DOI:
10.1039/C8RA07692H
(Paper)
RSC Adv., 2018,
8, 34937-34946
Mesophase behavior of new linear supramolecular hydrogen-bonding complexes†
Received
15th September 2018
, Accepted 4th October 2018
First published on 11th October 2018
Abstract
Thermal and mesophase behavior of four new series of hydrogen-bonded supramolecular complexes (In/IIm) were investigated by differential scanning calorimetry and phases identified by polarized light microscopy. All hydrogen-bonded complexes formed from 4-alkoxyphenylazobenzoic acid (In) and 4-(4′-pyridylazophenyl)-4′′-alkoxybenzoates (IIm). The results revealed that the prepared complexes are dimorphic, possessing smectic C and nematic phases. The comparison, made between the present series and previously investigated simpler, In/IIIm and angular, In/IVm analogues, revealed that increasing the length of the mesogenic core and/or linearity of complex increase the stabilities of both the smectic C and nematic phases.
1. Introduction
Mesomorphic properties of liquid crystal materials are largely affected by any change in their molecular structure. Several methods were reported to obtain highly stable nano structured systems from anisotropic particles and liquid crystals such as stable colloidal dispersions of halloysite clay nanotubes.1–3 Hydrogen bonding between carboxyl and pyridyl moieties proved to be extremely fruitful for the formation of supramolecular hydrogen-bonded liquid crystals (SMHBLCs).4–7 Thus, intermolecular hydrogen-bond interactions have shown great potential in the preparation of new liquid crystalline systems, especially in thermotropic liquid crystals.8–12 Extensive researches have been focused on the field of hydrogen-bonded liquid crystalline complexes.13–18 Most of the SMHLCs studied are based on the rod-like intermolecular hydrogen-bonding19–25 and there is much interest towards the formation of angular supramolecular hydrogen bonded liquid crystals.15 The concept of SMHLCs has been successively applied in many applications in scientific and technological fields.26–30 Number of hydrogen-bonded binary systems, based on pyridine as proton acceptors and carboxylic acid derivatives as the proton donors, have been investigated and reviewed and reviewed extensively.5,31–36 More recently, hydrogen-bonding between pyridines and benzoic acids moieties was used to induce nematic, smectic and columnar mesophases.5,37,38 On the other hand, the azobenzene units are of special interest due to their ability for trans-cis-isomerization upon irradiation with UV light. In supramolecular azo-based liquid crystals, via hydrogen bonding interaction, the rigid-rod core is lengthened, and thus induces liquid crystalline behaviour that may not occur in the individual components.39,40 Such induced mesomorphic behaviour is attributed to the formation of the elongated hydrogen-bonded complex. Moreover, the induction of liquid crystalline character is also associated with the strength of hydrogen bonds formed between the interacting components. This would accordingly be affected by the change in polarity and/or polarisability of both components.
Continuing our work, the present study is to investigate the thermal behavior resulting from intermolecular hydrogen-bond formation between the 4-alkoxyphenylazobenzoic acids,41,42 In, and 4-(4′-pyridylazophenyl)4′′-alkoxybenzoates,19 IIm. Therefore, we constructed all possible 1
:
1 supramolecular complexes In/IIm with various lengths of the terminal alkoxy chains in order to monitor the effect of similar and/or different proportionating of alkoxy-chain length on the mesophase formation, shown in Scheme 1. Also investigated, the effect of introducing additional azobenzene unit to the linear supramolecular complexes previously reported19 through hydrogen-bond formation between 4-alkoxy benzoic acids (IIIn) as proton donors and 4-(4′-pyridylazophenyl)4′′-alkoxybenzoates (IIm) as proton acceptors (IIIn/IIm). A comparison was made, also between the present series (In/IIm) and previously investigated angular isomer, In/IVm,15 to investigate the effect of the linearity of the structure on the mesophase stability.
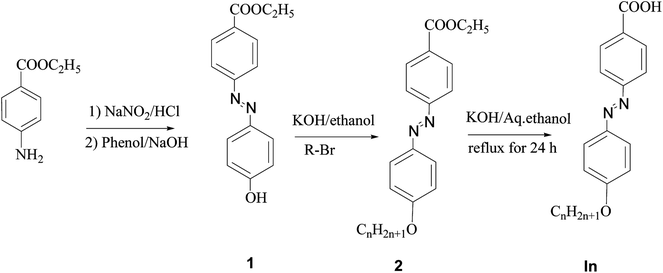 |
| Scheme 1 Preparation of 4-alkoxyphenylazobenzoic acids In. | |
2. Experimental
4-Hexyloxy benzoic acid, 4-octoyloxy benzoic acid, 4-decyloxy benzoic acid and 4-dodecyloxy benzoic acid were obtained from Merck (Germany). N,N′-dicyclohexylcarbodiimide (DCC) and 4-dimethylaminopyridine (DMAP) were purchased from Aldrich (Wisconsin, USA). All chemicals were used without further purification. All the solvents used such as dichloromethane, ethanol and methanol were of pure grade and purchased from Aldrich (Wisconsin, USA).
Calorimetric measurements were carried out using a TA Instruments Co. Q20 Differential Scanning Calorimeter (DSC; USA). The DSC was calibrated using the melting temperature and enthalpy of indium and lead. DSC investigation was carried out for small samples (2–3 mg) placed in aluminum pans. All measurements were achieved upon heating to stability temperature and cooling to room temperature with heating rate of 10 °C min−1 in inert atmosphere of nitrogen gas (30 ml min−1) and all transition recorded from the second heating scan.
Transition temperatures for the individual components and their 1
:
1 associated complexes (In/IIm), were determined by DSC, and the types of the mesophase identified by a standard polarized light microscope (PLM, Wild, Germany) attached with Mettler FP82HT hot stage. The temperature is measured by thermocouple attached to the temperature controller. Measurements were made twice and the results have accuracy in transition temperature within ±0.2 °C.
2.1. Preparation of ethyl 4-hydroxyphenylazobenzoate
Ethyl 4-aminobenzoate (0.01 mol) was dissolved in concentrated hydrochloric acid and cooled in ice-salt bath to 0 °C. To the resulting solution, a cold aqueous solution of sodium nitrite (0.03 mol) was added drop-wise with stirring. During the addition, the temperature did not exceed 2 °C. After complete addition, the cold mixture was added drop-wise to an ice-cold solution of phenol (0.01 mol) in sodium hydroxide (0.03 mol). The mixture was further stirred at 0 °C for one hour then acidified with dilute hydrochloric acid. The solid separated was filtered and crystallized twice from ethanol. The product was TLC pure and gave melting point 163 °C that agreed with that reported in the literature.41
2.2. Preparation of ethyl 4-n-alkoxyphenylazobenzoates
These were prepared by the method described previously.41 Ethyl 4-hydroxyphenylazobenzoate (0.001 mol) in ethanolic KOH (0.02 mol, 20 ml) to which the appropriate amount of 1-bromoalkane (0.0012 mol) was added drop-wise while stirring. Stirring was continued at room temperature for two days. The solid separated was filtered off, the solution evaporated and the residue crystallized twice from ethanol. The products were TLC pure and give transition temperatures as given in the literature.41
2.3. Preparation of 4-alkoxyphenylazobenzoic acids In
The esters (2) were hydrolyzed to the corresponding acids by refluxing in aqueous ethanolic potassium hydroxide (0.02 mol, 20 ml) for 24 hours then acidified by dilute HCl to give the corresponding acids. Again the products were crystallized twice from ethanol to give TLC pure solids that give transition temperatures agreeing with those reported in the literature (Scheme 2).41,43
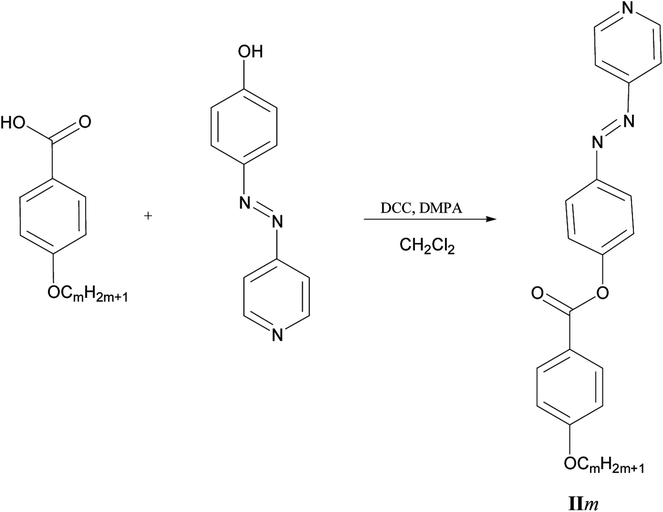 |
| Scheme 2 Preparation of 4-(4′-pyridylazophenyl)-4′′-alkyloxybenzoates IIm. | |
4-[2-[4-(Hexyloxy)phenyl]diazenyl]benzoic acid. Yield 85%, 1H NMR (300 MHz, CDCl3): δ/ppm: δ = 8.13 (m, 2H, Ar–H), 7.92 (m, 4H, Ar–H), 7.18 (m, 2H, Ar–H), 4.09 (t, J = 7.7 Hz, 2H,
), 1.98–1.74 (m, 2H,
), 1.40–1.31 (m, 6H, CH2), 0.93 (t, J = 7.1 Hz, 3H, CH3). Elemental analyses: found (calc.): C, 69.65 (69.92); H, 6.60 (6.79); N, 8.83 (8.58).
2.4. Preparation of 4-(4′-pyridylazophenyl)4′′-alkyloxybenzoate IIm
4-(4′-Pyridylazo)phenol (0.30 g, 1.35 mmol) with hexyloxybenzoic acid (0.30 g, 1.35 mmol), DCC (0.28 g, 1.35 mmol), and few crystals of 4-dimethylaminopyridine (DMAP) as a catalyst, were dissolved in 20 ml 25% mixture of CH2Cl2 and THF(1
:
3 CH2Cl2
:
THF); the mixture was stirred at room temperature for 48 h. The solid materials were filtered off and the solvent was removed under vacuum. The obtained crude product was purified by recrystallization from ethanol.
4-(4′-Pyridylazophenyl)-4′′-hexyloxybenzoate. Yield 77% 1H NMR (CDCl3) δ 8.81 (d, J = 8.3, 2H, Ar–H), 8.17 (d, J = 8.5, 2H, Ar–H), 8.05 (d, J = 8.4, 2H, Ar–H), 7.70 (d, J = 8.5, 2H, Ar–H), 7.43 (d, J = 8.5, 2H, Ar–H), 6.70 (d, J = 8.5, 2H, Ar–H), 4.08 (t, J = 6.5 Hz, 2H,
), 1.91–1.79 (m, 2H,
), 1.59–1.22 (m, 6H, CH2), 0.91 (t, J = 6.8 Hz, 3H, CH3). Elemental analyses: found (calc.): C, 71.76 (71.44); H, 6.63 (6.25); N, 10.22 (10.41).
2.5. Preparation of supramolecular hydrogen-bonded complexes, In/IIm
Supramolecular complexes (In/IIm), in the 1
:
1 molar ratios of any two complementary components, were prepared by melting the appropriate amounts of each component, stirring to give an intimate blend and then, cooling with stirring to room temperature (Scheme 3). For example to prepare the supramolecular complex I10/II8: 0.0382 mg of 4-decyloxyphenylazo-benzoic acid, I10 and 0.0431 mg of 4-(4′-pyridylazophenyl)-4′′-octyloxybenzoate II8 were mixed together using the method described above.
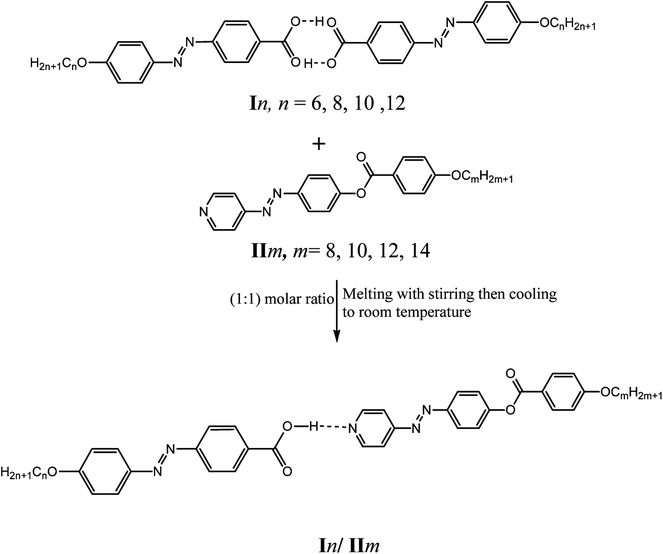 |
| Scheme 3 Preparation of 1 : 1 supramolecular hydrogen-bonded complexes (In/IIm). | |
All diagrams constructed by plotting transition temperatures of the supramolecular hydrogen-bonded complex versus alkoxy-chain length (n or m), the symbol “o” denotes crystal-mesophase, "●" smectic C-to-nematic mesophase and “□” nematic–isotropic transitions.
2.6. Structural confirmation
The purity of the prepared compounds were checked with thin-layer chromatography using TLC-sheets coated with silica gel (E. Merck), whereby single spots were detected by a UV-lamp.
The molecular formulae of the prepared compounds were confirmed via elemental analyses, infrared, 1H-NMR, and mass spectroscopy. The results agreed, within the permissible limits, with the proposed structures and with those reported in the literature.15,19
The formation of the supramolecular complexes (In/IIm) were confirmed by DSC investigation as well as Fourier Transform Infrared Spectroscopy (FTIR), which carried out using Nicolet iS 10 Thermo scientific.
3. Results and discussion
3.1. Fourier transform infrared spectroscopy (FTIR)
Infrared spectra measurements were performed for the individual components i. e. 4-alkoxyphenylazo benzoic acids (In) and 4-(4′-pyridylazophenyl)4′′-alkoxybenzoates (IIm) as well as to their supramolecular complexes (In/IIm). For example, the IR spectrum of I10 and II8 are given in Fig. 1 and 2, respectively. The infrared absorption spectra for all the homologues (In) were found to be identical with the mesomeric shift of the carboxylic carbonyl which attributed to the peak at 1678.5 cm−1. Therefore, the alkoxy-chain length (n) has no significant effect on the wave number of the peaks. This specific band shows that the carboxylic acid exists in the dimer form shown in Scheme 3 via intermolecular hydrogen bonding between the carboxylic groups.19 Similarly, the spectral data of the proton acceptors, IIm, shows that the alkyl chain length has again no effect on the observed IR spectra for a given homologues series. In order to confirm the formation of the supramolecular complexes, In/IIm, IR spectra for the prepared complexes were measured. Fig. 3 shows the IR spectra of the complex I10/II8 prepared from equal molar amounts of I10 and II8. A comparative IR spectra of I10, II8, and I10/II8 is shown in Fig. 3. It had been reported that43–47 the carbonyl stretching region as well as the Fermi resonance vibration bands (A-type, 3100 cm−1; C-type, 1925 cm−1) in the spectra of the complex is an important evidence of intermolecular hydrogen bonding, where, the lower wave number (
= 1678.5 cm−1) of C
O group stretching vibration of the cyclic carboxylic acid is shifted to a higher wave number (
= 1681.9 cm−1) of the hydrogen-bonded dimers.
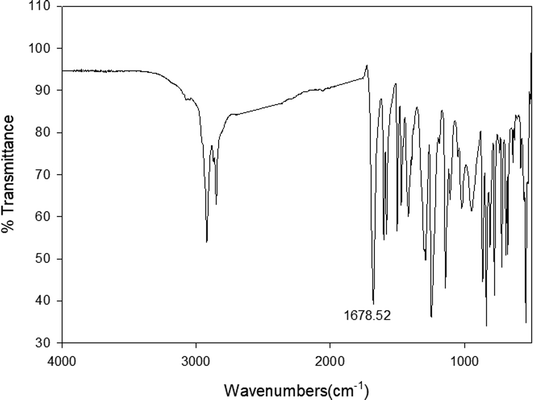 |
| Fig. 1 FTIR spectrum of the 4-decyloxyphenylazobenzoic acid, I10. | |
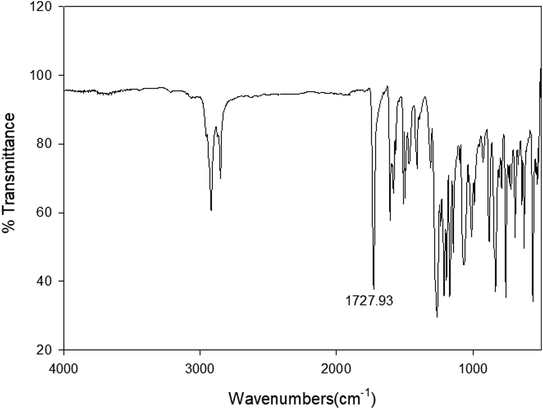 |
| Fig. 2 FTIR spectrum of 4-(4′-pyridylazophenyl)4′′-octyloxybenzoate, II8. | |
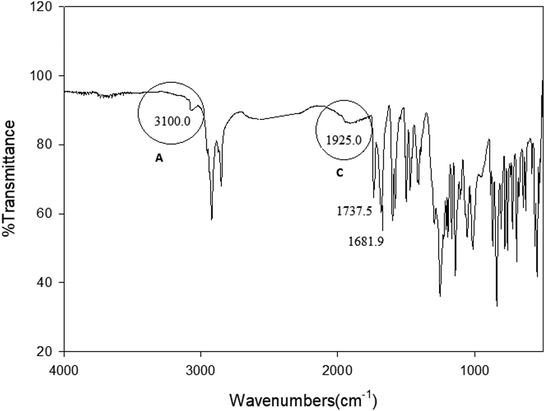 |
| Fig. 3 FTIR spectra of the 1 : 1 supramolecular complex I10/II8. | |
3.2. Phase behavior of 1
:
1 molar mixtures as a function of the alkoxy-chain length
The phase behavior of the prepared complexes In/IIm was investigated by PLM and DSC. PLM was used to detect phase transition temperatures as well as textures of the liquid crystal phases exhibited by the complexes In/IIm. PLM observations were verified by the DSC measurements and types of mesophases were identified for all prepared supramolecular complexes In/IIm.
It should be mentioned that, the mesophase behavior of the prepared 4-alkoxy phenylazo-benzoic acids In exhibit smectic C phase (SmC) with relatively high transition temperatures and nematic phase with very small range,15,41 while the azopyridines IIm exhibit smectic A phase (SmA) except the lower chain compound II8 is dimorphic possessing SmA and nematic phases.19 Therefore, it was interesting to investigate the phase behavior of the complexes resulting from mixing compounds In and IIm.
Transition temperatures and their corresponding values of transition enthalpies, as measured by DSC, for the 1
:
1 molar supramolecular complexes In/IIm are collected in Table 1. All transition temperatures were represented graphically once as a function of alkoxy-chain length (n) on the acid complement and another against (m) on the pyridine-based complement in Fig. 4 and 5; respectively. As can be seen from Table 1, Fig. 4 and 5, independent of either n or m of the acid and base components, smectic C and nematic mesophases are exhibited by all complexes in addition to, two crystalline phases given as Cr1 and Cr2. It can also be seen from Fig. 4 that the nematic phase range decreases gradually with the increase of the acid alkoxy-chain length (n). SmC phase range is increasing with increasing the acid alkoxy-chain length (n) and decreases gradually with the increase of base alkoxy-chain length (m).
Table 1 Phase transition temperatures (°C), enthalpy of transitions (kJ mol−1) and transition entropies (J mol−1 K−1), of the 1
:
1 supramolecular complexes (In/IIm)a
System |
TCr1–Cr2 |
TCr2–SmC |
ΔHCr2–SmC |
TSmC–N |
ΔHSmC–N |
ΔSSmC–N |
TN–I |
ΔHN–I |
ΔSN–I |
Abbreviations: TCr1–Cr2 = crystal to crystal transition; TCr2–SmC = crystal to smectic C phase transition; TSmC–N = smectic C to nematic transition; TN–I = nematic to isotropic liquid transition. ΔHCr2–SmC = crystal to smectic C phase transition; ΔHSmC–N = smectic C to nematic transition; ΔHN–I = nematic to isotropic liquid transition; ΔSSmC–N = smectic C to nematic transition; ΔSN–I = nematic to isotropic liquid transition. |
I6/II8 |
106.3 |
153.2 |
37.1 |
230.6 |
5.7 |
12.6 |
245.7 |
3.1 |
6.2 |
I6/II10 |
91.3 |
149.4 |
40.5 |
232.5 |
3.6 |
7.9 |
244.4 |
3.0 |
5.9 |
I6/II12 |
126.3 |
136.9 |
29.8 |
231.9 |
3.9 |
8.2 |
236.7 |
2.7 |
5.5 |
I6/II14 |
118.8 |
133.8 |
32.0 |
229.5 |
2.8 |
5.8 |
235.4 |
2.0 |
3.9 |
I8/II8 |
104.4 |
149.4 |
33.8 |
191.0 |
4.1 |
8.8 |
229.4 |
2.8 |
5.6 |
I8/II10 |
104.0 |
135.0 |
32.9 |
204.4 |
3.0 |
6.3 |
230.0 |
2.2 |
4.3 |
I8/II12 |
104.4 |
133.2 |
27.2 |
205.6 |
2.1 |
4.4 |
223.0 |
1.8 |
3.6 |
I8/II14 |
105.0 |
123.5 |
39.0 |
206.8 |
2.9 |
6.0 |
221.3 |
2.1 |
4.2 |
I10/II8 |
126.3 |
152.5 |
41.1 |
193.0 |
4.6 |
9.9 |
240.7 |
2.3 |
4.5 |
I10/II10 |
131.9 |
141.9 |
29.5 |
199.0 |
2.0 |
4.2 |
236.1 |
1.7 |
3.3 |
I10/II12 |
111.3 |
131.9 |
34.5 |
210.0 |
3.6 |
7.5 |
230.0 |
2.3 |
4.6 |
I10/II14 |
114.2 |
128.2 |
32.8 |
215.6 |
2.5 |
5.1 |
231.3 |
2.0 |
4.0 |
I12/II8 |
103.1 |
153.6 |
39.7 |
198.0 |
3.8 |
8.1 |
234.4 |
2.4 |
4.7 |
I12/II10 |
112.5 |
138.8 |
31.9 |
202.0 |
3.7 |
7.8 |
229.3 |
2.7 |
5.4 |
I12/II12 |
118.1 |
134.4 |
38.2 |
205.0 |
3.1 |
6.5 |
225.0 |
2.2 |
4.4 |
I12/II14 |
122.0 |
135 |
31.5 |
212.0 |
2.7 |
5.6 |
220.1 |
1.9 |
3.9 |
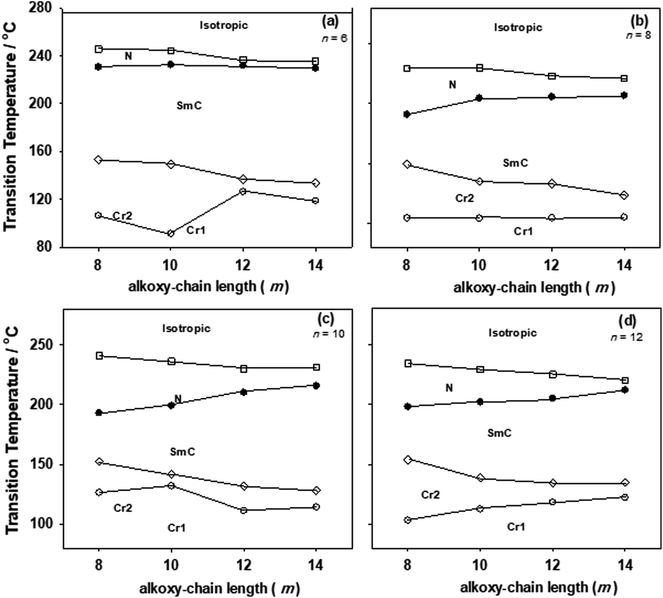 |
| Fig. 4 Effect of the alkoxy-chain length (m) of the pyridine-based derivatives (IIm) on mesophase behavior of the 1 : 1 supramolecular hydrogen-bonded complexes, (a) n = 6; (b) n = 8; (c) n = 10: (d) n = 12, TCr1–Cr2 = crystal 1 to crystal 2 transition (○); TCr2–SmC = crystal 2 to smectic C phase transition (◊); TSmC–N = smectic C to nematic transition (●);TN–I = nematic to isotropic liquid transition (□). | |
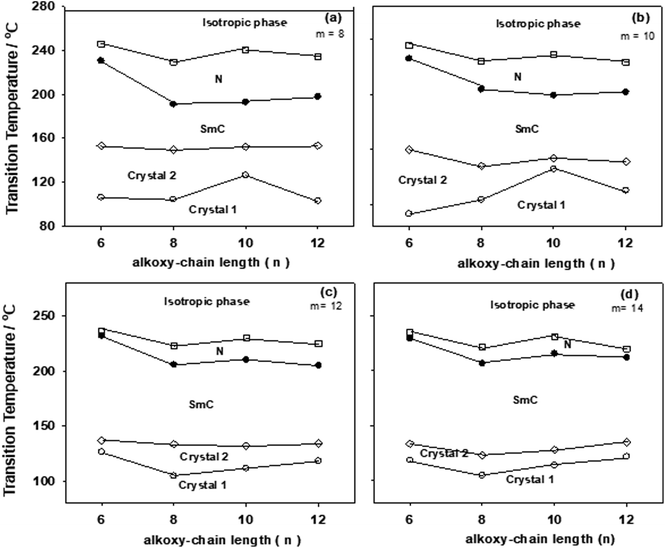 |
| Fig. 5 Effect of the alkoxy-chain length (n) of the pyridine-based derivatives (In) on mesophase behavior of the 1 : 1 supramolecular hydrogen-bonded complexes, (a) m = 8; (b) m = 10; (c) m = 12: (d) m = 14, TCr1–Cr2 = crystal 1 to crystal 2 transition (○); TCr2–SmC = crystal 2 to smectic C phase transition (◊); TSmC–N = smectic C to nematic transition (●);TN–I = nematic to isotropic liquid transition (□). | |
Fig. 4 showed that for a given value of m, the melting temperatures of the supramolecular complexes are slightly affected by the alkoxy-chain length of the acid complement (n). Therefore, the acid In or the azo-pyridine IIm components exhibit very small range of N phase, the resulted complexes In/IIm constructed via intermolecular hydrogen bond showed an nematic phase with relatively wide range. Representative examples of polarizing optical microscopic images of the mesophases are shown in Fig. 6. Fig. 6 show images of SmA phase of azo-pyridine II8, SmC phase and schlieren texture of nematic phase of the dimorphic complex I10/II8.
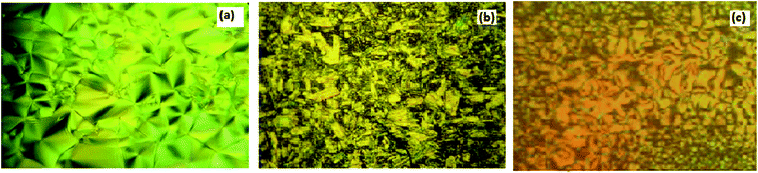 |
| Fig. 6 PLM textures of (a) SmA phase of II8 at 105.0 °C; (b) SmC phase of complex I10/II8 at 183.0 °C; and (c) nematic phase of the complex I10/II8 at 225.0 °C. | |
3.3. Effect of the extra phenylazo group on the mesophase behaviour of the supramolecular hydrogen-bonded complexes
In order to investigate the effect of incorporating an extra phenylazo group to molecules of the simple 4-alkoxybenzoic acids (IIIn) on their mesophase behaviour of 1
:
1 molar mixtures with the 4-(4′-pyridylazophenyl)4′′-alkoxybenzoates (IIIn/IIm), a comparison was made between the mesophase stabilities (TC) of 4-n-alkoxyphenylazo benzoic acids (In) supramolecular hydrogen-bonded complexes (In/IIm) and their corresponding 4-n-alkoxy benzoic acids (IIIn/IIm),19 as a function of alkoxy-chain length (n) and represented graphically in Fig. 7. The study revealed that increasing the length of the mesogenic core by a phenylazo moiety resulted in an increase of the stability of both the smectic and nematic mesophases. In addition, the SmC mesophase range observed in the present investigated mixtures In/IIm has been increased upon incorporation of the phenylazo group in IIIn/IIm.
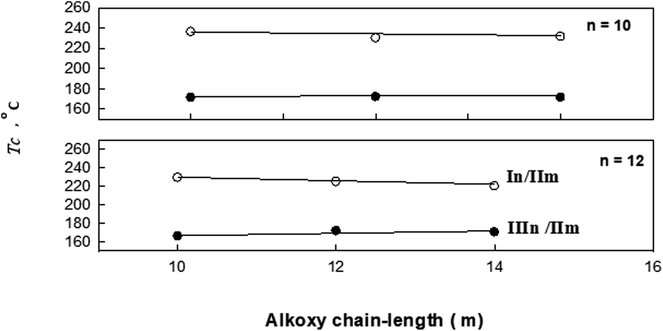 |
| Fig. 7 Effect of alkoxy-chain length (m) on mesophase behavior of the In/IIm and IIIn/IIm 1 : 1 supramolecular complexes. | |
3.4. Comparison between isomeric linear and angular supramolecular hydrogen-bonded complexes
It is interesting to compare the mesophase stability (TC) of the present series of linear supramolecular complexes (In/IIm) with the previously investigated angular complexes (In/IVm) in which only the base complement (IIm) is replaced by the 4-(3′-pyridylazophenyl)4′′-alkoxybenzoates, IVm.15 In such a comparison, the effect of a modification will be investigated on the stability of the mesophase (TC) as well as the type of mesophase observed. Fig. 8 represents the TC dependencies on the alkoxy-chain length (n) attached to the common 4-n-alkoxyphenylazo benzoic acid complement (In) for these two, linear and angular, complexes.
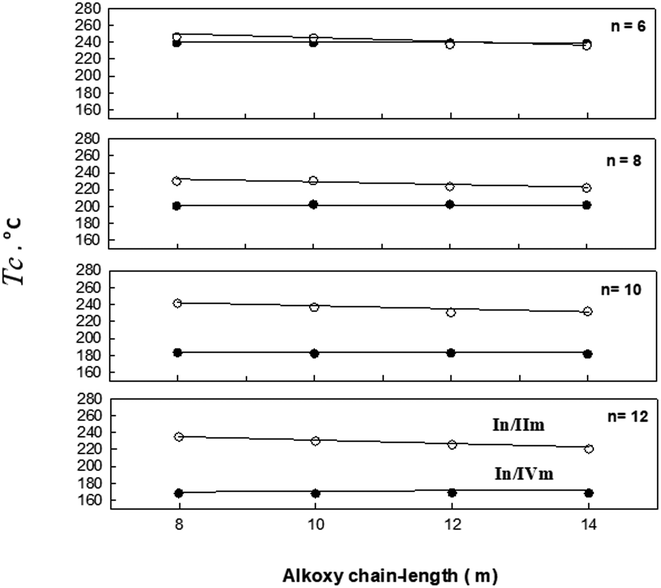 |
| Fig. 8 Effect of alkoxy-chain length (m) on mesophase behavior of the In/IIm and In/IVm supramolecular complexes. | |
3.5. Entropy changes
The entropies of the smectic C–to-nematic and nematic-to–isotropic transitions were estimated for all the 1
:
1 supramolecular hydrogen-bonded complexes (In/IIm), and the results are appended to Table 1. As seen from Table 1, independent of the length of the alkoxy-chains (n or m), all entropies of N–I transitions (ΔSN–I) are of lower values than those of the corresponding ΔSSmC–N transitions. The decrease observed in ΔSN–I is presumably a reflection of the increase in the biaxiality of the mesogenic group, resulted in the flexible terminal alkoxy-chain, being less strongly anchored at its end, giving the resulting decrease in conformational entropy.40 This dependency indicates that the length of the terminal substituents, whether on the acid (n) or the base (m) complements, has led to an irregular ΔSN–I. An explanation that the entropies do not correlate well with either of the two terminal alkoxy-chain length (n or m), may be related to the irregular change of lateral adhesion upon the increase of the total molecular length.48
4. Conclusion
New types of 1
:
1 hydrogen-bonded supramolecular complexes were prepared. The formation of complexes via intermolecular hydrogen bond between the complementary components was confirmed by DSC and FTIR spectroscopy. It was found that, their supramolecular complexes In/IIm exhibit relatively high range nematic phase in addition to the SmC phase. Moreover, the nematic phases are exhibited by all complexes regardless of the length of the terminal alkoxy chains either on the proton donor In or the proton acceptor IIm. A comparison was made between the present series of complexes and the previously investigated angular, In/IVm, and simple linear, IIIn/IIm, analogues, revealed that increasing the length of the mesogenic core, by inclusion of a phenylazo moiety, increases the stabilities of both the smectic C and nematic mesophases. It was also observed that the entropy change vary irregularly with either of the alkoxy-chain length n or m.
Conflicts of interest
The authors declare no conflict of interest.
Acknowledgements
Authors gratefully acknowledge the Deanship of Scientific Research, Taibah University for the support of this research work, research group No. 60333.
References
- A. A. E. Sakr, T. Zaki, O. Elgabry, M. A. Ebiad, S. M. El-Sabagh and M. M. Emara, Appl. Clay Sci., 2018, 160, 263–269 CrossRef CAS.
- S. Dutt, P. F. Siril and S. Remita, RSC Adv., 2017, 7, 5733–5750 RSC.
- L. Lisuzzo, G. Cavallaro, F. Parisi, S. Milioto and G. Lazzara, Ceram. Int., 2018 Search PubMed.
- D. Demus, J. W. Goodby, G. W. Gray, H. W. Spiess and V. Vill, Low Molecular Weight Liquid Crystals I: Calamitic Liquid Crystals, Handbook of Liquid Crystals, vol. 2A, John Wiley & Sons, 2011 Search PubMed.
- C. M. Paleos and D. Tsiourvas, Liq. Cryst., 2001, 28, 1127–1161 CrossRef CAS.
- C. M. Paleos and D. Tsiourvas, Angew. Chem., Int. Ed. Engl., 1995, 34, 1696–1711 CrossRef CAS.
- M. Naoum, A. Fahmi and M. Alaasar, Mol. Cryst. Liq. Cryst., 2008, 482, 57–70 CrossRef CAS.
- T. Kato and J. M. Frechet, J. Am. Chem. Soc., 1989, 111, 8533–8534 CrossRef CAS.
- T. Kato and J. M. Frechet, Macromolecules, 1989, 22, 3818–3819 CrossRef CAS.
- A. J. Thote and R. B. Gupta, Ind. Eng. Chem. Res., 2003, 42, 1129–1136 CrossRef CAS.
- M.-J. Brienne, J. Gabard, J.-M. Lehn and I. Stibor, J. Chem. Soc., Chem. Commun., 1989, 1868–1870 RSC.
- H. Ahmed, M. Hagar, M. Alaasar and M. Naoum, Liq. Cryst., 2018, 1–10 CrossRef.
- J. W. Goodby, R. J. Mandle, E. J. Davis, T. Zhong and S. J. Cowling, Liq. Cryst., 2015, 42, 593–622 CrossRef CAS.
- D. Janietz and M. Bauer, Macromol. Chem. Phys., 1991, 192, 2635–2640 CrossRef CAS.
- H. Ahmed and M. Naoum, Liq. Cryst., 2016, 43, 222–234 CrossRef CAS.
- M. Fouzai, R. Hamdi, S. Ghrab, T. Soltani, A. Ionescu and T. Othman, J. Mol. Liq., 2018, 249, 1279–1286 CrossRef CAS.
- M. Alaasar, S. Poppe, Q. Dong, F. Liu and C. Tschierske, Chem. Commun., 2016, 52, 13869–13872 RSC.
- H. K. Bisoyi and Q. Li, Chem. Rev., 2016, 116, 15089–15166 CrossRef CAS PubMed.
- M. Naoum, A. Fahmi and M. Alaasar, Mol. Cryst. Liq. Cryst., 2008, 487, 74–91 CrossRef CAS.
- M. Okumuş and Ş. Özgan, Liq. Cryst., 2014, 41, 1293–1302 CrossRef.
- A. Ashok Kumar, B. Sridevi, M. Srinivasulu, P. Chalapathi and D. Potukuchi, Liq. Cryst., 2014, 41, 184–196 CrossRef CAS.
- J. R. Wolf, T. Zhao, C. Landorf and D. J. Dyer, Liq. Cryst., 2014, 41, 721–730 CrossRef CAS.
- S. Sreehari Sastry, K. Lakshmi Sarada, K. Mallika, C. Nageswara Rao, S. Lakhminarayana and H. Sie Tiong, Liq. Cryst., 2014, 41, 1483–1494 CrossRef CAS.
- M. D. Miranda, F. V. Chávez, T. M. Maria, M. E. S. Eusebio, P. Sebastião and M. R. Silva, Liq. Cryst., 2014, 41, 1743–1751 CrossRef CAS.
- D. A. Paterson, A. Martínez-Felipe, S. M. Jansze, A. TM Marcelis, J. MD Storey and C. T. Imrie, Liq. Cryst., 2015, 42, 928–939 CAS.
- R. Dong, Y. Zhou, X. Huang, X. Zhu, Y. Lu and J. Shen, Adv. Mater., 2015, 27, 498–526 CrossRef CAS PubMed.
- Y. Yang and M. W. Urban, Chem. Soc. Rev., 2013, 42, 7446–7467 RSC.
- K. Liu, Y. Kang, Z. Wang and X. Zhang, Adv. Mater., 2013, 25, 5530–5548 CrossRef CAS PubMed.
- X. Yan, F. Wang, B. Zheng and F. Huang, Chem. Soc. Rev., 2012, 41, 6042–6065 RSC.
- D. González-Rodríguez and A. P. Schenning, Chem. Mater., 2010, 23, 310–325 CrossRef.
- T. Kato, in Handbook of Liquid Crystals, ed. D. Demus, J. W. Goodby, G. W. Gray, H.-W. Spiess and V. Vill, Wiley-VCH, Weinheim, 1998, vol. 2B, pp. 969–979 Search PubMed.
- T. Kato, N. Mizoshita and K. Kanie, Macromol. Rapid Commun., 2001, 22, 797–814 CrossRef CAS.
- T. Kato, Science, 2002, 295, 2414–2418 CrossRef CAS PubMed.
- C. Tschierske, J. Mater. Chem., 2001, 11, 2647–2671 RSC.
- T. Kato, N. Mizoshita and K. Kishimoto, Angew. Chem., Int. Ed., 2006, 45, 38–68 CrossRef CAS PubMed.
- J.-M. Lehn, Science, 1993, 260, 1762–1764 CrossRef CAS PubMed.
- A. Kohlmeier and D. Janietz, Liq. Cryst., 2007, 34, 65–71 CrossRef CAS.
- T. Kato and Y. Kamikawa, Handbook of liquid crystals, 2014 Search PubMed.
- S. M. Jansze, A. Martínez-Felipe, J. M. Storey, A. T. Marcelis and C. T. Imrie, Angew. Chem., 2015, 127, 653–656 CrossRef.
- P. J. Collings, M. Hird and C. Tschierske, Angew. Chem., Int. Ed. Engl., 1997, 36, 2017 Search PubMed.
- D. Janietz and M. Bauer, Macromol. Chem. Phys., 1991, 192, 2635–2640 CrossRef CAS.
- H. Ahmed, M. Naoum and G. Saad, Liq. Cryst., 2016, 43, 1259–1267 CrossRef CAS.
- M. Lizu, M. Lutfor, N. Surugau, S. How and S. E. Arshad, Mol. Cryst. Liq. Cryst., 2010, 528, 64–73 CrossRef CAS.
- W. Cleland and M. M. Kreevoy, Science, 1994, 264, 1887–1890 CrossRef CAS PubMed.
- A. Martinez-Felipe, A. G. Cook, J. P. Abberley, R. Walker, J. M. Storey and C. T. Imrie, RSC Adv., 2016, 6, 108164–108179 RSC.
- A. Martínez-Felipe and C. T. Imrie, J. Mol. Struct., 2015, 1100, 429–437 CrossRef.
- A. Ghanem and C. Noel, Mol. Cryst. Liq. Cryst., 1987, 150, 447–472 Search PubMed.
- C. Imrie, Liq. Cryst., 1989, 6, 391–396 CrossRef CAS.
Footnote |
† Electronic supplementary information (ESI) available. See DOI: 10.1039/c8ra07692h |
|
This journal is © The Royal Society of Chemistry 2018 |
Click here to see how this site uses Cookies. View our privacy policy here.