DOI:
10.1039/C8RA09048C
(Paper)
RSC Adv., 2018,
8, 41561-41565
Cu-catalyzed cross-coupling reactions of vinyl epoxide with organoboron compounds: access to homoallylic alcohols†
Received
1st November 2018
, Accepted 28th November 2018
First published on 12th December 2018
Abstract
Copper-catalyzed cross-coupling reactions of vinyl epoxide with arylboronates to obtain aryl-substituted homoallylic alcohols are described. The reaction selectivity was different to that of previously reported vinyl epoxide ring-opening reactions using aryl nucleophiles. The reaction proceeded under mild conditions, affording aryl-substituted homoallylic alcohols with high selectivity and in good to excellent yields. The reaction provides convenient access to aryl-substituted homoallylic alcohols from vinyl epoxide
Introduction
Transition-metal-catalyzed cross-couplings are among the most valuable C–C bond-forming reactions in modern organic synthesis.1 Compared with traditional carbon–halogen or carbon–OSO2R electrophiles,2 epoxides possess ring strain that makes them susceptible to ring opening. Through ring-opening reactions of epoxides, alcohol compounds can be easily obtained with the construction of a C–C bond.3 In recent years, various epoxide ring-opening reactions have been reported, including transition-metal-catalyzed Kumada and Negishi-type ring-opening/cross-coupling reactions of epoxides.4 Organoboron compounds have excellent stability and are commercially available. Therefore, many groups have reported Suzuki-type ring-opening/coupling reactions of epoxides.5 Furthermore, reductive ring-opening/coupling reactions and Heck-type reactions of epoxides have recently been reported.6
Vinyl epoxide is a special type of epoxide with rich chemical reactivity. The chemistry of vinyl epoxides is uniquely characterized by the conjugated reactivity of the epoxide and carbon–carbon double bond. Vinyl epoxides are easily prepared using simple synthetic routes and are important building blocks with vast potential in organic synthesis.7 Owing to its unique structural features, vinyl epoxide is prone to SN2′-type ring-opening/coupling reactions with aryl nucleophiles, affording alcohols containing a C
C bond. Some research groups have reported ring-opening/coupling reactions of vinyl epoxide with various types of aryl nucleophiles, including aryl bismuth compounds,8 aryl Grignard reagents,9 aryl boron compounds,10 aryl siloxane reagents,11 and organotin compounds (Scheme 1a). However, these previously reported works all afford aryl-substituted allylic alcohols. Exploring new chemical reaction selectivity is an interesting and challenging area of organic synthesis.
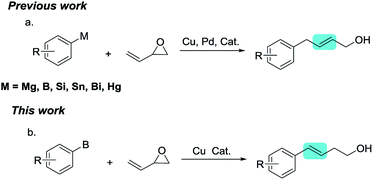 |
| Scheme 1 Cross-couplings reactions of vinyl epoxide with aryl vucleophiles. | |
Based on previous work on Cu-catalyzed ring-opening reactions of epoxides with organoboron,5c we now report the first example of the Cu-catalyzed ring-opening/cross-coupling of vinyl epoxides with arylboronates, which afforded aryl-substituted homoallylic alcohols (Scheme 1b). The selectivity of the ring-opening reaction was different to that observed previously for ring-opening reactions of vinyl epoxide with aryl nucleophiles. This methodology provides access to synthetically valuable aryl-substituted homoallylic alcohols from vinyl epoxides, which are valuable structural fragments in organic synthesis.
Experimental
We began our study by selecting m-methoxy phenylboronate (1a) and 3,4-epoxy-1-butene (2a) as model reaction substrates (Table 1). We first examined previously reported catalytic conditions for similar Cu-catalyzed ring-opening reactions of epoxides with organoboron compounds.5c,12 The product was observed, but in low yield (entry 1). Therefore, the previously reported conditions were not suitable for the ring-opening cross-coupling of vinyl epoxide. To assess the roles of diketone, nitrogen, and phosphine ligands, CuI was next evaluated as a copper source (entries 2–6). Using other diketone ligands afforded a lower yield (entries 2 and 3). Using phosphine (entries 4 and 5) and nitrogen (entry 6) ligands instead of diketone ligands increased the reaction yield, but still only obtained a moderate yield. Next, we explored the effect of copper sources on the reaction efficiency (entries 7 and 8). Under the same conditions, using CuCl instead of CuI significantly increased the reaction yield (entries 7 and 8 vs. entries 5 and 6). Pleasingly, using CuCl as the copper source resulted in good yields (89% GC yield and 85% isolated yield, entry 8). This might be due to strongly nucleophilic iodide ions affecting the reaction. In ether solvents, hardly any product was obtained (entries 9 and 10). Similarly, almost no product was obtained when using LiOMe as base (entry 11). In the absence of a copper source, no product formation was observed (entry 12).
Table 1 Optimization of the reaction conditions
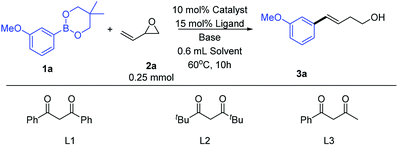
|
Entry |
Catalyst |
Ligand |
Base |
Solvent |
Yielda % |
Reaction conditions: catalyst (10 mol%), base (2.5 equiv.), ligand (15 mol%) in 0.6 mL solvent at 60 °C for 10 h under Ar atmosphere. No CuCl. Saturated NH4Cl was added after the reaction. The yield was determined by GC using benzophenone as internal standard (average of two GC runs). |
1 |
CuI |
L1 |
LiOtBu |
DMF |
21 |
2 |
CuI |
L2 |
LiOtBu |
DMF |
18 |
3 |
CuI |
L3 |
LiOtBu |
DMF |
12 |
4 |
CuI |
PPh3 |
LiOtBu |
DMF |
15 |
5 |
CuI |
Xantphos |
LiOtBu |
DMF |
41 |
6 |
CuI |
TMEDA |
LiOtBu |
DMF |
52 |
7 |
CuCl |
Xantphos |
LiOtBu |
DMF |
63 |
8 |
CuCl |
TMEDA |
LiOtBu |
DMF |
89 |
9 |
CuCl |
TMEDA |
LiOtBu |
THF |
Trace |
10 |
CuCl |
TMEDA |
LiOtBu |
Dioxane |
Trace |
11 |
CuCl |
TMEDA |
LiOMe |
DMF |
Trace |
12b |
— |
TMEDA |
LiOtBu |
DMF |
0 |
With optimized conditions in hand, we next examined the substrate scope (Table 2). To obtain aryl-substituted homoallylic alcohols, it was necessary to add saturated NH4Cl after the reaction was complete. Both electron-rich and electron-poor arylboronates afforded good product yields. A series of relevant functional groups, including ether (3a), halogen (3b and 3c), trifluoromethoxy (3d), cyano (3e), and trifluoromethyl (3f) groups, were well tolerated, affording good yields. Notably, aryl halides, including aryl chloride and aryl bromide (3b and 3c), did not hinder the transformation. Therefore, it was possible to perform subsequent cross-coupling reactions at the halogenated positions. A substrate bearing a bicyclic naphthyl group (3g) also participated in the reaction. Unfortunately, alkenylboronate did not participate in the reaction.
Table 2 Scope of the reactiona
Reaction conditions: vinyl Epoxides (0.25 mmol), arylboronic esters (2 equiv.), LiOtBu (2.5 equiv.). Saturated NH4Cl was added after the reaction was completed. 1–2 mL EtOAc was added after the reaction was completed, then stirred at room temperature for 1–4 h. |
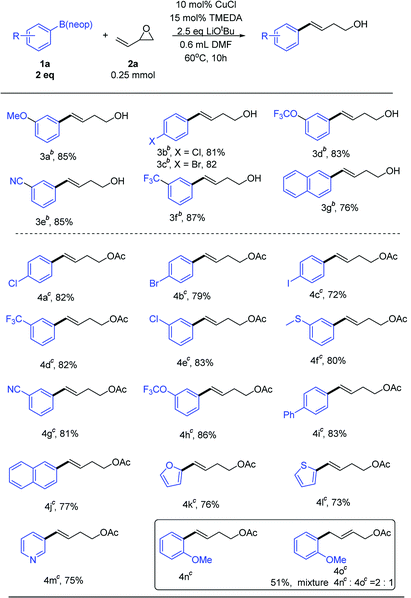 |
Free hydroxyl groups are relatively active functional groups. In many reactions, it is necessary to protect the hydroxyl groups. Hydroxyl groups can be protected with acetyl groups, which requires the use of acetyl chloride or acetic anhydride reagents. Use of these reagents is disadvantageous owing to certain hazards, such as severe irritation and corrosion. After the reaction was completed, then added 1–2 mL EtOAc and stirred at room temperature for 1–4 hour, we obtained acetyl-protected homoallylic (Table 2). Aryl halides, including aryl chloride and aryl bromide (4a and 4b) were also tolerated in this reaction. Notably, the higher activity of aryl iodide (4c) did not affect the transformation. Both electron-rich and electron-poor substrates afforded good yields of acetyl-protected products. A series of relevant functional groups, including trifluoromethyl (4d), halogen (4e), trifluoromethoxy (4h), and cyano (4g) groups, were well tolerated, affording good yields. Arylboronate bearing a sulfur atom (4f) also participated in the reaction. Other aromatic ring substrates, such as biphenyl (4i), naphthyl (4j), furan (4k), thiophene (4l), and pyridine (4m), also participated in the reaction, affording high yields. Steric hindrance at the ortho position resulted in a mixture of protected homoallylic and allylic alcohols (4n and 4o). The homoallylic alcohol compound was detected as the only product except for ortho-substituted substrate.13
To demonstrate the scalability of this method for homoallylic alcohol preparation, we performed the Cu-catalyzed opening-ring reaction of vinyl epoxide on a gram scale, obtaining 3a in 87% yield (Scheme 2). Aryl-substituted homoallylic alcohols are an important organic synthesis fragment, and can be easily converted into β-chlorotetrahydrofurans (3aa). Under chiral catalysis, they can also be converted into chiral β-chlorotetrahydrofurans.14 Furthermore, homoallylic alcohols can also be oxidized by m-CPBA to 3,4-epoxyalcohols (3ab). 3,4-Epoxyalcohols are important organic synthons that can participate in many reactions.15 In addition to these transformations, aryl-substituted homoallylic alcohols can also be converted into β-bromotetrahydrofuran, β-fluorotetrahydrofuran, and β-trifluoromethyl tetrahydrofuran.16 Previously, aryl-substituted homoallylic alcohols were generally synthesized through Wittig or alkene metathesis reactions. The Wittig reaction involves a long reaction route, relatively harsh conditions, and requires the use of strong bases. Meanwhile, the reaction can be controlled at low temperature (see, ESI†).17 For alkene metathesis, Grubbs catalyst is expensive, while commercial styrene is relatively less (see ESI†).18 The Heck reaction of aryl halides with 3-buten-1-ol, generally affords 4-arylbutanal as the major product (see ESI†).19 Therefore, to synthesize aryl-substituted homoallylic alcohols, the metal-catalyzed Sonogashira reaction of aryl halides with but-3-yn-1-ol is necessary, followed by alkyne reduction.20 These alternative methods clearly show that the reaction reported herein provides an efficient, economical, and convenient method for the synthesis of aryl-substituted homoallylic alcohols.
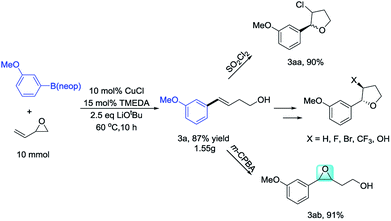 |
| Scheme 2 Synthesis of homoallylic alcohbbols via ring opening reaction. | |
To illustrate the reaction mechanism, we performed a series of reactions using (E)-4-phenylbut-2-en-1-ol (Table 3, eqn (1)). The isomerization product could not be obtained without adding reagents (entry 1). Adding 10 mol% CuCl or 15 mol% TMEDA also did not afford any isomerized product (entries 2 and 3). When 2.5 equiv. of LiOtBu was added, the isomerization product was obtained in high yield and no residual material was detected (entry 4). In contrast, adding 2.5 equiv. of LiCl did not afford any isomerization products (entry 5). This confirmed that lithium ions did not cause isomerization. Similarly, the LiOtBu can also promote the alkene isomerization of allylbenzene (eqn (2)). It is confirmed by these experiments that the LiOtBu is the cause of the alkene migration. Previous studies have indicated that the base, such as K3PO4, KF, KOH, NaOtBu, KOtBu,21 can promote the alkene isomerization of allylbenzene. Researchers have confirmed that the isomerization goes through an intramolecular base-promoted hydrogen transfer process (carbanion intermediate).21a,21e From these experiments, we proposed a possible catalytic cycle (Scheme 3). The copper salt first reacts with LiOtBu, generating LCu-OtBu (I). Transmetalation then produces LCu-Ar(II), which reacts with vinyl epoxide via an SN2′-type ring-opening/coupling to afford intermediate (III). Intermediate III then undergoes intramolecular hydrogen transfer and alkene isomerization to afford the aryl-substituted homoallylic alcohols.
|
 | (1) |
|
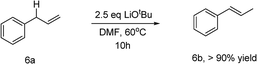 | (2) |
Table 3 Support experiments for the proposed mechanism
Entry |
Conditions |
RSM of 5a |
Yield of 5b |
1 |
— |
>99% |
0 |
2 |
10% CuCl |
>99% |
0 |
3 |
15% TMEDA |
>99% |
0 |
4 |
2.5 equiv. LiOtBu |
trace |
>95% |
5 |
2.5 equvi. LiCl |
100% |
0 |
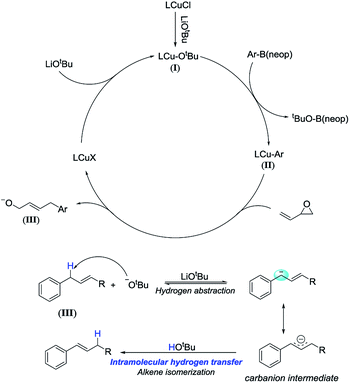 |
| Scheme 3 Proposed catalytic cycle. | |
Conclusions
In summary, we have developed the first Cu-catalyzed ring-opening reaction of vinyl epoxides with arylboronates. This reaction affords (E)-aryl-substituted homoallylic alcohols. The reaction selectivity was different to that of previous ring-opening reactions of vinyl epoxide with aryl nucleophiles. This reaction provides an effective method for the synthesis of aryl-substituted homoallylic alcohols, which are valuable synthetic intermediates in modern organic synthesis. The mild reaction conditions tolerate a wide variety of functional groups.
Conflicts of interest
There are no conflicts to declare.
Acknowledgements
This work was supported by 2017qd11, 2016GH15, and Anhui Province Major Special Projects (16030801108).
Notes and references
- F. Diederich and P. J. Stang, Metal-Catalyzed Cross-Coupling Reactions, Wiley-VCH, Weinheim, 1998 Search PubMed.
-
(a) R. Jana, T. P. Pathak and M. S. Sigman, Chem. Rev., 2011, 111, 1417 CrossRef CAS;
(b) S. Z. Tasker, E. A. Standley and T. F. Jamison, Nature, 2014, 509, 299 CrossRef CAS PubMed;
(c) A. Suzuki, Angew. Chem., Int. Ed., 2011, 50, 6722 CrossRef CAS PubMed;
(d) X. Y. Lu, M. L. Hong, H. P. Zhou, Y. Wang, J. Y. Wang and X. T. Ge, Chem. Commun., 2018, 54, 4417 RSC;
(e) X. Y. Lu, J. H. Liu, X. Lu, Z. Q. Zhang, T. J. Gong, B. Xiao and Y. Fu, Chem. Commun., 2016, 52, 5324 RSC;
(f) X.-Y. Lu, J.-S. Li, M.-L. Hong, J.-Y. Wang and W.-J. Ma, Tetrahedron, 2018, 74, 6979 CrossRef CAS.
-
(a) P. Crotti and M. Pineschi, in Aziridines and Epoxides in Organic Synthesis, Wiley-VCH Verlag GmbH & Co. KGaA, 2006, pp. 271–313 Search PubMed;
(b) V. V. Fokin and P. Wu, in Aziridines and Epoxides in Organic Synthesis, Wiley-VCH Verlag GmbH & Co. KGaA, 2006, pp. 443–477 Search PubMed;
(c) P. A. S. Lowden, in Aziridines and Epoxides in Organic Synthesis, Wiley-VCH Verlag GmbH & Co. KGaA, 2006, pp. 399–442 Search PubMed;
(d) H. Ohno, in Aziridines and Epoxides in Organic Synthesis, Wiley-VCH Verlag GmbH & Co. KGaA, 2006, pp. 37–71 Search PubMed;
(e) B. Olofsson and P. Somfai, in Aziridines and Epoxides in Organic Synthesis, Wiley-VCH Verlag GmbH & Co. KGaA, 2006, pp. 315–347 Search PubMed;
(f) C.-Y. Huang and A. G. Doyle, Chem. Rev., 2014, 114, 8153 CrossRef CAS.
-
(a) M. Alam, C. Wise, C. A. Baxter, E. Cleator and A. Walkinshaw, Org. Process Res. Dev., 2012, 16, 435 CrossRef CAS;
(b) C. Bonini, L. Chiummiento, M. T. Lopardo, M. Pullez, F. Colobert and G. Solladié, Tetrahedron Lett., 2003, 44, 2695 CrossRef CAS;
(c) C. Y. Huang and A. G. Doyle, J. Am. Chem. Soc., 2012, 134, 9541 CrossRef CAS;
(d) K. L. Jensen, E. A. Standley and T. F. Jamison, J. Am. Chem. Soc., 2014, 136, 11145 CrossRef CAS PubMed;
(e) D. K. Nielsen, C. Y. Huang and A. G. Doyle, J. Am. Chem. Soc., 2013, 135, 13605 CrossRef CAS PubMed.
-
(a) M. L. Duda and F. E. Michael, J. Am. Chem. Soc., 2013, 135, 18347 CrossRef CAS PubMed;
(b) Y. Takeda, Y. Ikeda, A. Kuroda, S. Tanaka and S. Minakata, J. Am. Chem. Soc., 2014, 136, 8544 CrossRef CAS PubMed;
(c) X. Y. Lu, C. T. Yang, J. H. Liu, Z. Q. Zhang, X. Lu, X. Lou, B. Xiao and Y. Fu, Chem. Commun., 2015, 51, 2388 RSC.
-
(a) Y. Zhao and D. J. Weix, J. Am. Chem. Soc., 2013, 136, 48 CrossRef PubMed;
(b) Y. Zhao and D. J. Weix, J. Am. Chem. Soc., 2015, 137, 3237 CrossRef CAS;
(c) S. Teng, M. E. Tessensohn, R. D. Webster and J. S. Zhou, ACS Catal., 2018, 8, 7439 CrossRef CAS.
- J. He, J. Ling and P. Chiu, Chem. Rev., 2014, 114, 8037 CrossRef CAS.
- S.-K. Kang, H.-C. Ryu, Y.-T. Hong, M.-S. Kim, S.-W. Lee and J.-H. Jung, Synth. Commun., 2001, 31, 2365 CrossRef CAS.
- Z. Zhao, G. L. Araldi, Y. Xiao, A. P. Reddy, Y. Liao, S. Karra, N. Brugger, D. Fischer and E. Palmer, Bioorg. Med. Chem. Lett., 2007, 17, 6572 CrossRef CAS.
-
(a) J. Kjellgren, J. Aydin, O. A. Wallner, I. V. Saltanova and K. J. Szabó, Chem.–Eur. J., 2005, 11, 5260 CrossRef CAS;
(b) J. P. Patterson, P. Cotanda, E. G. Kelley, A. O. Moughton, A. Lu, T. H. Epps 3rd and R. K. O'Reilly, Polym. Chem., 2013, 4, 2033 RSC;
(c) G. W. Roffe, G. J. Tizzard, S. J. Coles, H. Cox and J. Spencer, Org. Chem. Front., 2016, 3, 957 RSC.
- J. R. Herron, V. Russo, E. J. Valente and Z. T. Ball, Chem.–Eur. J., 2009, 15, 8713 CrossRef CAS PubMed.
-
(a) C. T. Yang, Z. Q. Zhang, Y. C. Liu and L. Liu, Angew. Chem., Int. Ed., 2011, 50, 3904 CrossRef CAS;
(b) G. Z. Wang, J. Jiang, X. S. Bu, J. J. Dai, J. Xu, Y. Fu and H. J. Xu, Org. Lett., 2015, 17, 3682 CrossRef CAS;
(c) Z. Q. Zhang, C. T. Yang, L. J. Liang, B. Xiao, X. Lu, J. H. Liu, Y. Y. Sun, T. B. Marder and Y. Fu, Org. Lett., 2014, 16, 6342 CrossRef CAS PubMed;
(d) Z. Q. Zhang, B. Zhang, X. Lu, J. H. Liu, X. Y. Lu, B. Xiao and Y. Fu, Org. Lett., 2016, 18, 952 CrossRef CAS PubMed;
(e) Y. Y. Sun, J. Yi, X. Lu, Z. Q. Zhang, B. Xiao and Y. Fu, Chem. Commun., 2014, 50, 11060 RSC.
-
(a) L. A. Batory, C. E. McInnis and J. T. Njardarson, J. Am. Chem. Soc., 2006, 128, 16054 CrossRef CAS PubMed;
(b) M. Brichacek, L. A. Batory and J. T. Njardarson, Angew. Chem., Int. Ed., 2010, 49, 1648 CrossRef CAS PubMed.
-
(a) X. Zeng, C. Miao, S. Wang, C. Xia and W. Sun, Chem. Commun., 2013, 49, 2418 RSC;
(b) W. Sun, X. Zeng, C. Miao, S. Wang and C. Xia, Synthesis, 2013, 45, 2391 CrossRef.
-
(a) A. Banerjee and H. Yamamoto, Org. Lett., 2017, 19, 4363 CrossRef CAS;
(b) C. Wang and H. Yamamoto, J. Am. Chem. Soc., 2014, 136, 1222 CrossRef CAS PubMed;
(c) Y. Q. Zhang, N. Funken, P. Winterscheid and A. Gansauer, Angew. Chem., Int. Ed., 2015, 54, 6931 CrossRef CAS PubMed.
-
(a) R. Lin, H. Sun, C. Yang, Y. Yang, X. Zhao and W. Xia, Beilstein J. Org. Chem., 2015, 11, 31 CrossRef PubMed;
(b) Y. Wang, M. Jiang and J.-T. Liu, Adv. Synth. Catal., 2016, 358, 1322 CrossRef CAS.
-
(a) B. J. Stokes, S. M. Opra and M. S. Sigman, J. Am. Chem. Soc., 2012, 134, 11408 CrossRef CAS PubMed;
(b) A. M. Del Hoyo, A. G. Herraiz and M. G. Suero, Angew. Chem., Int. Ed., 2017, 56, 1610 CrossRef CAS PubMed.
- P. R. Walker, C. D. Campbell, A. Suleman, G. Carr and E. A. Anderson, Angew. Chem., Int. Ed., 2013, 52, 9139 CrossRef CAS PubMed.
- M. van Gemmeren, M. Borjesson, A. Tortajada, S. Z. Sun, K. Okura and R. Martin, Angew. Chem., Int. Ed., 2017, 56, 6558 CrossRef CAS PubMed.
-
(a) X. Su, Y. Sun, J. Yao, H. Chen and C. Chen, Chem. Commun., 2016, 52, 4537 RSC;
(b) J. Tummatorn, S. Ruchirawat and P. Ploypradith, Chem.–Eur. J., 2010, 16, 1445 CrossRef CAS.
-
(a) D. J. Cram and R. T. Uyeda, J. Am. Chem. Soc., 1962, 84, 4358 CrossRef CAS;
(b) R. Neumann and Y. Sasson, J. Mol. Catal., 1985, 33, 201 CrossRef CAS;
(c) T. X. Luu, T. Lam, T. Le and F. Duus, Molecules, 2009, 14, 3411 CrossRef PubMed;
(d) I. Al-Maskery, K. Girling, S. D. Jackson, L. Pugh and R. R. Spence, Top. Catal., 2010, 53, 1163 CrossRef CAS;
(e) N. Qafisheh, S. Mukhopadhyay, A. V. Joshi, Y. Sasson, G.-K. Chuah and S. Jaenicke, Ind. Eng. Chem. Res., 2007, 46, 3016 CrossRef CAS.
Footnote |
† Electronic supplementary information (ESI) available. See DOI: 10.1039/c8ra09048c |
|
This journal is © The Royal Society of Chemistry 2018 |
Click here to see how this site uses Cookies. View our privacy policy here.