigment characterization of stone sculptures on the island of Crete dating from Venetian and Ottoman period, Heritage Sci., 2016, 4, 15 CrossRef.
- J. Agresti, A. A. Mencaglia and S. Siano, Development and application of a portable LIPS system for characterising copper alloy artefacts, Anal. Bioanal. Chem., 2009, 395, 2255–2262 CrossRef CAS PubMed.
- AMC Technical Brief No. 84, Beam sampling: taking samples at the micro-scale, Anal. Methods, 2018, 10, 1100–1102, 10.1039/c8ay90021c.
-
NIST Atomic Spectra Database (version 5.6.1), http://www.nist.gov/pml/atomic-spectra-database-physics.nist.gov/PhysRefData/ASD/LIBS/libs-form.html Search PubMed.
|
This journal is © The Royal Society of Chemistry 2019 |