Protective effects of Nigella sativa L. seed extract on lead induced neurotoxicity during development and early life in mouse models†
Received
20th July 2017
, Accepted 4th October 2017
First published on 5th October 2017
Abstract
Lead (Pb), a ubiquitous heavy metal and a known neurotoxicant, produces adverse effects on the brain via increased production of reactive oxygen species (ROS) and causes oxidative stress. In this study we examined the neuroprotective effects of the ethanolic extract of Nigella sativa L. seeds on Pb induced oxidative stress in the developing brain of mice. Mouse pups were exposed to low (0.1%) and high (0.2%) doses of Pb from the first day of pregnancy through their mothers (via drinking water) and lactation until post-natal day (PND) 21. The mRNA expression levels of superoxide dismutase (SOD1), peroxiredoxin (Prdx6), amyloid precursor protein (APP) common, APP695 and APP770 were examined in the cortex and hippocampus of the mouse brain excised on PND 21 by semi-quantitative RT-PCR. The free radical scavenging activity of ethanolic Nigella sativa L. extract was assessed by DPPH assay. The results showed that Pb exposure caused a significant decrease in the expression of SOD1, Prdx6 and APP695 and an increase in APP770 in both cortex and hippocampus in a dose dependent manner as compared to the control group. The expression of APP common remained unaltered. Histological assessment of the cortex and hippocampus demonstrated a decrease in the neuronal number and Nissl bodies. The administration of 250 and 500 mg kg−1 ethanolic Nigella sativa L. extract reversed the adverse effects by significantly increasing the expression of SOD1, Prdx6 and APP695 and decreasing the expression of APP770 in both the regions. These results strongly suggest that Nigella sativa L. supplementation greatly improves Pb-induced neurotoxicity in early life and provides neuroprotective and antioxidant potentials.
Introduction
Lead (Pb) is known as a potent environmental toxicant, affecting nearly every organ system of the human body particularly the developing brain, and hematopoietic, hepatic and renal systems.1 Pb has no apparent biological function in the human body, however, it continues to find its way into the body's interior and can be potent enough to cause disorder in the CNS and induce early brain aging.2
Oxidative stress is one of the major pathological and toxicological manifestations caused by Pb as it has the ability to bind to sulfhydryl groups present in the cysteine residues of antioxidant enzymes, causing conformational changes which results in their functional inactivation.3 Pb toxicity also results in lipid peroxidation and oxidation of hemoglobin, nucleic acids and proteins.4 All of these events make the cell extremely vulnerable and may lead to cell death or apoptosis.
Accumulating evidence from clinical and animal studies suggests that certain incidences in early life can manifest into many pathological conditions in the later stages of life.5,6 Infections in neonates can induce memory impairments in adults as suggested in schizophrenia.7 Moreover, exposure to Pb-contaminated drinking water results in fetal death and reduced birth rates.8 It is also associated with decline in IQ in children and various adverse behavioural effects.9,10 Pb exposure also contributes towards attention deficit hyperactivity disorder (ADHD) and aplastic anaemia in children.11,12 Disturbances of posture in children are also prominent effects of Pb on the CNS even at very low Pb exposure.13
Pb exposure also has deleterious effects on children's postnatal neurocognitive development.14 In early life, Pb may precondition the brain for developing neurodegenerative diseases by elevating the Aβ aggregates in the brain resulting in Alzheimer's disease (AD).15,16 Pb exposure significantly induces amyloidogenesis, senile plaque formation and protein alterations and thus, contributes to a disturbed amyloid processing pathway.17
Nigella sativa L. (commonly known as Black seed), belongs to the Ranunculaceae family native to Southwest of Asia,18 possessing several therapeutic effects including, antibacterial,19 anti-inflammatory20 and antioxidant properties.21,22
The present study focuses on the potential pathological role of the heavy metal Pb in inducing oxidative stress in mouse pups and explores the effective properties of the medicinal herb Nigella sativa L.. The present research work is an initiative to provide preliminary data on Pb-induced toxicity in the CNS during development and early life and the potential therapeutic benefits of a commonly used medicinal herb to combat neurotoxicity.
Materials and methods
Chemicals
Lead acetate trihydrate (Pb(CH3CO2)2·3H2O) was obtained from Merck (USA). Reverse transcriptase (RT), deoxynucleotide triphosphate (dNTPs) and Taq polymerase were obtained from Fermentas (Thermo Scientific, USA). 2,2-Diphenyl-1-picrylhydrazyl (DPPH; product code: 101087701) and electrophoresis chemicals were obtained from Sigma-Aldrich, unless noted otherwise. All chemicals were freshly prepared on the day of experiment to achieve maximum results.
Plant material and extraction
Dried Nigella sativa L. seeds were procured from a local spice market in Rawalpindi, Pakistan. The extract was prepared using Nigella sativa L. seeds (500 g) ground into fine powder and sieved with a 80 mesh sieve. The fine powder (10 g) was loaded into the main chamber of a Soxhlet extractor with distillation flasks containing 100% ethanol. Reduced pressure was maintained at 55 °C to concentrate the resulting extract by using a rotatory evaporator (R200 rotavapour Buchii) to remove the remaining traces of solvent. The dried extract was collected and stored at 4 °C until further use.
Proton nuclear magnetic resonance (1H-NMR) spectroscopic analysis
The 1H NMR spectra of Nigella sativa L. crude extract were recorded on a Bruker Avance III 500 MHz spectrometer. The chemical shifts (δ) were reported in ppm, while tetramethylsilane (TMS) is used as an internal reference standard during analysis of some samples.
In vitro assay for DPPH free radical scavenging activity
In order to assess the free radical quenching activity of Nigella sativa L. seed extract, the experimental procedure for 1,1-diphenyl-2-picrylhydrazyl (DPPH) scavenging activity was performed according to the protocol described by Singh et al.,23 with minor modifications. Briefly, 0.4 mL of DPPH methanolic solution (0.1 mM) was added to 1 mL Nigella sativa L. seed extract and standards (ascorbic acid and gallic acid) of different concentrations ranging from 1000 μg ml−1 to 0.001 μg ml−1, respectively. The absorbance was measured at 517 nm after 30 min of reaction using an OPTIMA SP-300 spectrophotometer. The % antioxidant activity (%AA) was calculated according to the following equation: % DPPH scavenging activity = 100(control absorbance − sample absorbance/control absorbance).
Animals
BALB/c mice were acquired from the National Institute of Health (NIH), Islamabad, Pakistan and housed at the animal facility of Atta-ur-Rahman School of Applied Biosciences (ASAB), National University of Sciences and Technology (NUST). After an acclimatization period of two weeks animals were bred and kept in metal cages at a constant temperature (25 ± 2 °C) and natural light–dark cycle (12–12 hours). Pregnant female BALB/c mice (35–45 g and 10–12 weeks of age) were used in this study. During the entire experimental period mice received distilled water ad libitum and fed with a standard diet consisting of (%): crude protein 30, crude fat 9, crude fiber 4 and moisture 10. All experiments were performed in compliance with the guidelines of the Institute of Laboratory Animal Research, Division on Earth and Life Sciences, National Institutes of Health, USA (Guide for the Care and Use of Laboratory Animals: Eighth Edition, 2011). The protocol was approved by the Internal Review Board (IRB), ASAB, NUST.
Experimental design
In this study 4 timely pregnant female mice were included in each experimental group (10 groups). Mice were kept in a ratio of 1
:
4 males and females, respectively, and females showing vaginal plugs were separated into the control and Pb-treated groups. Pb exposure doses of 0.1% and 0.2%, as described,24,25 were administered via drinking water. Pb exposure lasted for 42 days commencing from the first day of gestation until postnatal day (PND) 21. A total of eight male and eight female pups were randomly selected for studying the Pb-induced neurotoxicity and effects of Nigella sativa L.
Nigella sativa L. extract was given at a low dose of 250 mg kg−1 (ref. 26) and high dose of 500 mg kg−1 (ref. 27) mixed with normal feed. The control mice (Group 1) were only given deionized water and normal feed. Groups 2 and 3 were given deionized water and 500 mg kg−1 and 250 mg kg−1Nigella sativa L. extract, while Groups 4 and 5 were exposed to 0.1% and 0.2% Pb and normal feed, respectively, for 42 days. For Groups 6–9, exposure to 0.1% and 0.2% Pb was continued for 42 days while the administration of 500 mg kg−1 and 250 mg kg−1Nigella sativa L. extract was started after parturition until PND 21, respectively. The prophylactic group (Group 9) was given 500 mg kg−1Nigella sativa L. extract for the first 21 days (pre-natal) followed by administration of 0.2% Pb in distilled water for 21 days (PND 21). The detailed study design is provided in Table 1.
Table 1 Dose and administration of Pb and Nigella sativa L. extract: all the experimental animal groups included in this study have 4 pregnant females at the initiation of the experiments. Treatment of Pb and Nigella sativa L. seed extract lasted for a total duration of 42 days starting from the first gestation day until postnatal day 21. The pups were exposed to Pb and Nigella sativa L. seed extract through their mothers (pre-natal) and lactation until PND 21
Experimental groups |
Animals (n) |
Administration of Pb and Nigella sativa L. extract |
Duration (days) |
Group 1 (control) |
4 pregnant female mice |
Deionized distilled water + normal feed |
42 |
8 M and 8 F pups |
Group 2 |
4 pregnant female mice |
Deionized distilled water + 500 mg kg−1Nigella sativa L. extract |
42 |
8 M and 8 F pups |
Group 3 |
4 pregnant female mice |
Deionized distilled water + 250 mg kg−1Nigella sativa L. extract |
42 |
8 M and 8 F pups |
Group 4 |
4 pregnant female mice |
0.1% Pb in distilled water + normal feed |
42 |
8 M and 8 F pups |
Group 5 |
4 pregnant female mice |
0.2% Pb in distilled water + normal feed |
42 |
8 M and 8 F pups |
Group 6 |
4 pregnant female mice |
0.1% Pb in distilled water + 250 mg kg−1Nigella sativa L. extract |
42 |
8 M and 8 F pups |
Group 7 |
4 pregnant female mice |
0.1% Pb in distilled water + 500 mg kg−1Nigella sativa L. extract |
42 |
8 M and 8 F pups |
Group 8 |
4 pregnant female mice |
0.2% Pb in distilled water + 250 mg kg−1Nigella sativa L. extract |
42 |
8 M and 8 F pups |
Group 9 |
4 pregnant female mice |
0.2% Pb in distilled water + 500 mg kg−1Nigella sativa L. extract |
42 |
8 M and 8 F pups |
Prophylactic group |
4 pregnant female mice |
500 mg kg−1Nigella sativa L. extract (21 days) followed by 0.2% Pb in distilled water until PND 21 |
42 |
8 M and 8 F pups |
Gene expression studies
The animals in all groups were sacrificed after 24 hours of the completion of treatment. All mice were anesthetized and euthanized by cervical dislocation and the brain regions (cortex and hippocampus) were dissected out immediately and snap frozen in liquid nitrogen. RNA extraction was carried out according to the manufacturer's protocol using Tri-reagent. Qualitative assessment was performed by running RNA samples on 2% agarose gel, visualized by using a Dolphin-Doc imaging system (Wealtec Corp., USA). Extracted RNA was quantified using a BioPhotometer plus (Eppendorf, Germany) and an equal quantity of RNA (200 μg L−1) was used to reverse transcribe into cDNA (60 μL). PCR reactions were performed using a Swift Maxi Thermal Cycler Block ESCO, Singapore. The thermocycling conditions were 95 °C for 5 min followed by 95 °C for 30 s. The annealing temperature was set differently for each gene (β-actin, 55 °C; APP Common, 55 °C; APP695, 60 °C; APP770, 60 °C; SOD1, 58 °C and Prdx6, 60 °C). Then 35 cycles at 72 °C for 30 s and the final extension at 72 °C for 10 min were performed. β-Actin gene, used as an internal control was amplified with a specific primer pair (5′-GCCTTCCTTCTTGGGTATGG-3′/5′-C AGCTCAGTAACAGTCCGC-3′). The specific primer sequences of APP Common, APP695, APP770, SOD1 and Prdx6 are listed in Table 2.
Table 2 Primer sequences used for expression analysis of SOD1, Prdx6, APP Common, App770 and App695
Gene |
Forward primer |
Reverse primer |
SOD1 |
5′-GACAAACCTGAGCCCTAAG-3′ |
5′-CGACCTTGCTCCTTATTG-3′ |
Prdx6 |
5′-TTGATGATAAGGGCGGGAC-3′ |
5′-CTACCATCACGCTCTCTCCC-3′ |
APP Common |
5′-TGTGATCTACGAGCGCATGAACC-3′ |
5′-AAGACATCGTCGGAGTAGTTCTGC-3′ |
APP770 |
5′-TGCTCTGAACAAGCCGAGACC-3′ |
5′-CATGCAGTACTCTTCCGTGTC-3′ |
APP695 |
5′-GATGAGGATGTGGAGGATGG-3′ |
5′-GCTGCTGTCGTGGGAACTC-3′ |
The PCR products were separated on 3% agarose gel and visualized by using a Dolphin-Doc imaging system (Wealtec Corp., USA). The expression of the PCR products was analyzed by measuring the density in terms of minimum and maximum grey values. The density of each band was then normalized with the respective β-actin band and the expression was measured by dividing the desired gene density with β-actin and the results are presented as expression graphs.
Histological assessment
Heart perfusion was performed in accordance with the protocol of Gage et al.28 Tissue sections (4 μm) were deparaffinized in xylene for 10 min before being rehydrated in 70% isopropanol (10 min), and washed with ddH2O (5 min). Cresyl violet stain was poured over the tissue sections and left for 4 min. The sections were then washed with ddH2O and 70% acid alcohol (2 min) and later dried for 2 h and mounted with cover slips. The sections were visualized using an inverted microscope at 10× and 40× resolutions; the images were captured using Pixel Pro™ image analysis software (Labomed, USA).
Statistical analysis
Statistical analyses of the data were performed using a one-way analysis of variance (ANOVA) using GraphPad Prism 5.0. The data were represented as mean ± SEM with a 95% confidence interval. A p-value <0.05 was considered statistically significant.
Results
Free radical scavenging activity of Nigella sativa L. seed extract
The free radical quenching activity of the ethanolic extract of Nigella sativa L. seed and standards was measured by DPPH assay. The results demonstrated that Nigella sativa L. seed extract substantially inhibits free radicals in a dose dependent manner. The results were consistent with standard antioxidants: gallic acid and ascorbic acid (ESI Fig. 1†).
1H NMR investigation of Nigella sativa L. seed extract
The 1H NMR spectrum of Nigella sativa extract indicated the abundant presence of quinones, mainly thymoquinone and dihydroxythymoquinone. Quercetin and kaempferol which are important constituents of flavonoids, serpentine (an alkaloid) and coumarins were also identified (Fig. 1 and Table 3).
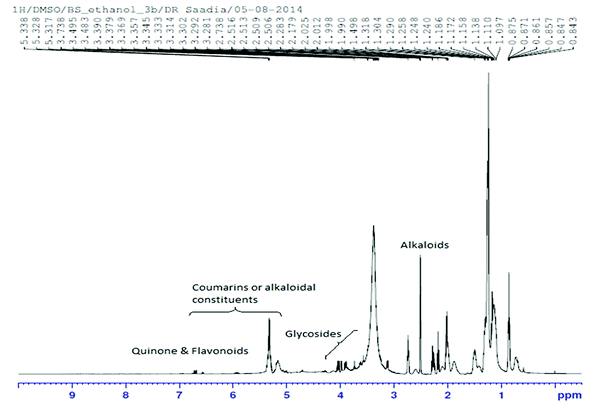 |
| Fig. 1
1H NMR spectrum of Nigella sativa seed extract in DMSO-D6 (D, 99.9%) + 0.05% v/v TMS. | |
Table 3 Compounds identified from Nigella sativa extract by 1H-NMR analysis
Compound name |
Alternative name |
Molecular formula |
Molecular weight |
Quercetin |
3,3′,4,5,7-Pentahydroxyflavone |
C15H10O7 |
302.2357 |
Thymoquinone |
p-Mentha-3,6-diene-2,5-dione |
C10H12O2 |
164.0837 |
Dihydroxythymoquinone |
3,6-Dihydroxy-p-mentha-3,6-diene-2,5-dione |
C10H12O4 |
196.0735 |
Coumarin |
2H-1-Benzopyran-2-one |
C9H6O2 |
146.1427 |
Serpentine |
Serpentine hydroxide |
C21H20N2O3 |
348.3951 |
Kaempferol |
Kaempferol 3-O-glucoside |
C21H20O11 |
448.3769 |
Histological examination of brain regional tissues
The histological assessment of the brain regional sections showed marked differences in the density of Nissl bodies among different experimental groups. The qualitative analysis of slides from cortex tissues showed that in the Pb-treated groups (0.1% Pb, 0.2% Pb) the cell density was notably reduced as compared to the healthy control group. However, in the 0.1% Pb + 250 mg and 500 mg Nigella sativa L.-treated groups, the cell density was improved due to the administration of Nigella sativa L. seed extract which may have enhanced the cell viability in Pb toxicity. Similar results were observed in the 0.2% Pb + 250 mg and 500 mg Nigella sativa L. seed extract-treated groups. The cortex tissues of the 250 mg and 500 mg Nigella sativa L.-treated groups were the same as the healthy control group (Fig. 2).
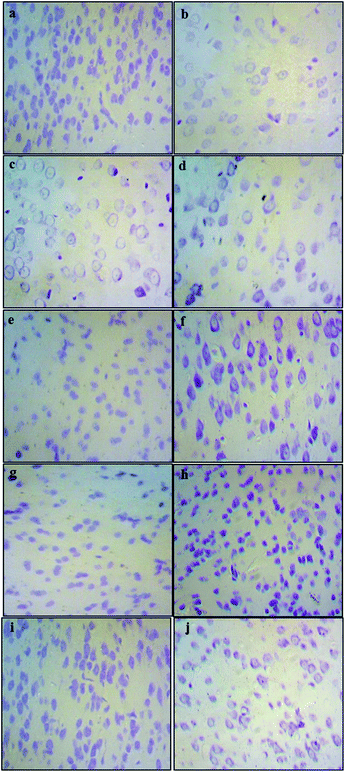 |
| Fig. 2 Nissl stained sections of the mouse cortex. The stained sections of the mouse cortex show marked neurotoxicity in comparison with the respective control group. Reduced cell count and structural deformity are also visible in the sections treated with Pb while restoration of cell count is evident after the administration of Nigella sativa L. seed extract. (a) Control, (b) Pb 0.1% treated, (c) Pb 0.2% treated, (d) Pb 0.1% + Nigella sativa L. (250 mg kg−1), (e) Pb 0.1% + Nigella sativa L. (500 mg kg−1), (f) Pb 0.2% + Nigella sativa L. (250 mg kg−1), (g) Pb 0.2% + Nigella sativa L. (500 mg kg−1), (h) Nigella sativa L. (250 mg kg−1), (i) Nigella sativa L. (500 mg kg−1), and (j) prophylactic group. | |
Effects of Pb and Nigella sativa L. seed extract on antioxidant enzymes
Pb treatment also resulted in a decreased expression of SOD1 observed in the 0.1% (0.26 ± 0.04 in the cortex and 0.28 ± 0.03 in the hippocampus) and 0.2% (0.22 ± 0.03 in the cortex and 0.22 ± 0.004 in the hippocampus) Pb-treated groups as compared to the control group (0.58 ± 0.01), whereas a significant increase in the expression was evident due to the co-administration of Nigella sativa L. seed extract (0.33 ± 0.002 in the cortex and in the hippocampus). No significant change in SOD1 expression was observed in the groups treated with Nigella sativa L. seed extract only. The prophylactic group showed a similar expression (0.51 ± 0.02 in the cortex and 0.52 ± 0.005 in the hippocampus) as observed in the control group.
Promising observations were also made for the Prdx6 expression, which was significantly decreased in the 0.1% (0.37 ± 0.01 in the cortex and 0.29 ± 0.01 in the hippocampus), and 0.2% (0.24 ± 0.01 in the cortex and 0.20 ± 0.006 in the hippocampus) Pb-treated groups as compared to the control group (0.63 ± 0.022). There was a significant increase in the expression of Prdx6 by co-administration of Nigella sativa L. seed extract as compared to the 0.1% (0.37 ± 0.01 in the cortex and 0.29 ± 0.01 in the hippocampus) and 0.2% Pb-treated groups (0.24 ± 0.01 in the cortex and 0.22 ± 0.06 in the hippocampus), and ultimately normalized similarly to the control group (0.58 ± 0.01). However, there was no difference in the expression of Prdx6 observed in the Nigella sativa L. seed extract only treated groups. The prophylactic group showed a similar expression to the control group (Fig. 3).
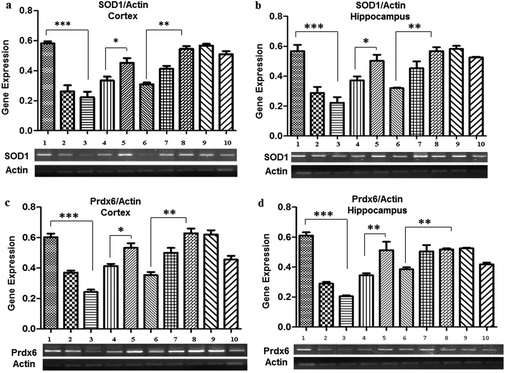 |
| Fig. 3 Gene expression analysis of oxidative stress markers: (a) SOD1 gene expression in the cortex, (b) SOD1 gene expression in the hippocampus, (c) Prdx6 gene expression in the cortex and (d) Prdx6 gene expression in the hippocampus of the mouse brain. In all these graphs, lane 1: control, 2: Pb 0.1% treated, 3: Pb 0.2% treated, 4: Pb 0.1% + Nigella sativa L. (250 mg kg−1), 5: Pb 0.1% + Nigella sativa L. (500 mg kg−1), 6: Pb 0.2% + Nigella sativa L. (250 mg kg−1), 7: Pb 0.2% + Nigella sativa L. (500 mg kg−1), 8: Nigella sativa L. (250 mg kg−1), 9: Nigella sativa L. (500 mg kg−1), and 10: prophylactic group. ***p < 0.001, **p < 0.01, *p < 0.05. | |
The effect of Pb and Nigella sativa L. seed extract on the gene expression of APP isoforms
Gene expression analysis of APP isoforms (APP Common, APP770, and APP695) was also performed by semi-quantitative PCR. The results demonstrated no marked difference in the expression level of APP common among all the groups, both in the cortex and the hippocampus (Fig. 4a and b).
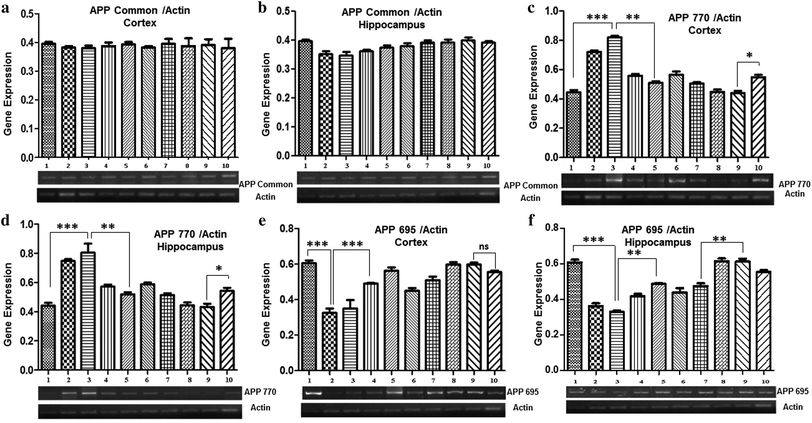 |
| Fig. 4 Gene expression analysis of APP isoforms: (a) APP common gene expression in the cortex, (b) APP common gene expression in the hippocampus, (c) APP770 gene expression in the cortex, (d) APP770 gene expression in the hippocampus, (e) APP695 gene expression in the cortex, and (f) APP695 gene expression in the hippocampus of the mice brain. In all these graphs, lane 1: control, 2: Pb 0.1% treated, 3: Pb 0.2% treated, 4: Pb 0.1% + Nigella sativa L. (250 mg kg−1), 5: Pb 0.1% + Nigella sativa L. (500 mg kg−1), 6: Pb 0.2% + Nigella sativa L. (250 mg kg−1), 7: Pb 0.2% + Nigella sativa L. (500 mg kg−1), 8: Nigella sativa L. (250 mg kg−1), 9: Nigella sativa L. (500 mg kg−1), and 10: prophylactic group. ***p < 0.001, **p < 0.01, *p < 0.05. | |
A significant increase in the expression of APP770 was observed in both the cortex and hippocampus of mice treated with 0.1% Pb (0.72 ± 0.01 in the cortex and 0.74 ± 0.04 in the hippocampus) and 0.2% Pb (0.82 ± 0.06 in the cortex and 0.80 ± 0.06 in the hippocampus) as compared to the control group (0.44 ± 0.01), whereas no significant difference was observed among the groups treated with Nigella sativa L. seed extract (250 mg kg−1 and 500 mg kg−1) and the control group. Interestingly, a significant decrease was observed after co-administration of 250 mg and 500 mg Nigella sativa L. seed extract in the 0.1% Pb (0.55 ± 0.04 in the cortex and 0.57 ± 0.01 in the hippocampus) and 0.2% Pb (0.50 ± 0.008 in the cortex and 0.58 ± 0.012 in the hippocampus) treated groups. The prophylactic group showed a slightly increased expression of APP as compared to the control group, however, it was markedly lower than the APP770 expression observed in the Pb-treated groups (0.54 ± 0.016 in the cortex and 0.54 ± 0.02 in the hippocampus) (Fig. 4c and d).
In both the cortex and hippocampus, the expression of APP695 was significantly (p < 0.01) decreased in the 0.1% Pb (0.35 ± 0.03 in the cortex and 0.36 ± 0.014 in the hippocampus) and 0.2% Pb treated groups (0.30 ± 0.03 in the cortex and 0.33 ± 0.007 in the hippocampus) in comparison with the control group (0.60 ± 0.01). However, the co-administration of Nigella sativa L. seed extract (250 mg kg−1 and 500 mg kg−1) showed a substantial increase in its expression level. A similar expression was also observed for the groups treated with 250 mg and 500 mg Nigella sativa L. seed extract as compared to the control group. The prophylactic group also showed a gradual increasing trend in the expression of APP695 (Fig. 4e and f). These observations have indicated the significant potential of Nigella sativa L. seed extract for stabilizing APP695 expression.
Discussion
The present study aimed to evaluate the toxicological and protective effects of Pb and Nigella sativa L., respectively. The free radical scavenging activity of Nigella sativa L. seed extract indicated its strong antioxidant potential which is equivalent to vitamin C, used as a control. Drastic histopathological modifications with a decreased number of cells and the absence of Nissl bodies in the Pb-treated groups revealed the deleterious effects of Pb on the brain during its development. However, the Pb groups treated with Nigella sativa L. seed extract showed more Nissl bodies, indicative of cell survival in these groups. Thus, these results prove the strong ability of Nigella sativa L. for reducing the Pb-induced toxicity and improving cell survival.
Our findings indicated that Pb exposure (0.1% and 0.2%) resulted in a notable downregulation in the expression of SOD1 in both the hippocampus and the cortex as compared to the control group. Pb increases the amount of free radicals which may lead to reduced activity and expression of SOD1.29 For brain development, the first 21 days after birth is the most critical period as the nervous system is prone to deleterious effects of heavy metals including Pb.30 The ethanolic Nigella sativa L. seed extract dietary supplementation helped in combating the oxidative damage and significantly enhanced the expression of SOD1. Interestingly, varied effects of Pb exposure on SOD1 were reported. The study by Moreira et al.31 reported the downregulation of SOD1 activity, while Soltaninejad et al.32 and Kim et al.33 reported a significant increase in SOD1 expression. However, these variations can be associated with the difference in the dosage and duration of Pb exposure. The reduced expression of SOD1 has also been linked to an early form of dementia enhanced accumulation of amyloid plaques and tau phosphorylation.34,35
Prdx6, another important cytosolic member of mammalian antioxidant defense systems, plays an important role in degrading H2O2 and other peroxides by using thiol groups of their cysteines as catalytically active centers.36 The downregulation of Prdx6 as observed in the current study is associated with the development of various pathological consequences including oxidative stress.37 Prdx6 is extensively involved in the healing of damaged skin by protecting blood vessels, during development38,39 and plays an important role in protection against oxidative damage-induced cataract formation,40 atherosclerosis,41 and parkinsonian dementia.42 Remarkably, the Pb exposure (0.1% and 0.2% Pb-treated groups) caused the downregulation of Prdx6 in a dose dependent manner. However, the significant upregulation in the expression of Prdx6 can be attributed to the antioxidant potential of Nigella sativa L., which reduces the ROS burden via quenching the free radicals.
Exposure to Pb during the early stages of growth and development induces alterations in gene expression in the brain.43 It also facilitates Aβ fibril formation and contributes to the accumulation of amyloid plaques.44 Therefore, the present study also elucidated the perturbations in the gene expression and transcript processing of APP due to Pb exposure during early development in mice. Moreover, we also assessed the potential of Nigella sativa L. extract to reverse these developmental changes. Our results indicated that Pb exposure causes significant changes in the expression of the APP isoforms during early life exposure and affects the NMDAR number in brain tissues.45 Enhanced NMDAR activation can cause transcriptional changes at the baseline. It can shift the expression of APP deficient KPI to KPI containing APP in the neuronal cells which results in altered expression and accumulation of Aβ.46,47 Relative expression analysis of APP770 in the hippocampus and the cortex which is a KPI containing APP, showed enhanced expression in Pb (0.1% and 0.2% Pb-treated groups) as compared to the control group. However, this trend was rerouted towards normal expression of APP770 in the Nigella sativa L. treated groups and was comparable to the control group. Pb competes and replaces calcium in cells thereby reducing the activity of enzymes and pathways associated with calcium signaling.48,49 Hence it is proposed that Nigella sativa L. may exert its effects via regulation of calcium signaling pathways or by reducing the activation of NMDAR.
Moreover, the reduced level of App695 (KPI deficient APP) in the Pb exposed pups (0.1% and 0.2% Pb treated groups) also suggested that the Pb exposure may cause a shift from APP695 to APP770 making neuronal cell more susceptible to amyloid deposition leading to the development of AD like pathologies. Similarly, the gene expression in the prophylactic group has also confirmed the protective effects of Nigella sativa L., however, an in depth understanding is required to elucidate the underlying mechanisms through which Nigella sativa L. exerts its effects at the transcription level.
Conclusion
The deleterious effects of Pb during development and early life were greatly reduced when supplemented with Nigella sativa L. seed extract, suggesting its strong antioxidant and neuroprotective potentials. However, further characterization of the compounds of Nigella sativa L. seed extract is required to determine the exact mechanism by which the phytochemicals exert antioxidant and neuroprotective effects.
Authors' roles
S. Z. has made a substantial contribution to the conception and design of the study and finalization of the manuscript; S. A. A. S. has made a substantial contribution to the acquisition and interpretation of the 1H NMR data; T. A. helped in the analysis of gene expression data and histological assessment; U. J. B. helped in all experimental work, analysis and interpretation of data, and drafting the article. All authors read and approved the final manuscript.
Conflicts of interest
The authors declare that there are no conflicts of interest.
Acknowledgements
This research was supported through MS student's research grant by the National University of Sciences and Technology (NUST), Islamabad, Pakistan. The authors would like to thank Dr Muhammad Qasim Hayat, expert in medicinal plant chemistry, Plant Biotechnology Department, ASAB, NUST, for providing laboratory facilities for plant extract preparation.
References
- K. Kalia and S. J. Flora, Strategies for safe and effective therapeutic measures for chronic arsenic and lead poisoning, J. Occup. Health, 2005, 47, 1–21 CrossRef CAS PubMed.
- M. C. Senut, P. Cingolani, A. Sen, A. Kruger, A. Shaik, H. Hirsch, S. T. Suhr and D. Ruden, Epigenetics of early-life lead exposure and effects on brain development, Epigenomics, 2012, 4, 665–674 CrossRef CAS PubMed.
- N. Ercal, H. Gurer-Orhan and N. Aykin-Burns, Toxic metals and oxidative stress part I: mechanisms involved in metal-induced oxidative damage, Curr. Trends Med. Chem., 2001, 1, 529–539 CrossRef CAS.
- Y. Ding, H. C. Gonick and N. D. Vaziri, Lead promotes hydroxyl radical generation and lipid peroxidation in cultured aortic endothelial cells, Am. J. Hypertens., 2000, 13, 552–555 CrossRef CAS PubMed.
- P. Grandjean and P. J. Landrigan, Developmental neurotoxicity of industrial chemicals, Lancet, 2006, 368, 2167–2178 CrossRef CAS.
- N. H Zawia and M. R. Basha, Environmental risk factors and the developmental basis for Alzheimer's disease, Rev. Neurosci., 2005, 16, 325–337 CAS.
- S. D. Bilbo, J. C. Biedenkapp, A. Der-Avakian, L. R. Watkins, J. W. Rudy and S. F. Maier, Neonatal infection-induced memory impairment after lipopolysaccharide in adulthood is prevented via caspase-1 inhibition, J. Neurosci., 2005, 25, 8000–8009 CrossRef CAS PubMed.
- M. Edwards, Fetal death and reduced birth rates associated with exposure to lead-contaminated drinking water, Environ. Sci. Technol., 2014, 48, 739–746 CrossRef CAS PubMed.
- R. L. Canfield, C. R. Henderson Jr., D. A. Cory-Slechta, C. Cox, T. A. Jusko and B. P. Lanphear, Intellectual impairment in children with blood lead concentrations below 10 μg per deciliter, N. Engl. J. Med., 2003, 348, 1517–1526 CrossRef CAS PubMed.
- R. E. Green and D. J. Pain, Potential health risks to adults and children in the UK from exposure to dietary lead in gamebirds shot with lead ammunition, Food Chem. Toxicol., 2012, 50, 4180–4190 CrossRef CAS PubMed.
- M. Ahamed, M. J. Akhtar, S. Verma, A. Kumar and M. K. Siddiqui, Enviornmental lead exposure as a risk for childhood aplastic anemia, BioSci. Trends, 2011, 5, 38–43 CrossRef CAS PubMed.
- S. Kim, M. Arora, C. Fernandez, J. Landero, J. Caruso and A. Chen, Lead, mercury, and cadmium exposure and attention deficit hyperactivity disorder in children, Environ. Res., 2013, 126, 105–110 CrossRef CAS PubMed.
- N. Pawlas, K. Broberg, S. Skerfving and K. Pawlas, Disturbance of posture in children with very low lead exposure, and modification by VDR FokI genotype, Ann. Agric. Environ. Med., 2014, 21, 739–744 CrossRef PubMed.
- S. Shah-Kulkarni, M. Ha, B. M. Kim, E. Kim, Y. C. Hong, H. Park, Y. Kim, B. N. Kim, N. Chang, S. Y. Oh, Y. J. Kim, B. Lee and E. H. Ha, Neurodevelopment in early childhood affected by prenatal lead exposure and iron intake, Medicine, 2016, 95, e2508 CrossRef CAS PubMed.
- H. Needleman, Lead poisoning, Annu. Rev. Med., 2004, 55, 209–222 CrossRef CAS PubMed.
- M. R. Basha, W. Wei, S. A. Bakheet, N. Benitez, H. K. Siddiqi, Y. W. Ge, D. K. Lahiri and N. H. Zawia, The fetal basis of amyloidogenesis: exposure to lead and latent overexpression of amyloid precursor protein and β-amyloid in the aging brain, J. Neurosci., 2005, 25, 823–829 CrossRef CAS PubMed.
- J. Wu, M. R. Basha, B. Brock, D. P. Cox, F. Cardozo-Pelaez, C. A. McPherson, J. Harry, D. C. Rice, B. Maloney, D. Chen, D. K. Lahiri and N. H. Zawia, Alzheimer's disease (AD)-like pathology in aged monkeys after infantile exposure to environmental metal lead (Pb):
evidence for a developmental origin and environmental link for AD, J. Neurosci., 2008, 28, 3–9 CrossRef CAS PubMed.
- A. Ahmad, A. Husain, M. Mujeeb, S. A. Khan, A. K. Najmi, N. A. Siddique, Z. A. Damanhouri and F. Anwar, A review on therapeutic potential of Nigella sativa: A miracle herb, Asian Pac. J. Trop. Biomed., 2013, 3, 337–352 CrossRef PubMed.
- M. Hanafy and M. Hatem, Studies on the antimicrobial activity of Nigella sativa seed (black cumin), J. Ethnopharmacol., 1991, 34, 275–278 CrossRef CAS PubMed.
- M. El Gazzar, R. El Mezayen, J. C. Marecki, M. R. Nicolls, A. Canastar and S. C. Dreskin, Anti-inflammatory effect of thymoquinone in a mouse model of allergic lung inflammation, Int. Immunopharmacol., 2006, 6, 1135–1142 CrossRef CAS PubMed.
- M. Burits and F. Bucar, Antioxidant activity of Nigella sativa essential oil, Phytother. Res., 2000, 14, 323–328 CrossRef CAS PubMed.
- N. Erkan, G. Ayranci and E. Ayranci, Antioxidant activities of rosemary (Rosmarinus Officinalis L.) extract, blackseed (Nigella sativa L.) essential oil, carnosic acid, rosmarinic acid and sesamol, Food Chem., 2008, 110, 76–82 CrossRef CAS PubMed.
- R. Singh, N. Singh, B. S. Saini and H. S. Rao, In vitro antioxidant activity of pet ether extract of black pepper, Indian J. Pharmacol., 2008, 40, 147–151 CrossRef PubMed.
- P. Gautam and S. Flora, Concomitant supplementation of gossypin, a bioflavonoid protects lead and ethanol induced oxidative stress in rats, J. Cell Tissue Res., 2011, 11, 2723–2729 Search PubMed.
- V. Pachauri, G. Saxena, A. Mehta, D. Mishra and S. J. Flora, Combinational chelation therapy abrogates lead-induced neurodegeneration in rats, Toxicol. Appl. Pharmacol., 2009, 240, 255–264 CrossRef CAS PubMed.
- M. A. Hadjzadeh, A. Khoei, Z. Hadjzadeh and M. Parizady, Ethanolic extract of nigella sativa L seeds on ethylene glycol-induced kidney calculi in rats, Urol. J., 2009, 4, 86–90 Search PubMed.
- Y. Kocyigit, Y. Atamer and E. Uysal, The effect of dietary supplementation of Nigella sativa L, on serum lipid profile in rats, Saudi Med. J., 2009, 30, 893–896 Search PubMed.
- G. J. Gage, D. R. Kipke and W. Shain, Whole animal perfusion fixation for rodents, J. Visualized Exp., 2012, 65 Search PubMed , pii: 3564.
- R. Sivaprasad, M. Nagaraj and P. Varalakshmi, Lipoic acid in combination with a chelator ameliorates lead-induced peroxidative damages in rat kidney, Arch. Toxicol., 2002, 76, 437–441 CrossRef CAS PubMed.
- H. Widmer, E. Bütikofer, M. Schlumpf and W. Lichtensteiger, Pre-and postnatal lead exposure affects the serotonergic system in the immature rat brain, Experientia, 1991, 47, 463–466 CrossRef CAS PubMed.
- E. G. Moreira, G. J. Rosa, S. B. Barros, V. S. Vassilieff and I. Vassillieff, Antioxidant defense in rat brain regions after developmental lead exposure, Toxicology, 2001, 169, 145–151 CrossRef CAS PubMed.
- K. Soltaninejad, A. Kebriaeezadeh, B. Minaiee, S. N. Ostad, R. Hosseini, E. Azizi and M. Abdollahi, Biochemical and ultrastructural evidences for toxicity of lead through free radicals in rat brain, Hum. Exp. Toxicol., 2003, 22, 417–423 CAS.
- S. Kim, J. Hyun, H. Kim, Y. Kim, E. Kim, J. Jang and K. Kim, Effects of lead exposure on nitric oxide-associated associated gene expression in the olfactory bulb of mice, Biol. Trace Elem. Res., 2011, 142, 683–692 CrossRef CAS PubMed.
- P. A. Adlard, R. A. Cherny, D. I. Finkelstein, E. Gautier, E. Robb, M. Cortes, I. Volitakis, X. Liu, J. P. Smith and K. Perez, Rapid restoration of cognition in Alzheimer's transgenic mice with 8-hydroxy quinoline analogs is associated with decreased interstitial Aβ, Neuron, 2008, 59, 43–55 CrossRef CAS PubMed.
- E. D. Roberson, K. Scearce-Levie, J. J. Palop, F. Yan, I. H. Cheng, T. Wu, H. Gerstein, G. Q. Yu and L. Mucke, Reducing endogenous tau ameliorates amyloid β-induced deficits in an Alzheimer's disease mouse model, Science, 2007, 316, 750–754 CrossRef CAS PubMed.
- A. Perkins, K. J. Nelson, D. Parsonage, L. B. Poole and P. A. Karplus, Peroxiredoxins: guardians against oxidative stress and modulators of peroxide signaling, Trends Biochem. Sci., 2015, 40, 435–445 CrossRef CAS PubMed.
- R. A. Poynton and M. B. Hampton, Peroxiredoxins as biomarkers of oxidative stress, Biochim. Biophys. Acta, 2014, 1840, 906–912 CrossRef CAS PubMed.
- A. Kümin, C. Huber, T. Rülicke, E. Wolf and S. Werner, Peroxiredoxin 6 is a potent cytoprotective enzyme in the epidermis, Am. J. Pathol., 2006, 169, 1194–1205 CrossRef PubMed.
- A. Kümin, M. Schäfer, N. Epp, P. Bugnon, C. Born-Berclaz, A. Oxenius, A. Klippel, W. Bloch and S. Werner, Peroxiredoxin 6 is required for blood vessel integrity in wounded skin, J. Cell Biol., 2007, 179, 747–760 CrossRef PubMed.
- J. H. Pak, T. I. Kim, M. Joon Kim, J. Yong Kim, H. J. Choi, S. A. Kim and H. Tchah, Reduced expression of 1-cys peroxiredoxin in oxidative stress-induced cataracts, Exp. Eye Res., 2006, 82, 899–906 CrossRef CAS PubMed.
- X. Wang, S. A. Phelan, C. Petros, E. F. Taylor, G. Ledinski, G. Jürgens, K. Forsman-Semb and B. Paigen, Peroxiredoxin 6 deficiency and atherosclerosis susceptibility in mice: significance of genetic background for assessing atherosclerosis, Atherosclerosis, 2004, 177, 61–70 CrossRef CAS PubMed.
- J. H. Power, J. M. Shannon, P. C. Blumbergs and W. P. Gai, Nonselenium glutathione peroxidase in human brain: elevated levels in Parkinson's disease and dementia with lewy bodies, Am. J. Pathol., 2002, 161, 885–894 CrossRef CAS PubMed.
- J. S. Schneider, W. Mettil and D. W. Anderson, Differential effect of postnatal lead exposure on gene expression in the hippocampus and frontal cortex, J. Mol. Neurosci., 2012, 47, 76–88 CrossRef CAS PubMed.
- H. Gu, G. Robison, L. Hong, R. Barrea, X. Wei, M. R. Farlow, Y. N. Pushkar, Y. Du and W. Zheng, Increased β-amyloid deposition in Tg-SWDI transgenic mouse brain following in vivo leadexposure, Toxicol. Lett., 2012, 213, 211–219 CrossRef CAS PubMed.
- T. R. Guilarte and J. L. McGlothan, Selective decrease in NR1 subunit splice variant mRNA in the hippocampus of Pb2+ exposed rats: implications for synaptic targeting and cell surface expression of NMDAR complexes, Mol. Brain Res., 2003, 113, 37–43 CrossRef CAS PubMed.
- K. Bordji, J. Becerril-Ortega, O. Nicole and A. Buisson, Activation of extrasynaptic, but not synaptic, NMDA receptors modifies amyloid precursor protein expression pattern and increases amyloid-β production, J. Neurosci., 2010, 30, 15927–15942 CrossRef CAS PubMed.
- P. Preece, D. J. Virley, M. Costandi, R. Coombes, S. J. Moss, A. W. Mudge, E. Jazin and N. J. Cairns, Amyloid precursor protein mRNA levels in Alzheimer's disease brain, Mol. Brain Res., 2004, 122, 1–9 CrossRef CAS PubMed.
- J. A. Lee, Y. Xing, D. Nguyen, J. Xie, C. J. Lee and D. L. Black, Depolarization and CaM kinase IV modulate NMDA receptor splicing through two essential RNA elements, PLos Biol., 2007, 5, e40 Search PubMed.
- G. E. Hardingham and H. Bading, The Yin and Yang of NMDA receptor signalling, Trends Neurosci., 2003, 26, 81–89 CrossRef CAS PubMed.
Footnote |
† Electronic supplementary information (ESI) available. See DOI: 10.1039/c7tx00201g |
|
This journal is © The Royal Society of Chemistry 2018 |