DOI:
10.1039/C7SC05447E
(Edge Article)
Chem. Sci., 2018,
9, 3276-3281
Access to benzo-fused nine-membered heterocyclic alkenes with a trifluoromethyl carbinol moiety via a double decarboxylative formal ring-expansion process under palladium catalysis†
Received
23rd December 2017
, Accepted 17th February 2018
First published on 23rd February 2018
Abstract
Direct access to pharmaceutically attractive benzo-fused nine-membered heterocyclic alkenes 3 with a trifluoromethyl carbinol moiety was achieved via a palladium-catalyzed double-decarboxylative formal ring-expansion process from six-membered trifluoromethyl benzo[d][1,3]oxazinones 1 to nine-membered trifluoromethyl benzo[c][1,5]oxazonines 3 in the presence of vinylethylene carbonates 2. Generation of a Pd-π-allyl zwitterionic intermediate was proposed in the catalytic cycle. The trifluoromethyl group in the benzoxazinanones 1 plays an important role throughout the transformation. Diastereoselective chemical transformations of products 3 were also demonstrated.
Introduction
Fluoro-functionalized heterocycles with diverse ring sizes and ring systems have been well studied in pharmaceuticals and agrochemicals.1 Thus, a remarkable number of publications have been dedicated to the development of efficient synthetic methods to construct fluoro-functionalized heterocycles.1,2 In particular, heterocyclic molecules with a trifluoromethyl carbinol moiety, i.e., CF3C(OR1)R2R3, have gathered much attention3–6 on account of their promising biological properties. Efavirenz4 (anti-HIV), trifluoromethylated artemisinins5 (anti-malarial), and fluralaner6 (insecticide and acaricide) are representative examples (Fig. 1).
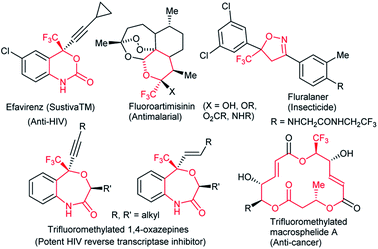 |
| Fig. 1 Biologically active heterocycles containing a trifluoromethyl carbinol moiety. | |
In this context, our group has been engaged in the development of novel synthetic methodologies for fluorine-containing heterocycles for decades.7 Including our reports,7 the present synthetic strategies for fluorinated heterocyclic molecules are mostly limited to the construction of five- and six-membered ring systems,1,2,7 while the synthesis of medium- to large-sized fluoro-functionalized heterocycles such as derivatives of benzo-oxazepine8 and macrosphelide A9 (Fig. 1) is extremely rare, despite the pharmaceutical importance of medium-sized heterocyclic compounds (non-fluorinated)10 and biologically active natural products.11 Very recently, Liu and co-workers reported an elegant method for the construction of fluoroalkyl-functionalized medium-/large-sized carbocyclic alkenes via an intramolecular radical trifluoromethylation–cyclization process.12 Recently, Zhao and co-workers successfully reported the palladium-catalyzed [5 + 4] and [6 + 4] cycloaddition reactions of azadienes with vinylethylene carbonates and vinyl oxetanes respectively in good yields and selectivities.13 We disclose herein the first synthesis of benzo-fused nine-membered heterocyclic alkenes 3 with a trifluoromethyl carbinol moiety and vinylethylene carbonates 214 (Scheme 1).
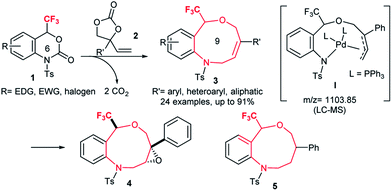 |
| Scheme 1 Direct access to benzo-fused nine-membered heterocyclic alkenes 3 with a trifluoromethyl carbinol moiety from six-membered oxazinones 1 and vinylethylene carbonates 2via palladium-catalyzed double decarboxylative cycloaddition and the further diastereoselective chemical transformations of 3. | |
The resulting trifluoromethylated heterocycles 3 have a benzo[c][1,5]oxazonine skeleton, and are not only medicinally attractive fluorine-containing heterocycles,1 but also expanded variants of well-known [1,4]oxazepine pharmaceuticals.15 Synthesis of the titled nine-membered compounds 3 were achieved from previously unknown trifluoromethylated benzoxazinanones 1 (six-membered ring) via a formal ring-expansion pathway under palladium catalysis. The reaction proceeded via the double decarboxylation (DDC)16 of 1 and vinylethylene carbonates 2 followed by a [5 + 4] cycloaddition reaction. The formation of Pd-complex I as an intermediate was proposed by LC-MS spectrometric analysis. This method provides an expedient access to trifluoromethylated benzo[c][1,5]oxazonines 3 with diverse functional groups in the aromatic moiety, including electron-donating, electron-deficient, and halogenic groups. Moreover, the alkene moiety in products 3 was further functionalized by conventional chemical transformations such as epoxidation to 4 and reduction to 5 (Scheme 1) with high diastereoselectivities which make this novel trifluoromethylated nine-membered skeleton more attractive as a template for drug discovery research. The presence of a trifluoromethyl group on 1 plays a pivotal role for their successful transformation to 3 based on comparative studies using non-CF3-varients of 1.
Results and discussion
We started a preliminary investigation with the reaction of trifluoromethyl (CF3) benzoxazinanone 1a and phenyl vinylethylene carbonate 2a in the presence of suitable palladium precursors and/or phosphine ligands (Table 1). We first attempted our reaction of 1a using similar Pd2(dba)3·CHCl3 conditions in the presence or absence of phosphine ligands, but the results were disappointing (entries 1–4). Moving on to Pd(PPh3)4 as a palladium precursor at 50 °C in THF furnished exclusively a nine-membered ring in good yield of 70% (entry 5). Motivated by this result, further optimization was carried out in different solvents. In toluene, a slight decrease in yield was observed, at 66% (entry 6), while in dichloroethane yield improved to 79% (entry 7). Lowering the temperature to room temperature (rt) furnished good yield (70%), but 40 hours were required to complete the reaction (entry 8). An excellent yield of 91% (89%) was observed by increasing the temperature to 80 °C (entry 9). Increasing the temperature further decreased yield dramatically (entry 10, see ESI for more details†).
Table 1 Optimization conditionsa
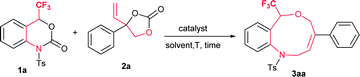
|
Entry |
Pd catalyst (with or without ligand) |
Solvent |
T [°C] |
t [h] |
Yieldc [%] |
Experiments were performed with 1a (0.1 mmol), 2a (0.15 mmol), 5 mol% Pd(PPh3)4 (0.05 mmol) in 1.0 mL solvent.
2a (0.12 mmol) was used.
Yields are 19F NMR yields with internal standard PhCF3 and yields (isolated) are also given in parentheses. dtbpmb = 1,2-bis(di-tert-butylphosphinomethyl)benzene. DCE = 1,2-dichloroethane.
|
1b |
10 mol% Pd2(dba)3·CHCl3 |
DCM |
rt |
24 |
— |
2b |
5 mol% Pd2(dba)3·CHCl3 |
THF |
40 |
24 |
— |
3 |
5 mol% Pd2(dba)3·CHCl3/10 mol% PCy3 |
THF |
40 |
24 |
— |
4 |
5 mol% Pd2(dba)3·CHCl3/10 mol% dtbpmb |
THF |
40 |
24 |
— |
5 |
5 mol% Pd(PPh3)4 |
THF |
50 |
7 |
75(70) |
6 |
5 mol% Pd(PPh3)4 |
Toluene |
50 |
36 |
68(66) |
7 |
5 mol% Pd(PPh3)4 |
DCE |
50 |
12 |
83(79) |
8 |
5 mol% Pd(PPh3)4 |
DCE |
rt |
40 |
79(70) |
9 |
5 mol% Pd(PPh3)4 |
DCE |
80 |
12 |
91(89) |
10 |
5 mol% Pd(PPh3)4 |
DCE |
Reflux |
12 |
40(34) |
Based on the optimized reaction conditions, the flexibility of the DDC reaction was scrutinized by using a broad array of vinylethylene carbonates (VECs) 2a–m with 1a. The results are summarized in Table 2. Both electron-withdrawing and electron-donating groups on the phenyl ring of 2 furnished good to excellent yields. VECs 2b–c, which have electron-donating groups (Me and OMe) at the p-position, reacted efficiently to afford the desired products 3 in excellent yields (3ab: 83%; 3ac: 78%) whereas VEC 2g, which contains an electron-withdrawing group (CF3) at the p-position, furnished moderate yield (3ag: 56%). Furthermore, halogen-substituted VECs (2d: F; 2e: Cl; 2f: Br) also underwent the DDC reaction very smoothly to furnish good to excellent yields (3ad: 69%; 3ae: 86%; 3af: 91%). Similarly, a highly electronegative atom (2h: F) and an electron-donating group (2i: OMe) at the o-position afforded excellent yields (3ah: 84% and 3ai: 88%). Noticeably, substrates bearing an electron-withdrawing group (F) and an electron-donating group (OMe) at the o-position furnished higher yields than p-substituted substrates. Moreover, the scope of VECs 2 was extended to heteroaryl systems (2j: 2-furyl; 2k: 2-thiophenyl) and the reaction proceeded smoothly to afford the desired products 3 in good yields (3aj: 76%; 3ak: 79%). Gratifyingly, non-aromatic substituent VEC 2l and extended π conjugate naphthalene-derived VEC 2m also underwent the cycloaddition reaction to furnish 3al and 3am in moderate to good yield (53% and 65%, respectively), thus significantly broadening the scope of substrate 2 of this DDC system (Table 2).
Table 2 Scope of vinylethylene carbonates (VECs) 2a
Experiments were performed with 1a (0.1 mmol), 2a–m (0.15 mmol), Pd(PPh3)4 (0.05 mmol) in 1.0 mL dry DCE with stirring at 80 °C for 12–16 h. Yields are isolated yields and 19F NMR yields with internal standard PhCF3 also shown in parentheses. 3aa: CCDC 1575063; 3aj: CCDC 1575065.
0.20 mmol of 2j was used.
0.20 mmol of 2k was used.
|
|
Spurred by this interesting result, a range of differently substituted CF3-benzoxazinanones 1b–e were further examined to better understand the DDC reaction (Table 3). Substituents on 1 with electronically dissimilar properties at different positions on the benzene ring were well tolerated to provide 3 in moderate to good yields. The substrate-bearing electron-donating methyl group on the benzene ring, 1b produced CF3-tetrahydrobenzoxazonine 3ba in 81% yield. The halogen-substituted CF3-benzoxazinanones 1c and 1e (F and Br) produced DDC products 3 in moderate to good yields (3ca: 69% and 3ea: 78%) (Table 3).
Table 3 Scope of benzoxazinanones 1a
Unless noted otherwise, the reaction was performed with 0.10 mmol of 1b–e as mentioned in Table 1. Yields are isolated yields and 19F NMR yields with internal standard (PhCF3) also shown in parentheses.
|
|
To ensure the effect of the CF3 group at the C-4 position, next we examined the reaction of benzoxazinanones 6, which contain different substituents at the C-4 position, with 2a (Scheme 2). In recent years, palladium-catalyzed cyclization reactions using vinyl benzoxazinanone 6a with a variety of substrates have been actively investigated by several groups.17 We thus first attempted the reaction of 6a with 2a. Interestingly, substrate 6a with a vinyl at the C-4 position produced a very different result. Under our best conditions, a vinyl-substituted benzoxazinanone 6a was converted to an intramolecular cyclization product 7 in 29% yield but no desired nine-membered cyclized product was observed (Scheme 2a). We next examined the reaction using 6b with a methyl group at the C-4 position instead, but were unable to furnish the desired product and the starting material 6b remained (Scheme 2b). Similar no conversion was obtained when we carried out the reaction of 6c having protected N-benzyl group (Scheme 2c). Although the reasons for the high reactivity of 1a are not clear, it might be due to the higher electrophilicity value of 1a induced by the strong electronegativity of the CF3 group (group electronegativity of CF3 is 3.45).18 To ensure the effect of the CF3 group at the C-4 position of 1a, we performed a DFT calculation. The electrophilic value of 1a having CF3 at the C-4 position was estimated to be 3.67 (ω (eV)) while that of 5b containing CH3 at the C-4 position (3.33) was lower (Table S6, Fig. S1 in ESI for details†).
 |
| Scheme 2 Reaction of benzoxazinanones 6a–c which contain different substituents at the C-4 position and N-protected group, with 2a under optimized conditions gave different results. | |
Interestingly, the X-ray crystallographic analysis of starting substrate 1a revealed that 1a has a sterically unfavourable cis-configuration between CF3 and tosyl groups (Fig. 2). Although the reasons for the stabilization of 1a in this configuration are not sure,19 the steric repulsion might be the additional factor for the high reactivity of 1a for decarboxylation reaction.
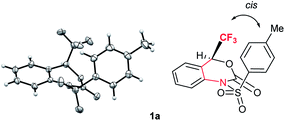 |
| Fig. 2 X-ray crystallographic analysis of 1a (CCDC 1575062†) revealed a sterically unfavourable cis-configuration between CF3 and tosyl groups. | |
To demonstrate the synthetic applicability of CF3-substituted tetrahydrobenzoxazonines 3, epoxidation and hydrogenation reactions were carried out as displayed in Scheme 3 based on the classical work of Still and Hoveyda.20 By using the Zhao's condition13a we performed the epoxidation of 3aa in the presence of m-CPBA at 0 °C to rt successfully transformed to epoxide 4 with 67% yield and >20
:
1 diastereoselectivity through the peripheral attack. The X-ray crystallographic structure of 4 (CCDC 1589030†) suggested that epoxidation proceeded via a less hindered convex approach. Hydrogenation of 3aa with H2 in the presence of Pd–C at rt furnished the desired product 5 (5
:
1 dr) in 74% yield (isolated as a single isomer) (Scheme 3).
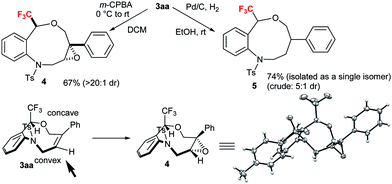 |
| Scheme 3 Diastereoselective derivatizations of tetrahydrobenzoxazonine 3aa. | |
A plausible reaction mechanism of the palladium-catalyzed DDC reaction of 1a with 2a to 3aa is portrayed in Scheme 4. The catalytic cycle is first initiated by the oxidative addition of Pd(0) with 2 followed by decarboxylation, which generates the π-allyl-Pd(II) complex II. The extremely nucleophilic nature of the alkoxide oxygen of II attacks the most electrophilic carbon atom attached to the CF3 group of 1a which triggers the opening of benzoxazinanone ring to generate reactive species III. Due to its highly reactive nature, species III immediately transforms into Pd-complex Ivia decarboxylation. Recently, Kleij et al. disclosed the similar kind of six membered Pd-complex with the support of DFT calculations.21 In our case, the formation of Pd-complex I was confirmed by LC-MS spectrometry (Fig. S2 in ESI for detail†) but we could not detect it by NMR (Fig. S3, in ESI for detail†).
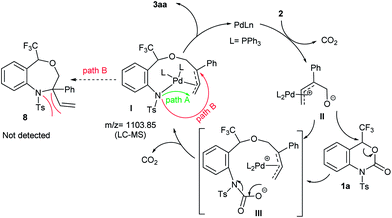 |
| Scheme 4 Plausible mechanism. | |
From complex I, there might be two possible pathways for the formation of two different cyclized products. Attack at the terminal position of the Pd-complex (path A) would generate the [5 + 4] cycloaddition product 3aa while internal attack (i.e., path B) of Pd-complex could result in [4 + 3] cycloaddition to furnish a seven-membered heterocycle 8. However, we did not obtain the [4 + 3] cycloaddition adduct 8. This may be attributed to steric hindrance of 8, i.e., the NTs group as well as the tetrasubstituted tertiary carbon center on 8.
Conclusions
In conclusion, we have established a novel and highly efficient methodology for the synthesis of benzo-fused nine-membered heterocyclic alkenes with a trifluoromethyl carbinol moiety through a palladium-catalyzed double decarboxylative formal ring expansion process. A combination of trifluoromethylated six-membered benzoxazinanones with vinylethylene carbonates resulted in direct access to previously unknown trifluoromethyl-functionalized nine-membered heterocycles. The trifluoromethyl substituent at the C-4 position of benzoxazinanones plays an important role in this transformation. Diastereoselective transformations of the benzo-fused nine-membered heterocyclic alkene were also achieved to demonstrate the synthetic utility of the products. Investigation of the formation of other medium-sized rings as well as enantioselective variants of the reaction are presently under way in our laboratory.
Conflicts of interest
There are no conflicts to declare.
Acknowledgements
This work was supported by JSPS KAKENHI Grant Number JP 16H01142 in the Middle Molecular Strategy, and the Advanced Catalytic Transformation (ACT-C) from the JST Agency (JPMJCR12Z7).
References
- Selected books and review:
(a)
V. A. Petrov, Fluorinated Heterocyclic Compounds: Synthesis, Chemistry and Applications, John Wiley & Sons, Inc., Hoboken, New Jersey, 2009 Search PubMed;
(b)
V. Nenajdenko, Fluorine in Heterocyclic Chemistry, Springer Cham, Heidelberg, New York, Dordrecht, London, 2014, vol. 1 Search PubMed;
(c)
V. Nenajdenko, Fluorine in Heterocyclic Chemistry, Springer Cham, Heidelberg, New York, Dordrecht, London, 2014, vol. 2 Search PubMed;
(d) J. Wang, M. S. Rosello, J. L. Acena, C. d. Pozo, A. E. Sorochinsky, S. Fustero, V. A. Soloshonok and H. Liu, Chem. Rev., 2014, 114, 2432–2506 CrossRef CAS PubMed;
(e)
A. A. Gakh and K. L. Kirk, Fluorinated Heterocycles, ACS Symposium Series, American Chemical Society, Washington, DC, 2009 Search PubMed;
(f) P. Das, E. Tokunaga and N. Shibata, Tetrahedron Lett., 2017, 58, 4803–4815 CrossRef CAS;
(g) H. Kawai and N. Shibata, Chem. Rec., 2014, 14, 1024–1040 CrossRef CAS PubMed.
-
(a) Y. V. Burgart, V. I. Saloutin and O. N. Chupakhin, Heterocycles, 2006, 69, 593–620 CrossRef CAS;
(b) V. Petrov and W. Marshall, J. Fluorine Chem., 2007, 128, 729–735 CrossRef CAS;
(c) G. K. S. Prakash, H. Vaghoo, C. Panja, A. Molnar, T. Mathew and G. A. Olah, Synthesis, 2008, 897–902 CrossRef CAS;
(d) Y. Kishi, H. Nagura, S. Inagi and T. Fuchigami, Chem. Commun., 2008, 3876–3878 RSC;
(e) P. Bannwarth, D. Gree and R. Gree, Tetrahedron Lett., 2010, 51, 2413–2415 CrossRef CAS;
(f) O. Lozano, G. Blessley, T. M. del Campo, A. L. Thompson, G. T. Giuffredi, M. Bettati, M. Walker, R. Borman and V. Gouverneur, Angew. Chem., Int. Ed., 2011, 50, 8105–8109 CrossRef CAS PubMed;
(g) B. T. Worrell, J. E. Hein and V. V. Fokin, Angew. Chem., Int. Ed., 2012, 51, 11791–11794 CrossRef CAS PubMed;
(h) H. Kawai, Y. Sugita, E. Tokunaga, H. Sato, M. Shiro and N. Shibata, Chem. Commun., 2012, 48, 3632–3634 RSC;
(i) G. Liu, Org. Biomol. Chem., 2012, 10, 6243–6248 RSC;
(j) D. Parmar and M. Rueping, Chem. Commun., 2014, 50, 13928–13931 RSC;
(k) J.-F. Zhao, X.-H. Duan, H. Yang and L.-N. Guo, J. Org. Chem., 2015, 80, 11149–11155 CrossRef CAS PubMed;
(l) J.-Q. Wu, S.-S. Zhang, H. Gao, Z. Qi, C.-J. Zhou, W.-W. Ji, Y. Liu, Y. Chen, Q. Li, X. Li and H. Wang, J. Am. Chem. Soc., 2017, 139, 3537–3545 CrossRef CAS PubMed.
-
(a) Y.-Y. Huang, X. Yang, Z. Chen, F. Verpoort and N. Shibata, Chem.–Eur. J., 2015, 21, 8664–8684 CrossRef CAS PubMed;
(b) A. Abouabdellah, J.-P. Begue, D. B. Delpon, J.-C. Gantier, T. T. T. Nga and T. D. Thac, Bioorg. Med. Chem. Lett., 1996, 6, 2717–2720 CrossRef CAS;
(c) S. Caron, N. M. Do, J. E. Sieser, P. Arpin and E. Vazquez, Org. Process Res. Dev., 2007, 11, 1015–1024 CrossRef CAS.
-
(a) S. M. E. Vrouenraets, F. W. N. M. Wit, J. V. Tongeren and J. M. A. Lange, Expert Opin. Pharmacother., 2007, 8, 851–871 CrossRef CAS PubMed;
(b) S. Li and J.-A. Ma, Chem. Soc. Rev., 2015, 44, 7439–7448 RSC;
(c) D. Mandala, W. A. Thompson and P. Watts, Tetrahedron, 2016, 72, 3389–3420 CrossRef CAS.
-
(a) J.-P. Begue and D. B. Delpon, ChemMedChem, 2007, 2, 608–624 CrossRef CAS PubMed;
(b) G. Magueur, B. Crousse, S. Charneau, P. Grellier, J.-P. Begue and D. B. Delpon, J. Med. Chem., 2004, 47, 2694–2699 CrossRef CAS PubMed.
-
(a) M. Gassel, C. Wolf, S. Noack, H. Williams and T. Ilg, Insect Biochem. Mol. Biol., 2014, 45, 111–124 CrossRef CAS PubMed;
(b) Y. Ozoe, Adv. Insect Physiol., 2013, 44, 211–286 Search PubMed;
(c) Y. Ozoe, M. Asahi, F. Ozoe, K. Nakahira and T. Mita, Biochem. Biophys. Res. Commun., 2010, 391, 744–749 CrossRef CAS PubMed;
(d) H. Williams, D. R. Young, T. Qureshi, H. Zoller and A. R. Heckeroth, Parasites Vectors, 2014, 7, 275, DOI:10.1186/1756-3305-7-275;
(e) J. Taenzler, C. Wengenmayer, H. Williams, J. Fourie, E. Zschiesche, R. K. Roepke and A. R. Heckeroth, Parasites Vectors, 2014, 7, 567, DOI:10.1186/s13071-014-0567-6.
-
(a) S. Ogawa, T. Nishimine, E. Tokunaga and N. Shibata, Synthesis, 2010, 3274–3281 CAS;
(b) S. Ogawa, N. Iida, E. Tokunaga, M. Shiro and N. Shibata, Chem.–Eur. J., 2010, 16, 7090–7095 CrossRef CAS PubMed;
(c) K. Matoba, H. Kawai, T. Furukawa, A. Kusuda, E. Tokunaga, S. Nakamura, M. Shiro and N. Shibata, Angew. Chem., Int. Ed., 2010, 49, 5762–5766 CrossRef CAS PubMed;
(d) H. Kawai, T. Kitayama, E. Tokunaga and N. Shibata, Eur. J. Org. Chem., 2011, 5959–5961 CrossRef CAS;
(e) H. Kawai, S. Okusu, E. Tokunaga, H. Sato, M. Shiro and N. Shibata, Angew. Chem., Int. Ed., 2012, 51, 4959–4962 CrossRef CAS PubMed;
(f) H. Kawai, Y. Sugita, E. Tokunaga, H. Sato, M. Shiro and N. Shibata, Chem. Commun., 2012, 48, 3632–3634 RSC;
(g) H. Kawai, T. Kitayama, E. Tokunaga, T. Matsumoto, H. Sato, M. Shiro and N. Shibata, Chem. Commun., 2012, 48, 4067–4069 RSC;
(h) H. Kawai, Y. Sugita, E. Tokunaga, H. Sato, M. Shiro and N. Shibata, ChemistryOpen, 2014, 3, 14–18 CrossRef CAS PubMed;
(i) Z. Huang, C. Wang, E. Tokunaga, Y. Sumii and N. Shibata, Org. Lett., 2015, 17, 5610–5613 CrossRef CAS PubMed.
- A. J. Cocuzza, D. R. Chidester, B. C. Cordova, R. M. Klabe, S. Jeffrey, S. Diamond, C. A. Weigelt, S. S. Ko, L. T. Bacheler, S. K. E. Viitanen and J. D. Rodgers, Bioorg. Med. Chem. Lett., 2001, 11, 1389–1392 CrossRef CAS PubMed.
-
(a) B. L. Wang, Z.-X. Jiang, Z.-W. You and F.-L. Qing, Tetrahedron, 2007, 63, 12671–12680 CrossRef CAS;
(b) D. P. Curran, M. K. Sinha, K. Zhang, J. J. Sabatini and D.-H. Cho, Nat. Chem., 2012, 4, 124–129 CrossRef CAS PubMed.
- Selected references for medium-size rings:
(a) R. E. TenBrink, J. M. McCall and H. G. Johnson, J. Med. Chem., 1980, 23, 1058–1060 CrossRef CAS PubMed;
(b) R. E. TenBrink, J. M. McCall, D. T. Pals, R. B. McCall, J. Orley, S. J. Humphrey and M. G. Wendling, J. Med. Chem., 1981, 24, 64–67 CrossRef CAS PubMed;
(c)
J. R. Tretter, US Pat., 3514449, 1970Chem. Abstr., 1970, 73, 35404;
(d)
J. Elks and C. R. Ganellin, Dictionary of Drugs, Chapman and Hall, London, 1st edn, 1990, p. 984 Search PubMed;
(e) Y. Satoh, A. H. Libby, C. Powers, T. J. Kowalski, D. H. White and E. F. Kimble, Bioorg. Med. Chem. Lett., 1994, 4, 549–552 CrossRef CAS;
(f) R. Kiyama, T. Honma, K. Hayashi, M. Ogawa, M. Hara, M. Fujimoto and T. Fujishita, J. Med. Chem., 1995, 38, 2728–2741 CrossRef CAS PubMed;
(g) K. C. Majumdar, RSC Adv., 2011, 1, 1152–1170 RSC;
(h) J. W. H. Watthey, T. Gavin and M. Desai, J. Med. Chem., 1984, 27, 816–818 CrossRef CAS PubMed.
- Selected references for medium-size ring containing natural products:
(a)
T. K. Devon and A. I. Scott in Handbook of Naturally Occurring Compounds, Academic Press, New York and London, 1972, vol. 2 Search PubMed;
(b) D. J. Faulkner, Nat. Prod. Rep., 1984, 1, 251–280 RSC;
(c) A. Hussain, S. K. Yousuf and D. Mukherjee, RSC Adv., 2014, 4, 43241–43257 RSC;
(d) J. Mallinson and I. Collins, Future Med. Chem., 2012, 4, 1409–1438 CrossRef CAS PubMed;
(e) D. M. Tapiolas, M. Roman, W. Fenical, T. J. Stout and J. Clardy, J. Am. Chem. Soc., 1991, 113, 4682–4683 CrossRef;
(f) F. Doi, T. Ohara, T. Ogamino, T. Sugai, K. Higashinakasu, K. Yamada, H. Shigemori, K. Hasegawa and S. Nishiyama, Phytochemistry, 2004, 65, 1405–1411 CrossRef CAS PubMed.
-
(a) L. Li, M. Deng, S.-C. Zheng, Y.-P. Xiong, B. Tan and X.-Y. Liu, Org. Lett., 2014, 16, 504–507 CrossRef CAS PubMed;
(b) J.-S. Lin, P. Yu, L. Huang, P. Zhang, B. Tan and X.-Y. Liu, Angew. Chem., Int. Ed., 2015, 54, 7847–7851 CrossRef CAS PubMed;
(c) Z.-J. Fang, S.-C. Zheng, Z. Guo, J.-Y. Guo, B. Tan and X.-Y. Liu, Angew. Chem., Int. Ed., 2015, 54, 9528–9532 CrossRef CAS PubMed;
(d) L. Li, Z.-L. Li, F. L. Wang, Z. Guo, Y.-F. Cheng, N. Wang, X.-W. Dong, C. Fang, J. Liu, C. Hou, B. Tan and X.-Y. Liu, Nat. Commun., 2016, 7, 13852 CrossRef PubMed;
(e) L. Li, Z.-L. Li, Q.-S. Gu, N. Wang and X.-Y. Liu, Sci. Adv., 2017, 3, e1701487, DOI:10.1126/sciadv.1701487.
-
(a) L.-C. Yang, Z.-Q. Rong, Y.-N. Wang, Z. Y. Tan, M. Wang and Y. Zhao, Angew. Chem., Int. Ed., 2017, 56, 2927–2931 CrossRef CAS PubMed;
(b) Z.-Q. Rong, L.-C. Yang, S. Liu, Z. Yu, Y.-N. Wang, Z. Y. Tin, R.-Z. Huang, Y. Lan and Y. Zhao, J. Am. Chem. Soc., 2017, 139, 15304–15307 CrossRef CAS PubMed;
(c) Y.-N. Wang, L.-C. Yang, Z.-Q. Rong, T.-L. Liu, R. Liu and Y. Zhao, Angew. Chem., Int. Ed., 2018, 57, 1596–1600 CrossRef CAS PubMed.
-
(a) A. Khan, L. Yang, J. Xu, L. Y. Jin and Y. J. Zhang, Angew. Chem., Int. Ed., 2014, 53, 11257–11260 CrossRef CAS PubMed;
(b) A. Khan, R. Zheng, Y. Kan, J. Ye, J. Xing and Y. J. Zhang, Angew. Chem., Int. Ed., 2014, 53, 6439–6442 CrossRef CAS PubMed;
(c) A. Khan, J. Xing, J. Zhao, Y. Kan, W. Zhang and Y. J. Zhang, Chem.–Eur. J., 2015, 21, 120–124 CrossRef CAS PubMed;
(d) L. Yang, A. Khan, R. Zheng, L. Y. Jin and Y. J. Zhang, Org. Lett., 2015, 17, 6230–6233 CrossRef CAS PubMed.
-
(a) P. G. Blain, Toxicol. Rev., 2003, 22, 103–110 CrossRef CAS PubMed;
(b) K. Nagarajan, J. David and G. A. Bhat, Indian J. Chem., 1985, 24, 840–844 Search PubMed;
(c) D. J. K. Balfour, Toxicolog, 1978, 9, 11–20 CrossRef CAS;
(d) J. M. Klunder, K. D. Hargrave, M. West, E. Cullen, K. Pal, M. L. Behnke, S. R. Kapadia, D. W. McNeil, J. C. Wu, G. C. Chow and J. Adams, J. Med. Chem., 1992, 35, 1887–1897 CrossRef CAS PubMed;
(e) E. A. Hallinan, T. J. Hagen, R. K. Husa, S. Tsymbalov, S. N. Rao, J.-P. vanHoeck, M. F. Rafferty, A. Stapelfeld, M. A. Savage and M. Reichman, J. Med. Chem., 1993, 36, 3293–3299 CrossRef CAS PubMed;
(f) M. Binaschi, A. Boldetti, M. Gianni, C. A. Maggi, M. Gensini, M. Bigioni, M. Parlani, A. Giolitti, M. Fratelli, C. Valli, M. Terao and E. Garattini, ACS Med. Chem. Lett., 2010, 1, 411–415 CrossRef CAS PubMed;
(g) A. Sapegin, S. Kalinin, A. Angeli, C. T. Supuran and M. Krasavin, Bioorg. Chem., 2018, 76, 140–146 CrossRef CAS PubMed.
- W. Guo, R. Kuniyil, J. E. Gomez, F. Maseras and A. W. Kleij, J. Am. Chem. Soc., 2018 DOI:10.1021/jacs.7b12608.
-
(a) T.-R. Li, F. Tan, L.-Q. Lu, Y. Wei, Y.-N. Wang, Y.-Y. Liu, Q.-Q. Yang, J.-R. Chen, D.-Q. Shi and W.-J. Xiao, Nat. Commun., 2014, 5, 5500 CrossRef CAS PubMed;
(b) Y. Wei, L.-Q. Lu, T.-R. Li, B. Feng, Q. Wang, W.-J. Xiao and H. Alper, Angew. Chem., Int. Ed., 2016, 55, 2200–2204 CrossRef CAS PubMed;
(c) L. A. Leth, F. Glaus, M. Meazza, L. Fu, M. K. Thogersen, E. A. Bitsch and K. A. Jorgensen, Angew. Chem., Int. Ed., 2016, 55, 15272–15276 CrossRef CAS PubMed;
(d) C. Guo, M. Fleige, D. J. Muller, C. G. Daniliuc and F. Glorius, J. Am. Chem. Soc., 2016, 138, 7840–7843 CrossRef CAS PubMed;
(e) Y.-N. Wang, B.-C. Wang, M.-M. Zhang, X.-W. Gao, T.-R. Li, L.-Q. Lu and W.-J. Xiao, Org. Lett., 2017, 19, 4094–4097 CrossRef CAS PubMed;
(f) C. Guo, D. J. Muller, M. Fleige, A. Lerchen, C. G. Daniliuc and F. Glorius, J. Am. Chem. Soc., 2017, 139, 4443–4451 CrossRef CAS PubMed.
- J. E. Huheey, J. Phys. Chem., 1965, 69, 3284–3291 CrossRef CAS.
- We found the close contact between F2C–F⋯C(Ar) (3.168 A) which is similar to the sum of the van der Waals radii of carbon and fluorine (3.05 A, Pauling 1939; 3.17 A Bondi, 1964). Thus, there might be the interaction between the π of the aromatic ring and fluorine. See the related references;
(a)
L. Pauling, The Nature of the Chemical Bond, Cornell Univ., Ithaca, 3rd edn, 1960 Search PubMed;
(b) A. Bondi, J. Phys. Chem., 1964, 68, 441–451 CrossRef CAS;
(c) K. Reichenbacher, H. I. Suss and J. Hulliger, Chem. Soc. Rev., 2005, 34, 22–30 RSC;
(d) J. A. K. Howard, V. J. Hoy, D. O'Hagan and G. T. Smith, Tetrahedron, 1996, 52, 12613–12622 CrossRef CAS;
(e) M. T. Scerba, S. Bloom, N. Haselton, M. Siegler, J. Jaffe and T. Lectka, J. Org. Chem., 2012, 77, 1605–1609 CrossRef CAS PubMed.
-
(a) A. H. Hoveyda, D. A. Evans and G. C. Fu, Chem. Rev., 1993, 93, 1307–1370 CrossRef CAS;
(b) W. C. Still and I. Galynker, Tetrahedron, 1981, 37, 3981–3996 CrossRef CAS;
(c) Z. Xu, C. W. Johannes, S. S. Salman and A. H. Hoveyda, J. Am. Chem. Soc., 1996, 118, 10926–10927 CrossRef CAS.
- W. Guo, L. M. Rodriguez, R. Kuniyil, E. Martin, E. C. E. Adan, F. Maseras and A. W. Kleij, J. Am. Chem. Soc., 2016, 138, 11970–11978 CrossRef CAS PubMed.
|
This journal is © The Royal Society of Chemistry 2018 |
Click here to see how this site uses Cookies. View our privacy policy here.