DOI:
10.1039/C8SC03754J
(Edge Article)
Chem. Sci., 2018,
9, 8871-8875
Copper-mediated 1,2-bis(trifluoromethylation) of arynes†
Received
22nd August 2018
, Accepted 20th September 2018
First published on 21st September 2018
Abstract
We herein describe an unprecedented 1,2-bis(trifluoromethylation) of arynes with [CuCF3] in the presence of an oxidant DDQ. The method allows the rapid construction of a new class of 1,2-bis(trifluoromethyl)arenes in one-step from aryne precursors under mild conditions. Its synthetic utility has been demonstrated in the preparation of bis(trifluoromethylated) molecules with potential pharmaceutical and materials science applications. Mechanistic studies indicated the presence of an o-trifluoromethyl aryl radical intermediate via CF3 group transfer from [CuCF3] to the aryne.
Introduction
Arynes are versatile reactive intermediates for the rapid synthesis of multifunctionalized arenes.1 In particular, multicomponent reactions of arynes in the presence of a transition metal can provide easy access to diverse 1,2-difunctionalized arenes.1a,b The two new bonds are formed in one step on the aryne intermediate to install orthogonal functional groups adjacent to each other (Scheme 1a). This strategy has only been recently applied by Hu's group and us to the synthesis of highly functionalized trifluoromethylated arenes, which are important building blocks in widely used pharmaceuticals and agrochemicals.2 Hu and co-workers developed a silver-mediated trifluoromethylation–iodination3 of arynes whereas we reported a copper-mediated trifluoromethylation–allylation protocol;4 both methods successfully realized vicinal difunctionalization of arenes involving C–CF3 bond and C–I/C–C bond construction in one-step from aryne intermediates. On the other hand, to install two identical vicinal functional groups onto arenes, metal-catalyzed aryne insertion to a σ-bond has been utilized. Under Pd, Cu or Pt catalysis, arynes/hetarynes can insert into heteroatom–heteroatom bonds such as Si–Si, Sn–Sn and B–B bonds to provide 1,2-bis(functionalized) arenes with high synthetic utility (Scheme 1b).5 However, such a method is not amenable to the preparation of bis-CF3 products due to the fact that an infeasible aryne insertion to a “CF3–CF3” bond would be required.6 We herein describe a new approach for 1,2-bis(functionalization) of arenes by reacting the aryne intermediate with an organometallic reagent twice, in this case [CuCF3], thereby achieving an unpresented 1,2-bis(trifluoromethylation) of arynes (Scheme 1c).
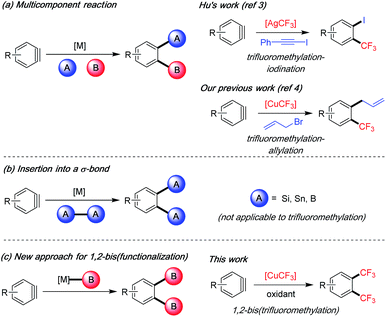 |
| Scheme 1 Transition metal-catalyzed/-mediated 1,2-difunctionalization of arynes and applications in trifluoromethylation reactions. | |
Results and discussion
During our investigation of the trifluoromethylation–allylation of aryne precursor 1a using the fluoroform-derived [CuCF3],4 we observed that the reaction produced an unexpected 1,2-bis(trifluoromethyl)arene product 2a (30% yield) when open to air without the electrophile allylbromide (Table 1, entry 1). The [CuCF3] reagent was prepared from CuCl, t-BuOK and CF3H as a solution in DMF according to Grushin's procedure,7,8 and stabilized with Et3N·3HF. The oxidative condition was crucial for the formation of 2a (Table 1, entry 2). Screening of various oxidants revealed that DDQ (2,3-dichloro-5,6-dicyano-1,4-benzoquinone) was capable of increasing the yield (58%) (Table 1, entries 3–7). A major side product was the mono-trifluoromethylated arene (regioisomeric mixture); its formation could be reduced by using DMSO as a co-solvent (1
:
1 ratio), thus further improving the yield (77%) (Table 1, entry 8). Reaction at room temperature was equally effective (Table 1, entry 9). Different DMF/DMSO ratios were tested showing that a larger amount of DMSO was generally beneficial for the reaction, and at 1
:
2 ratio (DMF/DMSO) the mono-CF3 side products could be completely suppressed (Table 1, entries 10 and 11). Other reaction parameters such as additives, co-solvents and reagent equivalents were screened with no further improvement.8 The reaction design requires at least two equivalents of [CuCF3] due to its role as a “carrier of CF3”; we found that four equivalents were necessary to provide the highest yield. However, this stable reagent can be prepared from inexpensive copper and CF3 sources at scale7d (fluoroform is an industrial byproduct and commercially available at <$0.10/mol),9 therefore justifying its use in excess.
Table 1 Optimization studies for 1,2-bis(trifluoromethylation) of aryne precursor 1aa
The scope of the reaction was subsequently investigated using various 2-(trimethylsilyl)aryltriflates 1 as the aryne precursors (Scheme 2). Although many methods are available for generating aryne intermediates,1f the 2-(trimethylsilyl)aryl triflates 1, developed by Kobayashi and co-workers in 1983,10 remain the most convenient and widely used precursors owing to the mild conditions (usually when exposed to fluoride) and broad synthetic applications.1a,bThey are either commercially available or can be prepared in a few steps at scale according to known procedures. In our reaction, conveniently no extra fluoride was needed to generate arynes from 1 due to the addition of Et3N·3HF as a stabilizer to the [CuCF3] reagent (in situ generating KF with t-BuOK).7 Moderate to good yields were obtained for symmetrical and unsymmetrical 1,2-bis(trifluoromethyl)arenes (2a–g). Functional groups such as acetal (2d), chloro (2l), bromo (2p, 2q), allyl (2n) and even silyl (2m) were tolerated. Hydroxy group (2r), on the other hand, was not compatible. Substituents adjacent to the reaction centre (2o, 2p) generally caused lower yields than remote substituents (2j, 2h). Oxabicyclic compounds containing the bis-CF3 moiety were also synthesized in reasonable yields (2s–u), they could serve as useful substrates for asymmetric ring-opening reactions11 towards drug analogue preparation. The bis-CF3 polyaromatic compound (2v) was also synthesized which may exhibit interesting material properties; the lower yield was mainly due to the solubility issue of the precursor. In some cases, larger amounts of DMSO were required to inhibit the formation of mono-CF3 side products (2e, 2f, 2k, 2t). Several products were very volatile, and their NMR yields were determined (2j, 2l, 2o). Pharmaceutical compounds containing two trifluoromethyl groups on arenes are well-precedented,12 however, the class of 1,2-bis(trifluoromethyl)arenes has been much less represented possibly due to the difficulty in their synthesis. Traditional methods often involved hazardous conditions (using SF4 and HF) and lengthy procedures from trifluoromethylated building blocks.13 Our approach is more operationally simple and general for synthesizing structurally diverse 1,2-bis(trifluoromethyl)arenes. Furthermore, aryne precursor 1w derived from estrone6 was successfully converted into the 1,2-bis(trifluoromethylated) derivative 2w (Scheme 3) demonstrating relevance of the current method to the modification of biologically active compounds.
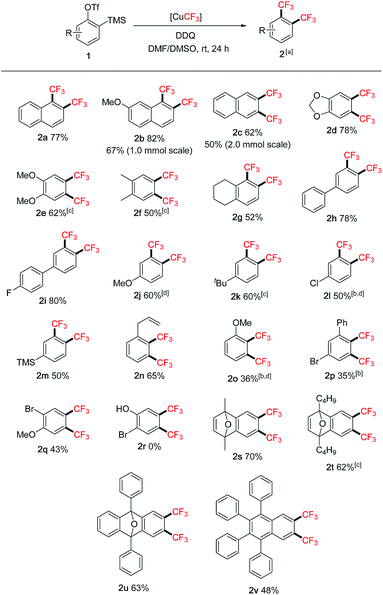 |
| Scheme 2 Scope of 1,2-bis(trifluoromethyl)arenes 2. aGeneral conditions: 1 (0.4 mmol), DDQ (0.8 mmol), [CuCF3] (1.6 mmol in 4.0 mL DMF), DMSO (8.0 mL), under argon. Isolated yields. bDMF : DMSO = 4.0 : 4.0 mL. cDMF : DMSO = 4.0 : 12.0 mL. dYield determined by 19F NMR analysis using benzotrifluoride as the internal standard. | |
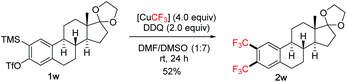 |
| Scheme 3 1,2-Bis(trifluoromethylation) of an estrone derivative. | |
The above 1,2-bis(trifluoromethyl)arenes 2 are useful intermediates for further transformations and their synthetic applications were explored (Scheme 4). Deoxygenation of compound 2u14 directly led to the bis(trifluoromethylated) 9,10-diphenylanthracene derivative 3. UV-Vis absorption and cyclic voltammetry (CV) studies showed decreased HOMO and LUMO energy levels compared with the parent compound (Scheme 4a).8 This “tuning” effect by the bis-CF3 groups could have potential applications in the development of organic semiconductors.15 Under protolytic defluorination protocols,16 trifluoromethylated ketone 4 could be obtained from 2e (Scheme 4b). The intramolecular reaction afforded compound 5 from 2p (Scheme 4c), which is a trifluoromethylated analogue of 9-fluorenone that has been recently shown as an effective metal-free photocatalyst.17 Finally, Sonogashira cross-coupling of 2q with a terminal alkyne afforded compound 6, which could provide access to bis(trifluoromethylated)benzofurans via cyclization of the –OMe group.18
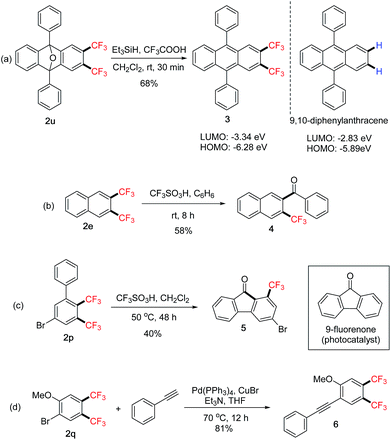 |
| Scheme 4 Further transformations of 1,2-bis(trifluoromethyl)arenes 2. | |
To gain more insights into the reaction mechanism, additional studies were performed (Scheme 5).8 In the 19F NMR experiment, we observed that the peak of the initial [CuICF3]7a quickly disappeared after adding DDQ and stirring for 5 min at rt, indicating a facile oxidation of [CuICF3] presumably to [CuIICF3]7c by DDQ (Scheme 5a). Subjecting mono-CF3 compound 7 to the standard conditions did not give any bis-CF3 products 2a or 2c, thus ruling out the C–H trifluoromethylation pathway (Scheme 5b).19 Adding a known radical scavenger TEMPO (2,2,6,6-tetramethyl-1-piperidinyloxy)20a to the reaction of 1d under standard conditions dramatically decreased the yield of 2d (Scheme 5c, 7% vs.78% cf.Scheme 2). Significant amounts of the mono-CF3 product (29%) and the CF3-containing dimer product (12%) were detected, however, only trace TEMPO–CF3 adduct was observed. Also, styrene derivatives20b,c were added to the standard conditions with 1d to trap any CF3-adducts, but only trace amounts were detected. These studies suggested that CF3 radicals were not likely to be present in the reaction. On the other hand, a radical clock experiment20d using substrate 1x gave both bis-CF3 product 2x and cyclized product 8, therefore hinting at the intermediacy of a transient aryl radical (Scheme 5d).
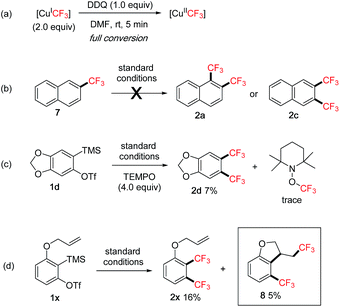 |
| Scheme 5 Mechanistic studies. | |
Based on the above studies and literature examples, we propose the following mechanism for the 1,2-bis(trifluoromethylation) of arynes (Scheme 6). The initial fluoroform-derived [CuICF3] is quickly oxidized by DDQ to [CuIICF3].7c The [CuIICF3] is capable of transferring a CF3 group to aryne A resulting in an aryl radical species B,21 supported by the radical clock experiment (cf.Scheme 5d) and our own observation of CF3 group transfer to alkenes with [CuIICF3] for generating alkyl radicals.22 Intermediate B reacts with a second equivalent of [CuIICF3] presumably leading to a CuIII–CF3 species C.20b Final reductive elimination affords the 1,2-bis(trifluoromethyl)arene product 2. Related reactions of aryl radicals and [CuCF3] to form aryl–CF3 bonds have been reported.23 There also exists the possibility that intermediate C may arise via carbocupration24 processes with [CuCF3] under oxidative conditions. For instance, aryne A may undergo carbocupration with a [Cu(CF3)n]25 species to give C. Alternatively, an arylcopper intermediate D may be formed first, which then reacts with another molecule of [CuIICF3] leading to C. It is difficult to pinpoint the exact pathway at the moment due to the complicated nature of the fluoroform-derived [CuCF3] reagent, especially in oxidized forms. A major side reaction was the formation of the mono-CF3 product E via protodemetallation of C (t-BuOH is present in the reagent and can act as a proton source). This pathway is inhibited by adding DMSO as a co-solvent, probably due to its role as a coordinating ligand to stabilize the copper complex C thus favouring reductive elimination.26
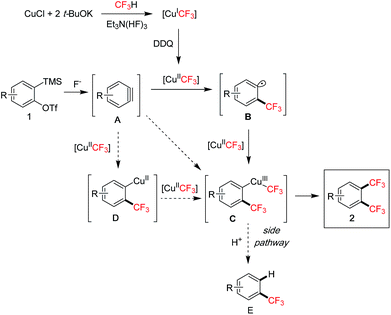 |
| Scheme 6 Proposed mechanism. | |
Conclusions
In conclusion, a novel 1,2-bis(trifluoromethylation) of arynes using [CuCF3] has been developed. By employing 2-(trimethylsilyl)aryl triflates as aryne precursors, structurally diverse 1,2-bis(trifluoromethyl)arenes can be synthesized in one-step under mild and safe conditions. Notably the ultimate source of CF3 in all of these valuable compounds is the inexpensive industrial waste fluoroform. New mechanistic insights will further the field of copper-mediated/-catalyzed trifluoromethylation–functionalization of arynes, and related studies are currently ongoing in our laboratory.
Conflicts of interest
There are no conflicts to declare.
Acknowledgements
This work was supported by the Research Grants Council of Hong Kong (CUHK 24301217) and the Chinese University of Hong Kong (the Faculty Strategic Fund for Research from the Faculty of Science and the Direct Grant for Research 4053276). Professor Qian Miao is also thanked for the use of their equipment.
Notes and references
- For reviews on aryne chemistry, see:
(a) R. A. Dhokale and S. B. Mhaske, Synthesis, 2018, 50, 1 CrossRef CAS;
(b) M. Feng and X. Jiang, Synthesis, 2017, 49, 4414 CrossRef CAS;
(c) J.-A. Garcia- López and M. F. Greaney, Chem. Soc. Rev., 2016, 45, 6766 RSC;
(d) R. Karmakar and D. Lee, Chem. Soc. Rev., 2016, 45, 4459 RSC;
(e) A. E. Goetz, T. K. Shah and N. K. Garg, Chem. Commun., 2015, 51, 34 RSC;
(f) S. Yoshida and T. Hosoya, Chem. Lett., 2015, 44, 1450 CrossRef CAS;
(g) C. Wu and F. Shi, Asian J. Org. Chem., 2013, 2, 116 CrossRef CAS;
(h) A. V. Dubrovskiy, N. A. Markina and R. C. Larock, Org. Biomol. Chem., 2013, 11, 191 RSC;
(i) P. M. Tadross and B. M. Stoltz, Chem. Rev., 2012, 112, 3550 CrossRef CAS PubMed;
(j) C. M. Gampe and E. M. Carreira, Angew. Chem., Int. Ed., 2012, 51, 3766 CrossRef CAS PubMed;
(k) H. H. Wenk, M. Winkler and W. Sander, Angew. Chem., Int. Ed., 2003, 42, 502 CrossRef CAS PubMed.
- For reviews on the synthesis and applications of trifluoromethylated arenes, see:
(a) C. Alonso, E. Martínez de Marigorta, G. Rubiales and F. Palacios, Chem. Rev., 2015, 115, 1847 CrossRef CAS PubMed;
(b) T. Liu and Q. Shen, Eur. J. Org. Chem., 2012, 2012, 6679 CrossRef CAS;
(c) O. A. Tomashenko and V. V. Grushin, Chem. Rev., 2011, 111, 4475 CrossRef CAS PubMed.
-
(a) Y. Zeng, L. Zhang, Y. Zhao, C. Ni, J. Zhao and J. Hu, J. Am. Chem. Soc., 2013, 135, 2955 CrossRef CAS PubMed;
(b) Y. Zeng and J. Hu, Chem.–Eur. J., 2014, 20, 6866 CrossRef CAS PubMed;
(c) Y. Zeng and J. Hu, Synthesis, 2016, 48, 2137 CrossRef CAS.
- X. Yang and G. C. Tsui, Org. Lett., 2018, 20, 1179 CrossRef CAS PubMed.
-
(a) H. Yoshida, J. Ikadai, M. Shudo, J. Ohshita and A. Kunai, J. Am. Chem. Soc., 2003, 125, 6638 CrossRef CAS PubMed;
(b) H. Yoshida, K. Tanino, J. Ohshita and A. Kunai, Angew. Chem., Int. Ed., 2004, 43, 5052 CrossRef CAS PubMed;
(c) H. Yoshida, S. Kawashima, Y. Takemoto, K. Okada, J. Ohshita and K. Takaki, Angew. Chem., Int. Ed., 2012, 51, 235 CrossRef CAS PubMed;
(d) M. Pareek, T. Fallon and M. Oestreich, Org. Lett., 2015, 17, 2082 CrossRef CAS PubMed.
- Aryne insertion in the disulfide S–S bond of CF3SSCF3 without transition metal has been reported for the synthesis of 1,2-bis(trifluoromethylthio)arenes, see: M. Mesgar and O. Daugulis, Org. Lett., 2017, 19, 4247 CrossRef CAS PubMed.
-
(a) A. Zanardi, M. A. Novikov, E. Martin, J. Benet-Buchholz and V. V. Grushin, J. Am. Chem. Soc., 2011, 133, 20901 CrossRef CAS PubMed;
(b) A. Lishchynskyi, M. A. Novikov, E. Martin, E. C. Escudero-Adán, P. Novák and V. V. Grushin, J. Org. Chem., 2013, 78, 11126 CrossRef CAS PubMed;
(c) P. Novak, A. Lishchynskyi and V. V. Grushin, Angew. Chem., Int. Ed., 2012, 51, 7767 CrossRef CAS PubMed;
(d) V. V. Grushin, Chem. Today, 2014, 32, 81 CAS.
- See ESI† for full details.
- J. B. Geri and N. K. Szymczak, J. Am. Chem. Soc., 2017, 139, 9811 CrossRef CAS PubMed.
- Y. Himeshima, T. Sonoda and H. Kobayashi, Chem. Lett., 1983, 12, 1211 CrossRef.
- M. Lautens and K. Fagnou, Proc. Natl. Acad. Sci. U. S. A., 2004, 101, 5455 CrossRef CAS PubMed.
- Y. Zhou, J. Wang, Z. Gu, S. Wang, W. Zhu, J. L. Aceña, V. A. Soloshonok, K. Izawa and H. Liu, Chem. Rev., 2016, 116, 422–518 CrossRef CAS PubMed.
-
(a) V. F. Lukmanov, L. A. Alekseeva, A. L. Burmakov and L. M. Yagupol’skij, J. Org. Chem. USSR, 1973, 9, 1046 Search PubMed;
(b) A. N. Alexeenko and V. P. Nazaretian, J. Fluorine Chem., 1994, 69, 241 CrossRef CAS;
(c) G.-D. Zhu, M. A. Staeger and S. A. Boyd, Org. Lett., 2000, 2, 3345 CrossRef CAS PubMed.
- N. Miyamoto, Y. Nakazawa, T. Nakamura, K. Okano, S. Sato, Z. Sun, H. Isobe and H. Tokuyama, Synlett, 2018, 29, 513 CrossRef CAS.
-
(a) H. Sun, A. Putta and M. Billion, J. Phys. Chem. A, 2012, 116, 8015 CrossRef CAS PubMed;
(b) Q. Miao, Adv. Mater., 2014, 26, 5541 CrossRef CAS PubMed.
-
(a) F. Wang and J. Hu, Chin. J. Chem., 2009, 27, 93 CrossRef CAS;
(b) A. Kethe, A. F. Tracy and D. A. Klumpp, Org. Biomol. Chem., 2011, 9, 4545 RSC.
- W. Schilling, D. Riemer, Y. Zhang, N. Hatami and S. Das, ACS Catal., 2018, 8, 5425 CrossRef CAS.
- A. J. Warner, A. Churn, J. S. McGough and M. J. Ingleson, Angew. Chem., Int. Ed., 2017, 56, 354 CrossRef CAS PubMed.
-
(a) M. Shang, S.-Z. Sun, H.-L. Wang, B. N. Laforteza, H.-X. Dai and J.-Q. Yu, Angew. Chem., Int. Ed., 2014, 53, 10439 CrossRef CAS PubMed;
(b) L. Chu and F.-L. Qing, J. Am. Chem. Soc., 2012, 134, 1298 CrossRef CAS PubMed.
-
(a) Z. Liang, F. Wang, P. Chen and G. Liu, Org. Lett., 2015, 17, 2438 CrossRef CAS PubMed;
(b) B.-S. Zhang, L.-Y. Gao, Z. Zhang, Y.-H. Wen and Y.-M. Liang, Chem. Commun., 2018, 54, 1185 RSC;
(c) N. Zhou, X. A. Yuan, Y. Zhao, J. Xie and C. Zhu, Angew. Chem., Int. Ed., 2018, 57, 3990 CrossRef CAS PubMed;
(d) A. Lishchynskyi, G. Berthon and V. V. Grushin, Chem. Commun., 2014, 50, 10237 RSC.
- K. Okuma, S. Sonoda, Y. Koga and K. Shioji, J. Chem. Soc., Perkin Trans. 1, 1999, 2997 RSC.
- X. Yang, L. He and G. C. Tsui, Org. Lett., 2017, 19, 2446 CrossRef CAS PubMed.
-
(a) G. Danoun, B. Bayarmagnai, M. F. Grüberg and L. J. Gooßen, Angew. Chem., Int. Ed., 2013, 52, 7972 CrossRef CAS PubMed;
(b) J.-J. Dai, C. Fang, B. Xiao, J. Yi, J. Xu, Z.-J. Liu, X. Lu, L. Liu and Y. Fu, J. Am. Chem. Soc., 2013, 135, 8436 CrossRef CAS PubMed; for alkyl radicals, see:
(c) X. Tan, Z. Liu, H. Shen, P. Zhang, Z. Zhang and C. Li, J. Am. Chem. Soc., 2017, 139, 12430 CrossRef CAS PubMed;
(d) H. Shen, Z. Liu, P. Zhang, X. Tan, Z. Zhang and C. Li, J. Am. Chem. Soc., 2017, 139, 9843 CrossRef CAS PubMed.
-
(a) D. S. Surry and D. R. Spring, Chem. Soc. Rev., 2006, 35, 218–225 RSC;
(b) Y. Shimizu and M. Kanai, Tetrahedron Lett., 2014, 55, 3727 CrossRef CAS;
(c)
S. J. Aves and D. R. Spring, in Patai Series: The Chemistry of Functional Groups. The Chemistry of Organocopper Compounds, ed. Z. Rappoport and I. Marek, John Wiley & Sons Ltd, Chichester, 2009, vol. 24, pp. 585–602 Search PubMed.
- [CuICF3] can be oxidized to [CuIII(CF3)4]−, although we did not detect any NMR signals for [CuIII(CF3)n] species upon oxidation with DDQ, see: A. M. Romine, N. Nebra, A. I. Konovalov, E. Martin, J. BenetBuchholz and V. V. Grushin, Angew. Chem., Int. Ed., 2015, 54, 2745 CrossRef CAS PubMed.
-
(a) M. Calligaris and O. Carugo, Coord. Chem. Rev., 1996, 153, 83 CrossRef CAS;
(b) W. Zierkiewicz and T. Privalov, Organometallics, 2005, 24, 6019 CrossRef CAS.
Footnote |
† Electronic supplementary information (ESI) available. See DOI: 10.1039/c8sc03754j |
|
This journal is © The Royal Society of Chemistry 2018 |
Click here to see how this site uses Cookies. View our privacy policy here.