DOI:
10.1039/C8RA08433E
(Paper)
RSC Adv., 2018,
8, 37184-37192
Novozym 40086 as a novel biocatalyst to improve benzyl cinnamate synthesis
Received
11th October 2018
, Accepted 31st October 2018
First published on 5th November 2018
Abstract
Benzyl cinnamate is one of the derivatives of cinnamic acid, which can be used as the main constituent in perfume, UV filters and medicines. In this work, several commercial immobilized lipases (Novozym 40086, Novozym 435 and Lipozyme TLIM) and free lipases (lipase A and B from Candida sp., and lipozyme from Thermomyces linuginosous) were used as catalysts for benzyl cinnamate preparation by the esterification of benzyl alcohol with cinnamic acid. The effect of various esterification parameters (reaction time, reaction temperature, lipase concentration and substrate ratio) on benzyl cinnamate yield were also optimized and evaluated using response surface methodology (RSM). Among all tested lipases, Novozym 40086, as a new commercial immobilized lipase from Rhizomucor miehei immobilized on acrylic resin beads, showed the best activity for the esterification. Esterification parameters were optimized as follows: reaction temperature 46.3 °C, substrate molar ratio 1
:
3 (cinnamic acid/benzyl alcohol), Novozym 40086 concentration 23.1 mg mL−1, reaction time 11.3 h, and maximum benzyl cinnamate yield (96.2 ± 1.4%) were achieved under the optimal conditions. Novozym 40086 can be reused 9 times without significant decrease in benzyl cinnamate yield (90.1% yield after nine times). The activation energy for the Novozym 40086-catalyzed esterification was 14.96 ± 0.25 kJ mol−1. These results showed that Novozym 40086 was a novel and efficient biocatalyst for the esterification, which can be used as a good alternative for benzyl cinnamate production.
1. Introduction
Cinnamic acid (3-phenylacrylic acid) is one kind of phenolic acid widely present in plants, which can be used in flavoring materials and skin care products.1–3 However, the high melting point (133 °C) and poor solubility of cinnamic acid limit its application. Benzyl cinnamate (melting point 34.5 °C), one of the derivatives of cinnamic acid, was often used as a flavoring agent and antioxidant in the food, cosmetic and medicine industries.4–11 Benzyl cinnamate has been approved as a flavor by the FDA and included by the Council of Europe in the list of substances graded A.4 Therefore, the preparation of benzyl cinnamate has been very interesting.10,12
Recently, benzyl cinnamate has been prepared by the esterification of benzyl alcohol with cinnamic acid using enzymes or chemicals as catalysts. Compared with chemical catalysts, due to the thermal instability of cinnamic acid, biocatalysts have attracted more attention for the esterification.13–16 For example, in the reports of Wang et al. and Zhang et al.,10,12 lipozyme TLIM was used as a biocatalyst to prepare benzyl cinnamate, and a high yield (97%) can be obtained. For the immobilized biocatalysts, the carrier features can affect the activity and stability of immobilized enzymes.17–21 Because the carrier of Lipozyme TLIM was silica gel, which can be easily destroyed during the mechanical mixing process and in the presence of by-product water formed in the esterification, the activity of Lipozyme TLIM decreased to 63% after reuse 3 times. Therefore, more efficient biocatalysts, especially for the immobilized enzymes, have been more popular alternative for benzyl cinnamate preparation.
In this study, for enhancing benzyl cinnamate synthesis, several commercial immobilized lipases (Novozym 40086, Novozym 435, Lipozyme TLIM) and free lipases (lipase A and B from Candida sp., and lipozyme from Thermomyces linuginosous) were used to catalyze the esterification. Effects of esterification parameters (reaction time, temperature, lipase concentration and substrate ratio) on the reaction were evaluated and optimized by RSM, and the lipase reusability was also investigated. HPLC-UV and HPLC-ESI-MS were used to analyze benzyl cinnamate synthesis. Thermodynamic and reaction kinetic were investigated.
2. Materials and methods
2.1. Materials
Cinnamic acid was provided from Nanjing Zelang Chemical Co., Ltd. (Nanjing China). Benzyl alcohol was purchased from Maikelin Biochem. Technol. Co., Ltd. (Shanghai China). Three commercial immobilized lipases (Novozym 40086, Novozym 435 and Lipozyme TLIM) and three free lipases (lipase A and B from Candida sp., and lipozyme from Thermomyces linuginosous) were purchased from Novozymes A/S (Bagsvaerd, Denmark).
2.2. Enzymatic esterification and immobilized enzyme reusability
The esterification of cinnamic acid (0.1 mmol) with benzyl alcohol (0.3 mmol) was carried out in 10 mL isooctane at 150 rpm and 40 °C. The esterification was initialized when lipase was added. Samples (20 μL) were taken out for analysis at specific time intervals.
To investigate the reusability of lipase, after the esterification finished, Novozym 40086 was firstly filtered, and then washed using isooctane for 6 times. Finally, the lipase was dried for the next use.
2.3. Analysis
Products were analyzed using HPLC with C18 column (250 mm × 4.6 mm, 5 μm), which was eluted with solvent 10% A (0.5%, v/v, glacial acetic acid/water) and solvent 90% B (methanol) at 0.5 mL min−1 at 35 °C and 280 nm.
In order to identify the product, the major ion ([M + Na]+) of benzyl cinnamate detected by HPLC-ESI-MS was 261 (required M 238). The capillary, collision and cone voltages were 3.0 kV, 5 V and 30 V, respectively. The ion source and desolvation temperatures were 80 °C and 150 °C, respectively. The flows of cone desolvation gas, desolvation gas and injection were 40 L h−1, 350 L h−1 and 20 μL min−1, respectively.
2.4. Experimental design for response surface methodology
A Box–Behnken design with 3 levels and 3 factors was used to evaluate the interaction of factors on the esterification (Table 1). Three factors and 3 levels were set as esterification temperature (20 °C, 40 °C and 60 °C), esterification time (3 h, 9 h and 15 h), and lipase concentration (5 mg mL−1, 20 mg mL−1 and 35 mg mL−1), respectively.
Table 1 RSM design and results of Novozym 40086-catalyzed the esterification for benzyl cinnamate preparation
Treatment no.a |
Reaction time A (h) |
Novozym 40086 concentration B (mg mL−1) |
Temperature C (°C) |
Benzyl cinnamate yield (%) |
Experiment numbers randomly. |
1 |
9 (0) |
20 (0) |
40 (0) |
89.2 ± 1.20 |
2 |
9 (0) |
20 (0) |
40 (0) |
91.0 ± 0.82 |
3 |
3 (−1) |
20 (0) |
20 (−1) |
24.1 ± 1.61 |
4 |
3 (−1) |
35 (1) |
40 (0) |
64.3 ± 0.60 |
5 |
15 (1) |
35 (1) |
40(0) |
91.4 ± 0.42 |
6 |
15 (1) |
20 (0) |
20(-1) |
88.6 ± 1.12 |
7 |
3 (−1) |
5 (−1) |
40 (0) |
67.9 ± 0.63 |
8 |
9 (0) |
20 (0) |
40 (0) |
92.0 ± 1.56 |
9 |
9 (0) |
35 (1) |
60 (1) |
92.3 ± 0.61 |
10 |
15 (1) |
5 (−1) |
40 (0) |
74.0 ± 0.31 |
11 |
3 (−1) |
20 (0) |
60 (1) |
94.2 ± 1.46 |
12 |
9 (0) |
5 (−1) |
20 (−1) |
28.0 ± 1.62 |
13 |
9 (0) |
5 (−1) |
60 (1) |
93.0 ± 1.19 |
14 |
15 (1) |
20 (0) |
60 (1) |
90.8 ± 2.19 |
15 |
9 (0) |
20 (0) |
40 (0) |
88.8 ± 0.52 |
16 |
9 (0) |
35 (1) |
20 (−1) |
75.2 ± 1.78 |
17 |
9 (0) |
20 (0) |
40 (0) |
85.8 ± 1.19 |
2.5. Statistical analysis
From the RSM design, the mathematical relationship between esterification parameters with benzyl cinnamate yield was as follow:
where Y is benzyl cinnamate yield, Xi and Xj represent reaction parameters, and β0, βi, βii, and βij are the constants.
3. Results and discussion
3.1. Biocatalyst screening
Fig. 1 shows that, when the three free lipases were used as biocatalysts for the esterification, the low benzyl cinnamate yields were obtained, which were attributed to the aggregation of free liquid lipases in isooctane system. Fig. 1 also shows that, at 24 h, the maximum benzyl cinnamate yield (92.3 ± 2.3%) was obtained using Novozym 40086 as biocatalyst, which was 1.7 times that (55.1 ± 1.6%) of Lipozyme TLIM and 1.8 times that (51.3 ± 2.3%) of free lipozyme from Thermomyces linuginosous. In the previous reports,10,12 immobilized lipase Lipozyme TLIM was used to prepare benzyl cinnamate. However, the immobilized carrier of Lipozyme TLIM was silica gel, which can be easily destroyed during the mixing process by mechanical agitation and the presence of water formed in the esterification.22,23 These resulted in the decrease of benzyl cinnamate yield to 63% at three times reuse of Lipozyme TLIM.10,12 Novozym 40086 was a new commercial lipase from Rhizomucor miehei immobilized on acrylic resin beads, which was very stable and easily separated from the esterification, and the maximum benzyl cinnamate yield (92.3 ± 2.3%) was achieved using Novozym 40086 as biocatalyst. Therefore, compared with other commercial immobilized lipases and free lipases used in the work, Novozym 40086 was the best alternative for the esterification, which was ascribed to the interfacial activation of the immobilized hydrophobic carrier (acrylic resin beads) and stabilization of open form of lipase by the immobilization. Similar effect of hydrophobic supports on the immobilized enzyme activity can also be found in other lipases.19,20,24,25
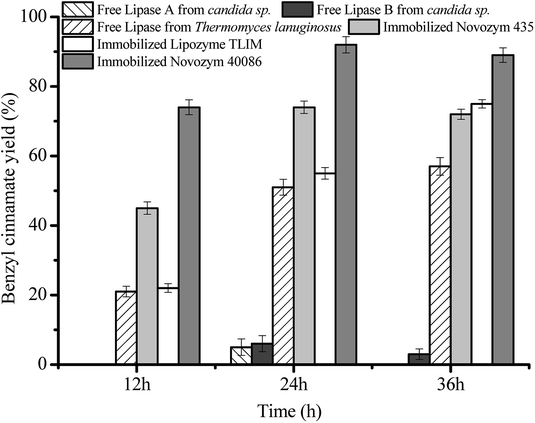 |
| Fig. 1 Effect of different lipases on benzyl cinnamate yield. Reaction conditions: substrate ratio 1 : 3 (cinnamic acid to benzyl alcohol, mol mol−1), lipase concentration 30 mg mL−1 at 150 rpm and 40 °C in isooctane. | |
3.2. Selection of solvent
Fig. 2 shows that, among the tested five solvents, high benzyl cinnamate yields can be obtained using isooctane (log
P, 4.5), heptane (log
P, 4.0) and hexane (log
P, 3.5) as solvents. These were attributed to the presence of non-polar solvents with high log
P (>3.0). Similar the enhancement of immobilized enzyme activity in non-polar solvent can also be found in other reactions.26–29 However, when heptane, hexane and toluene were used as solvents, the maximum benzyl cinnamate yields were obtained at 12 h, and with further increase of reaction time to 24 h, benzyl cinnamate yield decreased, which may be attributed to the dilution of cinnamic acid with reaction progress and more water into the reaction system from the atmosphere. Similar effect can also be found in other enzymatic esterification.22,23
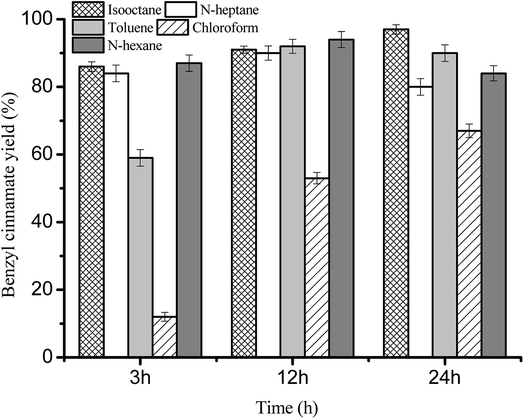 |
| Fig. 2 Effect of solvents on benzyl cinnamate yield. Reaction conditions: substrate ratio 1 : 3, Novozym 40086 concentration 30 mg mL−1 at 150 rpm and 40 °C. | |
From Fig. 2, we can found that the maximum benzyl cinnamate yield (97.1 ± 1.38%) was successfully achieved using isooctane as solvent, which was attributed the high boiling point (99 °C) and log
P (4.5) of isooctane. However, for the polar chloroform (log
P, 2.0), the lowest benzyl cinnamate yield (less than 70%) was obtained, which was due to the fact that the essential water on the surface of immobilized enzyme was deprived by the polar chloroform. Similar immobilized enzyme activity decreased in polar solvents can also be found in many reactions.30–32
3.3. Effect of substrate ratio
Varying substrate ratio from 1
:
1 (cinnamic acid to benzyl alcohol, mol mol−1) to 1
:
3, benzyl cinnamate yield increased from 83.2 ± 2.19% to 93.5 ± 1.29% at 24 h (Fig. 3). However, with the substrate molar ratio ranging from 1
:
4 to 1
:
6, the yield of benzyl cinnamate decreased from 87.4 ± 2.06% to 86.3 ± 2.0% at 24 h, which were attributed to the decrease of the concentration of cinnamic acid and more dead end enzyme-benzyl alcohol complex formation with the increase of benzyl alcohol concentration. These indicated that, at high benzyl alcohol concentration, the inhibition of benzyl alcohol on the immobilized lipase-catalyzed esterification was significant. Similar inhibition of alcohol on lipase activity can also be found in another reaction.33–36
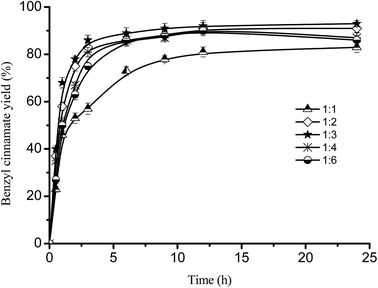 |
| Fig. 3 Effect of substrate ratio on benzyl cinnamate yield. Reaction conditions: Novozym 40086 concentration 30 mg mL−1 at 150 rpm and 40 °C in isooctane. | |
3.4. Effect of Novozym 40086 concentration
According to the previous methods,34,37,38 the initial reaction rates, defined as the initial benzyl cinnamate yield (≤15.0% benzyl cinnamate yield) per unit time (V0, mol (L−1 min−1)), were calculated from six experimental points of the yield–time profile, where the profiles were found to be approximately linear. Fig. 4 shows that, with the increase of Novozym 40086 concentration, the time to achieve equilibrium was shortened from >24 h (5 mg mL−1) to 9 h (30 mg mL−1), and the initial formation rate of benzyl cinnamate linearly increased from 1.8 × 10−3 mol (L−1 min−1) to 8.8 × 10−3 mol (L−1 min−1). These results indicated that, at lower Novozym 40086 concentration (<30 mg mL−1), the effect of external mass transfer can be neglected, and similar effect can also be confirmed by the effect of rotation rate (Fig. 5). However, at higher Novozym 40086 concentration, the presence of more solid immobilized lipase resulted in the diffusional limitation and the water in the reaction system wasn't enough for the immobilized lipase activity, which can slightly decrease benzyl cinnamate yield. Similar results of immobilized biocatalysts on the mass transfer can also be found in other studies.10,34,36,37,39,40
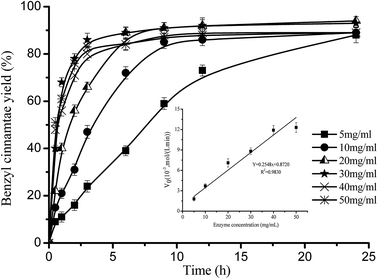 |
| Fig. 4 Effect of Novozym 40086 concentration on benzyl cinnamate yield. Reaction conditions: substrate ratio 1 : 3 at 150 rpm and 40 °C in isooctane. | |
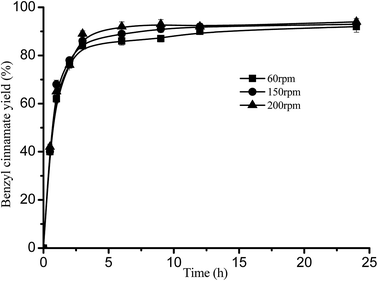 |
| Fig. 5 Effect of rotation rate on benzyl cinnamate yield. Reaction conditions: substrate ratio 1 : 3 and Novozym 40086 concentration 30 mg mL−1 at 40 °C in isooctane. | |
Fig. 5 shows that there was not significant influence of rotation rate on the reaction, which indicated that the esterification wasn't controlled by external mass transfer. These were agreed with the results of lipase concentration. Similar effect of the excess immobilized biocatalysts on the mass transfer can also be found in other reactions.17,34,36,37,40,41
3.5. Effect of esterification temperature
Due to the low boiling point (99 °C) of isooctane as reaction solvent and the volatility of benzyl alcohol (flash point, 100 °C), the highest reaction temperature was set as 70 °C.
Fig. 6 shows that, as the temperature increases from 20 °C to 60 °C, the formation rate of benzyl cinnamate rapidly increased, for example, initial esterification rate increased from 5.8 × 10−3 mol (L−1 min−1) of 20 °C to 1.54 × 10−2 mol (L−1 min−1) of 70 °C, and time to achieve equilibrium was shortened from >24 h (at 20 °C) to 3 h (at 70 °C). These indicated that the high thermodynamic stability of the immobilized Novozym 40086. Fig. 6 also shows that, as the temperature increases, the maximum benzyl cinnamate yield increased from 77.5 ± 2.3% of 20 °C to 92.6 ± 2.06% of 70 °C. These results was attributed to that, the esterification is an endothermic reaction, and the increase of temperature can increase the active molecules of the substrates and decrease the viscosity of reaction system, which is beneficial to more energetic collisions of the substrates to form benzyl cinnamate. Similar effect of temperature can also be found in other enzyme-catalyzed reactions.42–46
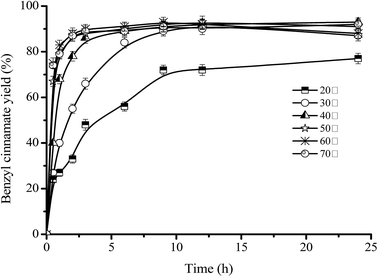 |
| Fig. 6 Effect of esterification temperature on benzyl cinnamate yield. Reaction conditions: substrate ratio 1 : 3, Novozym 40086 concentration 30 mg mL−1 at 150 rpm in isooctane. | |
3.6. Reaction thermodynamics and kinetics
Fig. 7 shows that initial reaction rate linearly increased with temperature ranging from 20 °C to 70 °C. Ea of Novozym 40086-catalyzed the reaction was 14.96 ± 0.25 kJ mol−1, which was higher than that (12.8 ± 0.1 kJ mol−1) of the benzyl propionate preparation using immobilized lipase as catalyst and lower than that (16.6 ± 0.7 kJ mol−1) of free lipase.34 The highest Ea of free lipase was due to the agglomerate in hydrophobic solvent and great mass transfer of reaction system. The higher Ea of benzyl cinnamate preparation using immobilized Novozym 40086 was attributed to the greater stearic hindrance of cinnamic acid than that of vinyl propionate. Arrhenius equation of benzyl cinnamate formation was ln
V0 = 1.0787 − 1799/T.
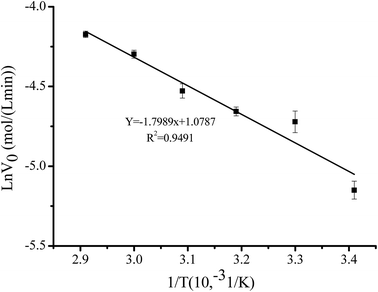 |
| Fig. 7 Relationship between initial esterification rate with temperature. Reaction conditions: substrate ratio 1 : 3, Novozym 40086 concentration 30 mg mL−1 at 150 rpm in isooctane. | |
According to the previous methods,47,48 non-linear fitting curves at different concentrations of cinnamic acid were achieved (Fig. 8), and initial reaction rate was described as follows:
where
V and
Vmax are initial and maximum esterification rates, respectively; [A], [B],
KmA and
KmB are the concentrations and apparent Michaelis constants of cinnamic acid and benzyl alcohol, respectively.
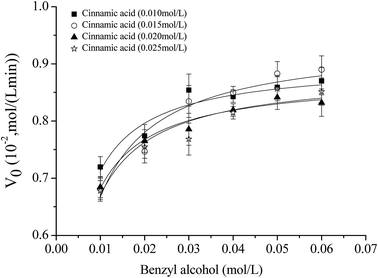 |
| Fig. 8 Non-linear fitting curves between initial esterification rate with the concentration of benzyl alcohol at different cinnamic acid concentrations (0.01 mol L−1, 0.015 mol L−1, 0.02 mol L−1, 0.025 mol L−1). Reaction conditions: benzyl alcohol concentrations ranging from 0.01 mol L−1 to 0.06 mol L−1 at 150 rpm and 40 °C in isooctane. | |
From Fig. 8, Vmax, KmA and KmB can be calculated as 9.0 × 10−3 mol (L−1 min−1), 3.2 × 10−3 mol L−1 and 1.2 × 10−3 mol L−1, respectively.
3.7. Model fitting
RSM is an empirical modeling technique used to evaluate the relationship between a set of controllable experimental factors and observed results. Modeling of esterification variables and benzyl cinnamate yield was performed by RSM to predict the highest possible yield of benzyl cinnamate. The results obtained for the models are listed in Tables 1 and 2. For benzyl cinnamate formation, the coefficient of the RSM model was 0.9649 (Table 2), which indicated that the model for benzyl cinnamate formation can successfully predict the relationship between benzyl cinnamate yield with reaction variables. Therefore, the quadratic polynomial model can be obtained from RSM as follows:
Benzyl cinnamate yield (%) = 89.18 + 11.97X1 + 7.54X2 + 19.30X3 + 5.25X1X2 − 16.98X1X3 − 11.98X2X3 − 6.24X12 − 8.54X22 − 8.51X32 |
Table 2 ANOVA analysis for response surface models of benzyl cinnamate yield
Sources |
Sum of squares |
Freedom |
Mean square |
F Value |
Prob > F |
Model |
7266.35 |
9 |
807.37 |
21.36 |
0.0003 |
Residual |
264.57 |
7 |
37.80 |
|
|
Lack of fit |
241.93 |
3 |
80.64 |
14.25 |
0.0133 |
Pure error |
22.64 |
4 |
5.66 |
|
|
Cor. total |
7530.93 |
16 |
|
|
|
R2 = 0.9649 |
|
|
|
|
|
The mutual interactions of three reaction factors on benzyl cinnamate yield were shown in Fig. 9. Benzyl cinnamate yield increased with the increases of reaction variables, and the maximal benzyl cinnamate yield appeared at 40–50 °C, 9–12 h, and >20 mg mL−1 Novozym 40086 concentration. For benzyl cinnamate formation, the effect of esterification parameters was temperature > reaction time > Novozym 40086 concentration (Fig. 9).
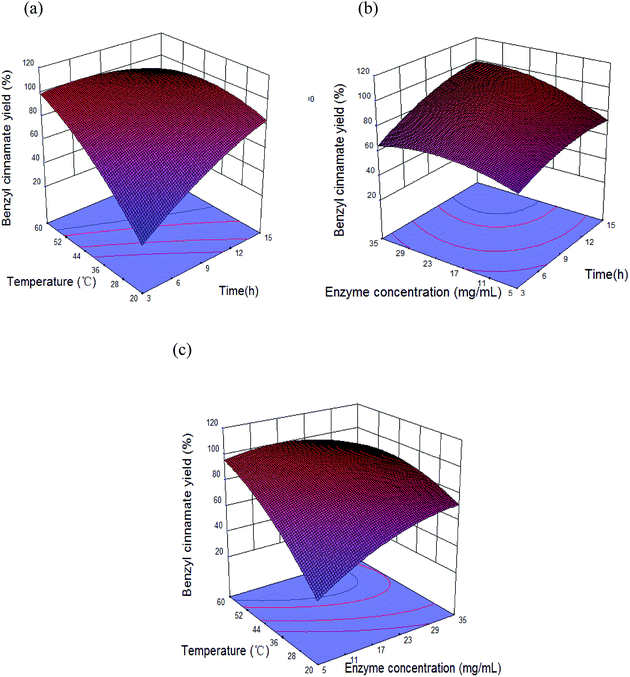 |
| Fig. 9 3D graphs between two parameters for benzyl cinnamate formation: (a) reaction time and temperature with 20 mg mL−1 Novozym 40086 concentration; (b) Novozym 40086 concentration and time at 40 °C; (c) Novozym 40086 concentration and temperature at 9 h. | |
3.8. Optimum esterification parameters and model verification
In order to test the reliability of the optimization results, the verification experiments were carried out. Result shows that there was no significant difference between the experimental yield (96.2 ± 1.4%) with the theoretical predicted yield (96.9%), which confirms the validity of the prediction model. Under the optimal conditions: temperature 46.3 °C, Novozym 40086 concentration 23.1 mg mL−1, molar ratio 1
:
3, reaction time 11.3 h, the yield of benzyl cinnamate reached 96.2 ± 1.4%, which was similar with the benzyl cinnamate yields (97%) in the previous reports.10,12 However, the time to achieve the maximum yield in our work (11.3 h) was less than half of the previous reports (≥24 h).10,12 And the Novozym 40086 concentration (23.1 mg mL−1) used in the work was lower than the previous reports (31 mg mL−1).10,12
3.9. Reusability of Novozym 40086 in isooctane
Fig. 10 shows that, under the optimized esterification conditions by RSM, Novozym 40086 can be reused 9 times without obvious benzyl cinnamate yield decrease, and benzyl cinnamate yield (90.1 ± 1.2%) was still higher than 90% at ninth time. The reuse time (9 times) of Novozym 40086 and benzyl cinnamate yield (90% at ninth time) in our work were great higher than that of Lipozyme TLIM (61.3% benzyl cinnamate yield at third time).10 The good reusability of Novozym 40086 was ascribed to the interfacial activation of the immobilized hydrophobic support (acrylic resin beads). Similar influence of hydrophobic support on the lipase activity can also be found in the immobilization of other lipases.19,20,24
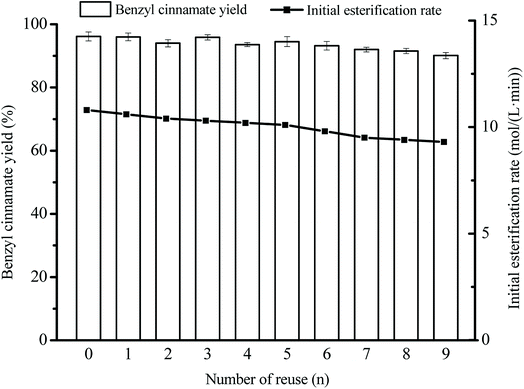 |
| Fig. 10 Reusability of Novozym 40086 in the esterification. Reaction conditions: substrate ratio 1 : 3, Novozym 40086 concentration 23.1 mg mL−1 at 150 rpm and 46.3 °C in isooctane. | |
4. Conclusions
In this paper, Novozym 40086 was successfully used as a novel biocatalyst to catalyze the esterification for benzyl cinnamate preparation. High yield of benzyl cinnamate (96.2 ± 1.4%) was achieved under the optimized conditions: temperature 46.3 °C, Novozym 40086 concentration 23.1 mg mL−1, molar ratio 1
:
3, reaction time 11.3 h. Under these conditions, Novozym 40086 can be used 9 times without obvious benzyl cinnamate decrease. Ea of benzyl cinnamate formation was 14.96 ± 0.25 kJ mol−1. These indicated Novozym 40086 is a good alternative for benzyl cinnamate preparation.
Conflicts of interest
There are no conflicts to declare.
Acknowledgements
We profoundly appreciate financial support from National Natural Science Foundation of China (31771937).
References
- A. Guniakrzyżak, K. Słoczyńska, J. Popiół, P. Koczurkiewicz, H. Marona and E. Pekala, Int. J. Cosmet. Sci., 2018, 40, 356–366 CrossRef PubMed.
- S. Adisakwattana, Nutrients, 2017, 9, 163 CrossRef PubMed.
- G. R. Silveira, K. A. Campelo, G. Lima, L. P. Carvalho, S. S. Samarao, O. Vieira-da-Motta, L. Mathias, C. R. R. Matos, I. J. C. Vieira, E. J. T. de Melo and E. J. Maria, Molecules, 2018, 23, 774 CrossRef PubMed.
- S. P. Bhatia, G. A. Wellington, J. Cocchiara, J. Lalko, C. S. Letizia and A. M. Api, Food Chem. Toxicol., 2007, 45, S40–S48 CrossRef PubMed.
- A. Aliboni, A. D'Andrea and P. Massanisso, J. Agric. Food Chem., 2011, 59, 282–288 CrossRef CAS PubMed.
- M. S. Shintre, R. S. Ghadge and S. B. Sawant, Biochem. Eng. J., 2002, 12, 131–141 CrossRef CAS.
- G. D. Yadav and S. Devendran, Process Biochem., 2012, 47, 496–502 CrossRef CAS.
- A. G. A. SÁ, A. C. de Meneses, P. H. H. de Araújo and D. de Oliveira, Trends Food Sci. Technol., 2017, 69, 95–105 CrossRef.
- S. M. Jakovetic, B. Z. Jugovic, M. M. Gvozdenovic, D. I. Bezbradica, M. G. Antov, D. Z. Mijin and Z. D. Knezevic-Jugovic, Appl. Biochem. Biotechnol., 2013, 170, 1560–1573 CrossRef CAS PubMed.
- Y. Wang, D. H. Zhang, N. Chen and G. Y. Zhi, Bioresour. Technol., 2015, 198, 256–261 CrossRef CAS PubMed.
- M. Dellagreca, L. Previtera, R. Purcaro and A. Zarrelli, J. Nat. Prod., 2007, 70, 1664–1667 CrossRef CAS PubMed.
- D. H. Zhang, J. Y. Zhang, W. C. Che and Y. Wang, Food Chem., 2016, 206, 44–49 CrossRef CAS PubMed.
- K. Huang, Y. Li, C. Kuo, Y. Twu and C. Shieh, Molecules, 2016, 21, 478 CrossRef PubMed.
- M. Adnan, K. Li, L. Xu and Y. Yan, Catalysts, 2018, 8, 96 CrossRef.
- N. Nobakht, M. A. Faramarzi, A. Shafiee, M. Khoobi and E. Rafiee, Int. J. Biol. Macromol., 2018, 113, 8–19 CrossRef CAS PubMed.
- S. Huang, P. Wu, J. Chen, C. Kuo and C. Shieh, Catalysts, 2018, 8, 338 CrossRef.
- W. Tischer and V. Kasche, Trends Biotechnol., 1999, 17, 326–335 CrossRef CAS PubMed.
- R. C. Rodrigues, C. Ortiz, Á. Berenguer-Murcia, R. Torres and R. Fernández-Lafuente, Chem. Soc. Rev., 2013, 42, 6290–6307 RSC.
- J. C. S. dos Santos, O. Barbosa, C. Ortiz, A. Berenguer-Murcia, R. C. Rodrigues and R. Fernandez-Lafuente, ChemCatChem, 2015, 7, 2413–2432 CrossRef CAS.
- E. A. Manoel, M. Pinto, J. C. S. dos Santos, V. G. Tacias-Pascacio, D. M. G. Freire, J. C. Pinto and R. Fernandez-Lafuente, RSC Adv., 2016, 6, 62814 RSC.
- E. P. Cipolatti, E. A. Manoel, R. Fernandez-Lafuente and D. M. G. Freire, Biotechnology Research and Innovation, 2017, 1, 26–34 CrossRef.
- N. Paludo, J. S. Alves, C. Altmann, M. A. Z. Ayub, R. Fernandez-Lafuente and R. C. Rodrigues, Ultrason. Sonochem., 2015, 22, 89–94 CrossRef CAS PubMed.
- L. P. Fallavena, F. H. F. Antunes, J. S. Alves, N. Paludo, M. A. Z. Ayub, R. Fernandez-Lafuente and R. C. Rodrigues, RSC Adv., 2014, 4, 8675 RSC.
- E. A. Manoel, J. M. Robert, M. C. C. Pinto, A. C. O. Machado, M. D. Besteti, M. A. Z. Coelho, A. B. C. Simas, R. Fernandez-Lafuente, J. C. Pinto and D. M. G. Freire, RSC Adv., 2016, 6, 4043 RSC.
- E. A. Manoel, J. C. S. dos Santos, D. M. G. Freire, N. Rueda and R. Fernandez-Lafuente, Enzyme Microb. Technol., 2015, 71, 53–57 CrossRef CAS PubMed.
- M. Pöhnlein, R. Hausmann, S. Lang and C. Syldatk, Eur. J. Lipid Sci. Technol., 2015, 117, 145–155 CrossRef.
- Z. Yang, Z. Guo and X. Xu, Food Chem., 2012, 132, 1311–1315 CrossRef CAS PubMed.
- M. D. Romero, L. Calvo, C. Alba and A. Daneshfar, J. Biotechnol., 2007, 127, 269–277 CrossRef CAS PubMed.
- Y. Zheng, X. M. Wu, C. Branford-White, X. Ning, J. Quan and L. M. Zhu, J. Mol. Catal. B: Enzym., 2009, 58, 65–71 CrossRef CAS.
- Z. T. Alismaeel, A. S. Abbas, T. M. Albayati and A. M. Doyle, Fuel, 2018, 234, 170–176 CrossRef CAS.
- R. C. Rodrigues and M. A. Z. Ayub, Process Biochem., 2011, 46, 682–688 CrossRef CAS.
- M. A. Amani, M. S. Davoudi, K. Tahvildari, S. M. Nabavi and M. S. Davoudi, Ind. Crops Prod., 2013, 43, 40–43 CrossRef CAS.
- K. S. Hari and N. G. Karanth, Biochim. Biophys. Acta, Biomembr., 2001, 1547, 262–267 CrossRef.
- K. C. Badgujar and B. M. Bhanage, Ind. Eng. Chem. Res., 2014, 53, 18806–18815 CrossRef CAS.
- C. Kuo, G. Chen, C. Chen, Y. Liu and C. Shiehd, Process Biochem., 2014, 49, 437–444 CrossRef CAS.
- S. D. Shinde and G. D. Yadav, Ind. Eng. Chem. Res., 2014, 53, 8706–8713 CrossRef CAS.
- S. Sun, L. Shan, Y. Liu, Q. Jin, Y. Song and X. Wang, J. Mol. Catal. B: Enzym., 2009, 57, 104–108 CrossRef CAS.
- D. L. Purich, Contemporary enzyme kinetics and mechanism, Elsevier Science Publishing Co. Inc, San Diego, 3rd edn, 2010 Search PubMed.
- S. Sun and B. Hu, Food Chem., 2017, 214, 192–198 CrossRef CAS PubMed.
- S. Sun, S. Zhu and Y. Bi, Food Chem., 2014, 158, 292–295 CrossRef CAS PubMed.
- Z. Guo and Y. Sun, Food Chem., 2007, 100, 1076–1084 CrossRef CAS.
- R. Yuryev, V. Kasche, Z. Ignatova and B. Galunsky, Protein J., 2010, 29, 181–187 CrossRef CAS PubMed.
- W. N. Li, B. Q. Chen and T. W. Tan, Appl. Biochem. Biotechnol., 2011, 163, 102–111 CrossRef CAS PubMed.
- W. J. Sun, H. X. Zhao, F. J. Cui, Y. H. Li, S. L. Yu, Q. Zhou, J. Y. Qian and Y. Dong, Chem. Cent. J., 2013, 7, 1–13 CrossRef PubMed.
- G. D. Yadav and S. B. Dhoot, J. Mol. Catal. B: Enzym., 2009, 57, 34–39 CrossRef CAS.
- Y. Wang, D. H. Zhang, J. Y. Zhang, N. Chen and G. Y. Zhi, Food Chem., 2016, 190, 629–633 CrossRef CAS PubMed.
- A. Cornish-Bowden and J. T. Wong, Biochem. J., 1978, 175, 969–976 CrossRef CAS PubMed.
- A. Cornish-Bowden, Fundamentals of Enzyme Kinetics, Butterworth & Co. (Publishers) Ltd., Southampton, 1979 Search PubMed.
|
This journal is © The Royal Society of Chemistry 2018 |
Click here to see how this site uses Cookies. View our privacy policy here.