DOI:
10.1039/C8RA06525J
(Paper)
RSC Adv., 2018,
8, 39005-39012
Application of high EPA-producing Mortierella alpina in laying hen feed for egg DHA accumulation
Received
2nd August 2018
, Accepted 8th November 2018
First published on 20th November 2018
Abstract
Polyunsaturated fatty acids (PUFAs), especially eicosapentaenoic acid (EPA, C20:5) and docosahexaenoic acid (DHA, C22:6), are beneficial for human health. In this study, we selected a high EPA content (30% in total fatty acids) strain of Mortierella alpina CCFM 698 that overexpressed an ω-3 fatty acid desaturase from Phytophthora parasitica, and investigated the cell growth and lipid accumulation of this strain in a 65 L airlift fermenter with glucose batch feeding. The maximum cell dry weight was 28.7 g L−1 and the highest total fatty acid content was 33.0% (w/w) in cell dry weight. The highest EPA yield was 1.8 g L−1. Both low and high dose supplementation of this strain into the feed of laying hens increased DHA accumulation in the yolk. The highest DHA content of 7.61 mg g−1 yolk was achieved in Fengda-1 laying hens with 4% supplementation and the DHA production per egg was 118.46 mg. However, Hy-Line Brown laying hens displayed a higher DHA production per egg and the value was 131.50, 131.72, 131.95 mg with 1.5%, 2%, 4% supplementation, respectively. The lowest ratio of ω-6/ω-3 PUFAs (3.53) was obtained in Hy-Line Brown laying hens with 4% supplementation. These results suggest that M. alpina CCFM 698 can be used as an alternative source of ω-3 PUFAs in feed to produce nutritious eggs with high DHA content.
Introduction
ω-3 Polyunsaturated fatty acids (PUFAs), such as eicosapentaenoic acid (EPA, C20:5) and docosahexaenoic acid (DHA, C22:6), are very important to human health and clinical studies have demonstrated their beneficial effects on the prevention and treatment of cardiovascular disease, immune disorders, diabetes and cancer.1–5 Marine fish oil is the main EPA and DHA source for humans, but due to human population growth and oceanic pollution, the current practice of harvesting fish is unsustainable.6
Mortierella alpina is an oleaginous filamentous fungus rich in various fatty acids and a source of arachidonic acid (AA, C20:4), which is used as an additive in infant formulas.7 The fungus has also been recognised as a potential source for commercial EPA production.8 Ando et al. overexpressed an endogenous ω-3 fatty acid desaturase in M. alpina 1S-4, and increased the EPA accumulation to 0.68 g L−1 with a maximum EPA content in total fatty acids of 40%.9 However, ω-3 fatty acid desaturase from M. alpina is only active at low temperatures, which cannot meet the demands of industrialised production. Recently, Okuda overexpressed ω-3 fatty acid desaturase SDD17 from Saprolegnia diclina in M. alpina 1S-4, resulting in an EPA content more than 18% of total fatty acids at room temperature, which increased to 26.4% after fermentation was optimised.10 Our laboratory also engineered an M. alpina that can produce EPA at room temperature through overexpression of an ω-3 fatty acid desaturase from Pythium aphanidermatum; the EPA content achieved was 18% of total fatty acids.8 We then overexpressed an ω-3 fatty acid desaturase from Phytophthora parasitica and obtained a strain named CCFM 698 that had a higher EPA content (30% of total fatty acids).
Hens' eggs are one of the most nutritious foods in daily diets due to their abundant fatty acids, high protein content, vitamins and minerals.11 However, the typical content of ω-3 PUFAs in eggs is low and the ratio of ω-6/ω-3 PUFAs is over 12
:
1, far exceeding the recommended intake ratio of 3–5
:
1, and thus not meeting the dietary requirements for PUFAs.12 Many studies have shown that laying hens can convert PUFAs in feed and enrich them in egg yolk, thereby improving the fatty acid composition of the yolk. The main additives used to produce ω-3 PUFAs in eggs are flaxseed, fish oil and microalgae. The fatty acid conversion rates of different additives in laying hens' feed differ. Petrovic et al. investigated the effects of feeding different concentrations of flaxseed rich in α-linolenic acid (ALA, C18:3) on egg PUFAs and found that the ratio of ω-6/ω-3 PUFAs decreased continuously during the first 5 weeks, and the main contributor to the increase of ω-3 PUFAs was ALA rather than DHA.12 Saleh studied the effects of different concentrations of fish oil on egg fatty acid composition and found that theω-3 PUFA content in yolk was significantly higher with supplementation than in the control group; supplementation at 2.5% was most favourable for increasing ω-3 PUFA content.13 Fredriksson et al. supplemented laying hens' feed with microalgae rich in EPA and ALA and found that the DHA concentration in egg yolk was significantly increased.14 However, the production of PUFAs in eggs through the addition of linseed, fish oil and microalgae to the feed of laying hens faces with some problems such as a low conversion rate of ALA, “fishy taint” or off-flavors, increased susceptibility to oxidation, high cost of fish oil and difficulties in the cultivation of microalgae.
As the stable production of PUFAs by M. alpina is feasible and some genetically modified strains are rich in EPA, adding this fungus to laying hens' feed to enhance the DHA levels in egg yolk is appealing. In our previous study we added M. alpina CCFM 442 with 6% EPA of total fatty acids to the diet of laying hens and investigated the change in yolk fatty acid content.15 With 10 days of supplemental feeding, the DHA and AA contents of the egg yolks in the addition group were respectively 3-fold and 2-fold higher than those of the control group and the ratio of ω-6/ω-3 decreased from 13
:
1 to 8
:
1, which is beneficial to human health. The egg yolk DHA was mainly converted from EPA when feeding with M. alpina and the EPA content of strain CCFM 422 was only 6%, leading to DHA production per egg of no more than 50 mg. Thus, a higher EPA strain should be selected to feed to laying hens for high DHA production. In this study, we chose the high EPA (30% of total fatty acids) strain M. alpina CCFM 698, which overexpresses an ω-3 fatty acid desaturase from P. parasitica, to investigate its fermentation optimisation and supplementation in laying hen feed for egg yolk DHA accumulation.
Materials and methods
Microorganism selection and cultivation
M. alpina CCFM 698 was obtained from our laboratory and preserved in the China General Microbiological Culture Collection Center (CGMCC no. 11820). M. alpina CCFM 698 was inoculated on potato dextrose agar plates and incubated for approximately 30 days at 25 °C. Spores collection and mycelium activation were performed as in our previous study.16 The activated seed culture was inoculated at 10% (v/v) into a 65 L airlift fermenter containing 40 L of batch-fed fermentation medium (30 g L−1 glucose, 20 g L−1 soybean meal, 1 g L−1 KH2PO4·H2O, 5 g L−1 KNO3 and 0.25 g L−1 MgSO4·7H2O). Airlift fermenters were maintained at 28 °C and ventilation is set at 0.5–1.5 v/v min−1 with fermenter pressure controlled at 0.5 bar. pH was maintained at 6.0 by autoaddition of 4 mol L−1 HCl or 6 mol L−1 NaOH and foam production was controlled by adding a defoaming agent (polypropylene glycol 2000). During the fermentation, sterile sampling was performed at every 24 h and the steam sterilisation was performed before and after sampling.
Determination of cell dry weight and glucose concentration
100 mL fermentation culture was collected in measuring cups, filtered with 200 mesh sieves and then washed with distilled water. The collected mycelia were frozen at −80 °C, freeze-dried, and the cell dry weight was measured by weighing method. The glucose concentration in the culture medium was measured using a glucose oxidase kit (Shanghai Rongsheng Biotech Co., Ltd., China) according to the manufacturers' instructions.
Analysis of mycelia, hens diet and egg fatty acid methyl esters
The mycelia were harvested by filtration and washed with distilled water, followed by freeze-drying, then fatty acids were extracted and methyl-esterified as described previously.17 The fatty acid compositions were analysed by GCMS-QP2010 Ultra (Shimadzu Co., Japan) with pentadecanoic acid (C15:0) as the internal standard to quantify the fatty acid methyl esters (FAMEs). The temperature programme was as described in our previous study.18 Analysis of the fatty acid composition of the hens' diet and egg yolks was the same as for the mycelia.
Preparation of mycelium feed and laying hens diet formulation
The standard hens' diet was provided by Rongda Poultry Co., Ltd. Dry mycelia was added to the standard diet at 0.5%, 1%, 1.5%, 2% or 4% (w/w) to prepare the mycelium-enriched feed. The laying hens' feeding experiment was performed in two batches (low doses of 0.5%, 1% or 1.5% mycelium feed and high doses of 2%, 4% mycelium feed). The laying hens received 14 h light per day with room temperature maintained at 20 °C. Sufficient feed and water was supplied for hens' ad libitum consumption. The overall experiment was divided into three phases: an adaptation period of feeding with the standard diet (14 days), a supplementation period of feeding with mycelium-enriched feed (14 days) and a wash-out period (14 days) of feeding with the standard diet. Thirty weeks old of Hy-Line Brown or Fengda-1 laying hens, from Rongda Poultry Co., Ltd, was individually housed. Fifteen hens was in each group and separately housed in the cages of 45 × 45 cm with a height of 45 cm. All experimental protocols were approved by the Animal Ethics Committee of Jiangnan University, China, and were performed according to the ethical guidelines of the European Community guidelines (Directive 2010/63/EU).
Results and discussion
Cell growth and lipid accumulation of M. alpina CCFM 698 in 65 L airlift fermenter
Airlift fermenters can best preserve mycelia because they have no mechanical agitation, which improves the production of biomass and metabolites.19 To obtain a high production of biomass and EPA in M. alpina CCFM 698, the strain was cultivated in a 65 L airlift fermenter (Fig. 1). The glucose concentration in the culture medium greatly influences the production of biomass and lipids; a high glucose concentration will slow the glucose consumption, cell growth and lipid accumulation, while a low glucose concentration will not meet the carbon requirements for growth and lipid accumulation.20,21 Therefore, to improve the utilisation rate of glucose and the accumulation of EPA, fermentation was conducted with glucose batch feeding. Our results showed that the glucose consumption rate was high, especially before 48 h (Fig. 1A), and glucose was exhausted at 264 h. Correspondingly, the cell dry weight increased quickly in the first 48 h, reaching a maximum of 28.7 g L−1 at 264 h, and then remaining stable (Fig. 1B). The highest total fatty acid content was also obtained at 264 h, reaching 33.0% (w/w) in cell dry weight (Fig. 1C). As shown in Fig. 1D, the highest EPA yield of 1.8 g L−1 was achieved at 336 h, which was much higher than that (0.6 g L−1) found in our previous M. alpina strain engineered to heterogeneously express an ALA-preferring delta-6 desaturase.22 In addition, the EPA yield in this study was similar to that of M. alpina expressing ω-3 fatty acid desaturases from P. aphanidermatum and S. diclina whose EPA yields were respectively 1.7 g L−1 and 1.8 g L−1.6,8 Recently, however, DuPont assembled 30 copies of 9 different heterologous genes in Y. lipolytica and the recombination strain accumulated 15% (w/w) EPA in cell dry weight, indicating that it is another promising strain for the production of EPA.23 In this study, the ω-6 PUFAs AA yield in M. alpina CCFM 698 was only 0.4 g L−1 at 336 h and the ratio of EPA/AA was nearly 5. The high yield of ω-3 PUFAs EPA and low yield of ω-6 PUFAs AA was beneficial for DHA accumulation in egg yolks when this strain was added to laying hen feed as the major egg DHA source was converted from ω-3 PUFAs in the diet.24
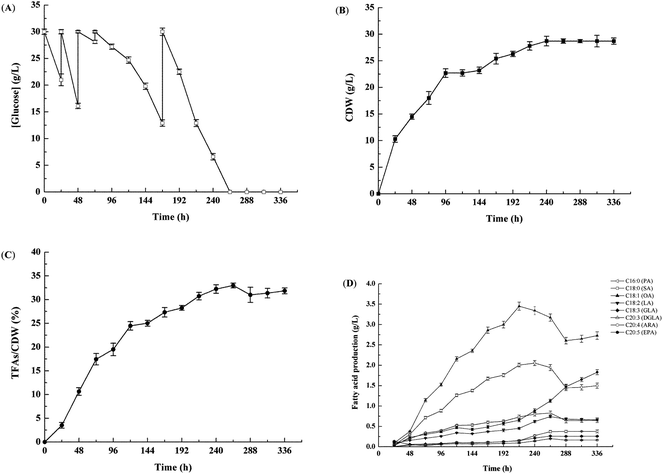 |
| Fig. 1 Cell growth and lipid accumulation of M. alpina CCFM 698 in 65 L airlift fermenter. (A) Glucose concentration in the growth media, (B) cell dry weight (CDW), (C) total fatty acids (TFAs) content (w/w, CDW), (D) fatty acid composition. | |
Fatty acid composition of standard diet and M. alpina CCFM 698
To obtain sufficient mycelia for feed supplementation, multiple batches of air lift fermentation were carried out and M. alpina CCFM 698 was harvested for use in a laying hen experiment. The composition of the standard diet and M. alpina biomass is shown in Table 1. Furthermore, the fatty acid content of the standard diet and M. alpina CCFM 698 is shown in Table 2.
Table 1 Composition of standard diet and M. alpina CCFM 698
|
Standard diet |
M. alpina CCFM 698 |
Metabolisable energy (MJ kg−1) |
12.02 ± 0.78 |
|
Crude protein |
15.08 ± 0.46 |
7.5 ± 0.33 |
Methionine |
1.28 ± 0.08 |
0.25 ± 0.02 |
Methionine + cystine |
2.32 ± 0.24 |
0.43 ± 0.03 |
Lysine |
3.45 ± 0.05 |
0.68 ± 0.05 |
Calcium |
3.52 ± 0.14 |
ND |
Non-phytate phosphorus |
0.53 ± 0.04 |
ND |
Table 2 Fatty acid content in standard diet and M. alpina CCFM 698a
Fatty acids |
Standard diet |
M. alpina CCFM 698 |
% of total fatty acids |
mg fatty acid/g diet |
% of total fatty acids |
mg fatty acid/g M. alpina |
Values are mean of three samples ± standard error of the mean. |
C14:0 |
— |
— |
0.67 ± 0.02 |
2.62 ± 0.08 |
C16:0(PA) |
11.69 ± 0.84 |
5.23 ± 0.48 |
9.42 ± 0.32 |
37.03 ± 1.26 |
C17:0 |
— |
— |
0.24 ± 0.01 |
0.93 ± 0.04 |
C18:0(SA) |
2.38 ± 0.15 |
1.06 ± 0.09 |
15.81 ± 1.04 |
62.17 ± 4.09 |
C18:1(ω-9,OA) |
33.59 ± 1.24 |
15.02 ± 1.65 |
16.77 ± 0.89 |
65.96 ± 3.50 |
C18:2(ω-6, LA) |
47.38 ± 2.31 |
21.18 ± 1.70 |
8.56 ± 0.42 |
33.64 ± 1.65 |
C18:3(ω-6, GLA) |
— |
— |
3.02 ± 0.13 |
11.89 ± 0.51 |
C18:3(ω-3, ALA) |
4.53 ± 0.30 |
2.02 ± 0.15 |
— |
— |
C20:0 |
— |
— |
1.36 ± 0.05 |
5.34 ± 0.20 |
C20:3(ω-6, DGLA) |
— |
— |
0.35 ± 0.00 |
1.37 ± 0.02 |
C22:3 |
— |
— |
2.76 ± 0.05 |
10.84 ± 0.20 |
C20:4(ω-6, AA) |
— |
— |
12.48 ± 0.74 |
49.08 ± 2.91 |
C20:5(ω-3, EPA) |
— |
— |
23.70 ± 1.13 |
93.20 ± 4.44 |
C22:0 |
— |
— |
2.42 ± 0.02 |
9.51 ± 0.08 |
C24:0 |
— |
— |
1.88 ± 0.04 |
7.40 ± 0.16 |
Total ω-6 PUFAs |
47.38 ± 2.31 |
21.18 ± 1.70 |
24.41 ± 0.98 |
95.98 ± 3.85 |
Total ω-3 PUFAs |
4.53 ± 0.30 |
2.02 ± 0.15 |
23.70 ± 1.02 |
93.27 ± 4.01 |
ω-6/ω-3 PUFAs |
10.46 ± 0.51 |
— |
1.03 ± 0.04 |
— |
The standard diet mainly contained oleic acid (OA, C18:1) and linoleic acid (LA, C18:2), but also contains a small amount of ALA, palmitic acid (PA, C16:0) and stearic acid (SA, C18:0). However, the composition of fatty acids in M. alpina CCFM 698 is various and reasonable. The PUFAs accounted for 51.64% of total fatty acids and the EPA content was up to 23.70%. The EPA content of this strain was much higher than that of our previous strain M. alpina CCFM 442, which had 6.09% EPA in total fatty acids, and M. alpina CCFM 698 also had a much lower ω-6/ω-3 ratio of 1.03 compared with that of M. alpina CCFM 442, whose value was 4.41.25 Thus, of the two strains, M. alpina CCFM 698 is a better feed additive to produce high DHA eggs, which are beneficial for the human health.
Effects of low dose supplementation of M. alpina CCFM 698 on egg yolk fatty acid composition
To investigate the effects of M. alpina CCFM 698 supplementation on egg yolk fatty acid composition, 0.5%, 1% and 1.5% dry mycelia were added to the standard diet of laying hens. The major PUFA trend in egg yolk during the supplementary feeding period is shown in Fig. 2. The DHA content of the 0.5% supplementation group showed only a slow upward trend in the whole supplementary period. The DHA content of the 1% supplementation group was higher than that of the 0.5% group and reached a peak value on the 10th day. In the 1.5% supplementation group, DHA accumulation was low in the first 5 days but increased rapidly from the 5th to 9th days, and then increased slowly. The egg yolk fatty acid composition at the end of the supplementation period is shown in Table 3. The egg yolk fatty acid compositions of the supplementation groups were similar, and there was no EPA accumulation in any group, which is most likely a result of rapid hepatic conversion to DHA, in accordance with other published finding.14,25 As shown in Tables 3 and 4, DHA in egg yolks increased with the increase of M. alpina CCFM 698 supplemental feeding dose. The DHA yield reached 7.31 mg g−1 egg yolk and 131.50 mg per egg in the 1.5% supplementation group, which was a 1.86- and 1.90- fold increase compared to the control. Correspondingly, the ω-6/ω-3 ratio in the egg yolks decreased to 3.93 in the 1.5% supplementation group while the ratio in the control group was 6.92. The low ω-6/ω-3 PUFAs ratio is more consistent with the recommended intake ratio.26
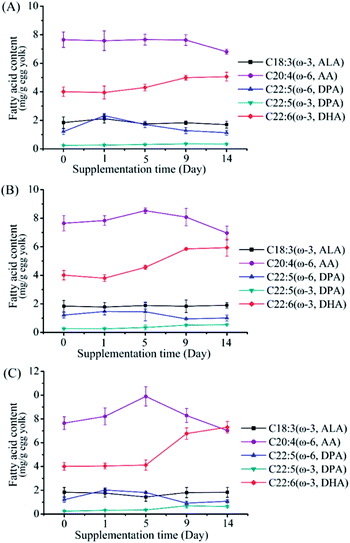 |
| Fig. 2 Changes of fatty acid composition in egg yolk of different groups in the supplementation period of feeding with mycelium-enriched feed. (A) 0.5% supplementation group, (B) 1% supplementation group, (C) 1.5% supplementation group. Values are mean of three samples ± standard error of the mean. | |
Table 3 Egg yolk fatty acid content (mg per g egg yolk) of low dose groups at the end of supplementation perioda
Fatty acids |
Control (0%) |
0.5% |
1% |
1.5% |
Values are mean of three samples ± standard error of the mean. Values with the same letter in the same row are not significantly. |
C16:0 |
48.24 ± 3.05a |
42.19 ± 1.93a |
44.98 ± 2.16a |
45.62 ± 2.31a |
C16:1 |
10.61 ± 1.21a |
10.86 ± 0.95a |
10.55 ± 0.56a |
10.44 ± 0.48a |
C18:0 |
27.71 ± 1.89a |
25.11 ± 1.04a |
27.26 ± 0.84a |
28.18 ± 0.47a |
C18:1(ω-9, OA) |
112.64 ± 7.90a |
98.13 ± 1.62a |
104.92 ± 3.47a |
111.08 ± 6.63a |
C18:2(ω-6, LA) |
32.21 ± 3.72a |
29.65 ± 3.50a |
32.08 ± 6.42a |
29.74 ± 2.27a |
C18:3(ω-6, GLA) |
0.32 ± 0.10a |
0.38 ± 0.05a |
0.35 ± 0.04a |
0.33 ± 0.02a |
C18:3(ω-3, ALA) |
1.87 ± 0.44a |
1.70 ± 0.24a |
1.90 ± 0.19a |
1.83 ± 0.42a |
C20:3(ω-6, DGLA) |
0.15 ± 0.03a |
0.16 ± 0.05a |
0.18 ± 0.03a |
0.19 ± 0.04a |
C20:4(ω-6, AA) |
7.23 ± 0.54a |
6.81 ± 0.18a |
6.96 ± 0.49a |
7.03 ± 0.09a |
C22:5(ω-6, DPA) |
1.14 ± 0.17a |
1.13 ± 0.18a |
1.01 ± 0.21a |
1.08 ± 0.14a |
C22:5(ω-3, DPA) |
0.28 ± 0.08a |
0.34 ± 0.06a |
0.54 ± 0.02b |
0.64 ± 0.10b |
C22:6(ω-3, DHA) |
3.92 ± 0.17a |
5.06 ± 0.31bc |
5.94 ± 0.60c |
7.31 ± 0.49d |
Total ω-6 PUFAs |
41.06 ± 3.61a |
38.12 ± 3.32a |
40.59 ± 7.01a |
38.37 ± 2.02a |
Total ω-3 PUFAs |
6.07 ± 0.41a |
7.89 ± 0.23b |
8.39 ± 0.75bc |
9.78 ± 0.58c |
ω-6/ω-3 PUFAs |
6.92 ± 0.28a |
5.07 ± 0.28b |
4.82 ± 0.45bc |
3.93 ± 0.20c |
Table 4 Performance, egg quality, conversion rate of EPA to DHA in laying hens of low dose groupsa
Fatty acids |
Control (0%) |
0.5% |
1% |
1.5% |
Values are mean of six samples ± standard error of the mean. Values with the same letter in the same row are not significantly. Feed conversion rate (%) = (laying rate × egg weight)/feed intake. EPA conversion rate (%) = (experimental group egg yolk DHA production—control group egg yolk DHA production)/EPA intake. |
Laying rate (%) |
87.14 ± 4.64a |
86.67 ± 4.91a |
85.72 ± 5.79a |
89.04 ± 3.96a |
Feed intake (g) |
118.05 ± 4.61a |
119.02 ± 3.32a |
118.78 ± 3.18a |
119.43 ± 5.54a |
Egg weight (g) |
68.09 ± 1.33a |
67.26 ± 1.57a |
66.87 ± 1.90a |
68.72 ± 2.66a |
Egg yolk weight (g) |
17.60 ± 0.82a |
17.64 ± 0.75a |
17.40 ± 0.66a |
18.02 ± 0.41a |
Egg albumen weight (g) |
44.53 ± 1.72a |
43.98 ± 2.36a |
43.62 ± 2.12a |
44.62 ± 2.34a |
Egg shell weight (g) |
5.96 ± 1.10a |
5.64 ± 0.85a |
5.85 ± 0.74a |
6.08 ± 1.03a |
Feed conversion rate (%) |
50.26 ± 2.64a |
48.97 ± 3.72a |
48.26 ± 4.23a |
51.23 ± 3.11a |
EPA intake (mg) |
0.00a |
55.46 ± 2.45b |
110.70 ± 3.21c |
166.66 ± 4.12d |
DHA per egg (mg) |
69.07 ± 2.01a |
89.41 ± 2.76b |
106.94 ± 3.34c |
131.50 ± 3.22d |
EPA conversion rate (%) |
0.00a |
36.67 ± 1.58b |
34.20 ± 1.72b |
37.39 ± 1.44b |
As the conversion of EPA in feed supplements to DHA in egg yolk is very important for industrialised applications, the conversion rate of EPA to DHA in the laying hens in the different groups was analysed (Table 4). The conversion rates of EPA to DHA were all more than 30% when M. alpina CCFM 698 was supplemented, which is much higher than the ALA conversion rate (6%) when linseed was fed as feed.27 The highest conversion rate was achieved in the 1.5% supplementation group, reaching 37%, which is slightly higher than that of microalgae (35%) but less than that when fish oil was given (55%).28 However, the cost of supplementary M. alpina CCFM 698 production is lower than that of fish oil.24 The highest DHA production per egg in the 1.5% supplementation group was 130 mg, nearly double that of the control group and also much higher than that of our previous study using M. alpina CCFM 442 (50 mg DHA per egg).25
Effects of high dose supplementation of M. alpina CCFM 698 on egg yolk fatty acid composition
To further increase DHA content in the egg yolks, higher supplemental feeding amounts of M. alpina CCFM 698 (2% and 4%) were given (Fig. 2). At the same time, to investigate whether M. alpina CCFM 698 is suitable for different laying hens, we selected two kinds of laying hens (Hy-Line Brown and Fengda-1) for the high dose feeding experiments. As shown in Fig. 3, the DHA content of egg yolk plateaued. The DHA content of all groups except the 2% supplementation group of Fengda-1 laying hens reached a peak on the 10th day, indicating that a further increase in feeding time does not increase the DHA content in egg yolk. The fatty acid composition when DHA content reached the highest value in each group is shown in Table 5. The highest DHA content when feed were supplemented at 2% and 4% was similar in Hy-Line Brown laying hens, reaching 7.57 and 7.54 mg g−1 egg yolk, respectively. In Fengda-1 laying hens, the highest DHA content with 2% supplement was just 6.41 mg g−1 egg yolk, significantly lower than that of Hy-Line Brown laying hens, indicating that the DHA accumulation capacity of the Fengda-1 laying hens with 2% supplement was a little weaker than that of Hy-Line Brown laying hens. However, when the does was increased to 4%, the highest DHA content was up to 7.61 mg g−1 egg yolk in Fengda-1 laying hens, similar to that in the Hy-Line Brown laying hens. In addition, the ratio of ω-6/ω-3 PUFAs decreased with the increase of supplement does, both in Hy-Line Brown laying hens and Fengda-1 laying hens. In addition to supplementing feed with M. alpina, other additives in laying hens' feed, such as fish oil, flaxseed, chia seed and microalgae can also enhance the DHA content in egg yolks.14,29,30 Thus, various sources of ω-3 PUFAs have great potential to produce high DHA content and thus more nutritious eggs. However, among these additives, M. alpina has many advantages, such as higher conversion of EPA to DHA, an advanced fermentation process, and lower cost (Table 6).25,31,32
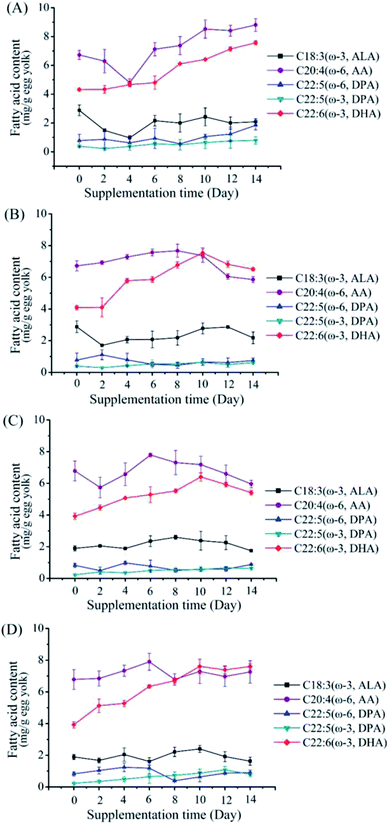 |
| Fig. 3 Changes of fatty acid composition in egg yolk of different groups in the supplementation period of feeding with mycelium-enriched feed. (A) 2% supplementation group in Hy-Line Brown laying hens, (B) 4% supplementation group in Hy-Line Brown laying hens, (C) 2% supplementation group in Fengda-1 laying hens, (D) 4% supplementation group in Fengda-1 laying hens. Values are mean of three samples ± standard error of the mean. | |
Table 5 Egg yolk fatty acid content (mg per g egg yolk) of high dose groups when DHA content reaching the highest valuea
Fatty acids |
Hy-Line Brown laying hens (control 0%) |
Hy-Line Brown laying hens (2%) |
Hy-Line Brown laying hens (4%) |
Fengda-1 laying hens (control 0%) |
Fengda-1 laying hens (2%) |
Fengda-1 laying hens (4%) |
Values are mean of three samples ± standard error of the mean. Values with the same letter in the same row are not significantly. |
C16:0 |
41.69 ± 5.33a |
56.58 ± 0.31b |
39.36 ± 2.92a |
42.62 ± 1.27a |
40.13 ± 2.75a |
40.86 ± 5.10a |
C16:1 |
13.99 ± 2.03ab |
16.93 ± 0.54b |
11.90 ± 1.79a |
14.28 ± 0.35ab |
12.05 ± 1.61a |
10.57 ± 1.33a |
C18:0 |
22.27 ± 1.14a |
28.48 ± 2.12ab |
23.67 ± 2.09a |
24.50 ± 0.57a |
23.93 ± 1.60a |
24.07 ± 2.09a |
C18:1(ω-9, OA) |
68.76 ± 4.15a |
88.17 ± 8.43a |
74.21 ± 4.06a |
71.15 ± 6.22a |
73.30 ± 4.70a |
75.54 ± 11.29a |
C18:2(ω-6, LA) |
31.55 ± 1.53a |
35.66 ± 5.88a |
29.66 ± 2.67a |
27.27 ± 0.29a |
31.49 ± 3.37a |
33.71 ± 4.07a |
C18:3(ω-6, GLA) |
0.51 ± 0.01a |
0.54 ± 0.03a |
0.43 ± 0.05a |
0.52 ± 0.19a |
0.52 ± 0.15a |
0.47 ± 0.12a |
C18:3(ω-3, ALA) |
2.24 ± 0.39a |
2.09 ± 0.20a |
2.77 ± 0.74a |
1.77 ± 0.33a |
2.38 ± 0.59a |
2.40 ± 0.23a |
C20:3(ω-6, DGLA) |
0.56 ± 0.02ab |
0.46 ± 0.08a |
0.54 ± 0.02ab |
0.61 ± 0.06ab |
0.67 ± 0.11b |
0.59 ± 0.07ab |
C20:4(ω-6, AA) |
7.07 ± 0.27a |
8.81 ± 0.44b |
7.37 ± 0.42a |
8.02 ± 0.35ab |
7.18 ± 0.54a |
7.29 ± 0.76a |
C22:5(ω-6, DPA) |
1.27 ± 0.92ab |
1.84 ± 0.32b |
0.65 ± 0.21a |
1.05 ± 0.28ab |
0.58 ± 0.13a |
0.63 ± 0.32a |
C22:5(ω-3, DPA) |
0.38 ± 0.03a |
0.79 ± 0.25c |
0.63 ± 0.16b |
0.25 ± 0.01a |
0.55 ± 0.07ab |
0.87 ± 0.26c |
C22:6(ω-3, DHA) |
4.09 ± 0.13a |
7.57 ± 0.14c |
7.54 ± 0.33c |
3.88 ± 0.22a |
6.41 ± 0.26b |
7.61 ± 0.45c |
Total ω-6 PUFAs |
40.95 ± 2.19a |
47.56 ± 6.85a |
38.65 ± 3.21a |
37.47 ± 0.59a |
40.45 ± 3.04a |
42.69 ± 5.63a |
Total ω-3 PUFAs |
6.57 ± 0.19a |
10.45 ± 0.59c |
10.95 ± 0.68c |
5.90 ± 0.54a |
9.34 ± 0.36b |
10.88 ± 0.33c |
ω-6/ω-3 PUFAs |
6.23 ± 0.15c |
4.52 ± 0.46ab |
3.53 ± 0.01a |
6.37 ± 0.49c |
4.33 ± 0.33ab |
3.93 ± 0.58a |
Table 6 Performance, egg quality, and conversion rate of EPA to DHA in laying hens of high dose groupsa
|
Hy-Line Brown laying hens (control 0%) |
Hy-Line Brown laying hens (2%) |
Hy-Line Brown laying hens (4%) |
Fengda-1 laying hens (control 0%) |
Fengda-1 laying hens (2%) |
Fengda-1 laying hens (4%) |
Values are mean of six samples ± standard error of the mean. Values with the same letter in the same row are not significantly. Feed conversion (%) = (laying rate × egg weight)/feed intake. EPA conversion (%) = (experimental group egg yolk DHA production—control group egg yolk DHA production)/EPA intake. |
Laying rate (%) |
86.11 ± 5.36a |
88.45 ± 6.55a |
87.43 ± 7.12a |
88.23 ± 4.86a |
87.64 ± 5.56a |
88.04 ± 6.96a |
Feed intake (g) |
118.25 ± 5.12a |
119.32 ± 4.23a |
117.59 ± 6.54a |
89.52 ± 4.43b |
90.02 ± 3.42b |
90.24 ± 6.22b |
Egg weight (g) |
67.88 ± 1.33a |
65.17 ± 1.62a |
66.88 ± 1.68a |
50.09 ± 1.12b |
51.26 ± 1.44b |
51.87 ± 1.88b |
Egg yolk (g) |
17.42 ± 0.88a |
17.40 ± 0.76a |
17.50 ± 0.92a |
15.03 ± 0.72b |
15.42 ± 0.54b |
15.56 ± 0.68b |
Egg albumen (g) |
44.69 ± 1.32a |
42.18 ± 1.66a |
43.54 ± 2.45a |
30.61 ± 1.61b |
31.32 ± 1.22b |
31.66 ± 1.14b |
Egg shell (g) |
5.77 ± 0.52a |
5.58 ± 0.49a |
5.84 ± 0.31a |
4.45 ± 0.30b |
4.52 ± 0.45b |
4.65 ± 0.35b |
Feed conversion (%) |
49.43 ± 2.32a |
48.31 ± 3.55a |
49.73 ± 3.87a |
50.26 ± 2.64a |
46.79 ± 3.72a |
48.26 ± 4.23a |
EPA intake (mg) |
0.00a |
220.42 ± 6.45b |
440.84 ± 9.21c |
0.00a |
166.87 ± 5.45d |
333.73 ± 8.44e |
DHA per egg (mg) |
71.24 ± 2.35a |
131.72 ± 3.58b |
131.95 ± 5.66b |
58.32 ± 2.12c |
98.84 ± 3.78d |
118.46 ± 4.35e |
EPA conversion (%) |
0.00a |
27.44 ± 2.64b |
13.77 ± 3.04c |
0.00a |
24.28 ± 3.15b |
18.02 ± 2.02d |
Conclusion
In the present study, we selected a high EPA content (30% of total fatty acids) strain of M. alpina CCFM 698 that overexpressed an ω-3 fatty acid desaturase from P. parasitica and investigated the cell growth and lipid accumulation of this strain in a 65 L airlift fermenter with glucose batch feeding. The maximum cell dry weight was 28.7 g L−1 and the highest total fatty acid content was 33.0% (w/w) in cell dry weight. The highest EPA yield was 1.8 g L−1. In addition, the strains were added to laying hens' feed for egg yolk DHA accumulation. Low or high dose supplementation of M. alpina CCFM 698 both increased DHA accumulation in egg yolks in Hy-Line Brown laying hens and Fengda-1 laying hens. The highest DHA content of 7.61 mg g−1 egg yolk was achieved in Fengda-1 laying hens with 4% strain supplementation. The lowest ratio of ω-6/ω-3 PUFAs (3.53) was obtained in Hy-Line Brown laying hens with 4% strain supplementation. These results show that M. alpina CCFM 698 can be used as an alternative source of ω-3 PUFAs in laying hen feed to produce nutritious high DHA eggs.
Author's contributions
X. T., C. G., S. D., S. S. and J. L. carried out the experiments and drafted the manuscript. X. T., H. C. and Z. G. analyzed the data and revised the manuscript. H. C., H. Z., Y. Q. C. and W. C. conceived and designed the study. All authors read and approved the final manuscript.
Conflicts of interest
The authors have no conflict of interest.
Acknowledgements
This research was supported by the National Natural Science Foundation of China (31722041, 31530056), the Fundamental Research Funds for the Central Universities (JUSRP51702A), the Natural Science Foundation of Jiangsu Province (BK20160172), the Project funded by China Postdoctoral Science Foundation (2017M611701), the Postdoctoral Science Foundation of Jiangsu Province (1701061C), and the Jiangsu Province “Collaborative Innovation Center for Food Safety and Quality Control”.
References
- U. Das, Prostaglandins, Leukotrienes Essent. Fatty Acids, 2002, 67, 1–12 CrossRef CAS.
- J. Hirahashi, K. Kawahata, M. Arita, R. Iwamoto, K. Hishikawa, M. Honda, Y. Hamasaki, M. Tanaka, K. Okubo and M. Kurosawa, Sci. Rep., 2014, 4 CAS.
- H. K. Maehre, I. J. Jensen, E. O. Elvevoll and K. E. Eilertsen, Int. J. Mol. Sci., 2015, 16, 22636–22661 CrossRef CAS PubMed.
- D. B. Jump, C. M. Depner, S. Tripathy and K. A. Lytle, Adv. Nutr., 2015, 6, 694–702 CrossRef CAS PubMed.
- C. Y. Chen, Y. C. Chen, H. C. Huang, S. H. Ho and J. S. Chang, Bioresour. Technol., 2015, 191, 407–413 CrossRef CAS PubMed.
- T. Okuda, A. Ando, H. Negoro, T. Muratsubaki, H. Kikukawa, T. Sakamoto, E. Sakuradani, S. Shimizu and J. Ogawa, Eur. J. Lipid Sci. Technol., 2015, 117, 1919–1927 CrossRef CAS.
- E. Sakuradani, A. Ando, S. Shimizu and J. Ogawa, J. Biosci. Bioeng., 2013, 116, 417–422 CrossRef CAS PubMed.
- C. Ge, H. Chen, T. Mei, X. Tang, L. Chang, Z. Gu, H. Zhang, W. Chen and Y. Q. Chen, Front. Bioeng. Biotechnol., 2017, 5, 89 CrossRef PubMed.
- A. Ando, Y. Sumida, H. Negoro, D. A. Suroto, J. Ogawa, E. Sakuradani and S. Shimizu, Appl. Environ. Microbiol., 2009, 75, 5529–5535 CrossRef CAS PubMed.
- T. Okuda, A. Ando, H. Negoro, T. Muratsubaki, H. Kikukawa, T. Sakamoto, E. Sakuradani, S. Shimizu and J. Ogawa, Eur. J. Lipid Sci. Technol., 2015, 117, 1919–1927 CrossRef CAS.
- S. Mattioli, A. Dal Bosco, M. Martino, S. Ruggeri, O. Marconi, V. Sileoni, B. Falcinelli, C. Castellini and P. Benincasa, J. Funct. Foods, 2016, 22, 454–462 CrossRef CAS.
- M. Petrovic, M. Gacic, V. Karacic, Z. Gottstein, H. Mazija and H. Medic, Food Chem., 2012, 135, 1563–1568 CrossRef CAS PubMed.
- A. A. Saleh, Emir. J. Food. Agric., 2013, 25, 605–612 CrossRef.
- S. Fredriksson, K. Elwinger and J. Pickova, Food Chem., 2006, 99, 530–537 CrossRef CAS.
- P. Dai, H. Chen, B. Yang, H. Wang, Q. Yang, H. Zhang, W. Chen and Y. Q. Chen, RSC Adv., 2016, 6, 1694–1699 RSC.
- H. Chen, G. Hao, L. Wang, H. Wang, Z. Gu, L. Liu, H. Zhang, W. Chen and Y. Q. Chen, Sci. Rep., 2015, 5 Search PubMed.
- L. Wang, W. Chen, Y. Feng, Y. Ren, Z. Gu, H. Chen, H. Wang, M. J. Thomas, B. Zhang, I. M. Berquin, Y. Li, J. Wu, H. Zhang, Y. Song, X. Liu, J. S. Norris, S. Wang, P. Du, J. Shen, N. Wang, Y. Yang, W. Wang, L. Feng, C. Ratledge, H. Zhang and Y. Q. Chen, PLoS One, 2011, 6, e28319 CrossRef CAS PubMed.
- G. Hao, H. Chen, Z. Gu, H. Zhang, W. Chen and Y. Q. Chen, Appl. Environ. Microbiol., 2016 DOI:10.1128/AEM.00572-00516.
- J. F. d. M. Burkert, R. R. Maldonado, F. M. Filho and M. I. Rodrigues, J. Appl. Chem. Biotechnol., 2005, 80, 61–67 CrossRef.
- X. Li, Y. Lin, M. Chang, Q. Jin and X. Wang, Bioresour. Technol., 2015, 181, 275–282 CrossRef CAS PubMed.
- X.-J. Ji, A.-H. Zhang, Z.-K. Nie, W.-J. Wu, L.-J. Ren and H. Huang, Bioresour. Technol., 2014, 170, 356–360 CrossRef CAS PubMed.
- H. Shi, H. Chen, Z. Gu, H. Zhang, W. Chen and Y. Q. Chen, Microb. Cell Fact., 2016, 15, 117 CrossRef PubMed.
- Z. Xue, P. L. Sharpe, S. P. Hong, N. S. Yadav, D. Xie, D. R. Short, H. G. Damude, R. A. Rupert, J. E. Seip and J. Wang, Nat. Biotechnol., 2013, 31, 734–740 CrossRef CAS PubMed.
- I. Fraeye, C. Bruneel, C. Lemahieu, J. Buyse, K. Muylaert and I. Foubert, Food Res. Int., 2012, 48, 961–969 CrossRef CAS.
- P. Dai, H. Chen, B. Yang, H. Wang, Q. Yang, H. Zhang, W. Chen and Y. Q. Chen, RSC Adv., 2016, 6, 1694–1699 RSC.
- A. P. Simopoulos, Biomed. Pharmacother., 2002, 56, 365–379 CrossRef CAS.
- C. Lemahieu, C. Bruneel, E. Ryckebosch, K. Muylaert, J. Buyse and I. Foubert, J. Funct. Foods, 2015, 19, 821–827 CrossRef CAS.
- C. Lemahieu, C. Bruneel, R. Termote-Verhalle, K. Muylaert, J. Buyse and I. Foubert, Algal Res., 2014, 6, 119–123 CrossRef.
- M. Kaewsutas, A. Sarikaphuti, T. Nararatwanchai, P. Sittiprapaporn and P. Patchanee, J. Funct. Foods, 2017, 29, 46–52 CrossRef CAS.
- R. Coorey, A. Novinda, H. Williams and V. Jayasena, J. Food Sci., 2015, 80, S180–S187 CrossRef CAS PubMed.
- W. J. Wu, A.-H. Zhang, C. Peng, L.-J. Ren, P. Song, Y. D. Yu, H. Huang and X. J. Ji, Bioresour. Bioprocess., 2017, 4, 8 CrossRef PubMed.
- H. Kikukawa, E. Sakuradani, A. Ando, S. Shimizu and J. Ogawa, J. Adv. Res., 2018 Search PubMed.
|
This journal is © The Royal Society of Chemistry 2018 |