DOI:
10.1039/C8RA07212D
(Paper)
RSC Adv., 2018,
8, 33968-33971
Transition metal/Brønsted acid cooperative catalysis enabled facile synthesis of 8-hydroxyquinolines through one-pot reactions of ortho-aminophenol, aldehydes and alkynes†
Received
29th August 2018
, Accepted 24th September 2018
First published on 3rd October 2018
Abstract
A convenient and straightforward three-component one-pot strategy has been developed for the synthesis of 8-hydroxyquinoline derivatives. Under the cooperative catalysis of silver(I) triflate and trifluoroacetic acid, ortho-aminophenol reacted with a range of aldehydes and alkynes under mild reactions, affording the corresponding 8-hydroxyquinoline derivatives with good to excellent yields. These transformations exhibited exceptional substrate generality and functional group compatibility.
Introduction
As a privileged scaffold, 8-hydroxyquinoline has been found in many natural products and drug candidates with remarkable biological and pharmaceutical activities.1–9 Moreover, 8-hydroxyquinolines have been gaining attention in metallocene complex catalyzed transformations, in which they are utilized as effective multidentate ligands.10–21 In view of the remarkable importance of this class of heterocyclic compounds, the field of 8-hydroxyquinoline synthesis is continuously gaining attention. Although 8-hydroxyquinolines could be prepared by hydrolysis of 8-haloquinolines22 or 8-aminoquinolines,23 the latter two quinolone derivatives were not easily accessible. The classical Skraup's procedure for quinoline derivatives suffered from harsh reaction conditions and poor yields.24–26 The Friedlander reaction was limited due to the instability of 2-aminobenzaldehyde, which is mostly in situ generated by reduction of 2-nitrobenzaldehyde derivatives.27,28 In the last 20 years, transition-metal or Brønsted-acid catalysis toward quinoline derivatives have been extensively studied.29–36 What's confusing is that 8-hydroxyquinolines were rarely involved in these reports.37–40 Considering the importance in pharmacology and functional materials chemistry, the development of more facile and economic synthetic approached for 8-hydroxyquinolines is highly desirable.
Initially 8-hydroxyquinoline was envisioned to arise from hydroarylation-cyclization of propargylamine C followed by oxidation/aromatization, which might to be formed through nucleophilic addition of acetylide-[M] B to iminium A. That is, under the cooperative catalysis of transition metal and Brønsted-acid, 8-hydroxyquinoline might be efficiently generated through one-pot reaction of ortho-aminophenol, aldehyde and alkyne (Scheme 1). Suitable catalyst match was considered to be crucial for this transformation.
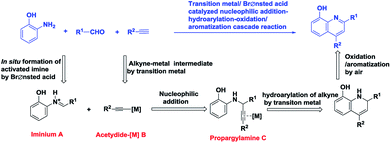 |
| Scheme 1 Possible synthetic pathway for 8-hydroxyquinoline. | |
Results and discussion
To establish proof of concept, we carried out the reaction of ortho-aminophenol 1, benzaldehyde 2a and phenylacetylene 3a as the archetypal. Our study began with the evaluation of different metal catalyst in the presence of one equivalent of TFA (Table 1, entry 1–6). We were pleased to find that the reaction with AgOTf as alkye-philic catalyst could generate the desired 8-hydroxyquinoline compound 4a in 72% yield with benzylamine 5a as the byproduct41 (entry 1). Other Brønsted acid were also examined (entry 7–9). Suitable strength of acidity proved to be crucial for the multicomponent transformation. TFA seemed to be the best. Control experiments indicated the necessity of the both catalysts. Without the participation of TFA, no product could be obtained. And the reaction got obviously suppressed in the absence of silver (entry 10 and 11). Unexpectedly, when the ratio of TFA increased to 400 mol%, 8-hydroxyquinoline compound 4a was collected with higher yield along with greatly reduced reaction time (entry 12 and 13). To our delight, when AgOTf decreased to 0.5 mol%, a parallel yield was also observed (entry 14–16). Solvent and temperature optimization revealed that other medias are not suitable in terms of yield comparable to that in DCE at 80 °C (entry 17–21).
Table 1 Optimization of reaction conditionsa

|
Entry |
TM |
BA |
Solvent |
Time/h |
Yieldb (4a) [%] |
Yieldb (5a) [%] |
Conditions: 1 (1.1 mmol, 1.1 equiv.), 2a (1 mmol, 1 equiv.), 3a (1.2 mmol, 1.2 equiv.), TM (transition metal, 5 mol%), BA (Brønsted acid, 100 mol%) in 4.0 mL solvent under atmosphere at 80 °C for 8 h. Isolated yield based on 2a. TFA (400 mol%). AgOTf (5 mol%) and TFA (400 mol%). AgOTf (1 mol%) and TFA (400 mol%). AgOTf (1 mol%) and TFA (200 mol%). AgOTf (0.5 mol%) and TFA (400 mol%). AgOTf (0.5 mol%) and TFA (400 mol%) at 60 °C. AgOTf (0.5 mol%) and TFA (400 mol%) at 100 °C. AgOTf (0.5 mol%) and TFA (400 mol%) at 60 °C. |
1 |
AgOTf |
TFA |
DCE |
8 |
72 |
12 |
2 |
AgOAc |
TFA |
DCE |
12 |
Complex |
|
3 |
Cu(OTf)2 |
TFA |
DCE |
8 |
63 |
15 |
4 |
Pd(OAc)2 |
TFA |
DCE |
10 |
— |
70 |
5 |
PdCl2 |
TFA |
DCE |
10 |
Complex |
6 |
Ph3PAuCl |
TFA |
DCE |
12 |
Complex |
7 |
AgOTf |
TfOH |
DCE |
8 |
38 |
50 |
8 |
AgOTf |
PTSA |
DCE |
8 |
23 |
— |
9 |
AgOTf |
H3PO4 |
DCE |
8 |
43 |
— |
10 |
AgOTf |
— |
DCE |
10 |
No reaction |
|
11 |
— |
TFA |
DCE |
14 |
68 |
14 |
12c |
— |
TFA |
DCE |
5 |
80 |
10 |
13d |
AgOTf |
TFA |
DCE |
1 |
96 |
— |
14e |
AgOTf |
TFA |
DCE |
1 |
95 |
— |
15f |
AgOTf |
TFA |
DCE |
6 |
75 |
16 |
16g |
AgOTf |
TFA |
DCE |
2 |
94 |
— |
17g |
AgOTf |
TFA |
Toluene |
4 |
80 |
10 |
18g |
AgOTf |
TFA |
MeCN |
6 |
74 |
12 |
19h |
AgOTf |
TFA |
THF |
5 |
No reaction |
20i |
AgOTf |
TFA |
DMF |
5 |
No reaction |
21j |
AgOTf |
TFA |
DCE |
2 |
Sluggish reaction |
With the optimized reaction conditions in hand (Table 1, entry 16), we examined the AgOTf/TFA system for one-pot synthesis of 8-hydroxyquinoline derivatives from ortho-aminophenol 1, various aldehydes 2 and alkynes 3. Table 2 illustrates the wide generality and substrate scope of this tandem reaction. To our delight, satisfactory yields were observed for all substrate examined. For the variation on aromatic aldehyde, different electron-donating or electron-withdrawing substituents at either the para-, meta- or ortho-position on the aryl group were all tolerated to yield 4a–q in high yields. Aromatic aldehydes with electron-donating group afforded better results than those with electron-withdrawing group (4a–d vs. 4e–f). Notably, strongly electron-deficient p-nitrobenzaldehyde gave a complex reaction, without desired product identified. Comparing with electron effect, the steric hindrance exerted less influence on these transformations. It should be noted steric crowded aromatic aldehydes with multiple substituents could work effectively in the one-pot cascade reactions to give 8-hydroxyquinoline derivatives 4l–q in acceptable yields. Aliphatic aldehyde and heteroaromatic aldehyde were effective substrates, generating 4r and 4s in 93% and 87% yield respectively. The reactions proceeded smoothly for aryl alkynes with either electron-withdrawing or electron-donating substituents present on the aromatic ring to afford the desired products in moderate to excellent yields (4t–4v). Electron density provided certain impact on the reactions, and those with electron-releasing group gave a slightly low yield. Alkyl alkyne was also an effective substrate. 8-Hydroxyquinoline derivative 4w was collected in nearly equivalent yield. The structures of obtained compounds were characterized by NMR spectrum and further confirmed by single-crystal X-ray diffraction analysis of compound 4c and 4e.
Table 2 Synthesis of 8-hydroxyquinoline derivatives from aminophenol, aldehydes and alkynesa,b

|
Conditions:1 (1.1 mmol), 2 (1 mmol), 3 (1.2 mmol.), AgOTf (0.5 mol%), TFA (400 mol%) in 4.0 mL DCE under atmosphere at 80 °C. Isolated yield based on 2. |
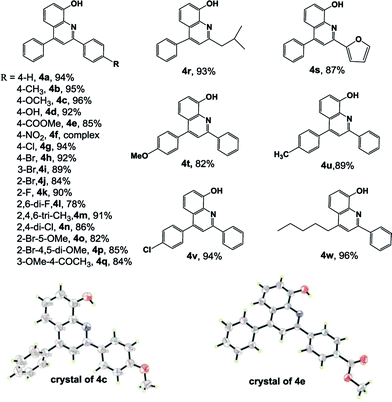 |
Experimental section
Materials and methods
All chemicals were obtained from commercial suppliers and used without further purification. Melting points were measured using a TY-60 microscopic apparatus. 1H NMR and 13C NMR spectra were recorded with Bruker 400M or 600M instruments. Chemical shifts were measured relative to tetramethylsilane (0.00 ppm) as internal standard.
Synthetic procedures for 8-hydroxyquinoline derivatives
Ortho-aminophenol (1.1 mmol) and aldehyde (1 mmol) were added into a round-bottomed flask with 4 mL dichloroethane. The vessel was then charged with silver(I) triflate (0.5 mol%) and trifluoroacetic acid (400 mol%). After stirring for 10 min, alkyne (1.2 mmol) was added dropwise. The resulting mixture was stirred at 80 °C until aldehyde consumed completely. The reddish-brown solution was cooled to room temperature, diluted with dichloromethane (10 mL) and water (10 mL), and then neutralized with NaHCO3 (aq) to pH 7. The aqueous layer was extracted with dichloromethane and the combined organic phase was dried over anhydrous sodium sulfate. After evaporation of solvent, the residue was purified by silica gel chromatography (petroleum ether/ethyl acetate).
Conclusions
In summary, we have disclosed a highly efficient and practical multicomponent reaction for the construction of 8-hydroxyquinoline scaffold under cooperative AgOTf/TFA catalysis with commercially available materials. A range of valuable 8-hydroxyquinoline derivatives are obtained in excellent yields under mild conditions. Various substituents are tolerated on aldehydes and alkynes, thus enabling the vast expansion of substituent architectures on 8-hydroxyquinoline framework.
Conflicts of interest
There are no conflicts to declare.
Acknowledgements
We are grateful to the support from the National Natural Science Foundation of China (21602207), the Foundation of Henan Educational Committee (17A150022) and the Doctoral Research Fund of Zhengzhou University of Light Industry (2014BSJJ009).
References
- S. Ariyasu, Y. Mizuseda, K. Hanaya and S. Aoki, Chem. Pharm. Bull., 2014, 62, 642–648 CrossRef CAS PubMed.
- W. Li, E. Gerard, O. Masaki, O. M. Bautista-Aguilera, I. Tsutomu, M. Ignacio, I. Isabel, S. Abdelouahid, M. B. H. Youdim and R. Alejandro, Eur. J. Med. Chem., 2014, 80, 543–561 CrossRef PubMed.
- M. Kubanik, H. Holtkamp, T. SC6hnel, S. M. F. Jamieson and C. G. Hartinger, Organometallics, 2015, 34, 5658–5668 CrossRef CAS.
- Y. N. Song, H. Xu, W. Chen, P. Zhan and X. Liu, MedChemComm, 2015, 6, 61–74 RSC.
- F. Prati, C. Bergamini, R. Fato, O. Soukup, J. Korabecny, V. Andrisano, M. Bartolini and M. L. Bolognesi, ChemMedChem, 2016, 11, 1284–1295 CrossRef CAS PubMed.
- M. Shamsipur, Z. Memari, M. R. Ganjali, P. Norouzi and F. Faridbod, J. Pharm. Biomed. Anal., 2016, 118, 356–362 CrossRef CAS PubMed.
- S. Narwal, S. Kumar and P. K. Verma, Res. Chem. Intermed., 2017, 43, 2765–2798 CrossRef CAS.
- B. Pippi, P. Reginatto, G. D. Machado, V. Z. Bergamo, D. F. Lana, M. L. Teixeira, L. L. Franco, R. J. Alves, S. F. Andrade and A. M. Fuentefria, Med. Mycol., 2017, 55, 763–773 CrossRef PubMed.
- X. Yang, P. Cai, Q. Liu, J. Wu, Y. Yin, X. Wang and L. Kong, Bioorg. Med. Chem., 2018, 26, 3191–3201 CrossRef CAS PubMed.
- W. M. Wang, H. X. Zhang, S. Y. Wang, H. Y. Shen, H. L. Gao, J. Z. Cui and B. Zhao, Inorg. Chem., 2015, 54, 10610–10622 CrossRef CAS PubMed.
- J. S. Carletto, K. C. Roux, H. F. Maltez, E. Martendal and E. Carasek, J. Hazard. Mater., 2008, 157, 88–93 CrossRef CAS PubMed.
- S. Roy, S. Basu, M. Anitha and D. K. Singh, Korean J. Chem. Eng., 2017, 34, 1740–1747 CrossRef CAS.
- X. Su, F. Yang, Y. Wu and Y. Wu, Org. Biomol. Chem., 2018, 16, 2753–2756 RSC.
- S. Y. Wang, W. M. Wang, H. X. Zhang, H. Y. Shen, L. Jiang, J. Z. Cui and H. L. Gao, Dalton Trans., 2016, 45, 3362–3371 RSC.
- W. M. Wang, Y. H. Ren, S. Wang, C. F. Zhang, Z. L. Wu, H. Zhang and M. Fang, Inorg. Chim. Acta, 2016, 453, 452–456 CrossRef CAS.
- F. Núñez-Zarur and R. Vivas-Reyes, J. Mol. Struct.: THEOCHEM, 2008, 850, 127–134 CrossRef.
- E. V. Nosova, G. N. Lipunova, T. V. Stupina, P. A. Slepukhin, M. S. Valova and V. N. Charushin, Russ. J. Gen. Chem., 2014, 84, 1771–1776 CrossRef CAS.
- O. B. Petrova, M. O. Anurova, A. A. Akkuzina, R. R. Saifutyarov, E. V. Ermolaeva, R. I. Avetisov, A. V. Khomyakov, I. V. Taydakov and I. C. Avetissov, Opt. Mater., 2017, 69, 141–147 CrossRef CAS.
- Y. Wu, T. Guo, D. Shu, W. Zhang, F. Luan, L. Shi and D. Guo, Luminescence, 2018, 33, 855–862 CrossRef CAS PubMed.
- Y. Tang, Y. Huo, H. U. Sheng, K. Zhang, F. Zhao and X. Ouyang, Chem. J. Chinese. U., 2014, 35, 48–53 CAS.
- S. Wu, X. Zhong, H. Zeng, W. You and W. Zhou, J. Lumin., 2018, 195, 120–125 CrossRef CAS.
- S. Xia, L. Gan, K. Wang, Z. Li and D. Ma, J. Am. Chem. Soc., 2016, 138, 13493–13496 CrossRef CAS PubMed.
- M. Gershon, D. D. Clarke and M. Gershon, Monatsh. Chem., 1996, 127, 331–337 CrossRef.
- S. E. Denmark and S. Venkatraman, J. Org. Chem., 2006, 71, 1668–1676 CrossRef CAS PubMed.
- H. Saggadi, D. Luart, N. Thiebault, I. Polaert, L. Estel and C. Len, RSC Adv., 2014, 4, 21456–21464 RSC.
- G. A. Ramann and B. J. Cowen, Tetrahedron Lett., 2015, 56, 6436–6439 CrossRef CAS.
- J. Marcocontelles, E. PeL Rezmayoral, A. Samadi, M. A. D. C. Carreiras and E. Soriano, Chem. Rev., 2009, 109, 2652–2671 CrossRef CAS PubMed.
- M. Fallahmehrjardi, Mini-Rev. Org. Chem., 2017, 14, 187–196 CAS.
- T. Iwai and M. Sawamura, ACS Catal., 2015, 5, 5031–5040 CrossRef CAS.
- E. Vessally, L. Edjlali, A. Hosseinian, A. Bekhradnia and M. D. Esrafili, RSC Adv., 2016, 6, 99781–99793 RSC.
- X. Xu, W. Liu, Z. Wang, Y. Feng, Y. Yan and X. Zhang, Tetrahedron Lett., 2016, 57, 226–229 CrossRef CAS.
- J. B. Bharate, R. A. Vishwakarma and S. B. Bharate, RSC Adv., 2015, 5, 42020–42053 RSC.
- H. Z. S. Huma, R. Halder, S. S. Kalra, J. Das and J. Iqbal, Tetrahedron Lett., 2002, 43, 6485–6488 CrossRef.
- X. Zhang, B. Liu, X. Shu, Y. Gao, H. Lv and J. Zhu, J. Org. Chem., 2012, 77, 501–510 CrossRef CAS PubMed.
- R. I. Khusnutdinov, A. R. Bayguzina and U. M. Dzhemilev, J. Organomet. Chem., 2014, 768, 75–114 CrossRef CAS.
- S. Naidoo and V. Jeena, Synthesis, 2017, 49, 2621–2631 CrossRef CAS.
- N. Sudhapriya, A. Nandakumar and P. T. Perumal, RSC Adv., 2014, 4, 58476–58480 RSC.
- X. Zhang, X. Xu, L. Yu and Q. Zhao, Asian J. Org. Chem., 2014, 3, 281–284 CrossRef CAS.
- Y. Zhang, P. Li and L. Wang, J. Heterocycl. Chem., 2011, 48, 153–157 CrossRef CAS.
- G. A. Ramann and B. J. Cowen, Molecules, 2016, 21, 1–23 CrossRef PubMed.
- Side product 5a was envisioned to be formed through reduction of imine by dihydroquinoline intermediate as proposed by Iqbal group's work (ref. 33).
Footnote |
† Electronic supplementary information (ESI) available. CCDC 1864450 and 1864452. For ESI and crystallographic data in CIF or other electronic format see DOI: 10.1039/c8ra07212d |
|
This journal is © The Royal Society of Chemistry 2018 |